ABSTRACT
In today’s modern world, there is a rapid increase in the control and systemic administration of drugs through the oral route, because of its various advantages like high patient compliance, easy dose administration and availability of various formulations. One of the novel approaches in this area is Gastro Retentive Drug Delivery Systems (GRDDs) is a systemic approach to increase the gastric residence time of the drug which results in the site-specific drug release of drug in the upper part of GIT for local and systemic effect of the drug. It is a unique approach that has become more popular in the last three decades. The sole purpose of this article is to summarize and present recent as well as past article which focuses on the GRDDs and their various aspects. This article addresses briefly on various aspects of GRDDs their classification, advantages and limitations, factors affecting gastric residence time, evaluation, recent advancements, regulatory requirements and future prospective of GRDDs.
INTRODUCTION
The technique of delivering a pharmaceutical product to particular therapeutic areas in humans and animals by a various of methods of administration, such as oral, transmucosal, topical, inhalation and intravenous, is known as drug delivery. The history of medicine delivery dates significant technology advancements didn’t happen until the 1970s. Since then, progress has concentrated on improving drug delivery that is not only targeted but also maintains a controlled release rate, increases cellular absorption and minimizes non-specific effects-all of which frequently necessitate an extended shelf-life and stability for the medication. Using an appropriate Drug Delivery System (DDS) is crucial to protecting the medication during the administration process and releasing it at the intended target site, even though the drug’s cytotoxicity and therapeutic efficacy still play major role. The oral route was the most reputable and practical method of drug administration. The pharmaceutical industry finds the enormous curative benefits of oral controlled release to be more appealing than the therapeutic benefits. Gastro-retentive drug delivery systems, which stay in the stomach, are preferred for the oral administration of medications having an absorption window in a specific section of the gastrointestinal tract (Figure 1). These methods support the highest level of bioavailability by continuously releasing the drug prior to it reaching the absorption window. Now before going in detail about the GRDDs, we have to take a look on gastrointestinal tract especially on the anatomy and physiology of stomach.
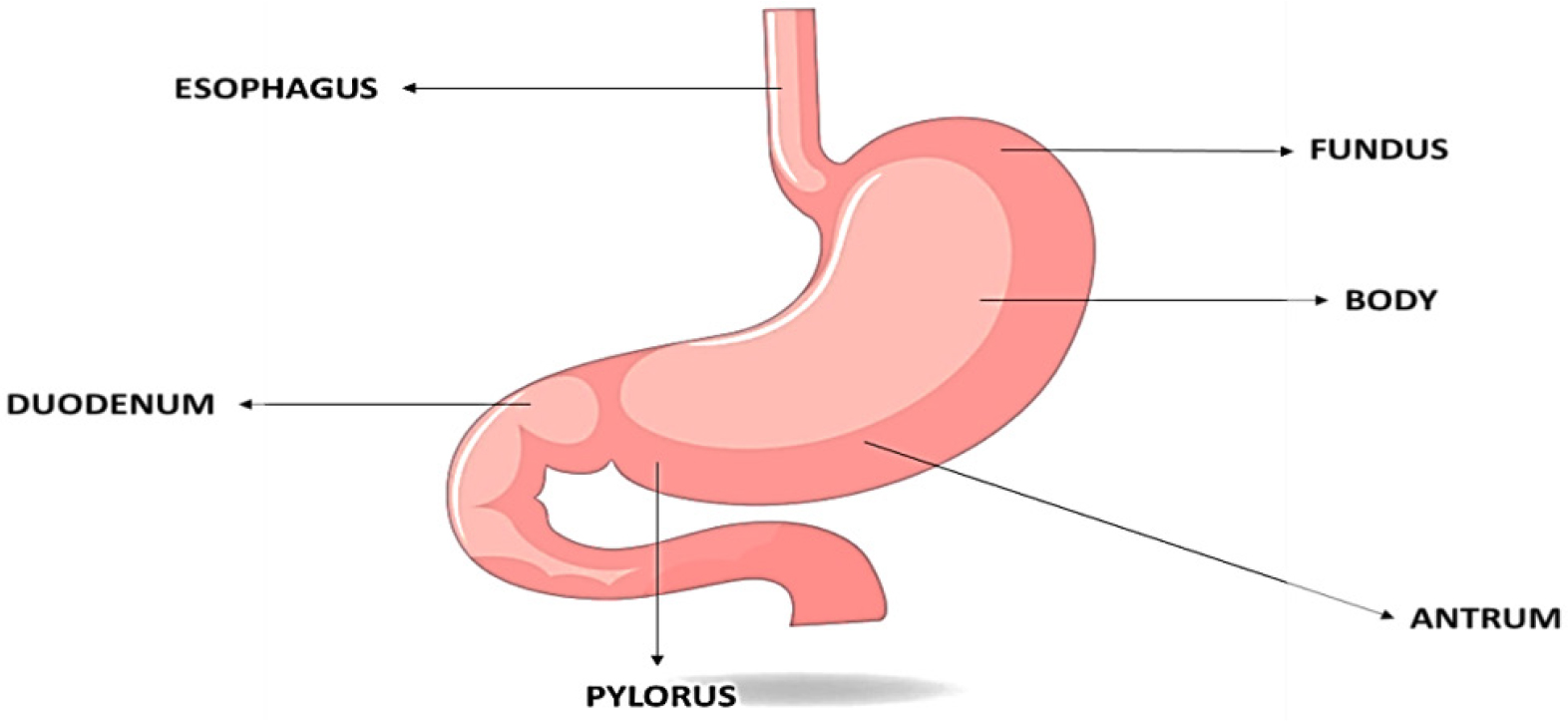
Figure 1:
Anatomy of stomach.
In multicellular animals, the GI tract, also known as the alimentary canal or the gastrointestinal tract, is the system of organs that absorbs food, breaks it down to release nutrients and energy and then excretes any leftover waste. The GI tract’s primary roles include secretion, absorption and motility, which aid in digestion (Muaddiet al., 2024; Pushpamalaret al., 2021; Turacet al., 2024; Waqaret al., 2024). The motility phases of GIT is generally divided in 4 phases as shown in Figure 2 i.e. phase 1 to phase 4, The most quiescent phase, Phase I (45-60 min), has few or no contractions; Phase II (30-45 min) has sporadic action potentials and contractions that progressively get stronger and more frequent as the phase goes on; and Phase III (5-15 min) is a brief interval of strong contractions and peristaltic waves that affect the proximal and distal gastric regions (also known as “housekeeper waves”). The starved stomach’s indigestible contents are eliminated during this phase; Phase IV (0-5 min) is a transitional period of waning activity before the subsequent cycle starts (Liaoet al., 2009). The gastrointestinal tract is made up of a number of organs that are sometimes grouped as cylinders, spheroids, or intermediate forms and they all share a similar structure as shown in Table 1. Transporting and breaking down food are the GI tract’s primary tasks (Streubelet al., 2006).
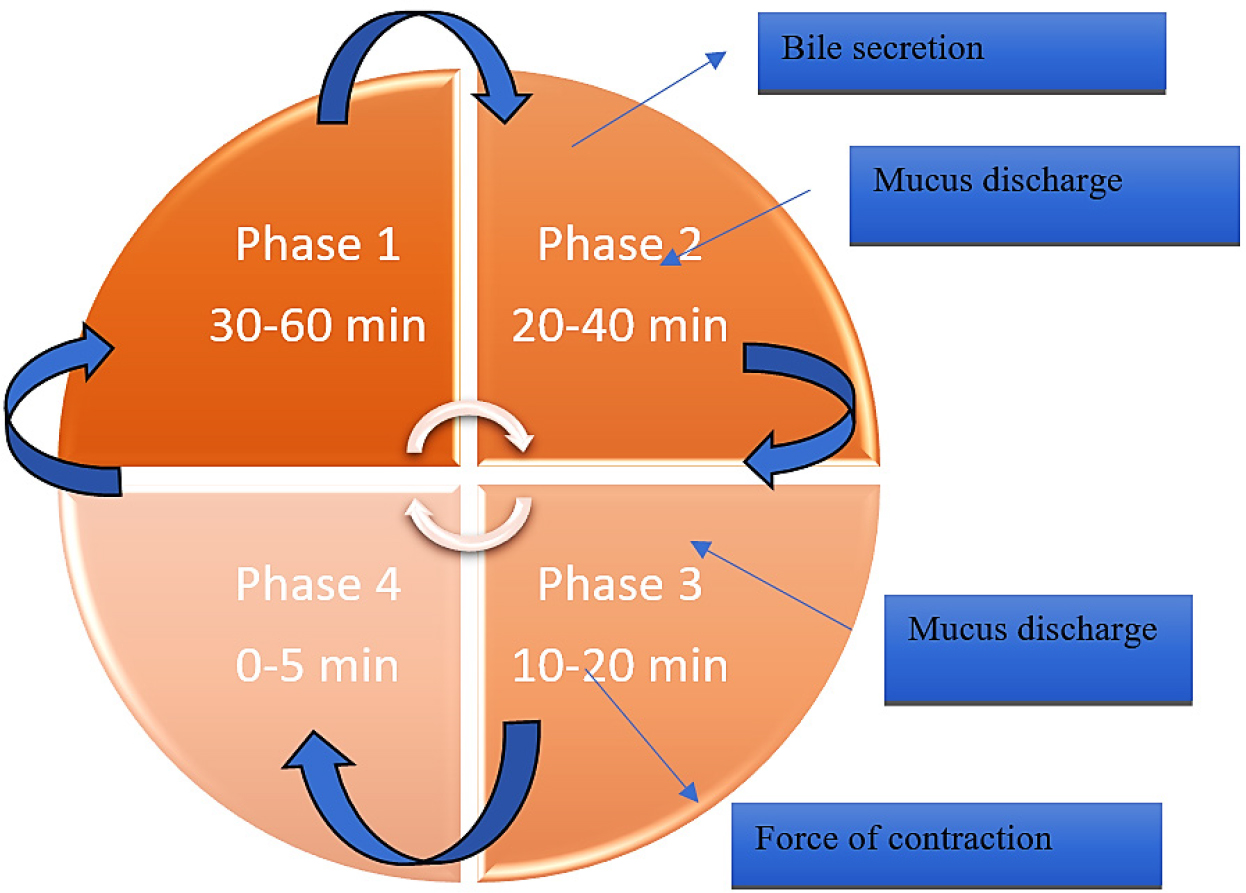
Figure 2:
The motility phases of GIT.
Length (cm) | Modes of absorption | pH | Absorption time (hr) | |
---|---|---|---|---|
Oral cavity | 15-20 | Passive diffusion. | 5.8-6.8 | short |
Stomach | 20 | Passive diffusion. | 1.2-3.5 | 0.25-3.0 |
Duodenum | 25 | Passive diffusion active transport active transport, facilitated transport, pinocytosis. | 4.6-6 | 1-2 |
Jejunum | 300 | Passive diffusion active transport facilitated transport, pinocytosis. | 6.3-7.3 | _ |
Ileum | 300 | Passive diffusion active transport facilitated transport, pinocytosis, ion pair. | 7.3-8.0 | 1-10 |
Cecum | 10-30 | Passive diffusion active transport facilitated transport, pinocytosis. | 7.5-8.0 | Short |
Colon | 150 | Passive diffusion active transport. | 7.9-8.0 | 4-20 |
Anatomy of GIT.
Drugs can be held back in the stomach for a longer period of time using the sustained drug delivery method known as GRDDS. The benefits of GRDDS include its capacity to boost bioavailability and improve the solubility of medications that are not readily soluble in high pH, allows for therapeutic level management to lessen the likelihood of fluctuations and may increase the half-life in order to decrease the amount of drug administration. Still these medications that cause stomach irritation or unstable gastric pH are not appropriate for use with this system can have noteworthy first-pass consequences. The GRDDS consists of multiple systems formulation, such as raft-forming, aggressive, high density, expandable and floating fascinating (Annisa, n.d.-b). Determining the types of GRDDS is the goal of this investigation. Getting ready (Chenet al., 2024). GRDDSs can improve the regulated administration of medications with an absorption window by continually releasing the medication for a longer amount of time prior to it reaching the absorption site. Sometimes it’s preferable to prolong a medication’s stomach retention in order to obtain advantages for therapy of medication absorbed via the proximal portion of the GIT (Gastrointestinal Tract) or exhibit low solubility in or degradation under an alkaline Ph or in GIT’s lowest region. GRDDS are beneficial for certain medications because they improve their Pharmacological efficiency, minimize medication waste, increase bioavailability, decrease drug waste, Prolonged the maintenance of steady state of drug release which reduces fluctuations in the therapeutic levels of drug in the blood (Chenet al., 2024). GRDDS have been one of the breakthroughs in the field of pharmacology. The other systems integrated into such novel drug delivery systems aim at enhancing the gastric retention of the drug in order to improve its absorption and bioavailability. What makes GRDDS unique is its ability to either float on, or stick to the gastric mucosa, allowing the dosage form to stay within these organs longer. GRDDS is mostly developed in the following forms: floating systems, bioadhesive systems, expandable systems. Each of the aforementioned types has advantages and disadvantages. Devices that work on the principle of floating remain above the gastric fluids so that the movement of the drug sitting underneath it from moving further down the GIT is diminished. Bioadhesive devices adhere to the inner wall of the stomach, which is beneficial for the drug that can cross the stomach. Expandable devices enhance in volume or balloons over upon swallowing to stop further ejaculation to the duodenum. In addition to being advantageous, GRDDS has a myriad of advantages that are of importance to the health care sector. Optimum positioning of these dosage forms in the stomach helps in the management of diseases of the GI tract. It is also possible to decrease the number of doses of the drug within a certain period, which is a very important point from the standpoint of safety and convenience for the patient. In this review article we will discuss in detail about various formulation approaches or types of GRDDs, selection criteria of drugs in GRDDs, advantages and limitation of GRDDs, clinical trial and global market of the Gastro retentive tablets or capsules, present and future prospective of Gastro retentive drug delivery system (Muaddiet al., 2024).
Factors controlling the gastro retention of the dosage form
Physical and chemical aspects
Formulation factor: One of the elements influencing the dosage form’s GRT is its shape. The potential for stomach retention of six shapes-ring, tetrahedron, cloverleaf, string, pellet and disk-was assessed in vivo. At 24 hr, the rings (3.6 cm in diameter) and tetrahedrons (each leg 2 cm long) showed almost 100% retention. However, the following showed 0% retention at 24 hr: string (12 cm~2 mm~2 mm/24 cm~2 mm~2 mm), pellets (4 mm), discs (2.5 cm diameter), 40-67% retention and cloverleaves (2.2-3.2 cm in diameter).
Size: The length of time a dose form is held in the stomach can also be influenced by its size. Larger pills are ejected during the housekeeping waves, whereas smaller ones are discharged from the stomach during the digesting phase (Tafishet al., 2023).
Stability based on pH
Physiological factors
The kinetics of drug absorption and the total amount absorbed can be significantly altered by physiological factors such as the mechanism of absorption, gender, age, ethnic origin, the human genome, or the health condition of the treated patient. This can change the pharmacokinetics of a drug and potentially its effect when compared to observed behaviour in healthy adults. When comparing patients to healthy populations, the main physiological parameters that are frequently changed are the intestinal surface area, epithelial permeability, gastric emptying rate and pH, transit times across the various intestinal segments, gastrointestinal enzyme and transporter expression and intestinal surface area (Stillhartet al., 2020).
Classification of GRDDs
The two primary categories of GRDDS are floating and non-floating systems (Figure 3). Based on how they float, floating systems are further divided into effervescent and non-effervescent systems, while non-floating systems are divided into four groups according to how they employ gastro retention.
