ABSTRACT
Background
Cancer, particularly breast cancer, remains a major global health challenge, prompting ongoing research into novel therapeutic targets. This study focuses on Aurora A protein kinase, a serine/threonine kinase crucial for mitotic regulation, which is often overexpressed in various cancers, as well as breast cancer.
Materials and Methods
Recent study was aimed to explore the inhibitory potential of Duartin, a plant-derived isoflavonoid, against Aurora A protein kinase. Using molecular docking studies, Duartin demonstrated a high binding affinity with Aurora A, forming stable interactions with key residues, suggesting its potential as a strong inhibitor. ADMET predictions, along with PASS and Swiss Target Prediction analyses, confirmed Duartin’s favorable drug-like belongings, including effective absorption, distribution, metabolism, and low toxicity.
Results
In vitro assays on MDA-MB-231 breast cancer cells revealed significant cytotoxic effects of Duartin, by means of an IC50 value of 13.42 µM. Additionally, time-dependent cytotoxicity assays showed sustained reduction in cell viability over 48 hr. Furthermore, treatment with Duartin significantly reduced the mRNA expression levels of Aurora A protein kinase in these cells, indicating potent inhibitory effects on its gene expression. The findings suggest that Duartin not only exhibits strong binding affinity and favourable ADMET properties but also effectively reduces cell viability and Aurora A protein kinase expression in breast cancer cells.
Conclusion
The outcomes of this investigation highlights Duartin’s potential as a promising therapeutic agent for breast cancer, warranting further preclinical and clinical investigations.
INTRODUCTION
In 2020 alone, an estimated 19.3 million new cases and 10 million deaths from cancer were reported, making it one of the most serious health issues in the globe (Sunget al., 2021). Despite advancements in early detection, diagnostic techniques and treatment modalities, the high mortality rate and the significant burden of disease necessitate continuous exploration for novel therapeutic targets and strategies (Liuet al., 2024). One of the promising avenues in cancer therapy is the targeting of specific molecular pathways that are dysregulated in cancer cells, principal to uncontrolled proliferation and survival. Among these, protein kinases have emerged as critical regulators of cell signalling and are thus attractive targets for cancer therapy (Bhullaret al., 2018).
Aurora kinases, a family of serine/threonine kinases, show a essential role in the regulation of mitosis (Fuet al., 2007). They ensure proper chromosome alignment, segregation and cytokinesis, thus maintaining genomic stability (Roshanet al., 2023). This family comprises three members: Aurora A, Aurora B and Aurora C, each with distinct yet overlapping functions during cell division (Willemset al., 2018). Aurora Several crucial processes in mitosis, such as spindle formation, chromosomal alignment, and centrosome maturation and separation, depend on a kinase in particular (Magnaghi-Jaulinet al., 2019).
Overexpression and hyperactivation of Aurora A have been frequently observed in various cancers, including breast (Ingebriktsenet al., 2024), hepatocellular (Jenget al., 2004), esophageal squamous cell carcinoma (Wanget al., 2006), head and neck (Mehraet al., 2013), and pancreatic cancers (Zhanget al., 2022). This aberrant expression correlates with poor prognosis, increased tumor violence, and resistance to conventional therapies (Zhang et al., 2004; Zhenget al., 2023). As a result, Aurora A kinase has gained significant attention as a potential therapeutic aim in oncology.
Aurora A kinase is located on chromosome 20q13, a region generally amplified in cancers (Keen and Taylor, 2004; Kitzenet al., 2010; Senet al., 1997). Its overexpression is associated with aneuploidy and chromosomal instability, hallmark features of cancer cells (Takeshitaet al., 2013). Aurora A contributes to the malignant phenotype by disrupting normal mitotic processes, leading to abnormal centrosome function, spindle assembly defects and improper chromosome segregation (Goldensonet al., 2015; Sasaiet al., 2008). These aberrations result in genomic instability, a driving force behind cancer progression and metastasis (Torchiaet al., 2009). Moreover, Aurora A interacts with several key oncogenic and tumor suppressor pathways (Linet al., 2020). Aurora A also inactivates the tumor suppressor protein p53 by phosphorylation, leading to impaired DNA damage response and apoptosis (Katayamaet al., 2012). These interactions determine the role of Aurora A in cancer development and highlight its possible as a therapeutic target.
