ABSTRACT
Background
The biological compounds known as peptides are found in nature. They are peptide (amide) links that connect short chains of amino acid monomers. Nowadays, peptide-based therapeutic treatments are employed because of their intriguing and prospective uses in drug design. These applications include minimal cytotoxicity, high specificity, tumor-penetrating ability, small size, and ease of modification. In this article, we examine a number of investigations into diverse Antimicrobial Peptides (AMPs) that have cytotoxic properties against cancer cells. The pharmacological analysis and synthesis of extremely short dipeptides form the foundation of the current research.
Materials and Methods
Using microwave-assisted solid phase peptide synthesis, six extremely short dipeptides (2a-f) were created, and their in vitro antibacterial and anticancer activity was assessed. Using the agar dilution technique, the antibacterial activity of produced dipeptides was evaluated against three fungi, two gram-positive and two gram-negative bacterial strains. Spectroscopy techniques such as Proton NMR, Carbon NMR, HPLC, and LC-MS were used to characterise the synthesised dipeptides. The MTT assay was used to test the anticancer activity of produced dipeptides against the HeLa and MCF-7 cell lines.
Results
The antibacterial activity of peptides 2a and 2d was shown to be superior to that of other synthetic peptides. With a MIC value of around 5-50 μg/mL, peptide 2d was shown to have strong antibacterial activity against Pseudomonas aeruginosa, Escherichia coli, Staphylococcus pyogenes, Staphylococcus aureus. With MFC in the range of 200-250 μg/mL, peptides 2b, 2c, and 2e demonstrated strong antifungal activity against Candida albicans.
Conclusion
According to the results of the present study, extremely short dipeptides that have been created are efficient in fighting cancer and bacteria while also being safe, affordable, and less harmful.
INTRODUCTION
Millions of deaths each time throughout the world caused by cancer. Conventional chemotherapy is still the most common treatment for cancer, although it is ineffective owing to side effects on healthy cells and the development of multidrug resistance in malignant cells. This dire scenario emphasizes the critical need for new treatment approaches to combat this lethal illness.1 Natural Antimicrobial Peptides (AMPs) have shown promising effect as chemotherapeutic agent against various microbes.2 Property of cancer cells when compared with microbial cells have shown enormous resembles which led to idea of developing Anticancer Peptides (ACPs) from Antimicrobial Peptides (AMPs).3 Also, the rise in the cases of antibiotic-resistant microbes has triggered the research and interest in novel ultrashort therapeutics which also include ultrashort peptides and thus can lead to effective management of the antibiotic-resistant microbes.4 Overuse and misuse of antibiotics have not only caused antimicrobial resistance but also, slow pace in the development of novel antibiotics.5 Since there is no major class of antibiotics, which has been discovered in recent times, the situation is far more substandard for gram-negative microbes where no more antibiotic drugs have been approved in over 50 years.6,7 AMPs are very promising and novel contenders for a new class of antibiotics. AMPs are active against various strains (such as gram-positive and gram-negative bacteria, fungi and virus) through various mechanisms of action such as membrane disruption, intra-cellular penetration, and immunomodulation.8 Usually, cationic peptides are more effective against the microbes, since they carry a positive charge, and they can interact with the membrane of the microbes9 also, some parts of the peptides are hydrophobic which results in the formation of amphipathic secondary and tertiary structures. Natural AMPs are very bulky in nature, restricting their bioavailability. These peptides are prone to hydrolysis from different peptidases.10 Thus, generation of short peptides came into focus. The idea is to generate different short chains of peptides instead of one long chain to enhance their selectivity and bioavailability.11 Grounded on the below- mentioned observation we envisaged using Fmoc defended rink amide4- Methyl Benzhydryl Amine (MBHA) resin and lipophilic tryptophan as core amino acid to develop a new structural class of anticancer and antimicrobial rectifiers. therefore, for the purpose of exploiting the remedial eventuality of ultra-short peptides we’ve designed dipeptide series using Fmoc defended rink amide MBHA resin.12 Fmoc protected amino acids were reacted with Hydroxy Benzotriazole (HOBt) and 2 (1H- Benzotriazole-1-yl) tetra methyl aminium Tetrafluoroborate (TBTU) to synthesize different extremely short dipeptides.13
MATERIALS AND METHODS
Experimental Section
Chemistry
All reagents were available from marketable suppliers and used without any sanctification unless else noted. Amino acids, detergents, coupling reagents and base, were bought from marketable sources similar as Alfa-aesar, Sigma-Aldrich, Merch, Avra, Chem-impex and TCI. Gallenkamp apparatus were used to determined melting point. BrukerAvance-III 400 spectrometer were used to record proton and carbon -13 spectra, 1H NMR (400 MHz), 13C NMR (100 MHz) respectively. For NMR spectra DMSO-d6 were used as a solvent. Processing of the spectra was performed with Topspin software. δ values were used for chemical shift of 1H NMR and coupling constant were used in Hertz (Hz). Ppm values were used for chemical shifts of 13C NMR which is relative to the solvent peak. Peptide synthesis was performed on Discover CEM microwave assisted peptide synthesizer with the help of Fmoc synthesis method. Shimadzu LC-MS mass spectrometer were used for mass spectra by using ESI method. Shimadzu SPD-M20A analytical HPLC system were used to analysed the peptides. For this purpose, a Kromasil-C18, 5 µm (4.6 x 250 mm ID) column were used. The Shimadzu HPLC preparative system by using a Luna 10 µM C18 (250 mm x 21.2, 10µm) column were used. Standard antibiotics such as gentamycin, amikacin, chloramphenicol, ciprofloxacin, norfloxacin, nystatin, and griseofulvin drugs were purchased from Sigma-Aldrich.