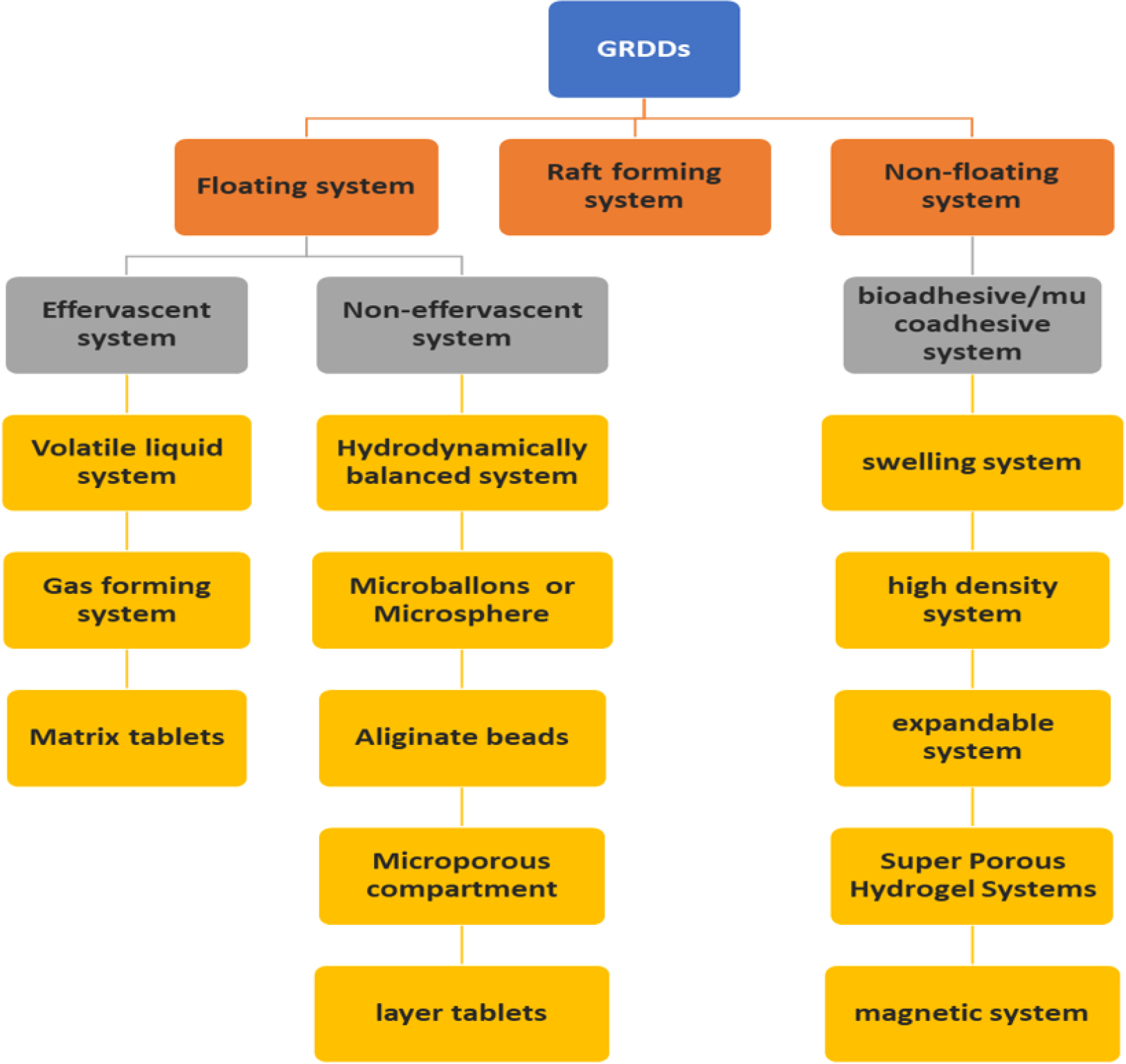
Figure 3:
Classification of GRDDs.
Floating GRDDs
In 1968 Davis initially reported floating systems, which have a lower bulk density than the stomach content. They have the ability to continually release the medication and stay fluid in the stomach for extended periods of time. The leftover system is finally vacuumed. Fasting causes the stomach to empty considerably more quickly and floating systems mostly rely on food to postpone emptying and supply enough fluid to sustain them. Because FDDS have a lower bulk density than gastric fluids, they stay buoyant in the stomach for a longer amount of time without slowing down the rate at which the stomach empties. Delivery systems that inflate to a point where they can’t pass through the pylorus are known to exist. As a result, the administered dose is kept in the stomach for an extended amount of time when the polymer comes into touch with gastric juice and absorbs water and swells. The medication is gradually removed from the system at the appropriate pace while it is floating on the stomach content. The stomach’s remaining system is emptied once the medication has been discharged. This might lead to higher GRT and more effective management of variations in the plasma drugs concentration. The floating drug delivery system is further divided into two parts, effervescent, non-effervescent systems and Raft forming (Figure 4) (Vinchurkaret al., 2022).
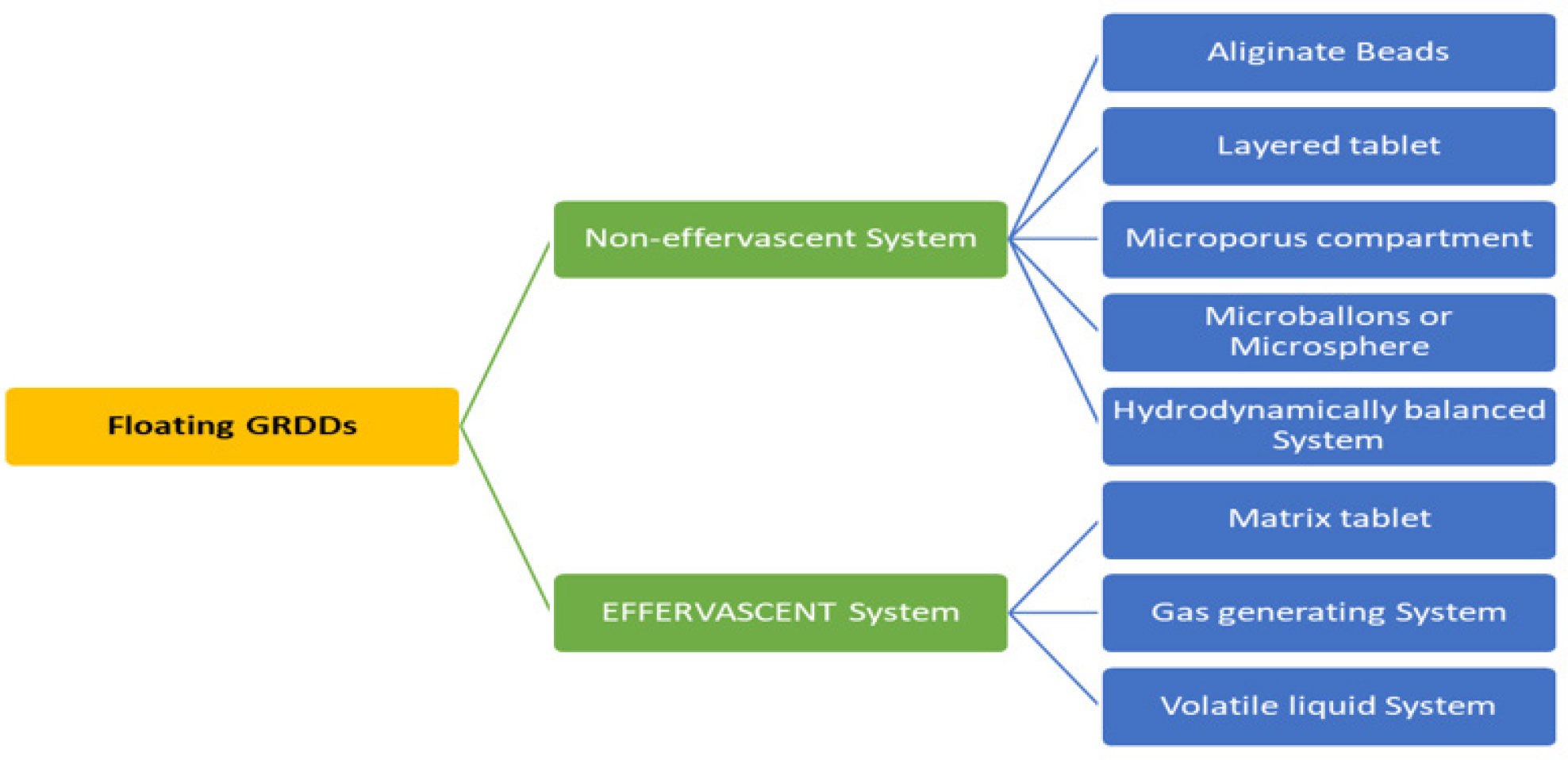
Figure 4:
Classification of Floating GRDDs.
Effervescent GRDDs
Effervescent systems use gas generating agents, carbonates (like sodium bicarbonate) and other organic acids (like citric and tartaric acid) in the formulation to create Carbon Dioxide (CO2) gas, which lowers the system’s density and causes it to float on the stomach fluid. Alternatively, a matrix with a liquid component can be included to create gas that evaporates at body temperature as shown in Figure 5 like pentoxyfilline. It falls into one of three classes: Volatile liquid system, Gas forming system and Matrix tablet.
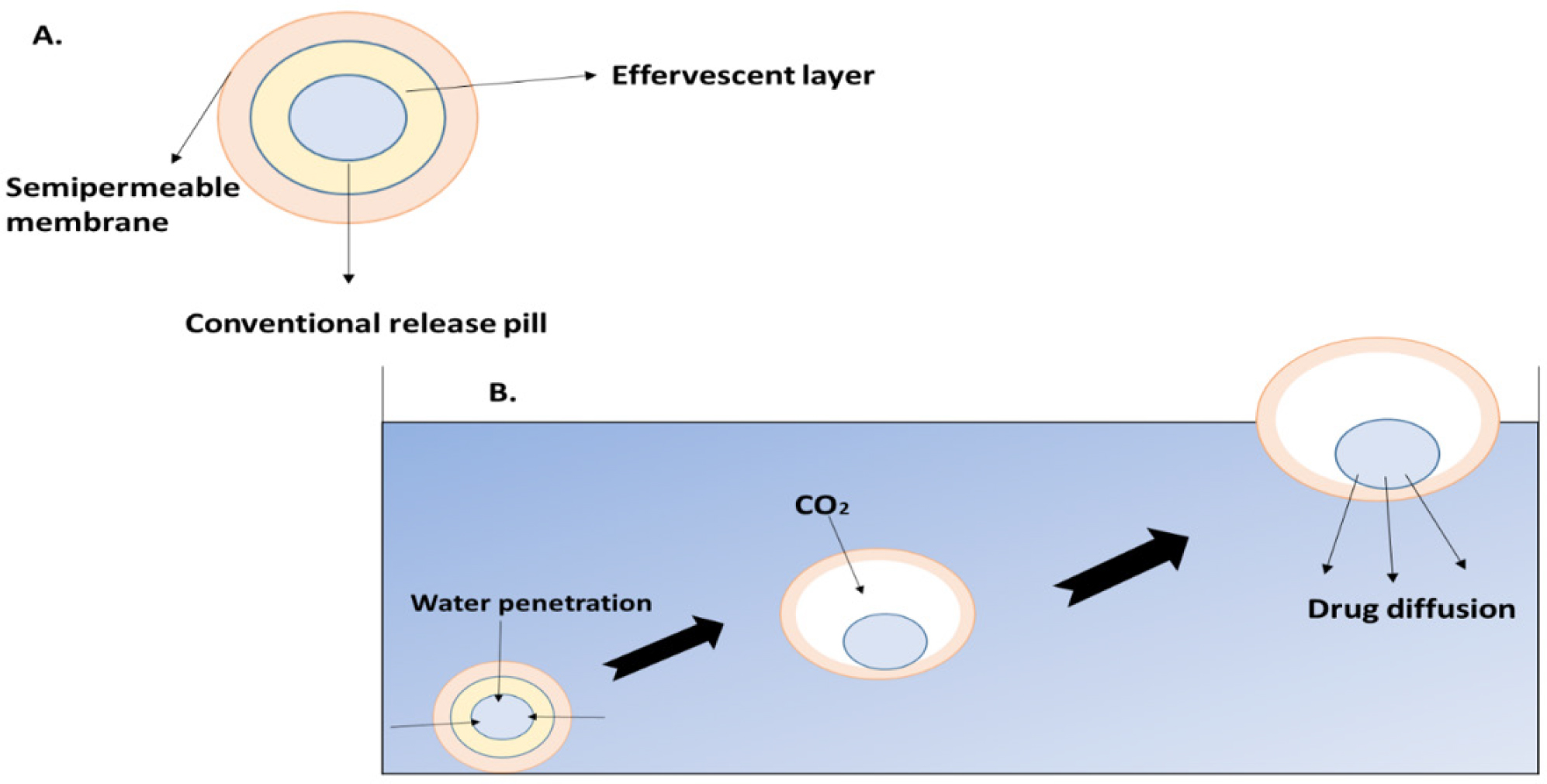
Figure 5:
Effervescent systems and mechanism of effervescent system.
Volatile liquid system
These systems include an inflatable chamber that expands in the stomach due to the presence of liquid ether, which gasifies at body temperature. In order to create these systems, a drug reservoir-which might be a drug or an impregnated polymeric matrix-is loaded into an inflated chamber and then sealed in a gelatin capsule. Following oral administration, the capsule dissolves, releasing the inflated chamber and drug reservoir. The medication is continually pumped into the stomach fluid from the reservoir. e.g. lamivudine (Rashmithaet al., 2020).
Gas generating system
Floating tablets with many unit types that produce CO2 have also been produced. The sustained release tablet that serves as the system’s seed is encased in two layers. In addition to tartaric acid, the inner layer is an effervescent layer that also contains sodium bicarbonate and the outermost layer is a swellable membrane layer that contains PVA, shellac and other materials. e.g. ranitidine hydrochloride.
Matrix tablets
The release of drugs via the polymer matrix of the matrix tablet is regulated by the collapsible spring as shown in Figure 6. Resin beads filled with bicarbonate and coated with a substance called ethyl cellulose are commonly used in the preparation of these systems. Water can permeate the covering since it is permeable but not soluble. As a result, the stomach produces CO2, which makes the beads float. Citric acid to sodium bicarbonate has been shown to have an ideal stoichiometric ratio of 0.76:1 for gas production. A combination of sodium alginate and sodium bicarbonate, extremely swollen hydrocolloids, light mineral oils and floating mini-capsules with a polyvinyl pyrrolidone, lactose and sodium bicarbonate core are some other described methods and materials. e.g. Satvudine (Shahet al., 2010).
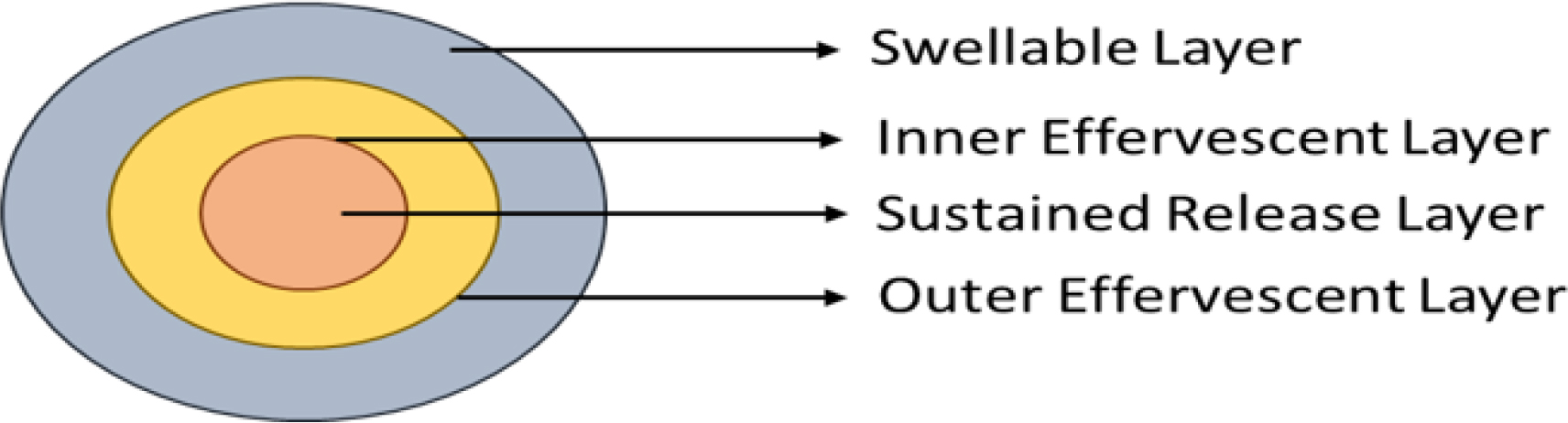
Figure 6:
GRDDs Matrix tablet.