Targeting the Aurora A kinase presents a promising therapeutic strategy due to its central role in mitosis and cancer pathogenesis. Inhibitors of Aurora A aim to disrupt its kinase activity, thereby inducing mitotic defects, cell cycle arrest, and apoptosis in cancer cells. The development of Aurora A inhibitors, along with combination strategies and novel inhibitors with improved selectivity holds promise for enhancing therapeutic outcomes and overcoming resistance.
MATERIALS AND METHODS
The crystal structure of human Aurora A protein kinase was downloaded from RCSB PDB database, with PDB ID: 1MQ4 (Nowakowskiet al., 2002). The structure was prepared using a standard receptor protocol. Swiss-PDB Viewer tools were used to check and correct any structural issues in the protein molecule; such include erroneous bond arrangements or missing atoms, or unresolved residues. Further, energy minimization was performed to repair distorted geometries via moving atoms and releasing internal constraints on the protein structure. Hydrogen atoms and Kollman United Atom Charges were then additional to the structure. Finally, the structure was saved in. pdbqt format using MGLTools.
Retrieval and preparation of the ligand molecules
A small isoflavonoid library of 39 plant-derived natural compounds was curated and docked in contradiction of the target protein. The structures of these ligand molecules were downloaded in mol format and converted to the PDB format using Open Babel tools. Hydrogen atoms were then added to the ligand molecules using PyMOL. Swiss-PDB viewer was employed to carry out energy minimization and relax the ligand structure to its lowest energy conformation. The structure of each ligand molecule was verified for any missing atoms, incorrect bond orders, or other structural issues using molecular modeling software. Appropriate atomic charges were subsequently assigned to the ligand molecules. Finally, the optimized and corrected ligand structures were saved in PDBQT format using MGLTools, which is essential for docking using AutoDock Vina.
Molecular docking
We utilized AutoDock Vina for the molecular docking process, performing blind docking of ligand molecules with the Aurora A protein kinase (Trottet al., 2010). The grid dimensions for the X, Y and Z coordinates were set to 67, 83, and 88 Å, respectively, centered at -42.34, 16.21, and 71.13. The grid spacing was fixed at 1.00 Å with an exhaustiveness parameter of 8. Binding affinity was evaluated and the resulting docked complexes were visualized by means of PyMOL and Discovery Studio Visualizer to analyze the interaction patterns between the ligand molecules and the Aurora A protein kinase.
ADMET (Absorption, Distribution, Metabolism, Excretion, and Toxicity) characteristics of Duartin
To assess the ADMET characteristics of a ligand molecule, we utilized online tools such as SwissADME (http://www.swissadme.ch/index.php) and pkCSM (https://biosig.lab.uq.edu.au/pkcsm/). Integrating the results from both SwissADME and pkCSM provides a thorough understanding of the ADMET properties of the top hit.
Swiss Target Prediction tool and PASS online are used to predict Duartin’s activity spectrum
This study utilized PASS Online (http://www.pharmaexpert.ru/passonline/) and the Swiss Target Prediction tool (http://www.swisstargetprediction.ch/) to identify the activity spectrum of Duartin. PASS Online (Prediction of Activity Spectra for Substances) is a web-based platform that predicts the biological and pharmacological activities of chemical compounds, helping researchers identify potential activities and properties of small molecules. Swiss Target Prediction is an online program that assists in identifying proteins that might interact with a particular substance by predicting possible targets for small molecules or medications.
Test compound and MDA-MB-231 breast cancer cells
Duartin (>97.0% GC(T)) was obtained from MedKoo Biosciences, Inc. and liquefied in DMSO (SRL) to prepare a stock solution with a concentration of 500 µg/mL. MDA-MB-231 cells were sourced from NCCS, Pune and cultured in DMEM supplemented by means of 10% FBS and 1% antibiotics.