Rink amide MBHA resin coupling (1) with Fmoc protected tryptophan to furnish Fmoc-NH- tryptophan MBHA resin (2)
Fmoc-MBHA resin (300 mg; 0.195 mmol) was first deprotected with 7.5 mL DMF in 20% piperidine at 60°C, 35 Watt (W) in microwave (MW) for 15 min and followed by coupling with Fmoc-Trp-OH (333 mg; 4 eq) through TBTU (250 mg; 4 eq), HOBt (105 mg; 4 eq), and DIPEA (169 µL; 5 eq) in 10 mL DMF at 50W, 60°C in MW for 3 hr to synthesized Fmoc-NH-tryptophan MBHA resin (2). Reaction monitoring was performed by Reverse Phase Thin Layer Chromatography (RPTHC) and coupling steps were monitored by *Kaiser test.
Synthesis of dipeptides (2a-2f) from Fmoc-NH- tryptophan MBHA resin (2)
Fmoc-NH-tryptophan MBHA resin (2) was subjected to Fmoc deprotection with 7.5 mL DMF in 20% piperidine at 60°C, 35 W in MW and appropriate Fmoc protected amino acids were coupled with Fmoc-Arg(pbf)-OH (506 mg; 4 eq)/Fmoc-Lys(mtt)-OH (488 mg; 4 eq)/Fmoc-His(trt)-OH (485 mg; 4 eq)/Fmoc-Ala-OH (243 mg; 4 eq)/Fmoc-Leu-OH (276 