Some common example of floating dosage forms is shown in Table 2.
Floating dosage form | Example | References |
---|---|---|
Floating tablets | Acetyl salicylic acid, Ciprofloxacin, Fluorouracil, Prednisolone, Theophylline, Acetaminophen Ampicillin, Atenolol, Captopril, Cinnarizine, isosorbide dinitrate, Verapamil HCL, Acyclovir, Amoxicillin trihydrate, Losartan. | (Adibkiaet al., 2013) |
Floating capsule | Diazepam, Misoprostol, Propranolol, Pepstatin, Ldopa and benserazide, Furosemide. | (Adibkiaet al., 2013) |
Floating granules | Ranetidine ibuprofene, cephalexine, indomethacine. | (Patelet al., 2022; Patelet al., 2007; Rajanikantet al., 2010; VenKateswarluet al., 2016) |
Floating microsphere | Aspirin, Terfendadine, Tranilast Ketoprofen, Verapamil, Orlistat, Acyclovir, Piroxicam, Rosiglatizone, Repagalinide, Nitrendipine, Acetohexamic Acid. | (Miyazakiet al., 1988) |
Floating dosage forms example.
Non-Effervescent GRDDs
Non-effervescent systems integrate a high concentration of sodium carboxymethyl cellulose, polysaccharides, matrix-formed polymers and numerous gel-forming, expanding cellulosic hydrocolloids through tablets or capsules. These gel formers, polysaccharides and polymers not only create a colloidal gel barrier that controls the amount of fluid stimulated into the appliance and the subsequent medication discharge, but they also moisturize stomach juice when they come into touch with it. The surrounding hydrocolloid coating’s hydration preserves the gel layer while the outside surface breaks down due to both dosage types. Dose elasticity takes on its form because to the air gathered by the expanded polymer, which also lowers volume. The creation of floating intragastric devices has made advantage of the previously described techniques. e.g. losartan potassium. The non effervescent GRDDs is further classified into 5 parts: Hydrodynamically balanced system, Microballons or Microsphere, Alginate beads, Microporous compartment and layer tablets (Mukundet al., 2012).
Hydrodynamically balanced system
Sheth and Tossounian created the HBS system for the first time in 1984. Consisting of multiple gel-forming hydrophilic polymers, it is a single unit dose form. Agar, carrageenan, sodium carboxymethylcellulose, Hydroxypropyl Cellulose (HPC), hydroxyethylcellulose, HPMC and alginic acid are a few of the polymers that go into creating the HBS system as shown in Figure 7. The medication and polymer are combined in this technique and the gelatin capsule is then filled. The medicine and gel-forming hydrophilic polymer can be mixed uniformly to create a floating tablet. The polymer hydrates and expands as it comes into contact with stomach juice, keeping the tablet’s bulk density at less than 1 g/cm3. As a result, the low-density systems extend the GRT by floating on the stomach fluid. Like ofloxacin.
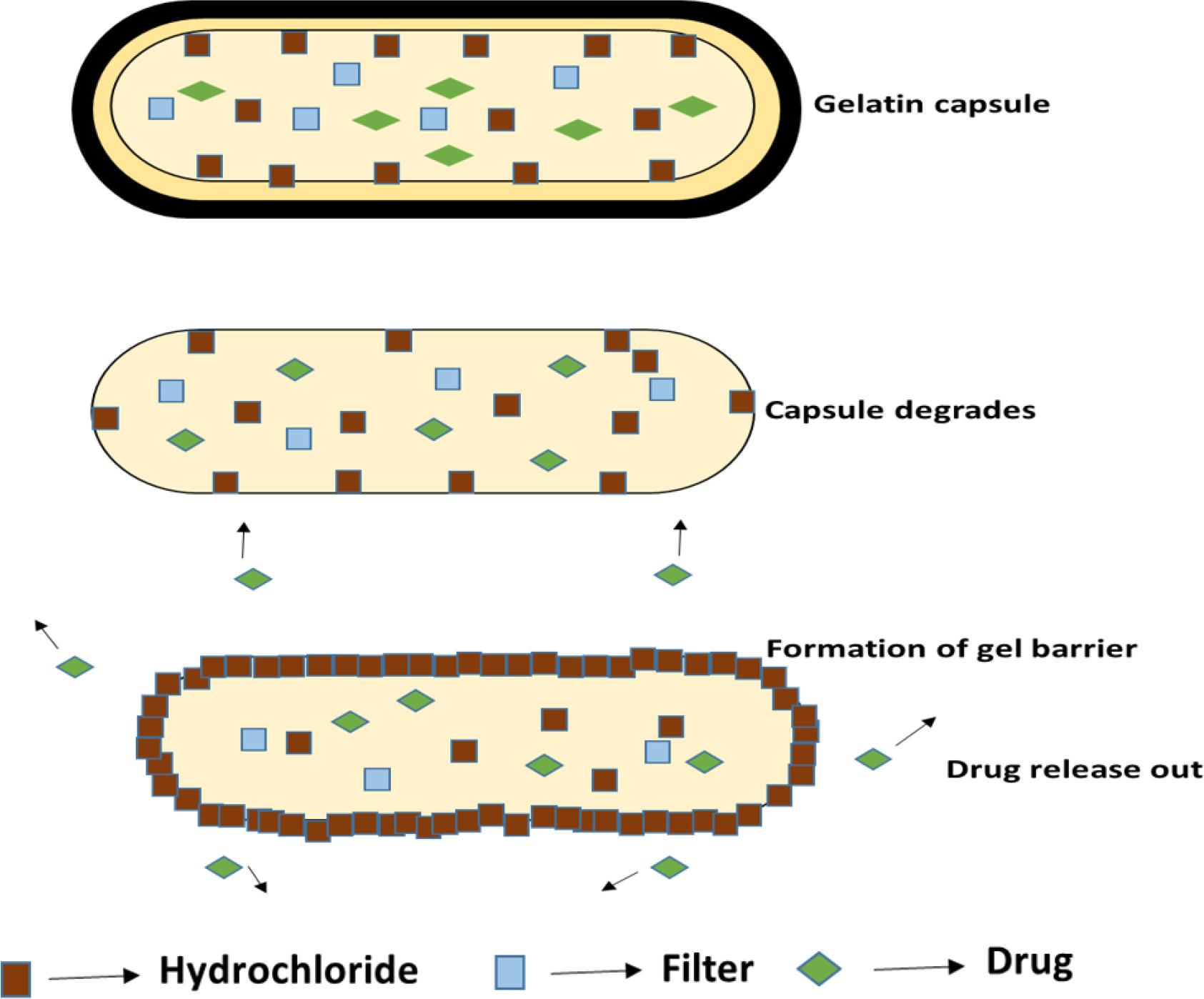
Figure 7:
Hydrodynamically balanced system.
Microballon/Microsphere
These multi-unit floating structures, known as drug-loaded microballoons or hollow microspheres, are created using straightforward solvent evaporation or solvent diffusion procedures. The polymers that are frequently used to create microballons are polycarbonate, cellulose acetate, calcium alginate, Eudragit S, agar and low-methoxylated pectin. These dosage forms’ buoyant tendency and drug release can be influenced by a number of formulation factors, including solvent concentration, plasticizer to polymer ratio and polymer quantity and this extend the Gastro retentive time of the drug e.g acyclovir (Jeganathet al., 2022).
Alginated beads
Alginate formulations with a high entrapment rate gave rise to a novel kind of floating drug delivery system that forms multi-unit floating spherical beads by utilizing the ionic gelation procedure. Calcium alginate that had been freeze-dried and precipitated when sodium alginate solution was added to an aqueous calcium chloride solution was used to create floating alginate beads with a diameter of around 2.5 mm. After being separated, the beads were snap-frozen in liquid nitrogen and freeze-dried for 24 hr at -40ºC. This resulted in the production of a porous system that gave a prolonged residence length of more than 5.5 hr in the stomach while remaining buoyant.
Microporous compartment
This method is based on enclosing a drug reservoir in a microporous space that has pores on both the top and bottom walls. To avoid the gastrointestinal surface coming into direct touch with the undissolved medication, the drug reservoir compartments outside walls are totally sealed. The delivery system hovers over the stomach contents thanks to the flotation chamber that holds trapped air. Through the opening, gastric fluid enters, dissolves the medication and then transports the dissolved medication continuously throughout the intestine for absorption (Tripathiet al., 2019).
Layered tablets
In a layered tablets more than one layer is present, the first layer is called the immediate release layer and it releases the first dose from the system. The second layer is called the sustained release layer and it absorbs the gastric fluid, forming an impermeable barrier on its surface made of colloidal gel and keeping its bulk density below 1 as a result the Gastro retentive time of these tablets is increased and they release the drug for extended period of time as shown in Figure 8.
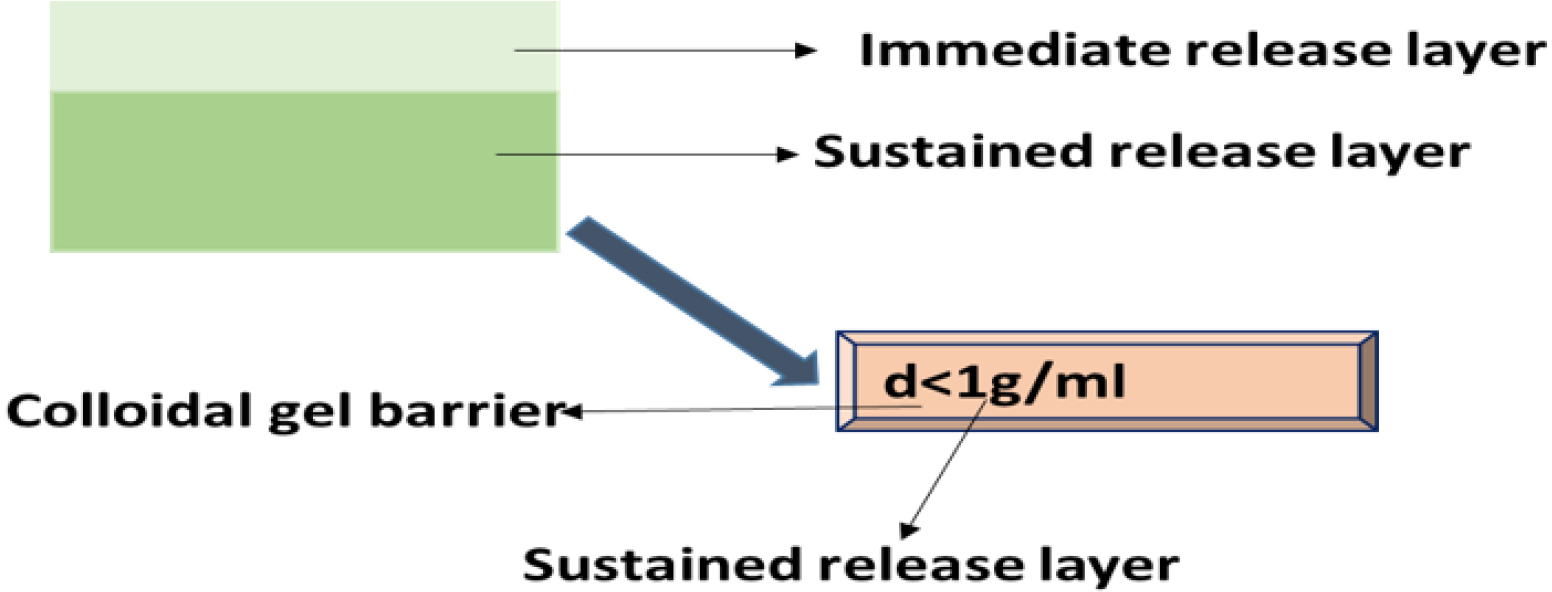
Figure 8:
Layered tablet.
Raft forming GRDDS
Raft forming systems are mostly taken into consideration for the administration of antacids and other drugs for gastro-infection and gastro-intestinal illnesses. When the gel-forming solution comes into touch with gastric fluid, it expands and forms a viscous, compact gel with trapped CO2 bubbles that forms an inflatable layer on top of the fluid and releases the medicine gradually into the stomach. As a result, these rafts forming system have a longer Gastro retentive time and release the medication over a longer duration of time as shown Figure 9 (Patilet al., 2011). This technique can provide comparatively stable plasma profiles by continuously releasing a pharmacological molecule. At room temperature, these hydrogels are liquid, but when they come into touch with bodily fluids or experience a pH shift, they gel. The purpose of this system’s design is to either decrease the frequency of dosing or enhance the effectiveness of the medication by localizing it at the site of action, lowering the dosage needed, or delivering the medication consistently. Additionally, the raft forming technology has several potential benefits, such as easier administration, improved patient compliance and straightforward production procedures (Lodhet al., 2020).
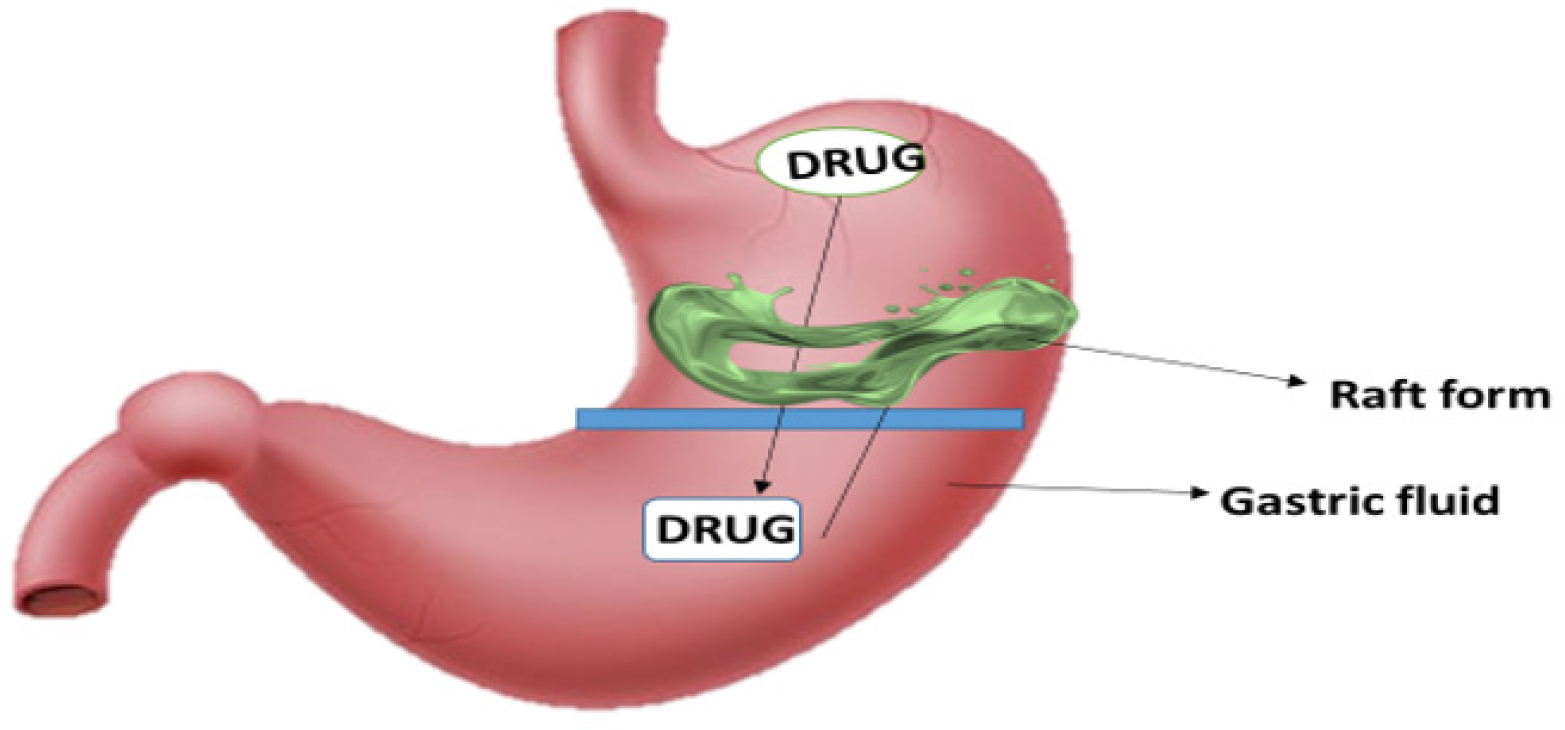
Figure 9:
Raft forming system.
