ABSTRACT
Background
Antibiotic-resistant bacteria pose a significant threat to public health, leading to the development of novel therapeutic approaches. Staphylococcus aureus, a common pathogenic microbe, is a major global health problem, with 21% to 30% of humans having been exposed to microorganisms for a long time.
Materials and Methods
To combat this, researchers have focused on developing novel antibiotics or alternative strategies. In silico techniques, such as computer simulations and molecular modelling, have been vital in the fight against antibiotic resistance and developing improved bacterial disease treatments.
Results and Discussion
Structure-based virtual screening of 48 compounds from ascidians of Indian coast against the ligand-binding domain of the α-hemolysin, Toxic Shock Syndrome Toxin-1 (TSST-1) and Panton-Valentine Leukocidin (PVL) enabled to identify a lead molecule, betulin, that exhibited a favourable binding mode and docking scores greater than -7 kcal/mol.
Conclusion
Antibacterial activity of Betulin was also confirmed with a maximum concentration of 15 mg/mL. Further studies are needed to validate its efficacy as a treatment for bacterial infections.
INTRODUCTION
The emergence of antibiotic-resistant bacteria creates an important threat to public health, making bacterial infections a major worldwide health problem (Uddinet al., 2021). To overcome these resistant microbes and ensure that patients receive excellent treatment, novel therapeutic approaches need to be established (Martens and Demain, 2017). Strict regulations and standards over the administration of antibiotics have been necessary for preventing the development of resistance (Leeet al., 2013).
The bacterium Staphylococcus aureus is frequently found in the skin and the upper respiratory system and was a part of the microbiome of the human body. It was a common pathogenic microbe that can lead to a variety of diseases, from minor infections of the skin to potentially fatal conditions like sepsis and pneumonia. There are currently continuous investigations being carried out; however, there are presently no authorized vaccines against S. aureus. A range of 21% to 30% of humans worldwide has been reported to have hosted the microorganism for a long period of time (Wikipedia Contributors, 2019). Due to the escalating antibiotic resistance of this illness, many standard antibiotics were becoming less effective, which makes prevention of the infection necessary. Research efforts have focused on developing novel antibiotics or alternative strategies for addressing S. aureus infections (Edward et al., 2023; Murugaiyanet al., 2022; Assefa and Alemayehu, 2023; Assiset al., 2017; Ghoshet al., 2019).
While anticipating the performance of potential medications, in silico techniques employ computer simulations, whereas in vitro approaches focus on testing these medications in a lab atmosphere (Wadoodet al., 2013). Perhaps a comprehensive strategy would consist of screening massive databases of substances and estimating their possible effectiveness against Staphylococcus aureus by employing simulations on computers and molecular modelling (Shakeret al., 2021). These strategies have been vital to the battle with resistance to antibiotics and for the development of improved bacterial disease treatments, ultimately leading to more effective and targeted medications. The method mentioned above possesses a limitation, yet they may not always be able to anticipate precisely how the compound would interact against the bacteria, thus might end up in inaccurate positives or negatives (Mohd, 2023). Drug development for S. aureus infections might benefit from the use of in silico approaches, which can speed up the process of finding new remedies.
S. aureus develops Panton-Valentine Leukocidin (PVL), a pore-forming toxin that triggers infections in soft tissues, which include the skin. It focuses on white blood cells, which compromise the immune system and permit the bacteria to proliferate (Linaet al., 2024; Hodilleet al., 2020). It is necessary to fully understand PVL’s function to create efficient strategies for treatment and prevent the development of antibiotic-resistant bacteria. Based on current studies, PVL might be a contributor to serious illnesses such as sepsis and necrotizing pneumonia (Huanget al., 2020; Löffleret al., 2013).
One of the life-threatening exotoxins generated by S. aureus is called TSST-1 and it is linked to Toxic Shock Syndrome (TSS). It has been linked to menstrual and non-menstrual TSS cases, Kawasaki syndrome, atopic dermatitis and neonatal TSS-like Exanthematous disease. TSST-1 is a single-chain polypeptide lacking disulphide bonds and behaves as a superantigen, binding to MHC class II molecules and activating T cells. Mutagenesis of potential MHC class II and TCR binding sites is crucial in vaccine development strategies (Gampferet al., 2002).
Staphylococcal α-hemolysin is a β-barrel pore-forming toxin secreted by S. aureus. It is expressed as a water-soluble monomeric protein that binds to target membranes and forms membrane-inserted heptameric pores (Sugawaraet al., 2023). Cellular processes affected by α-hemolysin include membrane damage, disruption of permeability barriers and channel formation in target cells. This toxin converts into a transmembrane pore by assembling an oligomeric β-barrel structure. It is involved in serious pathologies of humans and animals, affecting cell metabolism and causing diseases. α-hemolysin selectively permeabilizes cells to small molecules while excluding large ones, allowing Ca2+ ions to enter the cell (Menestrinaet al., 2001).
It has been proved that most of the bioactive compounds isolated from tunicate species exhibited strong antibacterial and anticancer activity. Potential compounds isolated from the tunicates of Indian coast were screened against the Staphylococcus aureus toxin proteins, particularly α-hemolysin, Toxic Shock Syndrome Toxin-1 (TSST-1) and Panton-Valentine Leukocidin (PVL) (Inoshimaet al., 2011; Löffleret al., 2010; Dingeset al., 2000).
MATERIALS AND METHODS
Ligand Molecules
Ascidians were the source of the active compounds that had been retrieved from public scientific database (Sudhandra, 2016; Kaleemullah, 2019; Praba, 2022; Mohammed, 2018). Based on the synthetic accessibility and ADMET characteristics, roughly 48 compounds were used in this investigation (Naveenet al., 2024). Following that, the ligand molecules were retrieved via the database known as PubChem and an aggregate of twenty compounds have been chosen for further investigation in molecular docking. The ligand molecules have been imported into the open-babel tool, which was previously accessible in the Pyrx docking software (Bintari and Risandiansyah, 2019; Snowet al., 2016), with the intention of transforming file formats and minimizing ligand energy. The ligand molecules underwent modification earlier than had been ready for the investigation.