mg; 4 eq)/Fmoc-Ile-OH (276 mg; 4 eq) in the presence of TBTU (251 mg; 4 eq), HOBt (106 mg; 4 eq) in 10 mL DMF and DIPEA 169 µL at 50 W, 60°C in MW for 3 hr and other protecting groups (fmoc, pbf, mtt, trt) and resin were cleaved by 9.5 mL TFA, 0.25 mL TIPS and 0.25 mL water to synthesize dipeptides 2a-f. Reaction monitoring was performed by RPTHC and coupling steps were monitored by Kaiser test. Synthesized peptides were characterized by using proton NMR, carbon-13 NMR, HPLC and LC-MS spectroscopy. Scheme for the synthesis of dipeptides by using microwave assisted solid phase peptide synthesis protocol were shown in Figure 2 and the structures of synthesized dipeptides were shown in Figure 3.

Figure 1:
Representative structure of designed dipeptides
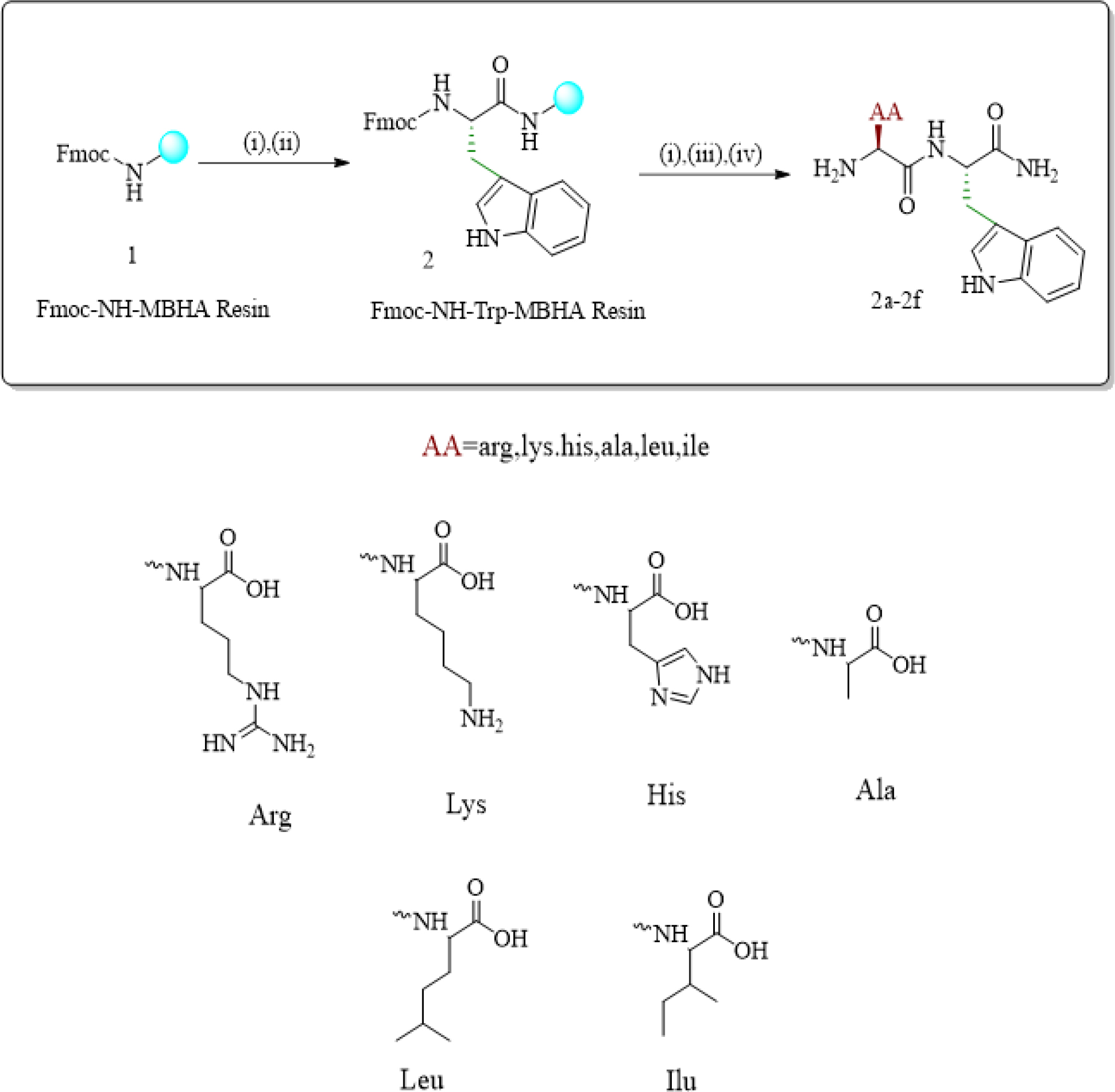
Figure 2:
Scheme for synthesis of dipeptides using microwave assisted solid phase peptide synthesis protocol.
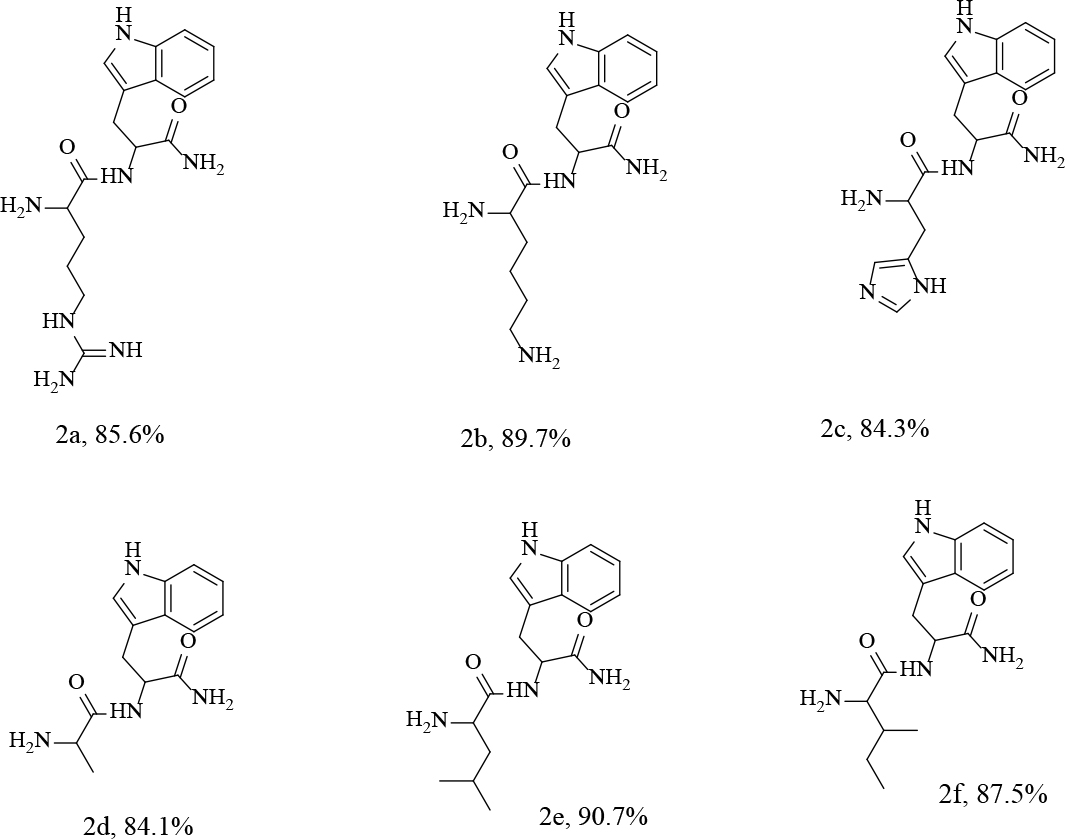
Figure 3:
Structures of the synthesized dipeptides (2a-f).