Raft Formation Based on Physical Mechanism
Bloating: In situ gel formation is the process by which materials expand to cover a desired space after absorbing water that comes from the surrounding environment.
Diffusion: It involves the precipitation or solidification of the polymer matrix due to the solvent from a polymer solution diffusing into the surrounding tissue. N-Methyl Pyrolidone is a mixture of polymers that may be used in this situation (Prajapatiet al., 2013).
The Raft Forming System’s Design
The raft forming system’s formulation is influenced by the drug’s physicochemical characteristics, the patient population, the ailment that has to be treated and marketing preferences. Anatomical and physiological aspects include membrane transport and tissue fluid pH; formulation factors include pH, gelation temperature, viscosity, osmolarity and spreadability; physicochemical factors include molecular weight, lipophilicity and molecular charge. In order to ensure that the dose form is stomach retained the following requirements must be met by the dosage form. They are listed in the following order:
Slow release of the medication from the body is recommended.
The dose form needs to be strong enough to endure the power of the stomach’s peristaltic waves as well as the ongoing churning, grinding and contractions.
Maintaining a specific gravity between 1.004 and 1.01 g/cm3 is necessary.
The dose form needs to be kept in the stomach for an extended period of time.
Enhanced adherence from patients.
Simple for the patient to administer.
The medical device should be simple to remove from the stomach once the medication is release (Andrewet al., 2022).
Non-Floating GRDDs
The gastro-retentive drug delivery system’s dosage form which does not float in the stomach but rather remains there by a distinct mechanism in the non-floating drug delivery system. This drug delivery system is pH dependant and dissolves at a certain pH. The medication may settle in the stomach, exhibiting bioadhesive and mucoadhesive qualities. The dosage form delivers the drug in a sustained way and at the intended place. The non-floating is further divided into 6 categories bioadhesive/mucoadhesive system, swelling system, high density system, expandable system, super porous hydrogel systems and magnetic system (Razaet al., 2022).
Mucoadhesion and Bioadhesion
The state in which interfacial forces hold two materials together for a prolonged amount of time-at least one of which is biological in nature-is referred to as bioadhesion. Three types of bioadhesion can be found in biological systems:
Type 1: adhesion, such as that seen in aggregation of platelets and healing of wounds, between two biological stages.
Type 2: The adherence of a biological phase to a synthetic substrate, such as the formation of biofilms on prosthetic devices and inserts and cell adhesion to culture dishes. Type 3: Adherence of an artificial substance to a biological substrate, for example, adhesion of synthetic hydrogels to soft tissues and adhesion of sealants to tooth enamel. In the context of drug delivery, the term “bioadhesion” refers to the attachment of a drug carrier system to a specific biological location as shown in Figure 10. The biological surface may be epithelial tissue or the mucus coat on the surface of a tissue. When adhesive attachment occurs to a mucus coat, the phenomenon is called “mucoadhesion.” Leung and Robinson defined mucoadhesion as the interaction between a mucin surface and a synthetic or natural polymer. Mucoadhesion should not be mistaken for bioadhesion, where the polymer is attached to the biological membrane. When the substrate is a mucus membrane, the term mucoadhesion is used (Shaikhet al., 2011).
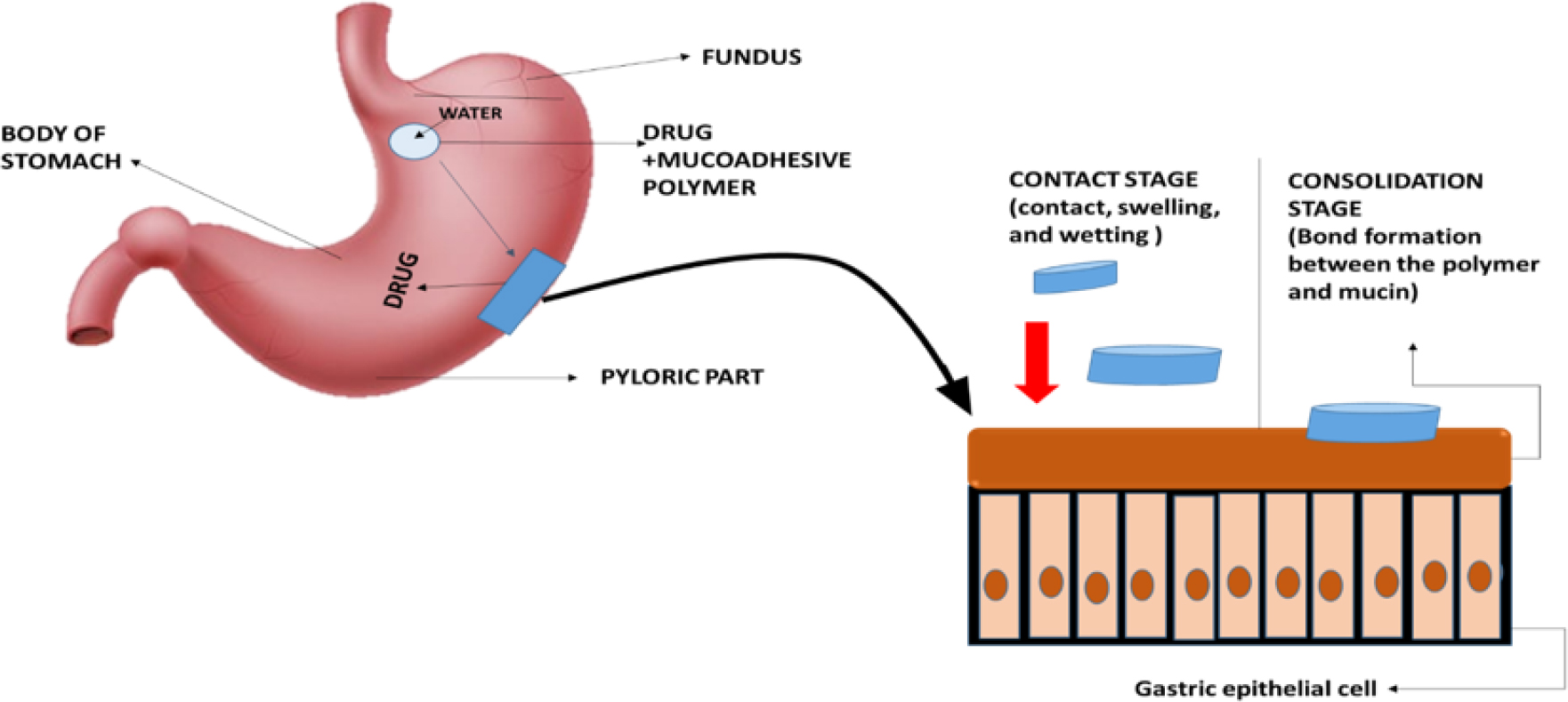
Figure 10:
Bioadhesive/ Mucodhesion mechanism.
Theories of mucoadhesion
Mucoadhesion may be explained by a number of broad theories, including mechanical, fractional, electronic, adsorption, wetting and diffusion theories.
Electronic theory: mucoadhesive polymer and the mucous membrane, which have distinct electrical charges, transfer electrons to form electrostatic attraction.
The theory of Adsorption: mucus and polymer adhesion that is brought about by intermolecular contact
Wetting theory: Capability of mucoadhesive polymer to spread on the mucus with respect to its surface tension
Diffusion theory: According to the theory of diffusion, an interpenetration layer forms when a polymer diffuses into mucus and vice versa.
Mechanical theory: The impact of surface roughness is taken into account in mechanical theory, wherein a rise in contact area promotes adhesion (Chatterjeeet al., 2017).
Muco-adhesive or bio-adhesive formulations were initially developed to control the dispensing of different types of medications and to increase GRT. In 1984, Park and Robinson provided the first description of the mucoadhesive system. Mucosal adhesive enhances the effectiveness of drug delivery by preserving a strong, long-lasting bond among the drug administration device and the site of absorption. The latest method, developed thirty-five years ago, uses mucoadhesive systems to prolong the duration of the stomach’s residency. Artificial or natural polymers adhere to mucosal tissues through a process called mucosal adhesion. Mucoadhesive drug delivery systems are made of a combination of polymers with specific surface properties and an active pharmaceutical ingredient (API). Mucoadhesive polymers have a strong affinity for mucosal tissues, which allows them to adhere to their surface. Muco-adhesion is one tactic that might be used in conjunction with the previously outlined process to achieve both physical and chemical retention of gastric retention. For medication delivery applications such tablets, patches, liposomes, gels, nano suspensions, micro/nanoparticles and colloidal dispersions, mucoadhesion is a useful strategy (Kumaret al., 2024).
Swelling system
Due to their large size, the pylorus sphincter blocks the passage of bloated dosage forms. A type of Gastro retentive system known as a swelling system enlarges dramatically when it comes into touch with stomach contents. These dose forms are retained in the stomach for extended periods of time because of their swelling. From time to time, the term “plug type dosage form” refers to gastric retentive dosage forms because of their tendency to become trapped at the pyloric sphincter. The use of polymeric materials that expand when exposed to gastric juice results in bigger dosage forms that are unable to pass through the pyloric sphincter. The preservation of the strongest therapeutic benefits and the reduction of swelling and polymer erosion’s numerous negative consequences. The size of the dose form is perfect for simple swallowing and effectively appropriate for stomach retention. The swollen behaviors of these formulations are induced by osmotic water absorption. The majority of gastrointestinal retention dosage forms that are unfoldable are composed of biodegradable polymers. polymer-containing materials. The bioerodible polymers used in these systems, which can resemble a tetrahedron, ring, planner membrane, four-label disk, or four-limbed cross, can be stretched in the stomach to enhance gastric retention (Hatwaret al., 2023).
High density system
The density of gastric contents is 1.004g/cm3, which is similar to water. When a patient is given high density pellets, they will recede to the bottom of the stomach, become stuck in the antrum’s folds and endure the stomach wall’s peristalsis 40, 41. High density systems have used sedimentation as a retention mechanism. It appears that a density of less than 3 g/cm3 is required to significantly extend the gastric residence duration. Because of their high density, barium sulphate, zinc oxide, iron powder and titanium dioxide can be employed to create such high-density systems. The main significant disadvantage of these systems is their technical difficulty in producing a substantial amount of medication (>50%) and the necessary density of 2.4-2.8 g/cm3 (Nitaveet al., 2014). For example Since theophylline is believed to be able to boost the body’s bioavailability, it is one of the medications that can be converted into high density Gastro retentive dose forms (Nuret al., 2021).
Expandable and unfoldable system
Typically, expandable Gastro Retentive Dosage Forms (GRDFs) are designed to allow for easy oral intake, an expanded form that is produced in the stomach and blocks passage through the pyloric sphincter and a final small form that is produced in the stomach when retention is no longer necessary, that is, once the GRDF has liberated its active ingredient, allowing evacuation. Either swelling or unwinding in the stomach might cause the enlargement. One of the greatest ways to delay stomach emptying may be through unfolding mechanisms. For the patient’s convenience, the unfolding mechanism is often administered as a hard gelatin capsule. Gastric acid causes the carrier to disintegrate in the stomach, releasing the GRDF system, which then unfolds or stretches out to take on its expanded structure. Long periods of time should be maintained by the carrier’s unfolded qualities (Tariqet al., 2015).
Magnetic system
In order to achieve target-specific action, this device is designed to incorporate a tiny internal magnet into the dosage form of a magnetically active molecule. These devices use an external magnet as a trigger to deliver drugs to specified sites (Figure 11). For instance, Ito et al. used bioadhesive granules containing ultrafine ferrite (g-Fe2O3) in rabbits that were kept in the stomach area for 2 hr after being exposed to an external magnet of 1700 G.Using Heidelberg capsules, Groning et al. used this method to calculate the stomach transit time of the magnet-incorporated dosage form. He discovered that the retention duration of the dosage form was more than 6 hr, compared to the control, which was 2.5 hr (Mayuret al., 2013).
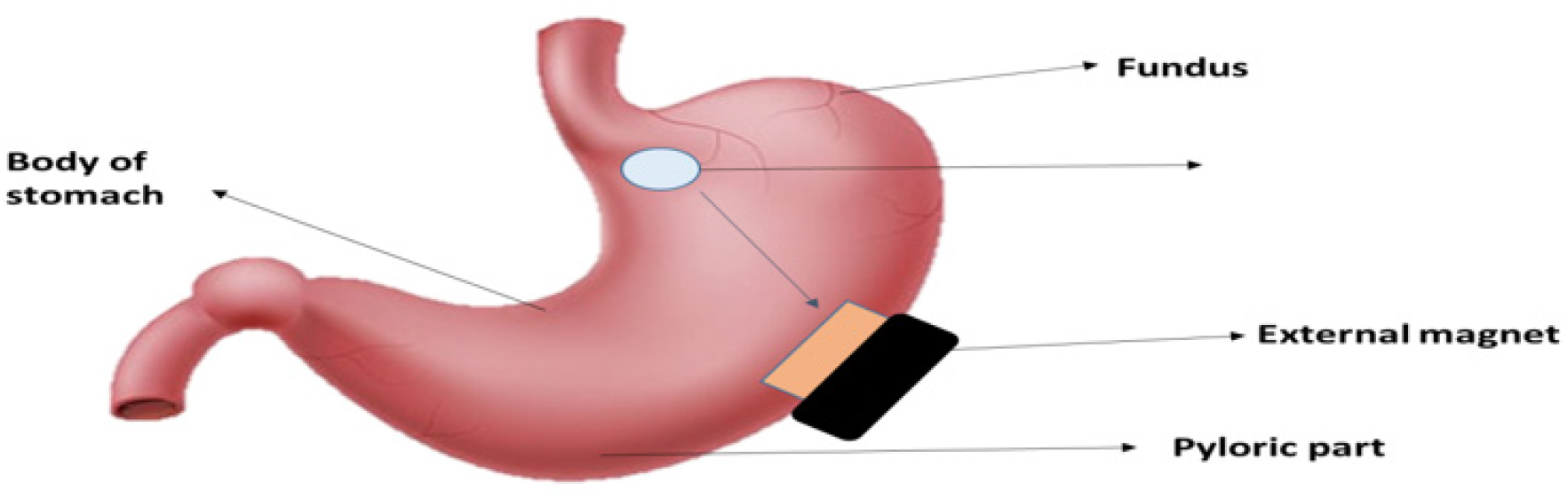
Figure 11:
Magnetic system.
Super Porous Hydrogel Systems
Despite being swellable, these systems differ enough from the traditional varieties to be classified separately, with pore sizes ranging from 10 nm to 10 μm. Conventional hydrogels absorb water extremely slowly; it may take many hours to achieve an equilibrium condition, during which the dosage form may prematurely evacuate. The average pore size of superporous hydrogel is greater than 100 μm and it swells to equilibrium size in less than a minute as a result of capillary wetting through many interconnected open pores that rapidly absorb water. Additionally, they have a significant swelling ratio (100 or above) and are designed to be strong enough to endure pressure from gastrointestinal contractions. Ac-Di-Sol (crosscarmellose sodium), a hydrophilic particle substance, is coformulated to achieve this. Hydrogels that may produce effective pore sizes greater than 10μm are referred to as superporous hydrogels. Because of their fast water absorption by capillary wetting through a multitude of interconnected open pores, SPHs have an average pore size of more than 100 microns and expand to a comparable size in less than a minute. Due to their propensity to inflate to enormous proportions-roughly 100 or more-SPHs need to be mechanically strong enough to withstand the pressure exerted by the contraction of the stomach. Hydrophilic particulate substance Ac-Di-Sol (Crosscarmellose Sodium) can be used to accomplish this. Due to the presence of interconnected tiny holes, a superporous hydrogel is a three-dimensional structure composed of a hydrophilic polymer that absorbs a significant amount of water in a short amount of time. SPHs have hundreds of times more surface area and a shorter diffusion distance than traditional hydrogels because of their porous nature. In contact with water, these features enable dried SPHs to grow very quickly and to a very enormous size. Due to these special qualities, SPHs were first employed in the development of a gastric retention device, which extends the duration that medications remain in the stomach in order to get oral, controlled drug administration over an extended period of time (Mahmoudet al., 2023).