Receptor Molecules
The 3D structures of about three different pathogenic protein receptors of the pathogenic bacteria Staphylococcus aureus have been retrieved from the Protein Data Bank Database. These receptor molecules have been selected for docking studies because of their significant role in the pathophysiology of the microorganisms that served as the targets of the ligand molecules. Since there are many different types of virulent factors, we implemented the specific one that generated the toxin in this case. They are α-hemolysin PDB 7ahl (Songetal., 1996), Toxic Shock Syndrome Toxin-1 (TSST-1) PDB 1qil (Papageorgiouet al., 1996), and Panton-Valentine Leukocidin (PVL) PDB 1pvl (Pédelacqet al., 1999). Using the Biovia Discovery Studio Tool, the protein or receptor molecule were altered in a number of ways prior to docking, such as water molecules being eliminated, missing atoms being replaced and energy distribution.
Molecular Docking
Using the AutoDock Vina program associated with the PyRX software (Kondapuramet al., 2021), the molecular docking was carried out. Following the induction of both the ligand and receptor molecules into the program, blind docking was carried out by completely covering the receptor molecule using a grid box. Following the standard approach, molecular docking had been carried out (Shawetaet al., 2021).
Antibacterial activity
In accordance with the established methodology, the materials required to perform antibacterial property testing were developed. Finally, betulin, the molecule exhibiting the highest binding affinity, has been recommended to conduct further in vitro research based on the findings. A 10 mg/mL concentration of cefpodoxime has been generated using Dimethyl Sulfoxide (DMSO) as a positive control. For evaluation purposes, the betulin was disintegrated into DMSO to provide a range of dosages, notably 5, 10 and 15 mg/mL. A DMSO-only negative control has been used in this study. Tests on betulin’s antibacterial activity were carried out through the well diffusion technique (Bhat and Nalawade, 2016). Agar plates have been employed for cultivating the isolated Staphylococus aureus strains and the cultures were incubated for 24 hr at 37 degrees Celsius. The inhibitory zone diameters of betulin, cefpodoxime and the negative control have been measured and compared.
RESULTS AND DISCUSSION
Binding Affinity Scores
The lead compounds exhibited excellent affinity with all of S. aureus’s virulence factors. More than three-quarters of the lead compounds had scores between -3 and -5 kcal/mol, whereas the remaining compounds showed scores between -5.1 and -7.6 kcal/mol (Table 1). Amongst the three receptor molecules, betulin possessed the most powerful binding affinity score (Figure 1). With PVL, the affinity score of betulin was -7.4 kcal/mol; with TSST-1, it was -7 kcal/mol and with α-hemolysin, it was -7.1 kcal/mol. While betulin’s interaction with α-hemolysin generated two hydrogen bonds at THR A:155 and ASP A:227, as well as an alkyl interaction at LEU A:219, betulin’s interaction with PVL developed a hydrogen bond interaction at HIS A:211 and a Pi-sigma interaction at TYR A:210 and with TSST-1 a hydrogen bond interaction at GLN A:123, Pi-alkyl and Pi-sigma interactions at PHE A:56. The interaction between betulin and α-hemolysin revealed two hydrogen bonds, while betulin and PVL only had one hydrogen bond (Figures 2–4). However, betulin and PVL had a higher binding affinity score than betulin and α-hemolysin. These results suggest that betulin has potential as a therapeutic agent against bacterial toxins such as PVL, TSST-1 and α-hemolysin due to its favourable interactions with these molecules. Betulin and PVL possessed an overall higher binding affinity, which would indicate a more potent inhibitory impact on toxicity triggered by PVL. Betulin’s versatility to become a feasible therapy remedy for bacterial toxin-mediated illnesses has been demonstrated by the broad range of interactions it has between different receptors. Subsequent in vitro studies might provide more insight into the effective use of betulin as a therapeutic medication.