Reaction conditions: (i) 7.5 mL DMF with 20% piperidine, at 60°C, 35W in MW for 15 min; ii) Fmoc-Trp-OH (333 mg; 4 eq), TBTU (251 mg; 4 eq), HOBt (106 mg; 4 eq), DIPEA (169 µL; 5 eq), in 10 mL DMF, 60°C,50W in MW for 3hr; (iii) TBTU (251 mg; 4 equiv), HOBt (106 mg; 4 eq), Fmoc-Arg(pbf)-OH (506 mg; 4 eq)/ Fmoc-Lys (mtt)-OH (488mg: 4 eq)/ Fmoc-His(trt)-OH (485 mg; 4 eq)/ Fmoc-Ala-OH (243mg; 4 eq)/ Fmoc-Leu-OH (276mg: 4 eq)/Fmoc-Ile-OH (276mg; 4 eq) DIPEA (169µL; 5 eq), 60°C, 50W in MW for 3 hr (iv) TFA: TIPS: water (10mL; 95:2.5:2.5), 3hr.
2-amino-N-(1-amino-3-(1H-indol-3-yl)-1-oxop ropan-2-yl)-5 guanidinopentanamide (2a)
Yield = 85.6%; White solid, m.p. 205-207°C; 1H NMR (400 MHz, DMSO): δ 10.93 (s, 1H, Ar-NH), 8.67 (d, J = 7.7 Hz, 2H, NH2), 8.18 (s, 1H, NH), 7.88 (t, J = 5.7 Hz, 1H, ArH), 7.68 (d, J = 7.24 Hz,1H, ArH) 7.33 (d, J = 7.1 Hz, 1H, ArH), 7.02-7.07 (m, 2H, CONH2), 7.03 (t, J = 17.1 Hz, 1H, ArH), 6.98 (t, J = 6.9 Hz, 1H, ArH), 4.55 (q, J = 8.0 Hz, 3H, NH2, aliphatic H), 3.82 (s, 1H, aliphatic H) 3.17-3.08 (m, 2H, aliphatic H) 3.03-2.97 (m, 2H, aliphatic H), 1.77–1.71 (m, 2H, aliphatic H), 1.57-1.53 (m, 2H, aliphatic H); 13C NMR (100 MHz, DMSO): δ 173.4, 168.8, 157.3, 136.5, 127.7, 124.3, 121.3, 119.0, 118.7, 111.7, 110.1, 54.0, 52.1, 28.9, 28.3, 24.3; LCMS (ESI); m/z [(M+H)+] calculated for C17H25N7O2 360.21, found 360.10; HPLC: tR= 9.8 min, 92.3% purity.
2,6- diamino-N-(1-amino-3-(1H-indol-3-yl)-1-oxop ropan-2-yl) hexanamide (2b)
Yield = 89.7%; Pale yellow solid, m.p. 202-204°C; 1H NMR (400 MHz, DMSO): δ 10.96 (s, 1H, Ar-NH), 8.72 (d, J = 7.8Hz, 1H, NH), 8.22 (s, 1H, ArH),7.99 (s, 1H, ArH), 7.69-7.67 (m, 1H ArH),7.367.09 (m, 2H, CONH2), 7.04 (t, J = 16.5 Hz, 1H, ArH), 6.98 (t, J = 7.0 Hz, 1H, ArH), 4.55 (q, J = 7.92 Hz, 5H, NH2, aliphaticH), 3.803.79 (m, 1H, aliphaticH), 3.183.13(m, 1H, aliphaticH), 3.022.99 (m, 1H,aliphatic H), 2.782.73 (m, 2H, aliphatic H), 1.751.72 (m,2H, aliphatic H), 1.571.54 (m, 2H, aliphatic H), 1.381.34 (m, 2H, aliphatic H); 13C NMR (10MHz, DMSO): δ 173.5, 168.9, 136.5, 127.7, 123.4, 121.4, 118.9, 111.7, 110.2, 54.1, 52.3, 40.4, 30.9, 28.2, 26.8, 21.3; LCMS (ESI): m/z [(M+H)+] calculated for C18H27N5O2 332.20, found 332.10; HPLC: tR=10.8 min, 94.7% purity.
2aminoN(1amino3(1Hindole3yl)1oxopropan2yl)_ 3(1Himidazol4yl)Propenamaide(2c)
Yield = 84.3%; light brown solid, m.p. 212214°C; 1H NMR (400 MHz, DMSO): δ 10.97 (s, 1H, ArNH), 9.06 (s, 1H, ArH), 8.76 (d, J = 7.1 Hz, 1H, NH), 8.35 (s, 1H, ArH) 7.93 (s, 1H, ArH), 7.71 (d, J = 7.8 Hz, 1H, ArH), 7.46 (s, 1H, ArH), 7.367.08 (m, 2H, CONH2), 7.04 (t, J= 16.4 Hz, 1H, ArH), 6.99 (t, J = 7.0 Hz, 1H, ArH), 4.59-4.54 (m, 3H, NH2, aliphatic H);13C NMR (100 MHz, DMSO): δ 174.1, 167.8, 136.5, 134.9, 127.6, 124.3, 121.4, 119, 118.7, 118.5, 111.8, 109.9, 54.2, 51.5, 28.8, 26.8; LCMS (ESI): m/z [(M+H)+] calculated for C17H20N6O2 341.17, found 341.05; HPLC: tR = 10.7 min, 95.5% purity.