Cell culture and cytotoxicity assay
MDA-MB-231 cells were cultured in DMEM by 10% FBS and 1% penicillin-streptomycin at 37ºC with 5% CO2 until confluency. The cells were then seeded into 96-well plates at 5,000 cells/well and grown for 48 hr. They were treated by Duartin (0-1000 µM) for 72 hr, with control wells left untreated. Subsequently treatment, 100 µL of 5 mg/mL MTT solution was additional to each well and incubated at 37ºC for 3-4 hr. The MTT solution was substituted with 100 µL of DMSO to solubilize the formazan crystals, and absorbance was measured at 570 nm (reference at 630 nm). Cell viability (%) was considered as [Absorbance of treated cells/Absorbance of control cells] × 100. Additionally, a time-dependent viability assay was performed at 24, 48, and 72 hr using the IC50 concentration of Duartin.
mRNA expression of Aurora A protein kinase in Duartin treated MDA-MB-231 breast cancer cells
Breast cancer cells (MDA-MB-231) were treated with the IC50 concentration of Duartin for 48 hr. RNA was taken out using TRIzol Reagent (Thermo Fisher). cDNA was made by using cDNA Synthesis Kit (Almanac, Cat# ALS-MB72). The quantitative qRT-PCR was conducted consuming SYBR Green (Almanac, Cat# ALS-MB76) on an Applied Biosystems RT-PCR System. The 2-ΔΔCT method was working to calculate the relative fold change in gene expression. The primer sequence used for the amplifications was as: Aurora A protein kinase, forward: 5′-CCACCTTCGGCATCCTAATA-3′ and reverse: 5′-TCCAAGTGGTGCATATTCCA-3′ and GAPDH, forward: 5′-GAAGGTGAAGGTCGGAGTC-3′ and reverse: 5′-GAAGATGGTGATGGGATTTC-3′ (Wanget al., 2019).
RESULTS