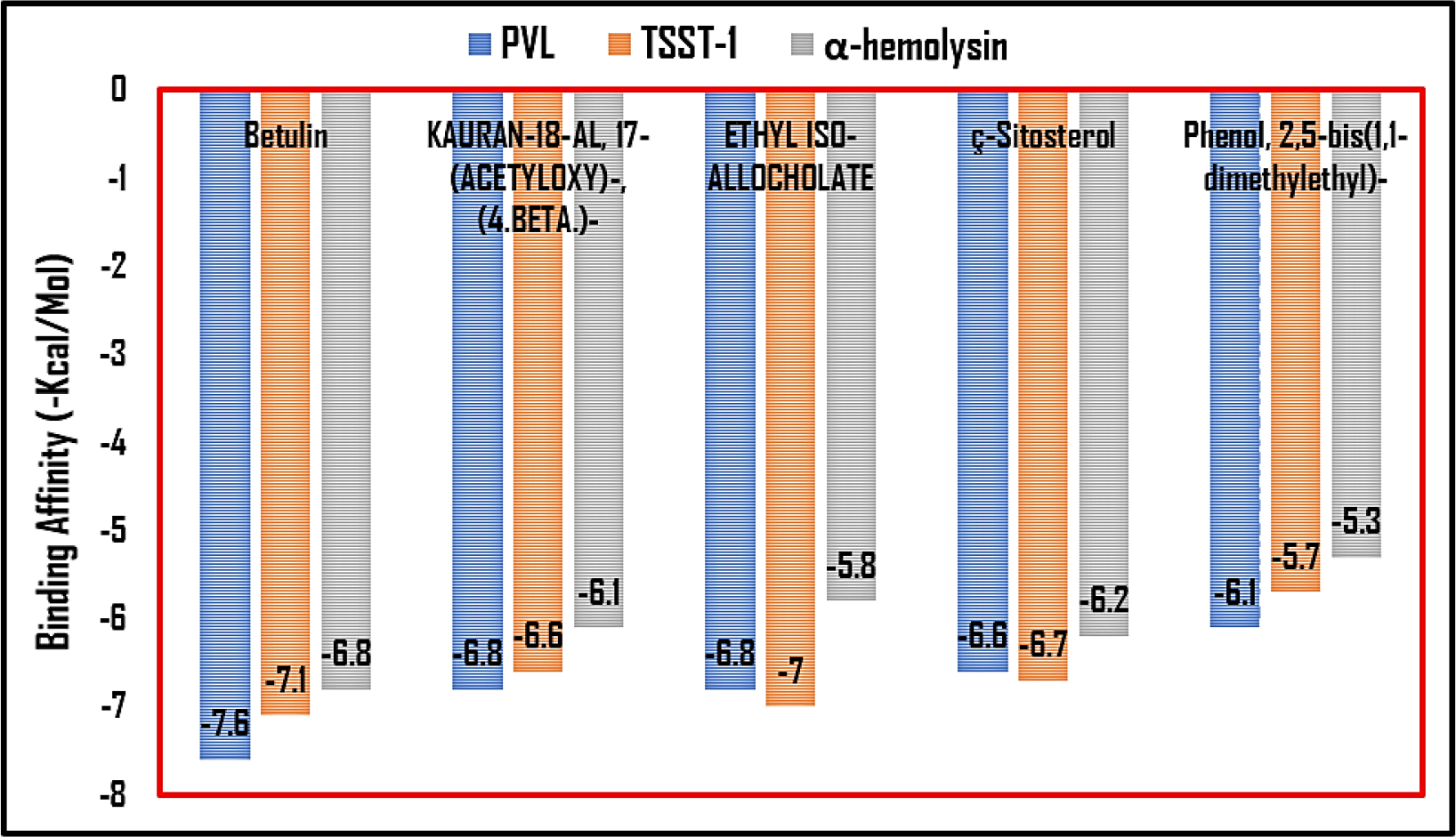
Figure 1:
Bar graph illustrating the leading lead compounds against virulence receptors based on their binding affinity score.
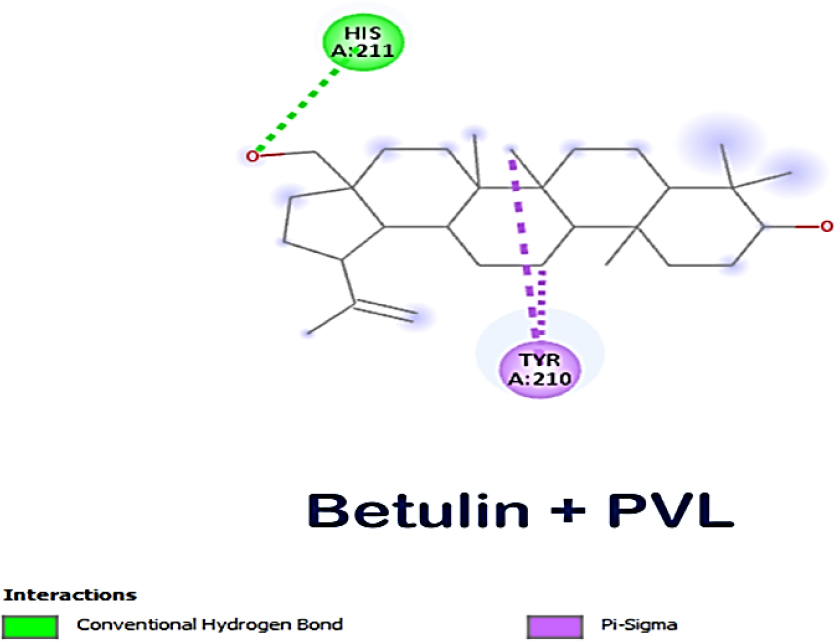
Figure 2:
Molecular interaction between betulin and PVL.
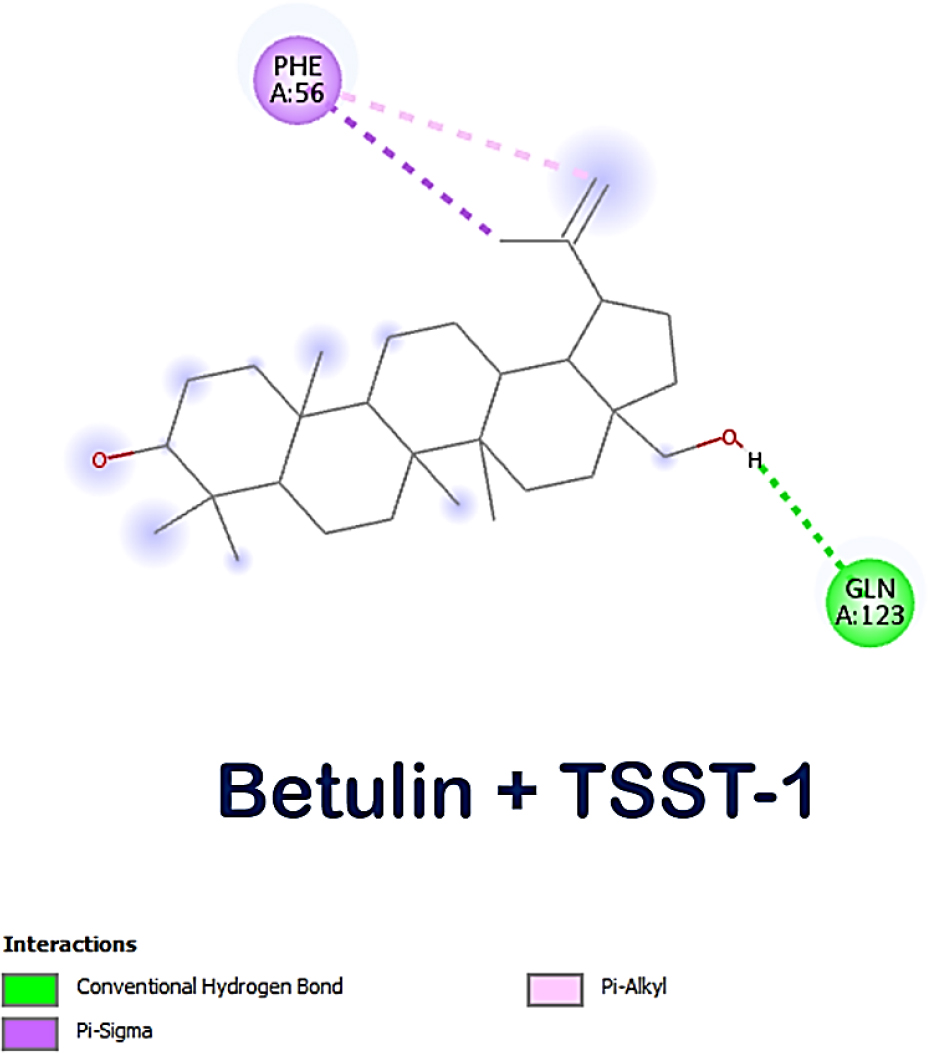
Figure 3:
Molecular interaction between betulin and TSST-1.