2-amino-N-(1-amino-3-(1H-indole-3-yl)-1-oxo porpan-2-yl)propenamide(2d)
Yield = 84.1%; light brown solid, m.p. 195197°C; 1H NMR (400 MHz, DMSO):δ 10.91 (s, 1H, ArNH), 8.59 (d, J = 8.0 Hz, 1H, NH), 8.08 (s, 1H, ArH), 7.697.62 (m, 1H, ArH), 7.34 (d, J = 8.0 Hz, 1H, ArH), 7.187.07 (m, 2H, CONH2), 7.03 (t, J = 16.0 Hz, 1H, ArH), 6.98 (t, J= 6.9 Hz, 1H, ArH), 4.564.50 (m, 3H, NH2, aliphatic H), 3.833.81 (m, 1H, aliphatic H) 3.173.12 (m, 1H, aliphatic H), 3.012.95 (m, 1H, aliphatic H), 1.34 (d, J = 6.9Hz, 3H, CH3); 13C NMR (100 MHz, DMSO): δ 173.3, 169.7, 127.7, 124.2, 121.3, 119.1, 118.7, 111.7, 110.2, 54.0, 48.4, 28.3, 17.7; LCMS (ESI): m/z [(M+H)+] calculated for C14H18N4O2 275.15, found 275.85; HPLC: tR = 17.7 min, 95.3% purity.
2-amino-N-(1-amino-3-(1H-indole-3-yl)-1-oxoprop an-2-yl)-4-methtylpentanamaide (2e)
Yield = 90.7%; white solid, m.p. 223-225°C 1H NMR (400MHz, DMSO):δ 10.91 (s, 1H, Ar-NH), 8.76 (d, J = 8.0 Hz, 1H, NH), 8.14 (s, 1H, ArH), 7.66 (d, J = 7.8 Hz, 1H, ArH), 7.59 (s, 1H, ArH), 7.357.07 (m, 2H, CONH2), 7.057.01 (m, 1H, ArH), 6.996.97 (m, 1H, ArH), 4.55 (q, J = 7.92Hz, 3H, NH2, aliphatic H), 3.833.71 (m, 1H, aliphatic H), 3.163.11 (m, 1H, aliphatic), 3.042.98 (m, 1H, aliphatic H), 1.671.60 (m, 1H, aliphatic H), 1.581.51 (m, 2H, aliphatic H), 0.89 (t, J = 6.3Hz, 6H, CH3); 13C NMR (100 MHz, DMSO): δ 173.2, 172.4, 136.5, 127.7, 124.1, 121.3, 118.9, 118.6, 111.7, 110.2, 54, 51.1, 40.7, 28.3, 23.8, 22.1, 21.5; LCMS (ESI): m/z [(M+H)+] calculated for C17H24N4O2 317.19 found 317.15; HPLC: tR = 10.1 min, 93.5% purity.
2- amino-N-(1-amino-3-(1H-indole-3-yl)-1-oxo propan-2-yl)3—methylpenatanamide(2f)
Yield = 87.5%; White solid, m.p. 234-236°C; 1H NMR (400 MHz, DMSO); 10.90 (s, 1H,Ar-NH), 8.60 (d, J =7.9 Hz, 1H, NH), 8.09 (s, 1H, ArH), 7.66 (d, J = 7.8 Hz, 1H, ArH), 7.59 (s, 1H, ArH), 7.357.19 (m, 2H, CONH2), 7.097.03 (m, 1H, ArH), 6.99 (t, J = 5.7 Hz, 1H, ArH), 4.58 (q, J = 7.8 Hz, 3H, NH2, aliphatic H), 3.733.67 (m, 1H, aliphatic H), 3.163.11 (m,2H, aliphatic H), 3.032.97 (m, 1H, aliphatic H), 1.891.82 (m, 1H, aliphatic H), 1.511.45 (m, 1H, aliphatic H), 1.151.07 (m, 1H, aliphatic H), 0.92 (d, J = 6.8 Hz, 3H, CH3), 0.85 (t, J = 7.4 Hz, 3H, CH3); 13C NMR (100 MHz, DMSO) δ 173.2, 168.2, 136.5, 127.7, 124.2, 121.3, 118.9, 118.6, 111.7, 110.1, 56.9, 53.8, 39.7, 28.3, 24, 15, 11.6; LCMS (ESI): m/z[(M+H)+] calculated for C17H24N4O2 317.19 found 317.10; HPLC: tR = 12.1 min, 95.3% purity.
Biological Evaluation
Antibacterial evaluation (in vitro)
By using agar dilution method, all six dipeptides were screened for in vitro antibacterial activity. For antibacterial screening, reference standard drugs i.e. chloramphenicol, ciprofloxacin, gentamycin, norfloxacin and amikacin were used against two gram positive bacterial strains Streptococcus pyrogenus (MTCC 442) and Strephylococcus aureus (MTCC 96) and two gram negative bacterial strains Pseudomonas aeruginosa (MTCC 1688) and Escherichia coli (MTCC 443).