The highest binding affinity was observed between Duartin and the Aurora A protein kinase. The binding affinity results are represented in Table 1 and Figures 1A-C. The interaction patterns of the top five hits are depicted in Figure 1C. Duartin formed several conventional hydrogen bonds with the LYS41 and ALA213 residues of Aurora A protein kinase. Additionally, Duartin formed various other interactions, as well as van der Waals, carbon hydrogen, Pi-sigma, alkyl, and Pi-alkyl bonds, with the LYS143, GLY142, GLY140, GLU260, THR217, GLY216, LEU139, PRO214, TYR212, VAL147, LEU263, LEU210, ALA160, LYS162 and ASP274 residues of Aurora A.
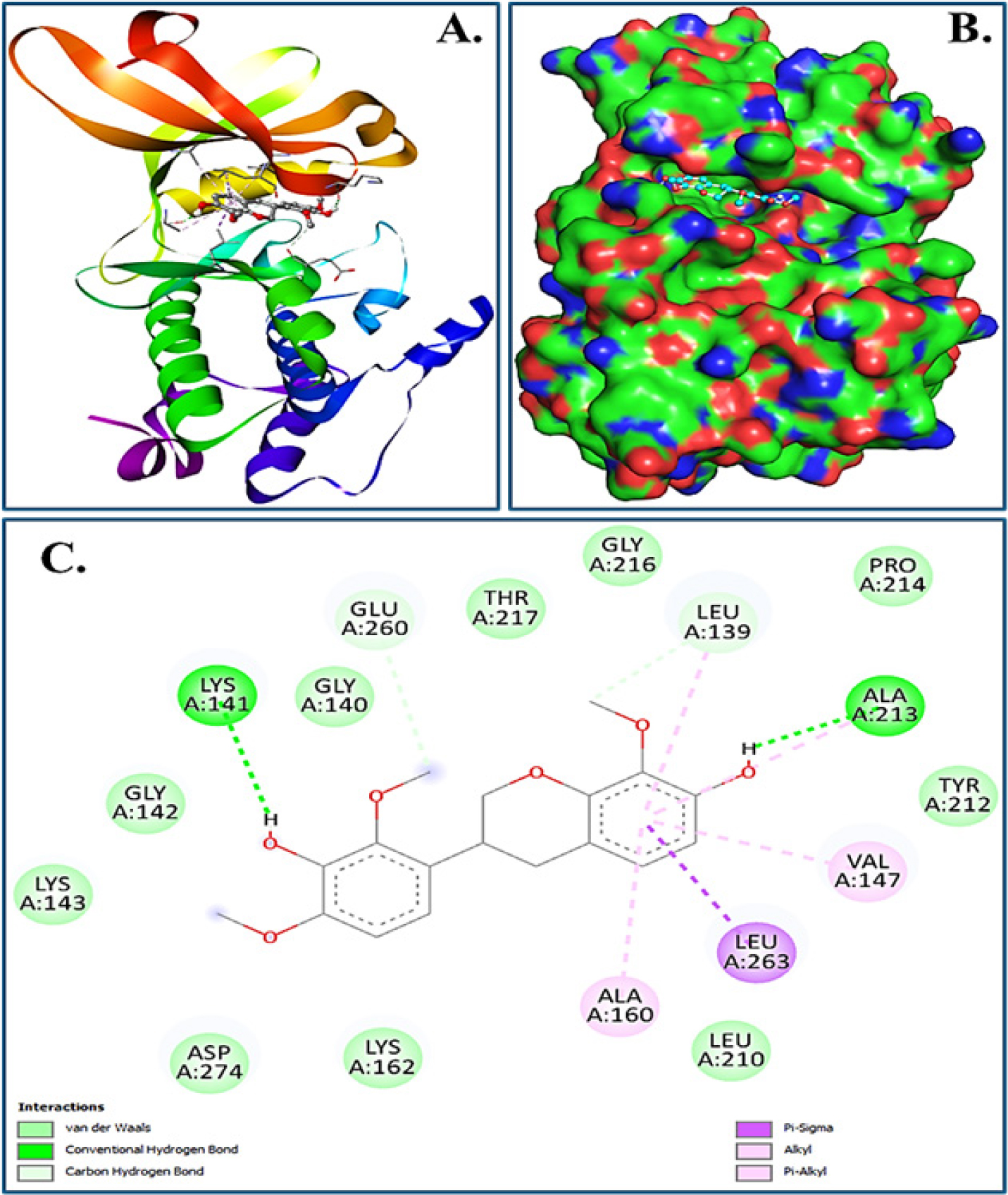
Figure 1:
Molecular docking of Duartin with Aurora A protein kinase. (A) Cartoon representation, (B) Surface view representing binding of Duartin within deep groove and (C) 2D docked complex showing interaction of Duartin with various amino acid residues of Aurora A protein kinase.
Name of the ligand | Target protein | Binding Energy (kcal/mol) | pKi | Target protein’s amino acids interacting with ligand molecules |
---|---|---|---|---|
Duartin | Aurora-A protein kinase. | Aurora-A protein kinase. | 5.94 | Conventional hydrogen bonds: LYS41, ALA213 van der Waals and other interactions: LYS143, GLY142, GLY140, GLU260, THR217, GLY216, LEU139, PRO214, TYR212, VAL147, LEU263, LEU210, ALA160, LYS162, ASP274. |
Binding affinity and interaction of Duartin with Aurora-A protein kinase.
ADMET predictions, PASS analysis and Swiss Target Prediction
ADMET estimates, PASS examination, and SwissTargetPrediction collectively encourage the drug-like characteristic of Duartin. The ADMET properties of Duartin, presented in Table 2, demonstrate favourable characteristics in terms of absorption, distribution, metabolism, excretion, and lack of toxicity. Furthermore, the outcomes of PASS Analysis and SwissTargetPrediction analysis, represented in Table 3 and Figure 2 respectively, not only confirm but also elaborate on the diverse biological properties associated with Duartin.