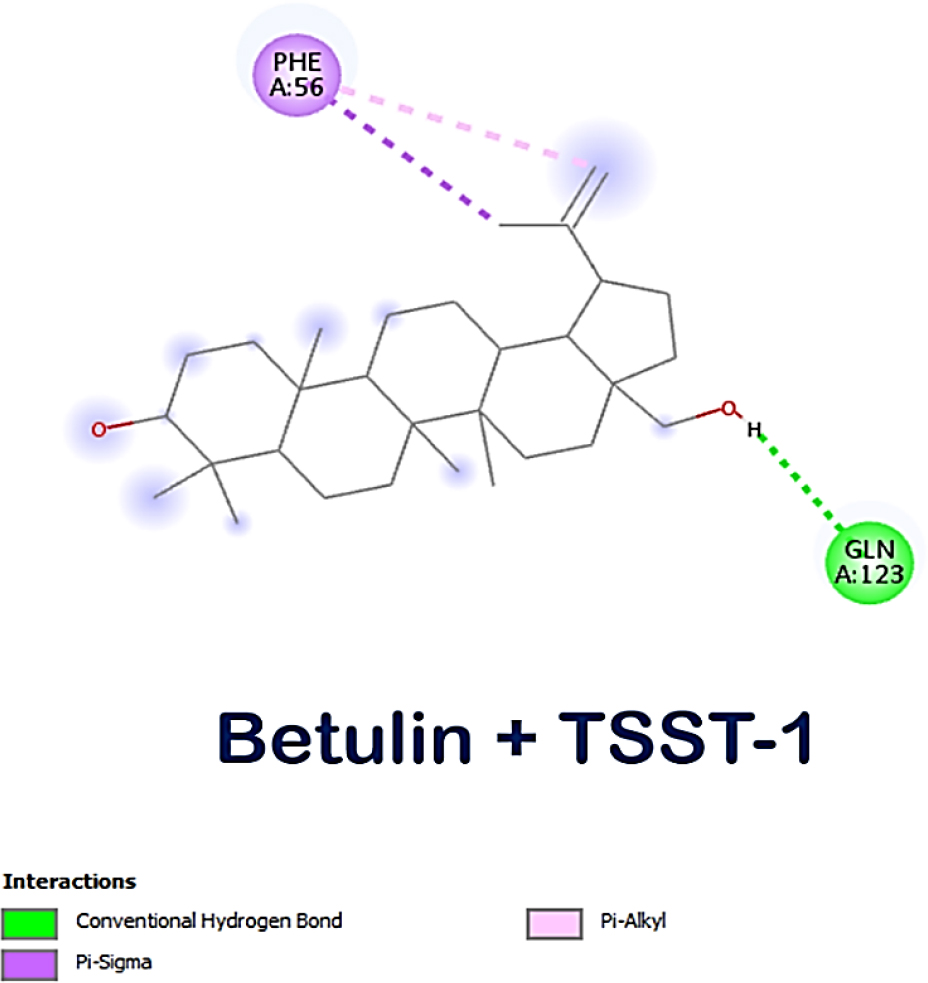
Figure 4:
Molecular interaction between betulin and α-hemolysin.