In the synthesized library compound with Trp-Arg and Trp-Ala were found to show most of activities. Peptides leads with Arg, mainly shown activity as antibacterial agent against E. coli and P. aerginosa. Arg with Trp have activity against E. coli and P. aerginosa. Compound 2a and 2d were found to have good antibacterial activity against E. coli strain with MIC = 10-50 µg/mL with comparison of chloramphenicol and ciprofloxacin as a reference drug, also the same peptides (2a and 2d) were found to have better antibacterial activity against P. aeruginosa with MIC = 525 µg/mL with the comparison of chloramphenicol, ciprofloxacin and norfloxacin, whereas peptide 2d was found to have moderate antibacterial activity against S. pyogenus and S. aureus with the comparison of chloramphenicol and ciprofloxacin and the MIC value of the compound was shown against these stains, were found to be 12.66 ± 0.28 µg/mL and 51.00 ± 1.73 µg/mL respectively. Ala having CH3 group favours activity of bacterial strains i.e. Eschirichia coli, Pseudomonas aeruginosa, Streptococcus pyrogenus, Staphylococcus aureus. Also, Arg has more cationic sides (NH2) favours antibacterial activity against gram negative bacterial strains i.e. Pseudomonas aeruginosa and Escherichia coli.
Antifungal evaluation (in vitro)
Agar dilution method was used in the screening of all six dipeptides for in vitro antifungal evaluation. For antifungal activity, reference standard drugs griseofulvin and nystatin were used against three fungal strains i.e. Candida albicans (MTCC 227), Aspergillus niger (MTCC 282) and Aspergillus clavatus (MTCC 1323). Compound 2b, 2c and 2e were found to show antifungal activity against Candida albicans with MFC value 200-250 µg/mL was found to be better than griseofulvin.
In vitro anticancer evaluation
MTT assay was used for anticancer activity of all six dipeptides against HeLa and MCF-7 cell lines. Peptides against HeLa cell lines have good activity whereas peptides against MCF-7 cell lines have fairly low activity.
RESULTS
The physicochemical properties of synthesized dipeptides were shown in Table 1. The synthetic methodology of final dipeptides as illustrated in Figure 2. In Table 2, antibacterial and antifungal activity were shown. The HeLa and MCF-7 cell lines were used for evaluation of anticancer activity of synthesized dipeptides. Doxorubicin was selected as the reference standard drug. The IC50 value of all synthesized dipeptides is presented in Table 3.
Compound Code | Sequence | Molecular Formula | Molecular Weight | Melting Point | Colour | EI-MS (m/z) | HPLC retention time (tR) mins | Percent Purity % |
---|---|---|---|---|---|---|---|---|
2a | RW-CONH2 | C17H25N7O2 | 359.42 | 205-207°C | White solid | 360.10 [M+1]+ | 9.826 | 92.35 |
2b | KW-CONH2 | C18H27N5O2 | 331.41 | 202-204°C | Pale yellow solid | 332.10 [M+1]+ | 10.873 | 94.7 |
2c | HW-CONH2 | C17H20N6O2 | 340.39 | 212-214°C | Light brown solid | 341.05 [M+1]+ | 10.770 | 95.51 |
2d | AW-CONH2 | C14H18N4O2 | 274.31 | 195-197°C | Light brown solid | 274.85 [M+1]+ | 17.768 | 95.3 |
2e | LW-CONH2 | C17H24N4O2 | 316.39 | 223-225°C | White solid | 317.15 [M+1]+ | 10.144 | 93.5 |
2f | IW-CONH2 | C17H24N4O2 | 316.39 | 234-236°C | White solid | 317.10 [M+1]+ | 12.163 | 95.3 |
Physico-chemical properties of synthesized dipeptides.
In vitro antibacterial activity MICa (μg/mL) | In vitro antifungal activity MFCb (μg/mL) | ||||||
---|---|---|---|---|---|---|---|
Compound code | ECc | PAd | SAe | SPf | CAg | ANh | ACi |
2a | 12.66±0.28 | 6.50±0.43 | 101.33±2.30 | 125.66±2.08 | 506.66±11.54 | >1000 | 504.66±8.08 |
2b | 62.50±0.50 | 52.00±2.00 | 102.00±2.00 | 50.66±1.15 | 241.66±14.43 | 507.33±12.70 | >1000 |
2c | 61.66±1.44 | 102.33±4.04 | 116.66±14.43 | 251.33±2.30 | 248.33±2.88 | >1000 | >1000 |
2d | 50.66±1.15 | 22.00±5.19 | 51.00±1.73 | 12.66±0.28 | >1000±17.32 | >1000 | >1000 |
2e | 51.66±1.52 | 126.00±1.73 | 52.00±3.46 | 101.66±2.88 | 247.66±4.04 | >1000 | 498.66±2.30 |
2f | 124.66±0.57 | 250.66±1.15 | 102.33±4.04 | 63.33±1.44 | >1000±13.27 | >1000 | >1000 |
Gentamycin | 0.06±0.01 | 1.06±0.11 | 0.26±0.01 | 0.56±0.11 | – | – | – |
Amikacin | 0.04±0.01 | 1.16±0.28 | 0.29±0.04 | 5.50±0.50 | – | – | – |
Chloramphenicol | 51.66±2.88 | 52.33±2.08 | 51.00±1.00 | 52.33±2.08 | – | – | – |
Ciprofloxacin | 26.33±2.30 | 25.66±1.15 | 51.66±2.88 | 51.33±3.21 | – | – | – |
Norfloxacin | 10.66±1.15 | 10.66±1.15 | 11.33±2.30 | 10.66±2.08 | – | – | – |
Nystatin | – | – | – | – | 102.66±3.05 | 103.33±3.05 | 102.66±2.51 |
Griseofulvin | – | – | – | – | 502.00±2.00 | 104.00±4.58 | 102.00±2.64 |
In vitro
antibacterial and antifungal activities of synthesized dipeptides.