Formulation strategies of GRDDs
Selection of polymers
Numerous features are available for choosing the polymer in dosage form formulation and various drug delivery systems to improve the medication’s release activity and impact. The medicine will release in its maximum action and strength based on the perfect properties of the specific polymer. Here are a few of the traits that were covered:
- It should be environmentally inert and compatible.
- Easy to administer, non-toxic and simple to make.
- It is less expensive and has superior mechanical strength.
The polymer should not interact with the medication molecule and should be inert to the host tissue. Scaffolds for skin tissue engineering and synthetic skin replacements have both been developed using polymeric biomaterials. Additionally, polymers are utilized in transdermal and cutaneous medication delivery carrier formulations (Vermaet al., 2024). With benefits like increased residence time, improved penetration, site-specific adhesion and enzymatic inhibition, site-specific mucoadhesive polymers of the new generation will surely be used. In floating drug delivery systems, polymers as shown in Table 3 are often used to target the administration of drugs to the stomach or another particular area of the gastrointestinal system. Chitosan, pectin, xanthan gum, guar gum, gellan gum, karkaya gum, psyllium, starch, husk, starch, alginates and other natural polymers have been investigated for their possible use in stomach-specific medication administration (Javaidet al., 2017).
Sl. No. | Polymers/Excipients | Approaches | References |
---|---|---|---|
1 | Polycarbophil, Alginic acid sodium carboxymethyl cellulose (NaCMC) HPMC, Polyacrylate, Hydroxethyl Cellulose (HEC), Carrageenans Hydroxypropyl Cellulose (HPC), Polystyrene, or Agar etc., | Hydrodynamically Balanced Systems (HBS). | (Asaneet al., 2008) |
2 | Sodium alginate, Sodium bicarbonate, Acid neutralizer. | Raft systems integrating alginate gels. | (Asaneet al., 2008) |
3 | polycarbophil (polyacrylic acid cross-linked with divinyl glycol), Carbopol/carbomer (carboxy polymethylene), Sodium carboxymethyl cellulose (cellulose carboxymethyl ether sodium salt). | Bioadhesive/mucoadhesiveSystem. | (Kavithaet al., 2011) |
4 | Polyethylene oxide, iron powder, e, Barium Sulphate, Titanium dioxide. | High density system polymers. | (Jainet al., 2023) |
5 | Magnetic granules. | Magnetic System. | (Jainet al., 2023) |
6 | HPMC K 100 LV, HPMC K 100 M, HPMC K4M. | Floating System. | (Jainet al., 2023) |
7 | Polyvinyl alcohol, Poly D, L-lactic-co-glycolic acid. | Hollow microspheres/ Microballoon. | (Rangarajet al., 2010) |
8 | Chitosan, poly-L-lysine [16, 17], dextran, amino-poly oxyethylene. | Alginate beads. | (Leeet al., 1995) |
Polymers used various GRDDs formulations.
Natural polymers used in formulation of GRDDs
Natural or biodegradable polymers are found in nature and can be made by living things. They do not exhibit any negative effects on the health of people or the environment. Since these plant components are naturally carbohydrates, the majority of natural polymers are harmless and biocompatible. Natural sources are inexpensive and may be readily gathered in various seasons in huge numbers. Due to their extensive application in industries, their productions are now being promoted in emerging nations (Nagariyaet al., 2010). Some commonly used natural polymers are: Xanthan, starch, cellulose, Guar Gum and Chitosan, an extracellular polysaccharide made of glucose, mannose and glucuronic acid, xanthan gum is a natural, biosynthetic, edible gum that is utilized as a thickening and stabilizer in a variety of foods. Alkaline deacetylation of chitin produces chitosan, a naturally occurring polymer that is non-toxic, biocompatible and biodegradable. Because of these characteristics, chitosan is a promising material for the creation of both traditional and innovative Gastrointestinal (GI) medication and gene delivery systems. The seeds of Cyamopsis tetragonolobus (Family Leguminosae) are the source of guar gum, a naturally occurring nonionic polymer. Gaur gum is utilized in pharmaceuticals as a polymer in floating drug delivery systems, as well as a binder and disintegrant in solid dosage forms (Sarcanet al., 2018).
Techniques of formation of nanoparticles and Microparticles used in GRDDs
The characteristics of nanoparticles are significantly influenced by their production techniques. The preparation techniques have an impact on the drug loading capacity, drug release patterns and the size of particles and surface characteristics of nanoparticles. As a result, choosing an appropriate preparation technique is crucial. These techniques have to be selected based on the targeted nanoparticles’ characteristics (Subediet al., 2016).
Solvent Evaporation technique
Due to its simplicity of formulation and adaptability to many adjustments for creating micro and nano spherical systems with enhanced features, the technique of solvent evaporation is one of the most used techniques. In order to create polymeric particles, Vanderhoff et al. initially patented it in 1979 as a polymer emulsification method. Several subsequent patents contain claims pertaining to this technique. This method involves dispersing the polymer or micro or nano capsule coating agent in a volatile solvent that is insoluble in the liquid production vehicle phase. Next, the coated polymer solution is used to dissolve or distribute the inner component or the medication to be encapsulated. To achieve the right dimension range of the microsphere, the core material is disseminated in the liquid production vehicle phase by applying the stirring at a specific speed. This method creates an emulsion between the continuous phase and the polymer solution. The core also dissolves in the coating solution, which causes the polymer to shrink around it or create matrix microspheres when heat is applied or the volatile solvent evaporates while being stirred as shown in Figure 12. (Maniruzzamanet al., 2012)
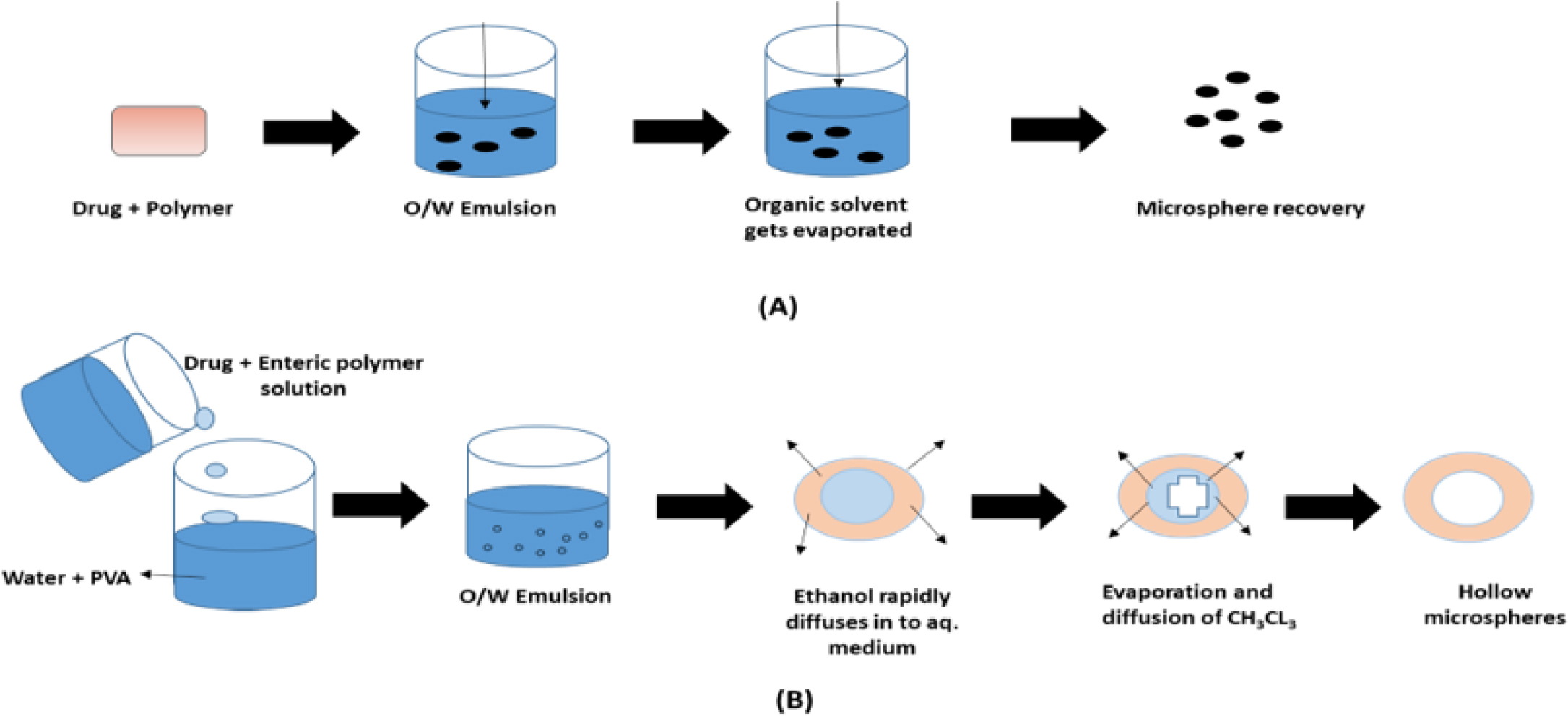
Figure 12:
(A): Solvent evaporation technique; (B) Emulsion solvent diffusion technique.
Hot melt extrusion
The technology of hot-melt extrusion was initially developed at the very end of the 18th century for the production of lead pipes. After that time, the hot melt extruder as shown in Figure 13 has been employed in the production of pipes, sheets and bags as well as in the rubber, plastic and food industries. More than half of all plastic goods, such as bags, sheets and pipes, are now made by HME thanks to the development of high throughput screening. As a result, different polymers have been melted and formed into a variety of forms for a range of industrial and residential uses. Since HME has shown itself to be a reliable technique for creating a variety of drug delivery systems, the pharmaceutical sector has also found use for it. Pumping raw materials through a heated barrel at a high, regulated temperature and pressure to create a product with a consistent density and shape is known as extrusion. While Follonier and his colleagues initially investigated the use of hot-melted technology to produce prolonged release polymer-based pellets of different freely soluble medications, Breitenbach was the first to introduce the development of the melt extrusion method in pharmaceutical manufacturing operations. Compaction and transformation of mixtures from a granular or powdered mixture into a uniformly shaped product are key components of HME. By forcing polymeric components and active substances, including any additives or plasticizers, through an orifice or die under regulated pressure, temperature, feeding rate and screw speed, polymers are melted and formed into products of various shapes and sizes (Crowleyet al., 2007). Complex blends of functional excipients and active medications make up hot-melt extruded dosage forms. In general, functional excipients can be categorized as bulking agents, matrix carriers, release modifying agents, antioxidants, thermal lubricants and other additives. A variety of excipients can be used to provide hot-melted extruded medications certain qualities that are comparable to those found in conventional dosage forms. Plasticizers may reduce the processing temperatures required for HME, which would lessen the degradation of drugs and carriers. The addition of different functional excipients can alter the release of drugs from these systems. The rate-modifying agent’s characteristics can either raise or reduce the active compound’s rate of dissolution. It may be necessary to add antioxidant compounds, acid acceptors and/or light-absorbing substances to systems that exhibit oxidative or free radical deterioration during processing or storage. The active ingredient is embedded in the carrier formulation, which is frequently made up of one or more “meltable” compounds and other functional excipients, in hot-melt extruded medication delivery systems. Typically, the meltable material is a polymer or wax with a low melting point (Vantimittaet al., 2022).
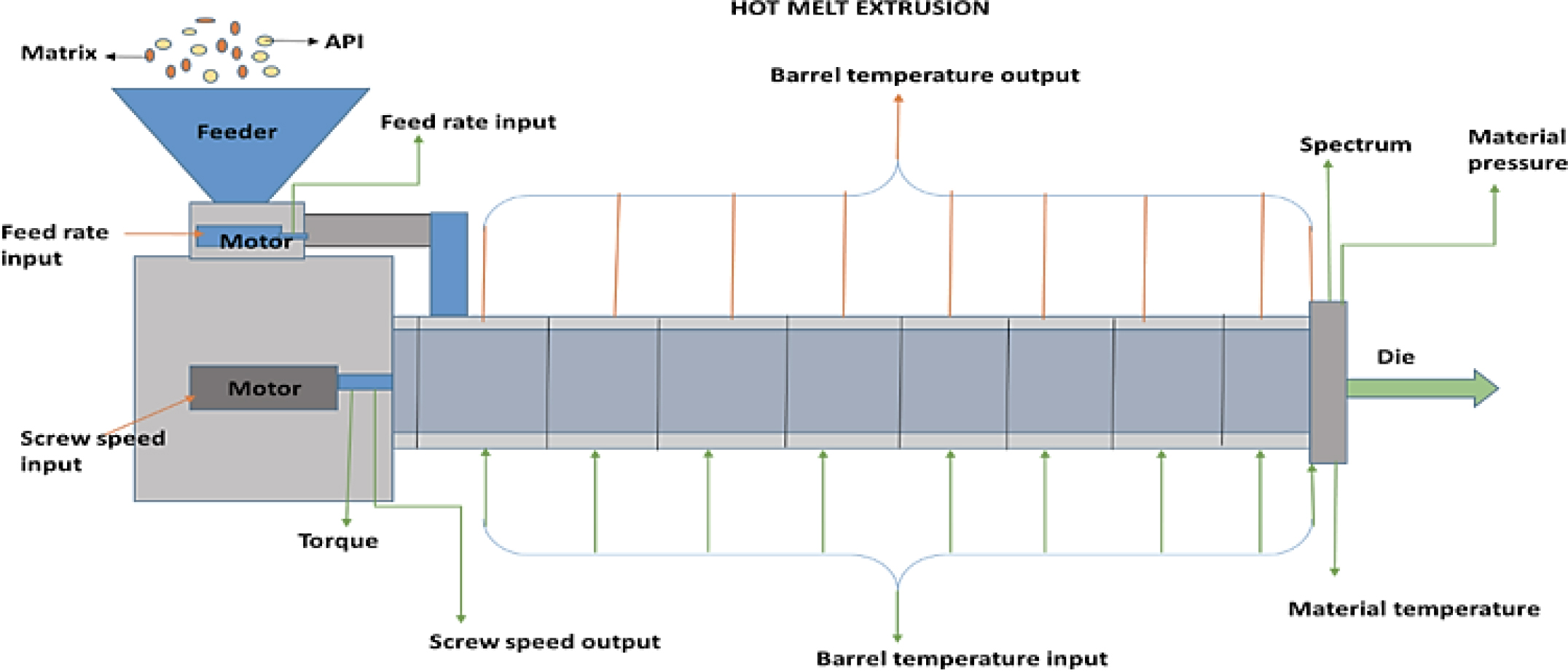
Figure 13:
Hot melt extrusion technique.
Advantages and limitation of GRDDs
Advantages
There are various advantages of GRDDs and some them are listed below:
Improved medication absorption, regulated drug administration and drug delivery for the stomach’s local action.
Because of the gradual and regulated pace, the mucosal irritation is reduced.
Excellent for treating gastrointestinal conditions including gastro-esophageal reflux.
For formulation, basic, everyday equipment is sufficient.
Drug delivery that is site specific (Rathodet al., 2016).
Treatment for gastrointestinal conditions such as Helicobacter pylori infection and GERD.
For medications with poor intestinal absorption, a floating drug delivery device is a practical solution.
The floating drug delivery technology can increase medication efficiency by lowering the body’s counteractivity.
Sustained release may cause flip-flop pharmacokinetics for medications with relatively short half-lives.
For medications that are absorbed through the stomach, the floating drug delivery devices are advantageous. For instance, ferrous salts, antacids, etc.