Sl. No. | Name of the molecule | Binding Affinity | ||
---|---|---|---|---|
PVL | TSST-1 | α-hemolysin | ||
1 | Betulin | -7.6 | -7.1 | -6.8 |
2 | KAURAN-18-AL, 17-(ACETYLOXY)-, (4.BETA.)- | -6.8 | -6.6 | -6.1 |
3 | ETHYL ISO-ALLOCHOLATE | -6.8 | -7 | -5.8 |
4 | ç-Sitosterol | -6.6 | -6.7 | -6.2 |
5 | Phenol, 2,5-bis (1,1- dimethylethyl)- | -6.1 | -5.7 | -5.3 |
6 | Humulene epoxide II | -5.9 | -6.2 | -5.5 |
7 | 1,2-Benzenedicarboxylic acid, mono (2-ethylhexyl) ester | -5.7 | -5.5 | -5.3 |
8 | 6,9,12-Octadecatrienoic acid, phenylmethyl ester, (Z,Z,Z)- | -5.6 | -4.4 | -3.9 |
9 | Spathulenol | -5.6 | -6.3 | -5.5 |
10 | Bicyclo3.1.1heptane-2,3-diol, 2,6,6-trimethyl (2,3-Pinanediol) | -5.6 | -5.6 | -5.1 |
11 | Phenol, 2,6-bis(1,1-dimethylethyl)-4-methy (BHT) | -5.5 | -5.7 | -5.2 |
12 | Benzoic acid, 4-ethoxy- ethyl ester | -5.4 | -4.5 | -4.5 |
13 | 1-HEXYL-2-NITROCYCLOHEXANE | -5.2 | -5.7 | -4.9 |
14 | (Z)-9-Octadecenamide | -5.1 | -3.7 | -3.5 |
15 | Didodecyl phthalate | -5 | -5 | -4.1 |
16 | Octadecane, 1-(ethenyloxy)- | -4.9 | -4.6 | -3.7 |
17 | Octadecanoic acid | -4.8 | -3.6 | -4.2 |
18 | OLEIC ACID | -4.7 | -4.7 | -4 |
19 | Acetic acid, trifluoro-, 2,2-dimethylpropyl ester | -4.6 | -5.3 | -4.5 |
20 | E-2-TETRADECEN-1-OL | -4.6 | -4.2 | -3.7 |
21 | 9-OCTADECENAL | -4.6 | -3.8 | -3.6 |
22 | Acetic acid, octadecyl ester | -4.4 | -3.7 | -3.2 |
23 | Tridecanoicacid, methyl ester | -4.4 | -3.8 | -3.1 |
24 | (R)-(-)-(Z)-14-Methyl-8-hexadecen-1-ol | -4.4 | -4.4 | -3.8 |
25 | 1-EICOSANOL | -4.4 | -3.9 | -3.5 |
26 | 1-HEXACOSANOL | -4.4 | -3.5 | -3.1 |
27 | DODECANAL | -4.4 | -4.8 | -3.8 |
28 | n-Hexadecanoic acid | -4.3 | -5.1 | -3.8 |
29 | 1-Tridecanol | -4.3 | -3.7 | -3.4 |
30 | Undecanoic acid | -4.3 | -4.1 | -3.9 |
31 | Myristic acid | -4.3 | -4.1 | -3.9 |
32 | Oleyl Alcohol | -4.2 | -4.7 | -3.3 |
33 | Trichloroacetic acid, hexadecyl ester | -4.1 | -4.5 | -3.9 |
34 | 1,2,3-Propanetriol, 1-acetate | -4.1 | -4 | -4.1 |
35 | Heptadecanoic acid | -4.1 | -5 | -3.6 |
36 | 1-Hexadecanol | -4 | -4.9 | -4.2 |
37 | 7-Hexadecenoic acid, methyl ester, (Z)- | -4 | -3.7 | -4.3 |
38 | NONADECANAMIDE | -4 | -3.6 | -4.1 |
39 | N-METHYLTAURINE | -3.9 | -3.6 | -4.4 |
40 | Oxirane, ((hexadecyloxy)methyl)- | -3.8 | -4.7 | -3.7 |
41 | Pentanoic acid, 10-undecenyl ester | -3.7 | -3.8 | -3.7 |
42 | OCTADECANAL | -3.7 | -4.2 | -3.5 |
43 | 1-HEPTATRIACOTANOL | -3.7 | -3.9 | -3.8 |
44 | OXIRANE, DODECYLO | -3.7 | -4.8 | -3.6 |
45 | 𝛼-D-Galactopyranoside, methyl | -3.7 | -5.3 | -5.5 |
46 | ERUCIC ACID | -3.6 | -3.8 | -4.8 |
47 | ISOPROPYL LINOLEATE | -3.4 | -4.5 | -3.6 |
48 | Dodecanoic acid | -3.4 | -4.1 | -4.2 |
Binding Affinity Score of Lead Compounds S. aureus virulence receptors.
Betulin may have the potential to be an antimicrobial towards the toxins produced by bacteria; however, it additionally has the capability to damage bacterial membrane integrity and interfere with the formation of biofilms (Guillaume et al., 2024), which further supports its potential as a therapy for bacterial infections. To completely understand betulin’s potential as a therapeutic choice, more study needs to be conducted to Figure out the mechanism by which it interfaces with bacterial toxins and inhibits their ability to function. Studies examining the cytotoxic effects of betulin on mammalian cells validated the drug’s safety and efficacy for clinical use (Zucoet al., 2002). Furthermore, investigating betulin’s possible synergistic effects with currently available antibiotics can provide valuable perspectives on combination therapy approaches for the treatment of bacterial infections. But it’s too early to make definitive opinions about betulin’s efficacy as a bacterial infection treatment until these clinical studies have concluded and reliable evidence has been acquired. Betulin may have some advantages, but it’s best to proceed cautiously and wait for more studies to validate its medicinal use.
Antibacterial activity
The outcomes disclosed that the betulin was having antibacterial activity; at the highest concentration of 15 mg/mL, it was 16 mm in diameter; at 10 mg/mL, it was 14 mm; and at 5 mg/mL, it was 12 mm (Figure 5). The zone of inhibition developed by the positive control against S. aureus had been 18 mm in diameter (Table 2). In this case, betulin was determined to suppress bacterial growth at various concentrations in a study assessing its antibacterial activity. Betulin exhibited a zone of inhibition against S. aureus that had been equivalent to the positive control at the highest tested dose. These results indisputably established that betulin possesses antimicrobial behaviour and it was discovered that its effectiveness corresponds precisely to the compound’s concentration. The results also show that betulin’s effectiveness in inhibiting bacterial growth is comparable to that of the positive control used in the experiment. Further research is needed to confirm and expand upon these findings, as they hold promise for the potential medicinal use of betulin in the future. In addition to its antimicrobial properties, betulin’s potential as a medicinal agent could also be explored in terms of its anti-inflammatory and antioxidant effects (Tuliet al., 2021). Previous studies have suggested that betulin may have the ability to reduce inflammation and oxidative stress in the body, which could have significant implications for the treatment of various diseases and conditions.