Peptides | Sequence | Compound name | IC50 ± SD (µg/mL) HeLa | IC50 ± SD (µg/mL) MCF-7 |
---|---|---|---|---|
2a | RW-CONH2 | Arg-Trp-CONH2 | 6.12±0.60 | 95.11±3.02 |
2b | KW-CONH2 | Lys-Trp-CONH2 | 17.01±1.98 | 89.38±2.38 |
2c | HW-CONH2 | His-Trp-CONH2 | 31.20±0.80 | 90.14±4.31 |
2d | AW-CONH2 | Ala-Trp-CONH2 | 15.16±0.11 | 86.12±3.22 |
2e | LW-CONH2 | Leu-Trp-CONH2 | 19.21±2.87 | >100 |
2f | IW-CONH2 | Ile-Trp-CONH2 | 25.28±3.90 | >100 |
Drug | Doxorubicin | – | 3.02±0.18 | 0.05±0.006 |
In vitro
anticancer activity of synthesized dipeptides.
All the data were represented as mean ± standard error of the mean. The experiments were performed in triplicate.
Microwave assisted solid phase peptide synthesizer were used to synthesize six dipeptides and also evaluated for their in vitro anticancer and antimicrobial activity. Proton NMR, carbon-13 NMR, LC-MS mass spectroscopy and HPLC were used for characterization of all dipeptides. Antibacterial activity against Escherichia coli strain of compound 2a and 2d were found to be significant with MIC value 10-15 µg/mL and same peptides were found better antibacterial activity against P. aeruginosa with MIC = 5 – 25 µg/mL. Dipeptide 2a has shown moderate activity against HeLa cell line with IC50 = 6.12 ± 0.60 µg/mL.
DISCUSSION
Fmoc protected rink amide MBHA resin were used for the synthesis of ultra short dipeptides. For this tryptophan as core amino acid and with key amino acid variation of histidine, alanine, arginine, lysine, leucine, isoleucine and phenyl alanine as AA (arg, lys, his, ala, leu, ile), (Figure 1). Short dipeptides were prepared by solid phase peptide synthesis method using microwave assisted solid phase peptide synthesizer14 in which resin was used as a solid support. Reaction of peptide synthesis, first Fmoc protected resin (1) was swelled for 10-20 min in Dimethylformamide (DMF) solvent15 than transferred into the vessels. Deprotection of Fmoc group during microwave-assisted solid phase peptide synthesis in preloaded MBHA resin was obtained by 7.5 mL DMF in 20% piperidine for 15 min to get free resin which have free amino terminal. In the presence of DIPEA, TBTU, HOBt and Fmoc-Trp-OH Amino Acid (AA1) in DMF for 3 hr in microwave method was first coupled with the free amino group.16 By using 20% piperidine in 7.5 mL DMF for 15 min the Fmoc group was removed to yield coupling of resin to first AA1.17 The coupling and deprotection cycle was repeated with different Fmoc protected amino acids till the desired sequence have been synthesized. After synthesizing desired dipeptides, the protecting groups and resin were cleaved by TFA, TIPS and water for 3 hr.18 The analytical HPLC were used to check the purity of desired dipeptides. Based on the analytical data, the crude peptide was subjected to purification using preparative HPLC.19 After removing of solvent under reduced pressure, desired fractions of pure dipeptides were obtained. The purity of synthesized dipeptides was performed using analytical HPLC. The characterization of synthesized dipeptides was performed by using proton NMR, carbon-13 NMR Mass Spectroscopy (LC-MS). The percent purity obtained for synthesized peptides was found to be in the range of 92-95%. The purified peptides were stored at -40°C in the deep freezer.
All the synthesized compounds have shown broad range of anticancer activity with IC50 values of (6.12±0.60 µg/mL to more than 100 µg/mL). In vitro antimicrobial studies showed that the most potent compound 2a having MIC value 12.66 ± 0.28 µg/mL against Escherichia coli.
CONCLUSION
Antimicrobial (two gram negative and two gram positive bacterial strains) and antifungal activities of all six synthesized dipeptides were evaluated. In this study HeLa and MCF-7 cell lines were used to evaluate anticancer activity of synthesized dipeptides. Although the peptides gave inhibitory activity against HeLa cell lines. And some peptides gave inhibitory activity against bacterial and fungal cells. Peptides 2a was identified as the most potent compound with MIC value 12.66 ± 0.28 µg/mL against Escherichia coli.