For medications with a short half-life, the sustained-release drug delivery method lowers the frequency of doses.
Despite the first pass impact caused by changes in plasma drug concentration, bioavailability improves; continual drug release maintains a necessary plasma drug concentration (Singhet al., 2012).
Limitation of GRDDs
The main drawback of floating systems is that they need a high enough stomach fluid content to administer the medication. The dose forms must be taken with at least 200-250 milliliters of water in a glass.
High mucus turnover rates in bioadhesive systems make the issue worse and persistent mucus adhesion causes discomfort (Aliet al., 2022).
The stomach’s mucous membrane is constantly renewing, which causes unpredictable adhesion
Since the length of time GRDDS remains in the stomach varies depending on the digestive state, it is introduced into the digestive system after a meal.
The person must be positioned upright for the medication to stay in the stomach.
The swelling mechanism based on hydrogel requires plenty of time to swell. Size-increasing drug delivery systems pose a risk to life after repeated doses because of the potential for long-term stomach retention. Among the disadvantages of super porous systems is the difficulty in storing highly hydrolyzable and biodegradable polymers (Porwalet al., 2017).
Clinical Applications of GRDDs
Gastro retentive drug delivery system (GRDDs) has many clinical applications as shown Figure 14, some of which are as followings:
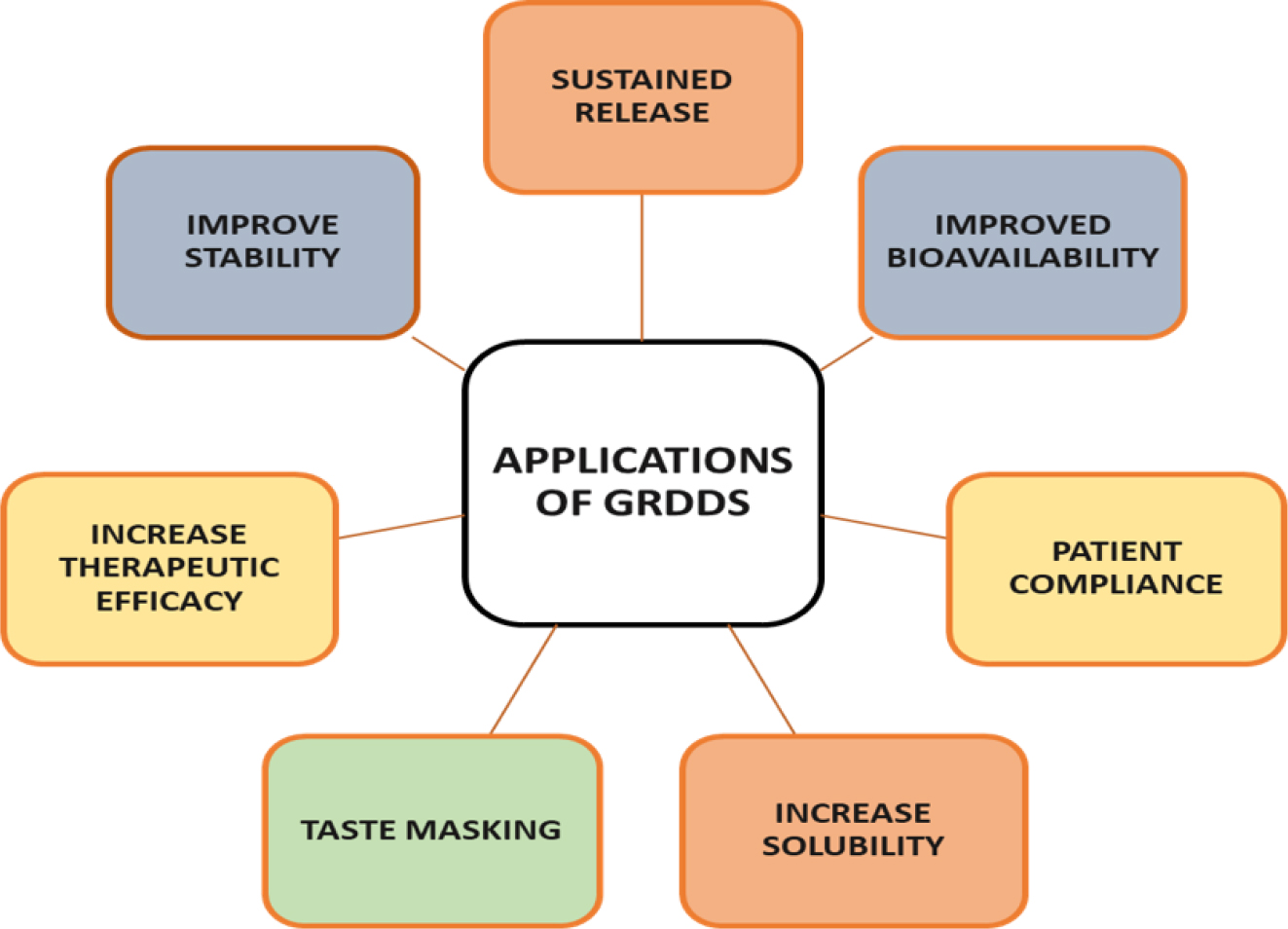
Figure 14:
Application of GRDDs.
Treatment of hypertension
According to the 2012 Health Statistics, one out of three persons had elevated blood pressure. Long-term care is necessary because this is turning into a chronic illness. Since several antihypertensive medications have:
A narrow window of absorption such as furosemide, atenolol and diltiazem
Short half-lives such as losartan and furosemide
Instability such as captopril at high pH values.
Low solubility such as verapamil, furosemide and propranolol at high Ph.
Colon degradation such as metoprolol.
GRDDS may be an appropriate choice for managing hypertension. By extending the stomach residence period, GRDDS targets site-specific release of drugs in the upper part of the digestive tract for either systemic or local effects. Various antihypertensive gsatrorentative medications, such as diazepam, misoprostol, propranolol, pep statin, L-dopa, benserazide and furosemide, increase the gastric residence time, bioavailability and, as a result, reduce the dose of the drug, dosing frequency and patient compliance. These site-specific administration of drugs decreases unwanted side effects of administered drug because it can minimize the body’s counteractivity, leading to higher drug efficiency (Zanke et al., 2022).
Treatment of H pylori infection
The idea of using nanoparticles as a gastroenterative medication delivery method was created in order to destroy Helicobacter pylori (H. pylori) colonies that had spread far into the stomach mucosal lining. Due to the dual ability of nanoparticles to adhere to surfaces and allow medications to enter the mucous layer. Heparin solution is added to a solution of chitosan with magnetic stirring at room temperature in order to create nanoparticles such as pH-responsive chitosan/heparin. An integrated medicine might be shielded from harmful stomach acids by the nanoparticles, which seemed to possess a particle size of 130-300 nm, a positive surface charge and stability at pH 1.2-2.5. Nanoparticles attached to and penetrate cell-cell junctions that are made and interact locally leading to greatly suppressing H. pylori infections (Garget al., 2014).
Treatment of gastric and duodenal ulcers
Peptic Ulcer Disease (PUD), sometimes referred to as a condition known as peptic ulcer, stomach ulcer, or gastric ulcer, is a highly common chronic illness of the stomach that is mostly brought on by damage or weakening of the stomach lining. The following Gastro Retentive Drug Delivery Systems (GRDDS) will be useful in gastro retention of different dosage forms for the treatment of peptic ulcers: floating, bioadhesive, high density, swellable, raft forming, superporous hydrogel and magnetic systems. GRDDS offers a way to regulate the release of substances that are taken up by the upper intestine’s active transport. For medications that are absorbed paracellularly, it also permits regulated distribution without compromising bioavailability (Senet al., 2023). Due to its exceptional gel-forming capacity to treat peptic ulcers, alginates may also be utilized to create gels that are appropriate for Gastro retentive applications. This is because alginate has anti-inflammatory and antioxidant qualities, as well as good stability in stomach pH. Because of alginate’s superior safety and biodegradability profile, researchers are working to create a Gastro retentive medication delivery method to treat peptic ulcers (Jakasaniyaet al., 2024).
Treatment of epilepsy
Although pregabalin is used to treat partial epilepsy, its absorption mostly takes place in the stomach and to a lesser amount in the upper gastrointestinal tract. For this reason, pregabalin is a good choice for the floating delivery system. Because they lengthen the drug’s stomach residence time, which releases the medication for a longer period of time and enhances its blood bioavailability, pregabalin floating tablets are designed and produced to treat epilepsy (Meenakshiet al., 2013).
Treatment of Diabetes
In comparison to current conventional and other controlled release dosage forms, GRDF provide us new and significant therapeutic possibilities for the delivery of anti-diabetic drugs since they not only extend dose intervals but also improve patient compliance. Because they offer continuous, regulated administration of these medications at the absorption site, GRDF are particularly useful in the delivery of sparingly soluble and insoluble medications. Many antidiabetic medications have the disadvantage of having a brief half-life in the stomach, despite being more effectively absorbed from the upper portion of the gastrointestinal system. certain disadvantages are mitigated by the Gastro retentive dose forms, which also boost the bioavailability of certain antidiabetic medications. For the administration of insulin and oral hypoglycemics, many Gastro retentive systems have been studied. Chlorpropamide (36-48 hr), Glibenclamide (18-24 hr), Glipizide (12-18 hr), Glimepiride (24 hr), Rosiglitazone (12-24 hr) and other medications with longer half-lives cannot be considered the best candidates for Gastro retentive systems. In contrast, medications like Metformin (6-8 hr), Phenformin (8-12 hr), Repaglinide (2-3 hr) and Nateglinide (2-3 hr) are the best candidates for the Gastro retentive strategy. These medications also have adequate stomach absorption, which is a crucial feature needed for a Gastro retentive strategy. The creation of Gastro retentive systems for medications like as metformin, glipizide, rosiglitazone and glyburide has involved the use of many techniques (Ahmedet al., 2017). some common marketed GRDDs are shown in Table 4.
Sl. No. | Active ingredient | Brand name | Drug class | Company name | References |
---|---|---|---|---|---|
1 | Misoprostal | Cytotec® | Synthetic prostaglandin E1 analog. | Pfizer Medical | (Khalafet al., 2023) |
2 | Ciprofloxacin | Cifran OD® | Antibiotic agent (fluoroquinolone class). | Sun pharma. | |
3 | Ofloxacin | Oflin OD® | Antibiotic agent (fluoroquinolone class). | Zydus Healthcare Limited. | |
4 | L-DOPA and Benserazid | Madopar® | Dopaminergic antiparkinsonism agent. | Abbott | |
5 | L-DOPA and Benserazide | Prolopa® | Dopaminergic antiparkinsonism agent. | Hoffmann-La Roche Limited. | |
6 | Propanolol | Ciplar LA ® | Beta-blockers | Cipla | (Shaikh et al., 2018) |
7 | Metprolol | Asoprol (25mg) ® | Beta-blockers. | A S Pharmaceutical (India) Pvt. Ltd. | |
8 | Verapamil HCl | Covera HS® | Beta-blockers. | DURECT Corporation. | |
9 | Atenolol | Tenormin (100 mg) ® | Beta blocker. | Abbott |
Marketed GRDDs formulations.
Evaluation of GRDDs
The evaluation of Gastro retentive drug system involves in vitro and in vivo assessment.
In vitro Assessments
Bulk density
It speaks about particle packing. Using a bulk density apparatus, bulk density of prepared granules was assessed. It may be expressed in gm/mL and is provided by the equation below:
Tapped density
Angle of repose
The angle of repose can be used to quantify the force of resistance forces during a loose powder or grains. This may be the maximum angle that may be formed between the horizontal plane and the surface of a pile of grains or powder. At a definitive height (h), the granules were allowed to flow between the funnel that was fixed to a stand. The crest and radius of the granule pile were then measured in order to determine the angle of repose.
Where h is the heap’s height, r is its radius, θ is the angle of repose and θ=tan-1 (h/r).
Compressibility index
By closely examining the powder’s bulk density and tapped density and therefore the pace at which it packs down, one may assess the powder’s flow ability.
In vitro drug release
A USP paddle-style dissolving assembly can be used for in vitro dissolution investigations. 900 mL of the dissolving medium is mixed with GRDDs products, such as floating tablets, microspheres and nanoparticles, equal to the drug dosage and agitated at 100 rpm at 37±0.5ºC. Samples are taken out at predetermined intervals and subjected to analysis using any appropriate analytical technique, including UV spectroscopy (Patelet al., 2010; Ramabargaviet al., 2013).
Solubility Studies
Since a medicine needs to have some restricted water solubility in order to produce a therapeutic response, it is crucial to understand its solubility properties in aqueous environments. The drug’s saturated solution was prepared in a constant volume at pH 1.2, buffers were added and the resultant solutions were stored at room temperature for 24 hr with intermittent shaking to determine the solubility quantitatively. U.V. spectrophotometry was used to filter and evaluate the resultant solutions for drug dissolution at a wavelength of 275 nm (λmax) (Gundaet al., 2015).
Friability
A Roche friabilator was used to assess the tablets’ friability. A sample of 20 tablets or tablets with a known weight (Wo) are dedusted in a drum for a predetermined amount of time (100 revolutions) and then weighed (W) once more. The weight loss was used to compute the percentage friability, as shown in the equation below, no more than 1% of the body weight should be lost (Kumaret al., 2013).
Weight variation
To conduct the USP weight variation test, 20 tablets are weighed separately, the average weight is determined and the weight of each tablet is compared to the average. If there are no more than 2 tablets that deviate from the % restriction or if no tablet varies by more than twice the percentage limit, the tablets pass the USP test.
Floating lag time and total floating time
The USP XXII paddle equipment was used to assess the produced formulations’ floating properties under sink circumstances. The investigation was conducted with a temperature of 37±0.5ºC and 90ºC of hydrochloric acid buffer pH 1.2 as the medium. The floating lag time-the amount of time needed for the tablet to float on the stomach fluid after being introduced-and the duration of floating-the amount of time that dosage forms stay buoyant-were measured. Visual inspection of the test tablets’ integrity was done throughout the investigation (Fouladiet al., 2012).
Release kinetics
Eqs. 1-4 illustrate how the release data was fitted to different kinetic models, including zero order and first order, in order to ascertain drug release kinetics
Where Qt is the percentage of drug release at time t and K0 is the release rate.
Where K1 is the release rate constant and Q is the percentage of medication release.
Where KH is the Higuchi release rate constant and Q is the percentage of drug release.
Where KHC is the Hixon-Crowell rate constant and Q is the percentage of drug release. Furthermore, as shown in Eq. 5, the medication release mechanism of the matrix tablet devices was ascertained using a straightforward semi-empirical model (Korsemeyer-Peppas).
where n is the release exponent that indicates the drug release mechanism, Qt is the amount of the released drug at time t, Q∞ is the total amount of drug (the whole dosage) and kkp is the constant that incorporates the structural and geometric features of the controlled release device.
For tablets and other cylindrical geometries, Fickian diffusion is represented by n=0.45, non-Fickian (anomalous) diffusion by 0.45<n<0.89 and case 2 diffusion by n=0.89. Only points within the 10-60% drug release range were utilized to fit data to the model, with the exception of the Higuchi model, which employed a range of 10-70% (Kumaret al., 2013; Fouladiet al., 2012; Wanget al., 2003).
Determination of Drug content
The biomedical pharmaceutical, food and environmental industries have made extensive use of the Inductively Coupled Plasma Atomic Emission Spectrometer (ICP-AES) as a quantitative analysis, characterisation and quality control tool for multi-elemental assays. Compared to traditional absorbance techniques, this approach has a large dynamic range, good sensitivity and specificity. The accuracy, precision and efficiency of ICP-AES techniques have greatly increased with the addition of sample equipment (Parikhet al., 2008).
In vivo assessments
While there are a number of in vitro methods for assessing the gastro-retentive capabilities of dosage forms, in vivo methods are thought to be the most trustworthy. Furthermore, any novel GRDDS would have a better chance of receiving a patent or registering with the FDA if it included in vivo assessment data. As a result, many in vivo methods are discussed for researching the gastro-retentive capabilities of dose forms. Ultrasonography, g-Scintigraphy, Gastroscopy, Roentgenography and Magnetic Marker Monitoring (Wasiullahet al., 2022).