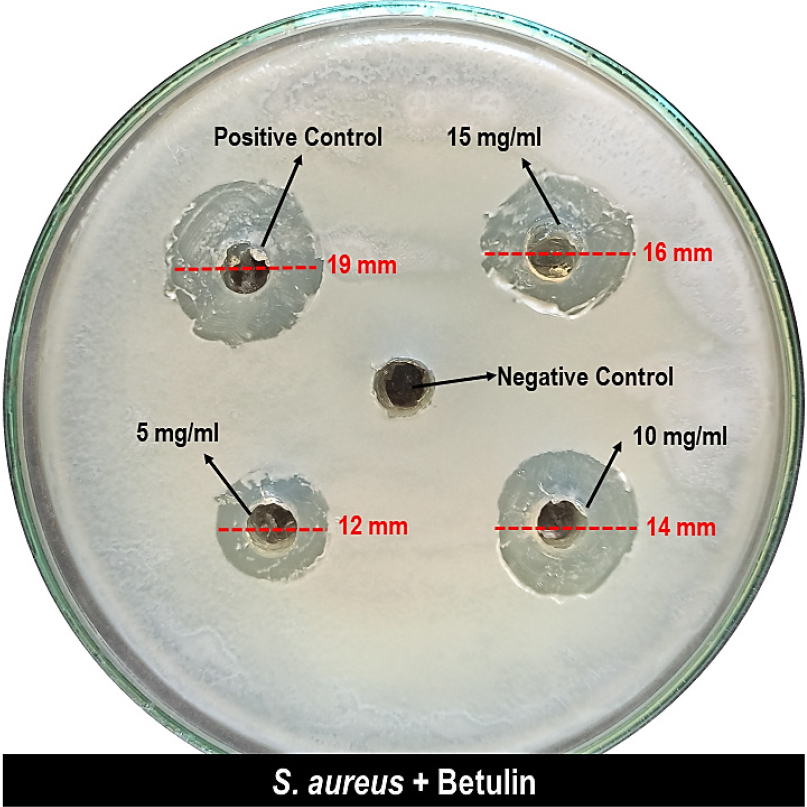
Figure 5:
Zone of inhibition of betulin at various concentrations against S. aureus.
Name of Pathogen | Zone of Inhibition (mm in diameter) | ||||
---|---|---|---|---|---|
Positive Control | Negative Control | 5 mg/mL | 10 mg/mL | 15 mg/mL | |
Staphylococcus aureus | 18 | 0 | 12 | 14 | 16 |
Betulin activity at various concentrations against S. aureus.
Further investigations into these possible therapeutic advantages may result in the creation of novel medications or therapies that optimise betulin’s potential for improved outcomes for patients. Furthermore, a comprehensive assessment of betulin’s safety and toxicity characteristics is required to ensure that it’s appropriate for usage in medicinal applications. All things considered; the findings of this study suggest the delightful potential of betulin as a simple therapeutic molecule with an abundance of possible implications for human health.
CONCLUSION
In conclusion, the results of this study suggest that betulin had the capability to effectively prevent the development of Staphylococcus aureus, a prevalent bacterium responsible for a variety of diseases in people. The noticeable zone of inhibition determined at 10 and 15 mg/mL doses reveals betulin’s potential antimicrobial properties. These outcomes encourage further investigations on betulin as a possible medication for infections caused by bacteria. It must be remembered to keep in mind that the investigation did not investigate the potential for betulin-causing bacteria to become resistant to this substance in the future, which could impact the ability of the medication to be effective as an antibacterial agent during the decades to come. However, these results may not be applicable to all Staphylococcus aureus strains, similarly, as bacterial sensitivity to betulin may vary depending upon genetic characteristics and other environmental factors.
Cite this article:
Mohan NK, Kumar M, Padmanaban A, Farhanaaz, Samad Z, Hajamohideen AJA. In silico Identification of Ascidian-Based Lead Compound with Antibacterial Activity for Staphylococcus aureus Toxin Proteins α-Hemolysin, TSST-1 and PVL. Int. J. Pharm. Investigation. 2025;15(3):313-24.