References
- Tornesello AL, Borrelli A, Buonaguro L, Buonaguro FM, Tornesello ML. Antimicrobial peptides as anticancer agents: functional properties and biological activities. Molecules. 2020;25(12):2850 [PubMed] | [CrossRef] | [Google Scholar]
- Giuliani A, Pirri G, Nicoletto S.. Antimicrobial peptides: an overview of a promising class of therapeutics. Open Life Sci. 2007;2(1):1-33. [CrossRef] | [Google Scholar]
- Seyfi R, Kahaki FA, Ebrahimi T, Montazersaheb S, Eyvazi S, Babaeipour V, et al. Antimicrobial Peptides (AMPs): roles, functions and mechanism of action. Int J Pept Res Ther. 2020;26(3):1451-63. [CrossRef] | [Google Scholar]
- Yu WD, Sun G, Li J, Xu J, Wang X.. Mechanisms and therapeutic potentials of cancer immunotherapy in combination with radiotherapy and/or chemotherapy. Cancer Lett. 2019;452:66-70. [CrossRef] | [Google Scholar]
- Kim MK, Kang N, Ko SJ, Park J, Park E, Shin DW, et al. Antibacterial and antibiofilm activity and mode of action of magainin 2 against drug-resistant Acinetobacter baumannii. Int J Mol Sci. 2018;19(10):3401-15. [PubMed] | [CrossRef] | [Google Scholar]
- Smith PA, Koehler MF, Girgis HS, Yan D, Chen Y. Optimized arylomycins are a new class of Gram-negative antibiotics. Nature. 2018;561:189-94. [CrossRef] | [Google Scholar]
- Laxminarayan R, Duse A, Wattal C, Zaidi AK, Wertheim HF, Sumpradit N, et al. Antibiotic resistance-the need for global solutions. Antibiotic resistance—the need for global solutions. Lancet Infect Dis. 2013;13(12):1057-98. [PubMed] | [CrossRef] | [Google Scholar]
- Koo HB, Seo J.. Antimicrobial peptides under clinical investigation. Pept Sci. 2019;111(5):24122 [CrossRef] | [Google Scholar]
- Jakubczyk A, Karaś M, Rybczyńska-Tkaczyk K, Zielińska E, Zieliński D. Current trends of bioactive peptides-new sources and therapeutic effect. Current trends of bioactive peptides—new sources and therapeutic effect. Foods.. 2020;9(7):846 [PubMed] | [CrossRef] | [Google Scholar]
- Schally A. V., Nagy A.. Cancer chemotherapy based on targeting of cytotoxic peptide conjugates to their receptors on tumors. Eur. J. Endocrinol.. 1999;141:1-4. [PubMed] | [CrossRef] | [Google Scholar]
- Wilson KR, Sedberry S, Pescatore R, Vinton D, Love B, Ballard S, et al. Microwave‐ assisted cleavage of Alloc and allyl ester protecting groups in solid phase peptide synthesis. J Pept Sci. 2016;22(10):622-7. [PubMed] | [CrossRef] | [Google Scholar]
- Mader JS, Hoskin DW. Cationic antimicrobial peptides as novel cytotoxic agents for cancer treatment. Expert Opin Investig Drugs. 2006;15(8):933-46. [PubMed] | [CrossRef] | [Google Scholar]
- Behrendt R, White P, Offer J.. Advances in Fmoc solid‐phase peptide synthesis. J Pept Sci. 2016;22(1):4-27. [PubMed] | [CrossRef] | [Google Scholar]
- Shenmar K, Sharma KK, Wangoo N, Maurya IK, Kumar V, Khan SI, et al. Synthesis, stability and mechanistic studies of potent anticryptococcal hexapeptides. Eur J Med Chem. 2017;132:192-203. [PubMed] | [CrossRef] | [Google Scholar]
- Vinogradov AA, Yin Y, Suga H.. Macrocyclic peptides as drug candidates: recent progress and remaining challenges. J Am Chem Soc. 2019;141(10):4167-81. [PubMed] | [CrossRef] | [Google Scholar]
- Pedersen SL, Tofteng AP, Malik L, Jensen KJ. Microwave heating in solid-phase peptide synthesis. Chem Soc Rev. 2012;41(5):1826-44. [PubMed] | [CrossRef] | [Google Scholar]
- Aletras A, Barlos K, Gatos D, Koutsogianni S, Mamos P.. Preparation of the very acid-sensitive Fmoc-Lys (Mtt)-OH Application in the synthesis of side-chain cyclic peptides and oligolysine cores suitable for the solid-phase assembly of MAPs and TASPs. Int J Pept Protein Res.. 1995;45(1995):Array-96. [CrossRef] | [Google Scholar]
- Han Y, Barany G.. Novel S-Xanthenyl protecting groups for cysteine and their applications for the N α-9-fluorenylmethyloxycarbonyl (Fmoc) strstegy of peptide Synthesis 1-3. J Org Chem. 1997;62(12):3841-8. [CrossRef] | [Google Scholar]
- Mant CT, Kondejewski LH, Cachia PJ, Monera OD, Hodges RS. Analysis of synthetic peptides by high-performance liquid chromatography. Meth Enzymol.Meth Enzymol. 1997;289:426-69. [PubMed] | [CrossRef] | [Google Scholar]