Regulatory considerations for Gastro retentive products
Good Manufacturing Practises (GMP) and current Good Manufacturing Practises (cGMP)
The authenticity, purity and contamination-free nature of the raw materials used in the production of gastroentative medications are guaranteed by the Good Manufacturing Practices and current good manufacturing practices. The manufacturing procedure follows the guidelines set out to uphold the requirements. Sufficient steps for quality control are implemented. The produced medication has the required quality when it is put on the market. In order to accomplish the aforementioned goals, each licensee develops methods and procedures for adhering to the recommended medication manufacturing process, which ought to be recorded in a manual and retained for review and reference (Chowet al., 1998).
Regulatory approval process
Drug sponsors must provide the FDA with considerable evidence of safety and efficacy gathered from sufficient and well monitored clinical studies in order for the FDA to evaluate and approve their products. The Code of Federal Regulations (CFR) contains the current rules for conducting clinical trials and for submitting, reviewing and approving clinical data for pharmaceutical substances in the United States (see, for example, 21 CFR Parts 50, 56, 312 and 314). The FDA has the authority to administer drug product approval and regulation. These rules are applicable to investigational new drug applications, new drug applications, orphan pharmaceuticals, over-the-counter human medications and abbreviated new drug applications for generic medications (Rohillaet al., 2013).
Clinical trial
The World Health Organization defines a clinical trial as any research study that allocates people prospectively to one or more health-related treatments in order to assess the impact on health outcomes. It has become commonplace around the world to rely on clinical trial technique to produce scientific evidence about the efficacy of treatments for all chronic illnesses. Clinical trials may be separated into five different phases, which are trials in phases 0, I, II, III and IV. Drug trials have led to the characterization of the several stages of clinical trials, which is the focus of this article (Brodyet al., 2016).
Labeling and Packaging
Package labels must adhere to the CFR labeling requirements, which include safeguarding the public from harmful ingredients and false health claims. The fact that foods and dietary supplements do not need FDA approval prior to marketing, in contrast to prescription medications, presents a challenge with regard to the need to safeguard the public. For this reason, the FDA constantly checks marketed goods for illegal substances like stimulants, anabolic steroids and weight-loss medications. The FDA initiates most of its own monitoring, but it also receives complaints from doctors and customers (Alomaret al., 2020).
Post-Market Surveillance
The practice of monitoring marketed gastroentative pharmaceuticals for Adverse Drug Reactions (ADRs) following clinical trials is known as Post Marketing Surveillance (PMS). PMS studies are regarded as phase IV studies as the majority of medications might not be able to be sold until they have successfully completed phase III clinical trials. Rare Adverse Drug Reaction (ADRs) will only be partially revealed by clinical studies used to assess the safety and effectiveness of any new medication. Furthermore, finding “rare” (1 in 1000) and “very rare” (1 in 10,000) ADRs often only happens at the post-marketing stage (Suranaet al., 2010).
Recent advancement in GRDDs
Various methods have been proposed to increase the gastric residence of drug delivery systems in the upper part of the gastrointestinal tract, including Floating Drug Dosage Systems (FDDS), swelling or expanding systems, mucoadhesive systems, magnetic systems, modified-shape systems, high density systems and other delayed gastric emptying devices. In recent years, scientific and technological advancements have been made in the research and development of Gastro retentive drug delivery systems, which involve the use of novel and copolymers, multicomponent systems,3d printing technology and biodegradable materials that easily degrade in the body without causing any side effects (Klausneret al., 2003).
Copolymers
Copolymers are chains of polymers made up of two or more different monomer units. Copolymers where the sequencing of the monomer units is controlled synthetically are of special interest. Diblock copolymers, which are two separate homopolymer chains covalently bonded to one another at a single location, represent one extreme. Copolymers have been used extensively in the production of Gastro retentive drug delivery systems in recent years because they provide controlled, adjustable and targeted drug release (Moraet al., 2024).
3D printing technology
Using a layer-by-layer technique, three-Dimensional (3D) printing technology can produce tangible 3D things. With the use of Computer-Aided Design (CAD) software, 3D printing technology allows for the on-demand creation of nearly any size and form of object. 3D printing technology makes it possible to generate a large range of dose forms in the pharmaceutical industry. By printing many active pharmacological components and creating various geometries, it has been used to create oral solid dosage forms with varying release characteristics. By changing the dosage form’s structure, 3D printing technology may readily adjust the medication release. Complex interior features including internal walls, hollow channels, holes, numerous material regions and different medication distributions may all be handled by this technique. This enables the production of different drug delivery systems, controlled release, delayed release and fast release systems based on structural designs that are not feasible with conventional pharmaceutical manufacturing procedures. With the help of this technology, complex formulations like combined dosage forms with mixed release kinetics can be prepared in a new way. This can result in customized on-demand dosage forms that have an exact and adjustable dose to match the needs and characteristics of each patient, improving therapeutic results and patient compliance (Zhanget al., 2013).
Biodegradable materials
For medication delivery, a wide range of biodegradable material-from synthetic to natural and hybrid-have been researched and created; several of these are included in this theme issue and recent reviews. Another type of biodegradable polymers with successful clinical use as DDS is polyanhydride. Polyanhydrides are made to degrade on the surface in order to combat polyesters’ undesired bulk-degrading tendency. Because the rate of hydrolysis at the surface is far quicker than the rate at which water penetrates the sample’s core, the hydrolytically labile anhydride linkage, when combined with a hydrophobic backbone, limits biodegradation at the surface (Adebisi et al., 2010).
Recent patents of GRDDs
In the recent time various patents are filed and obtain in various formulation of Gastro retentive dosage forms, some of them are:
- Osmotic Gastro retentive Drug Delivery System with Self-Regulation.
- A Gastro retentive item for detecting alcohol.
- 3D printing of various gastric retentive drug delivery system dosage forms.
- Cannabinoids in a low-density system.
- Alcohol and acetaldehyde dehydrogenase-containing stomach retention sustained-release preparation and its manufacturing process.
- Long-term gastric retention with an ultralong floating hydrogel raft (Anothraet al., 2023).
Future prospective of GRDDs
One of the biggest problems facing the pharmaceutical business is the GRT of the traditional dose form, particularly for medications that are absorbed from the upper intestine. Although further research is required to address its flaws, the development of GRDDS will aid in overcoming the disadvantages of the traditional dosage form. Numerous research on GRDDS, including those involving floating, expandable and mucoadhesive systems, have been conducted thus far using the single system method GRDDS has bright prospects for the future. There are several methods that GRDDS can be utilized to enhance medication distribution to the stomach with further study and advancement (Tebaldi et al., 2002).
Smart Polymers
Stimuli-responsive polymers, often referred to as smart polymers, are substances that may alter their characteristics in reaction to outside stimuli like light, pH, temperature, or electric fields. Drug delivery systems made of smart polymers can release medications in reaction to particular triggers, including variations in the body’s pH or temperature (Jain et al., 2016).
Personalize medicine
To put it simply, personalized medicine is the practice of prescribing pharmacogenetic and pharmacogenomic information to determine which specific treatments are most appropriate for a certain individual. A review is given on the foundations of customized medicine. Numerous technologies are employed, such as genotyping single nucleotide polymorphisms. proteomics, haplotyping and gene expression research using biochips and microarrays. In the future of customized medicine, when diagnosis and treatment are combined, molecular diagnostics will be crucial. Genotype-based selection of patients for successful cancer therapy is one example of the customized medical strategy, which aims to avoid individuals who might not respond or experience unwanted side effects. Because it will shorten the medication development cycle and lower expenses, personalized medicine is commercially viable (Voraet al., 2023).
Artificial intelligence and machine learning
Drug delivery systems are being revolutionized by AI, which makes tailored, adaptive and targeted treatments possible. Pharma researchers and medical professionals may increase medication efficacy, reduce side effects and improve patient outcomes by utilizing AI’s skills in data analysis, pattern identification and optimization. Pharmacokinetics and pharmacodynamics have undergone a revolution thanks to AI-based techniques. Compared to conventional experimental techniques, they provide a number of benefits. Predicting pharmacokinetic characteristics, simulating drug distribution and clearance in the body and optimizing medication dose and delivery routes are all possible using AI-based models. Artificial Intelligence (AI)-based computational techniques for PBPK models can streamline their creation and improve its parameters, negating the need for human clinical trials and animal research. Big data and Artificial Intelligence (AI) enable computational pharmaceutics, which transforms the medication distribution process by offering a more effective, economical and data-driven method. It makes it possible to optimize medication formulations, customize treatments, comply with regulations and lower risk, all of which eventually improve patient results and drug manufacturing procedures. All things considered, integrating AI technology has enormous potential to speed up medication development, enhance patient outcomes and transform the pharmaceutical sector, advancing its transition from era 4.0 to era 5.0 (Timko et al., 2011).
Remote activation
Remote activation of IN GRDDs allows for on-demand regulated release patterns, which may improve therapeutic efficacy and lower systemic toxicity. Recent years have seen the development of several novel materials that are sensitive to magnetic fields, ultrasonic, Near-Infrared (NIR) light, or visible light. This responsiveness may be remotely activated to offer variable scheduling and dosage magnitude control. Here, we examine triggerable materials, which may be nano- to macro-scale and are triggered by a variety of inputs (Kantak et al., 2013).
CONCLUSION
In the recent time various drugs are formulated as Gastro retentive drug delivery system with a motive of sustained and control release of the drug for the local and systemic effects. GRDDs contain immense promise for the future of the drug delivery. To produce a required Gastro retentive dosage, form various approaches has been used like the floating system, non-floating system and raft forming system among which the floating system is the best approach for increasing gastric residence time. However, there are many challenges and limitations that have to be overcome in order to produce drugs with a prolonged gastric residence time the recent advancement in this field like the use of correct drug molecules and excipients, the use of various natural polymers,3d printing, biodegradable materials improve the GRDDs and in future various technologies like A.I. (Artificial Intelligence), 3D Technology, remote activation and various upcoming technologies will make it one of the most prominent way of drug administration.
Cite this article:
Mishra S, Shukla P, Tiwari R. Exploring the Potential of Gastro Retentive Drug Delivery Systems: An Insightful Perspective. Int. J. Pharm. Investigation. 2025;15(3):313-24.
REFERENCES
- A Zanke, A. A., H Gangurde, H. H., B Ghonge, A. B., & S. Chavan, P. (2022). Recent advance in gastro retentive drug delivery system (GRDDS). Asian Journal of Pharmaceutical Research, 12(2), 143–149. https://doi.org/10.52711/2231-5691.2022.00022
- Adebisi, A., & Conway, B. R. (2011). Gastroretentive microparticles for drug delivery applications. Journal of Microencapsulation, 28(8), 689–708. https://doi.org/10.3109/02652048.2011.590613
- Adibkia, K., Ghanbarzadeh, S., Mohammadi, G. et al. (2013). Gastro retentive drug delivery systems: A review. JRPS, 2(2), 190–204.
- Ahmed, M. G. (2017). Development and in vitro evaluation of rosuvastatin tablets by floating drug delivery system. Asian Journal of Pharmaceutics, 11(2). https://doi.org/10.22377/ajp.v11i02.1262
- Ali, M., & Manoj, Y. V. (2022). A scientific overview on gastro retentive drug delivery system. World Journal of Pharmaceutical Research, 11(4), 379–393. https://doi.org/10.20959/wjpr20224-23457
- Alomar, M., Tawfiq, A. M., Hassan, N., & Palaian, S. (2020). Post-marketing surveillance of suspected adverse drug reactions through spontaneous reporting: Current status, challenges and the future. Therapeutic Advances in Drug Safety, 11, Article 2042098620938595. https://doi.org/10.1177/2042098620938595
- Andrew, A. (2022). A review on raft-forming drug delivery system – Mechanism and its significance. Australas Med J. International Journal of Pharmacognosy. 2018;5(6). Shinde HA. In-situ raft forming system: a review, 15(2):336-7, 337–349.
- Anothra, P., Pradhan, D., Halder, J., Ghosh, G., & Rath, G. (2023). Gastroretentive drug delivery system in cancer chemotherapy. Current Drug Delivery, 20(5), 483–496. https://doi.org/10.2174/1567201819666220608141124
- Asane, G. S., Nirmal, S. A., Rasal, K. B., Naik, A. A., Mahadik, M. S., & Rao, Y. M. (2008). Polymers for mucoadhesive drug delivery system: A current status. DDIP. Drug Development and Industrial Pharmacy, 34(11), 1246–1266. https://doi.org/10.1080/03639040802026012
- Brody, T. (2016). Food and dietary supplement package labeling-guidance from FDA’s warning letters and Title 21 of the Code of Federal Regulations. Comprehensive Reviews in Food Science and Food Safety, 15(1), 92–129. https://doi.org/10.1111/1541-4337.12172
- C Shaikh, S. C., Sanap, D., Bhusari, D. V., Jain, S., Kochar, P. P., & Sanchati, V. N. (2018). Formulation and evaluation of ibuprofen gastro-retentive floating tablets. Universal Journal of Pharmaceutical Research, 3(4). https://doi.org/10.22270/ujpr.v3i4.178
- Chatterjee, B., Amalina, N., SenGupta, P. et al. (2017). Mucoadhesive polymers and their mode of action: A recent update. Journal of Applied Pharmaceutical Sciences, 7(5), 195–203.
- Chaudhari, K. D., Nimbalwar, M. G., Singhal, N. S., Wrushali A. Panchale, Jagdish V. Manwar, & Ravindra L. Bakal. (2021). Comprehensive review on characterizations and application of gastro-retentive floating drug delivery system. GSC Advanced Research and Reviews, 7(1), 35–44. https://doi.org/10.30574/gscarr.2021.7.1.0070
- Chawla, G., & Bansal, A. (2003). A means to address regional variability in intestinal drug absorption. Pharmaceutical Technology, 27(2), 50–68.
- Chen, H., Pan, L., Zhang, C., Liu, L., Tu, B., Liu, E., & Huang, Y. (2024). Gastroretentive raft forming system for enhancing therapeutic effect of drug-loaded hollow mesoporous silica on gastric ulcers. Advanced Healthcare Materials, 13(22), Article e2400566. https://doi.org/10.1002/adhm.202400566
- Chow, S.-C., & Pong, A. (1998). An overview of the regulatory approval process in drug development. Drug Information Journal, 32(1_suppl), 1175S–1185S. https://doi.org/10.1177/00928615980320S102
- Crowley, M. M., Zhang, F., Repka, M. A., Thumma, S., Upadhye, S. B., Battu, S. K., McGinity, J. W., & Martin, C. (2007). Pharmaceutical applications of hot-melt extrusion: Part I. Drug Development and Industrial Pharmacy, 33(9), 909–926. https://doi.org/10.1080/03639040701498759
- Fouladi, F., & Mortazavi, S. A. (2012). Preparation and in-vitro evaluation of gastro retentive bupropion hydrochloride tablets. Tropical Journal of Pharmaceutical Research, 11(3), 351–359. https://doi.org/10.4314/tjpr.v11i3.3
- Garg, T., Kumar, A., Rath, G., & Goyal, A. K. (2014). Gastro retentive drug delivery systems for therapeutic management of peptic ulcer. Critical Reviews in Therapeutic Drug Carrier Systems, 31(6), 531–557. https://doi.org/10.1615/CritRevTherDrugCarrierSyst.2014011104
- Gunda, R. K. (2015). Design, formulation and evaluation of atenolol gastro retentive floating tablets. Asian Journal of Pharmaceutics, 9(4), S34–S42. https://doi.org/10.22377/ajp.v9i4.484
- Hatwar, P. R., Bakal, R. L., Dere, M. D. et al. (2023). A review on: Gastro-retentive drug delivery system. WJPR, 12(12), 277–290.
- Jain, K. K. (2002). Personalized medicine. Current Opinion in Molecular Therapeutics, 4(6), 548–558.
- Jain, S., & Srinivasan, B. (2023). An insight on the strategical approach of gastro-retentive drug delivery systems, 3(2).