REFERENCES
- Abebe, A. A., & Birhanu, A. G. (2023, December 1). Methicillin resistant Staphylococcus aureus: Molecular Mechanisms Underlying Drug Resistance Development and Novel Strategies to Combat. Infection and Drug Resistance, 16, 7641–7662. https://doi.org/10.2147/IDR.S428103
- Assis, L. M., Nedeljković, M., & Dessen, A. (2017, March). New strategies for targeting and treatment of multi-drug resistant Staphylococcus aureus. Drug Resistance Updates: Reviews and Commentaries in Antimicrobial and Anticancer Chemotherapy, 31, 1–14. https://doi.org/10.1016/j.drup.2017.03.001
- Bintari, Y. R., & Risandiansyah, R. (2019, May 4). In silico Study to Assess antibacterial activity from Cladophora sp. on peptide deformylase: Molecular Docking Approach. Borneo Journal of Pharmacy, 2(1), 20–23. https://doi.org/10.33084/bjop.v2i1.717
- Dinges, M. M., Orwin, P. M., & Schlievert, P. M. (2000, January 1). Exotoxins of Staphylococcus aureus. Clinical Microbiology Reviews, 13(1), 16–34. https://doi.org/10.1128/CMR.13.1.16
- Douglas, E. J. A., Wulandari, S. W., Lovell, S. D., & Laabei, M. (2023, May 13). Novel antimicrobial strategies to treat multi‐drug resistant Staphylococcus aureus infections. Microbial Biotechnology, 16(7), 1456–1474. https://doi.org/10.1111/1751-7915.14268
- Gampfer, J., Thon, V., Gulle, H., Wolf, H. M., & Eibl, M. M. (2002, January). Double mutant and formaldehyde inactivated TSST-1 as vaccine candidates for TSST-1-induced toxic shock syndrome. Vaccine, 20(9–10), 1354–1364. https://doi.org/10.1016/s0264-410x(01)00470-4
- Ghosh, C., Sarkar, P., Issa, R., & Haldar, J. (2019, April). Alternatives to conventional antibiotics in the era of antimicrobial resistance. Trends in Microbiology [Internet], 27(4), 323–338. https://www.cell.com/trends/microbiology/fulltext/S0966-842X(18)30286-5#sec0110. https://doi.org/10.1016/j.tim.2018.12.010
- Hamion, G., Aucher, W., Mercier, A., Tewes, F., Menard, M., Bertaux, J., Girardot, M., & Imbert, C. (2024, April 1). Insights into betulinic acid as a promising molecule to fight the interkingdom biofilm Staphylococcus aureus–Candida albicans. International Journal of Antimicrobial Agents, 63(6), 107166–107166. https://doi.org/10.1016/j.ijantimicag.2024.107166
- Hodille, E., Plesa, A., Bourrelly, E., Belmont, L., Badiou, C., Lina, G., & Dumitrescu, O. (2020, November 20). Staphylococcal panton-valentine leucocidin and gamma haemolysin target and lyse mature bone marrow leucocytes. Toxins, 12(11), 725. https://doi.org/10.3390/toxins12110725
- Huang, J., Zhang, T., Zou, X., Wu, S., & Zhu, J. (2020). Pantonvalentine leucocidin carrying Staphylococcus aureus causing necrotizing pneumonia inactivates the JAK/STAT signaling pathway and increases the expression of inflammatory cytokines. Infection, Genetics and Evolution [Internet], 86, Article 104582. https://www.sciencedirect.com/science/article/pii/S1567134820304135. https://doi.org/10.1016/j.meegid.2020.104582
- Inoshima, I., Inoshima, N., Wilke, G. A., Powers, M. E., Frank, K. M., Wang, Y., & Bubeck Wardenburg, J. (2011, September 18). A Staphylococcus aureus pore-forming toxin subverts the activity of ADAM10 to cause lethal infection in mice. Nature Medicine, 17(10), 1310–1314. https://doi.org/10.1038/nm.2451
- Kaleemullah Khan, B. (2019). Molecular Taxonomy and Bioprospecting potential of selected Ascidians [Unpublished PhD thesis]. Thiruvalluvar University.
- Kondapuram, Karani, S., Sarvagalla, S., Selvaraj, C. M., Coumar, & Mohane, S. (2021). Chapter 22 Docking-based virtual screening using PyRx tool: Autophagy target Vps34 as a case study. Molecular Docking for Computer-aided Drug Design [Internet]. In Academic Press (pp. 463–477). https://www.sciencedirect.com/science/article/pii/B9780128223123000199
- Lee, C.-R., Cho, I. H., Jeong, B. C., & Lee, S. H. (2013, September 12). Strategies to minimize antibiotic resistance. International Journal of Environmental Research and Public Health [Internet], 10(9), 4274–4305. https://www.ncbi.nlm.nih.gov/pmc/articles/PMC3799537/. https://doi.org/10.3390/ijerph10094274
- Lina, G., Vandenesch, F., & Etienne, J. A brief history of Staphylococcus aureus Panton Valentine leucocidin [Internet]. Retrieved May 4, 2024, http://www.antimicrobe.org/h04c.files/history/PVL-long.pdf
- Löffler, B., Hussain, M., Grundmeier, M., Brück, M., Holzinger, D., Varga, G., Roth, J., Kahl, B. C., Proctor, R. A., & Peters, G. (2010, January 8). Staphylococcus aureus Panton-Valentine leukocidin is a very potent cytotoxic factor for human neutrophils. PLOS Pathogens A. Cheung (Ed.), 6(1), Article e1000715. https://doi.org/10.1371/journal.ppat.1000715
- Löffler, B., Niemann, S., Ehrhardt, C., Horn, D., Lanckohr, C., Lina, G., Ludwig, S., & Peters, G. (2013, October). Pathogenesis of Staphylococcus aureus necrotizing pneumonia: The role of PVL and an influenza coinfection. Expert Review of Anti-Infective Therapy, 11(10), 1041–1051. https://doi.org/10.1586/14787210.2013.827891
- Martens, E., & Demain, A. L. (2017, March 1). The antibiotic resistance crisis, with a focus on the United States. The Journal of Antibiotics [Internet], 70(5), 520–526. https://www.nature.com/articles/ja201730. https://doi.org/10.1038/ja.2017.30
- Menestrina, G., Dalla Serra, M. D., & Prévost, G. (2001, November). Mode of action of β-barrel pore-forming toxins of the staphylococcal α-hemolysin family. Toxicon, 39(11), 1661–1672. https://doi.org/10.1016/s0041-0101(01)00153-2
- Mohammed Kaleem Arshan ML. Studies on few Biotechnological Potential of chosen Ascidians from Mandapam Coast, India. (2018) [Unpublished PhD thesis]. Thiruvalluvar University.
- Mohd, Y. (2023, July 10). Insights into the in silico research: Current scenario, advantages, limits and future perspectives. Life in silico, 1(1), 13–25.
- Murugaiyan, J., Kumar, P. A., Rao, G. S., Iskandar, K., Hawser, S., Hays, J. P., Mohsen, Y., Adukkadukkam, S., Awuah, W. A., Jose, R. A. M., Sylvia, N., Nansubuga, E. P., Tilocca, B., Roncada, P., Roson-Calero, N., Moreno-Morales, J., Amin, R., Kumar, B. K., Kumar, A., van Dongen, M. B. M. (2022, February 4). Progress in alternative strategies to combat antimicrobial resistance: Focus on antibiotics. Antibiotics, 11(2), 200. https://doi.org/10.3390/antibiotics11020200
- Nalawade, T. M., Bhat, K. G., & Sogi, S. (2016). Antimicrobial activity of endodontic medicaments and vehicles using agar well diffusion method on facultative and obligate anaerobes. International Journal of Clinical Pediatric Dentistry, 9(4), 335–341. https://doi.org/10.5005/jp-journals-10005-1388
- Naveen Kumar, M., Abdul Jaffar Ali, H., Muneeswari, K., Amuthanila, P., & Farhanaaz, Z. S. (2024, August 15). In silico identification of ascidian based lead compounds against virulence proteins of Pseudomonas aeruginosa. African Journal of Biological Sciences [Internet], 6 (p. Si4). https://www.afjbs.com/uploads/paper/132ccb0dca7bc65609ce365fa98ec113.pdf
- Papageorgiou, A. C., Quinn, C. P., Beer, D., Brehm, R. D., Tranter, H. S., Bonventre, P. F., & Acharya, K. R. (1996, August). Crystal structure of a biologically inactive mutant of Toxic Shock Syndrome toxin-1 at 2.5 A resolution. Protein Science, 5(8), 1737–1741. https://doi.org/10.1002/pro.5560050826
- Pédelacq, J. D., Maveyraud, L., Prévost, G., Baba-Moussa, L., González, A., Courcelle, E., Shepard, W., Monteil, H., Samama, J. P., & Mourey, L. (1999, March 1). The structure of a Staphylococcus aureus leucocidin component (LukF-PV) reveals the fold of the water-soluble species of a family of transmembrane pore-forming toxins. Structure, 7(3), 277–287. https://doi.org/10.1016/s0969-2126(99)80038-0
- Praba, L. K. (2022). Ascidian derived bioactive agents and related pharmacological properties [Unpublished PhD thesis]. Thiruvalluvar University.
- Shaker, B., Ahmad, S., Lee, J., Jung, C., & Na, D. (2021, October). In silico methods and tools for drug discovery. Computers in Biology and Medicine [Internet], 137, Article 104851. https://pubmed.ncbi.nlm.nih.gov/34520990/. https://doi.org/10.1016/j.compbiomed.2021.104851
- Shaweta, S., Akhil, S., & Utsav, G. (2021, November 12). Molecular Docking studies on the anti-fungal activity of Allium sativum (Garlic) against Mucormycosis (black fungus) by BIOVIA discovery studio visualizer 21.1.0.0 21.1.0.0. Annals of Antivirals and Antiretrovirals, 28–32. https://doi.org/10.17352/aaa.000013
- Snow Setzer, M., Sharifi-Rad, J., & Setzer, W. N. (2016, September 12). The search for herbal antibiotics: An in silico Investigation of Antibacterial Phytochemicals. Antibiotics, 5(3), 30. https://doi.org/10.3390/antibiotics5030030
- Song, L., Hobaugh, M. R., Shustak, C., Cheley, S., Bayley, H., & Gouaux, J. E. (1996, December 13). Structure of Staphylococcal alpha -Hemolysin, a heptameric Transmembrane Pore. Science, 274(5294), 1859–1866. https://doi.org/10.1126/science.274.5294.1859
- Sudhandra Karthi, K. N. (2016). Efficacy of bioactive compounds from marine ascidians [Unpublished PhD thesis]. Madurai Kamarajar University.
- Sugawara, T., Yamashita, D., Kato, K., Peng, Z., Ueda, J., Kaneko, J., Kamio, Y., Tanaka, Y., & Yao, M. (2015, December 15) Retrieved October 10, 2023. Structural basis for pore-forming mechanism of staphylococcal α-hemolysin. Toxicon [Internet], 108, 226–231. https://www.sciencedirect.com/science/article/abs/pii/S0041010115300933?via%3Dihub. https://doi.org/10.1016/j.toxicon.2015.09.033
- Tuli, H. S., Sak, K., Gupta, D. S., Kaur, G., Aggarwal, D., Chaturvedi Parashar, N., Choudhary, R., Yerer, M. B., Kaur, J., Kumar, M., Garg, V. K., & Sethi, G. (2021, December 3). Anti-inflammatory and anticancer properties of birch bark-derived betulin: Recent developments. Plants, 10(12), 2663. https://doi.org/10.3390/plants10122663
- Uddin, T. M., Chakraborty, A. J., Khusro, A., Zidan, B. R. M., Mitra, S., Emran, T. B., Dhama, K., Ripon, M. K. H., Gajdács, M., Sahibzada, M. U. K., Hossain, M. J., & Koirala, N. (2021, October 23). Antibiotic Resistance in microbes: History, mechanisms, Therapeutic Strategies and Future Prospects. Journal of Infection and Public Health, 14(12), 1750–1766. https://doi.org/10.1016/j.jiph.2021.10.020
- Wadood, A., Ahmed, N., Shah, L., Ahmad, A., Hassan, H., & Shams, S. (2013, September). In silico drug design: An approach which revolutionarised the drug discovery process. OA Drug Design and Delivery, 1(1). https://doi.org/10.13172/2054-4057-1-1-1119
- Wikipedia contributors. Staphylococcus aureus [Internet]. (2019). Wikipedia. Wikimedia Foundation. https://en.wikipedia.org/wiki/Staphylococcus_aureus
- Zuco, V., Supino, R., Righetti, S. C., Cleris, L., Marchesi, E., Gambacorti-Passerini, C., & Formelli, F. (2002, January). Selective cytotoxicity of betulinic acid on tumor cell lines, but not on normal cells. Cancer Letters, 175(1), 17–25. https://doi.org/10.1016/s0304-3835(01)00718-2