- Jakasaniya, P., Patel, J., Dudhat, K., & Mori, D. (2024). Formulation and optimization of gastro-retentive in situ gel of antiepileptic agent by using a Box-Behnken factorial design. Proceedings of the Indian National Science Academy, 1–4. https://doi.org/10.1007/s43538-024-00343-5
- Javaid, M. U., ul Ain, Q., Tahir, U. et al. (2017). A summarized review about natural polymers’ role in floating drug delivery systems and in-vivo evaluation studies. International Current Pharmaceutical Journal, 6(4), 23–26.
- Jeganath, S. (2022). Recent approaches of Gastro retentive drug delivery system – A review. Asian J Pharm, 16(1). https://doi.org/10.3390/pharmaceutics11040193
- Kantak, M. N., Kumar, L., Bhide, P. J., & Shirodkar, R. K. (2023). Oral gastroretentive film of lacidipine for the treatment of gastroparesis. Assay and Drug Development Technologies, 21(3), 97–109. https://doi.org/10.1089/adt.2022.091
- Kavitha, K., Kumar, M. R., & Singh, S. J. (2011). Novel mucoadhesive polymers: A review. Journal of Applied Pharmaceutical Sciences, 37–42.
- Khalaf, M. M., Alinejad, S. S., Sajad, O. et al. (2023). e1-e19. Journal of Population Therapeutics and Clinical Pharmacology, 30(5). https://doi.org/10.47750/jptcp.2023.30.05.001
- Klausner, E. A., Lavy, E., Friedman, M., & Hoffman, A. (2003). Expandable gastroretentive dosage forms. Journal of Controlled Release, 90(2), 143–162. https://doi.org/10.1016/s0168-3659(03)00203-7
- Kumar, S., Das, M., Gupta, K. S., Kumar, R. et al. (2013). Design, development, optimization and evaluation of gastro-retentive floating tablets of atenolol. Der Pharmacia Lettre, 5(3), 436–456.
- Kumar, V., Somkuwar, S., & Singhai, A. K. (2024). A recent update on gastro-retentive drug delivery systems. GSCBPS, 27(1), 125–144.
- Lee, B.-J., & Min, G.-H. (1995). Preparation and release characteristics of polymer-reinforced and coated alginate beads. Archives of Pharmacal Research, 18(3), 183–188. https://doi.org/10.1007/BF02979193
- Liao, D.-H., Zhao, J.-B., & Gregersen, H. (2009). Gastrointestinal tract modelling in health and disease. World Journal of Gastroenterology, 15(2), 169–176. https://doi.org/10.3748/wjg.15.169
- Lodh, H., Sheeba, F. R., Chourasia, P. K., Pardhe, H. A., & Pallavi, N. (2020). Floating drug delivery system: A brief review. Asian Journal of Pharmacy and Technology, 10(4), 255–264. https://doi.org/10.5958/2231-5713.2020.00043.4
- Mahmoud, D. B., & Schulz-Siegmund, M. (2023). Utilizing 4D printing to design smart gastroretentive, esophageal and intravesical drug delivery systems. Advanced Healthcare Materials, 12(10), Article e2202631. https://doi.org/10.1002/adhm.202202631
- Maniruzzaman, M., Boateng, J. S., Snowden, M. J., & Douroumis, D. (2012). A review of hot-melt extrusion: Process technology to pharmaceutical products. ISRN Pharmaceutics, 2012(1), Article 436763. https://doi.org/10.5402/2012/436763
- Mayur, C., Senthilkumaran, K., & Hemant, G. (2013). Super porous hydrogels: A recent advancement in gastro-retentive drug delivery system. Indones. J. Pharm., 24(1), 1–3.
- Meenakshi, P., & Naazneen, S. (2013). Gastro retentive drug delivery system-a novel approach for the management of diabetes mellitus. Inventi Rapid NDDS, 2, 1–7.
- Miyazaki, S., Yamaguchi, H., Yokouchi, C., Takada, M., & Hou, W. M. (1988). Sustained-release and intragastric-floating granules of indomethacin using chitosan in rabbits. Chemical & Pharmaceutical Bulletin, 36(10), 4033–4038. https://doi.org/10.1248/cpb.36.4033
- Mora-Castaño, G., Domínguez-Robles, J., Himawan, A. et al. (2024). Current trends in 3D printed gastro-retentive floating drug delivery systems: A comprehensive review. International Journal of Pharmacy, 633, Article 124543.
- Muaddi, H., Kearse, L., & Warner, S. (2024). Multimodal approaches to patient selection for pancreas cancer surgery. Current Oncology, 31(4), 2260–2273. https://doi.org/10.3390/curroncol31040167
- Mukund, J. Y., Kantilal, B. R., & Sudhakar, R. N. (2012). Floating microspheres: A review. Brazilian Journal of Pharmaceutical Sciences, 48(1), 17–30. https://doi.org/10.1590/S1984-82502012000100003
- Nagariya, A. K., Meena, A. K., Jain, D. et al. (2010). Potential of natural polymer in the gastro-retentive floating drug delivery system: A review. Journal of Pharmacy Research, 3, 916–922.
- Nitave, S. A., Patil, V. A., & Kagalkar, A. A. (2014). Review on gastro-retentive drug delivery system (GRDDS). International Journal of Pharmaceutical Sciences Review and Research, 27, 90–95.
- Nur, A., Fiskia, E., & Tjiroso, B. (2021). Evaluation profile in vitro release gastro-retentive high-density tablet theophylline using sodium alginate and PVP. E3S Web Conf. EDP Sci., 328 Article 01001.
- Nurhalifah, N., Sundawan, P. D., Veronita, S. C., Puji Destria, S. I., Nuryamah, S., & Yuniarsih, N. (2022). Literature review article: Drug delivery system held in the stomach (Gastro retentive). Journal of Social Research, 2(1), 126–133. https://doi.org/10.55324/josr.v2i1.472
- Parikh, D. C., & Amin, A. F. (2008). In vitro and in vivo techniques to assess the performance of gastro-retentive drug delivery systems: A review. Expert Opinion on Drug Delivery, 5(9), 951–965. https://doi.org/10.1517/17425247.5.9.951
- Patel, D. M., Patel, N. M., Patel, V. F., & Bhatt, D. A. (2007). Floating granules of ranitidine hydrochloride-gelucire 43/01: Formulation optimization using factorial design. AAPS PharmSciTech, 8(2), Article Article 30. https://doi.org/10.1208/pt0802030
- Patel, K., Patidar, D., & Sharma, N. (2022). A recent advantage on Gastro retentive drug delivery system: An overview. Journal of Pharmaceutical Negative Results, 13(Suppl. 10), 4521–4529. https://doi.org/10.47750/pnr.2022.13.S10.547
- Patel, S., Aundhia, C., Seth, A. et al. (2010). Microsponge: A novel approach in gastro-retention drug delivery system (GRDDS). Journal of Advanced Pharmaceutical Technology and Research, 1(3), 283–290. https://doi.org/10.4103/0110-5558.72416
- Patil, C., Baklilwal, S., Rane, B. et al. (2011). Floating microspheres: A promising approach for gastric retention. Int. J. Pharm. Res Dev., 2, 12.
- Porwal, A., Dwivedi, H., & Pathak, K. (2017). Decades of research in drug targeting using gastro retentive drug delivery systems for antihypertensive therapy. Brazilian Journal of Pharmaceutical Sciences, 53(3), Article e00173. https://doi.org/10.1590/s2175-97902017000300173
- Prajapati, V. D., Jani, G. K., Khutliwala, T. A., & Zala, B. S. (2013). Raft-forming system-An upcoming approach of gastro retentive drug delivery system. Journal of Controlled Release, 168(2), 151–165. https://doi.org/10.1016/j.jconrel.2013.02.028
- Pushpamalar, J., Meganathan, P., Tan, H. L., Dahlan, N. A., Ooi, L.-T., Neerooa, B. N. H. M., Essa, R. Z., Shameli, K., & Teow, S.-Y. (2021). Development of a polysaccharide-based hydrogel drug delivery system (DDS): An update. Gels, 7(4), 153. https://doi.org/10.3390/gels7040153
- Rajanikant, P., Nirav, P., Patel, N. M. et al. (2010). A novel approach for dissolution enhancement of ibuprofen by preparing floating granules. International Journal of Research in Pharmacy and Science, 1(1), 57–64.
- Ramabargavi, J. L., Pochaiah, B., Meher, C. et al. (2013). Formulation and in vitro evaluation of gastro retentive floating tablets of glipizide. Journal of Chemical and Pharmaceutical Research, 5(2), 82–96.
- Rangaraj, G., Kishore, N., Dhanalekshmi, U. M. et al. (2010). Design and study of formulation variables affecting drug loading and its release from alginate beads. Journal of Pharmaceutical Sciences and Research, 2(2), 77.
- Rashmitha, V., Pavani, S., & Rajani, T. (2020). An update on floating drug delivery system: A review. International Journal of Advances in Pharmacy and Biotechnology, 6(4), 9–18. https://doi.org/10.38111/ijapb.20200604003
- Rathod, H. J., Mehta, D. P., & Yadav, J. S. (2016). A review on gastro-retentive drug delivery systems. PharmaciaTutor, 4(7), 29–40.
- Raza, M., Jayswal, M. G., Ahmed, A. et al. (2022). A review on gastro-retentive drug delivery system. WJP Pharm. PharmSci, 11(9), 624–640.
- Rohilla, A., Singh, R. K., Sharma, D. et al. (2013). Phases of clinical trials: A review. Int. J. Pharm. Chem. Biol. Sci., 3(3), 700.
- Sarcan, E. T., Silindir-Gunay, M., & Ozer, A. Y. (2018). Theranostic polymeric nanoparticles for NIR imaging and photodynamic therapy. International Journal of Pharmaceutics, 551(1–2), 329–338. https://doi.org/10.1016/j.ijpharm.2018.09.019
- Sen, O., Manna, S., Nandi, G., Jana, S., & Jana, S. (2023). Recent advances in alginate-based gastro retentive technologies for drug delivery applications. Medicine in Novel Technology and Devices, 18, Article 100236. https://doi.org/10.1016/j.medntd.2023.100236
- Shah, S., & Pandya, S. (2010). A novel approach in gastro retentive drug delivery system: Floating drug delivery system. International Journal of Pharmaceutical Sciences and Research, 1(6), 7–18.
- Shaikh, R., Raj Singh, T. R., Garland, M. J., Woolfson, A. D., & Donnelly, R. F. (2011). Mucoadhesive drug delivery systems. Journal of Pharmacy and Bioallied Sciences, 3(1), 89–100. https://doi.org/10.4103/0975-7406.76478
- Singh, R. P., & Rathore, D. S. (2012);3. Gastro retention: A means to address local targeting in the gastric region. Pharmacophore, 6–2012, 287–300.
- Stillhart, C., Vučićević, K., Augustijns, P., Basit, A. W., Batchelor, H., Flanagan, T. R., Gesquiere, I., Greupink, R., Keszthelyi, D., Koskinen, M., Madla, C. M., Matthys, C., Miljuš, G., Mooij, M. G., Parrott, N., Ungell, A.-L., de Wildt, S. N., Orlu, M., Klein, S., & Müllertz, A. (2020). Impact of gastrointestinal physiology on drug absorption in special populations—An UNGAP review. European Journal of Pharmaceutical Sciences, 147, Article 105280. https://doi.org/10.1016/j.ejps.2020.105280
- Streubel, A., Siepmann, J., & Bodmeier, R. (2006). Gastro retentive drug delivery systems. Expert Opinion on Drug Delivery, 3(2), 217–233. https://doi.org/10.1517/17425247.3.2.217
- Subedi, G., Shrestha, A. K., & Shakya, S. (2016). Study of effect of different factors in formulation of micro and nanospheres with solvent evaporation technique. Open Pharmaceutical Sciences Journal, 3(1), 182–195. https://doi.org/10.2174/1874844901603010182
- Surana, A. S., & Kotecha, R. K. (2010). An overview on various approaches to oral controlled drug delivery system via gastro retention. International Journal of Pharmaceutical Sciences Review and Research, 2(2), 68–72.
- Tafish, A. M., Ebraheem, A. S., El Naggar, E. E. et al. (2023). Gastro retentive drug delivery systems: A summarized overview. ODR, 3(1), 40–56.
- Tariq, A., Bashir, I., Khan, K. I. et al. (2015). Structural components of gastro-retentive drug delivery systems. Am. J. Pharm. Res, 5(04).
- Tebaldi, M. L., Belardi, R. M., & Poletto, F. S. (2016). Smart polymers: Synthetic strategies, supramolecular morphologies and drug loading. In M. Hosseini, A. S. H. Makhlouf (Eds.), (pp. 147–164). Springer International Publishing. https://doi.org/10.1007/978-3-319-26893-4_7
- Timko, B. P., Dvir, T., & Kohane, D. S. (2010). Remotely triggerable drug delivery systems. Advanced Materials, 22(44), 4925–4943. https://doi.org/10.1002/adma.201002072
- Tripathi, J., Thapa, P., Maharjan, R., & Jeong, S. H. (2019). Current state and future perspectives on Gastro retentive drug delivery systems. Pharmaceutics, 11(4), 193. https://doi.org/10.3390/pharmaceutics11040193
- Turac, I.-R., Porfire, A., Iurian, S., Crișan, A. G., Casian, T., Iovanov, R., & Tomuță, I. (2024). Expanding the manufacturing approaches for gastroretentive drug delivery systems with 3D printing technology. Pharmaceutics, 16(6), 790. https://doi.org/10.3390/pharmaceutics16060790
- Vantimitta, S. R., & Jeganath, S. (2022). Novel approaches of gastro-retentive drug delivery system: A review. International Journal of Health Sciences, 6(Suppl. 1), 3464–3476. https://doi.org/10.53730/ijhs.v6nS1.5549
- VenKateswarlu, K., & Chandrasekhar, K. B. (2016). Development and statistical optimization of sustained release gastro-retentive floating tablets of cephalexin. Marmara Pharmaceutical Journal, 20(2), 172–183. https://doi.org/10.12991/mpj.20162070534
- Verma, P., Rezaei, L., Govindarajan, R., Greig, N. H., & Donovan, M. D. (2024). Gastroretentive delivery approach to address pH-dependent degradation of (+)- and (-)-phenserine. AAPS PharmSciTech, 25(7), 198. https://doi.org/10.1208/s12249-024-02903-w
- Vinchurkar, K., Sainy, J., Khan, M. A., Mane, S., Mishra, D. K., & Dixit, P. (2022). Features and facts of a Gastro retentive drug delivery system-a review. Turkish Journal of Pharmaceutical Sciences, 19(4), 476–487. https://doi.org/10.4274/tjps.galenos.2021.44959
- Vora, L. K., Gholap, A. D., Jetha, K., Thakur, R. R. S., Solanki, H. K., & Chavda, V. P. (2023). Artificial intelligence in pharmaceutical technology and drug delivery design. Pharmaceutics, 15(7), Article 1916. https://doi.org/10.3390/pharmaceutics15071916
- Wang, L., Marley, M., Jahansouz, H., & Bahnck, C. (2003). Determination of content uniformity and distribution characteristics of an investigational drug in its tablet dosage form and granule by ICP-AES. Journal of Pharmaceutical and Biomedical Analysis, 33(5), 955–961. https://doi.org/10.1016/s0731-7085(03)00364-9
- Waqar, M. A., Mubarak, N., Khan, A. M., Khan, R., Shaheen, F., & Shabbir, A. (2024). Advanced polymers and recent advancements on gastroretentive drug delivery system; a comprehensive review. Journal of Drug Targeting, 32(6), 655–671. https://doi.org/10.1080/1061186X.2024.2347366
- Wasiullah, M., Yadav, P., Yadav, P. et al. (2022). A review on role of GMP in pharmaceutical industry and their benefits. World Journal of Pharmaceutical Research, 12(1), 298–311. https://doi.org/10.20959/wjpr20231-26645
- Zhang, Y., Chan, H. F., & Leong, K. W. (2013). Advanced materials and processing for drug delivery: The past and the future. Advanced Drug Delivery Reviews, 65(1), 104–120. https://doi.org/10.1016/j.addr.2012.10.003