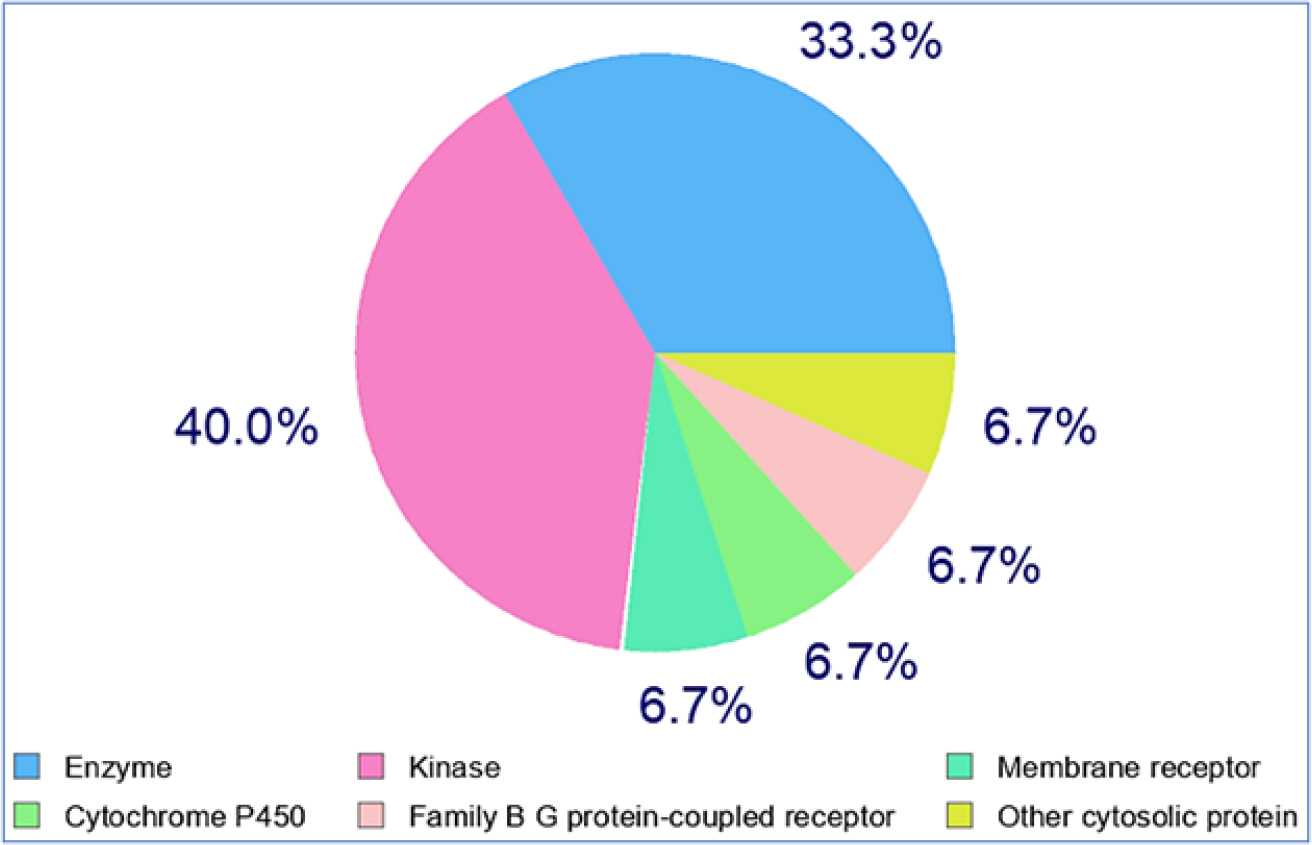
Figure 2:
SwissTargetPrediction analysis showing diverse biological properties linked to Duartin.
Properties | |||||||||||||
---|---|---|---|---|---|---|---|---|---|---|---|---|---|
Absorption | Distribution | Metabolism | Excretion | Toxicity | |||||||||
Models | Intestinal absorption (human) | VDss (human) | BBB permeability | CNS permeability | CYP | Total clearance | AMES toxicity/Hepatoxicity | ||||||
Substrate | Inhibitor | ||||||||||||
2D6 | 3A4 | 1A2 | 2C19 | 2C9 | 2D6 | 3A4 | |||||||
Unity | Numeric (% absorbed) | Numeric (log L/kg) | Numeric (Log BB) | Numeric (Log PS) | Categorical (yes/no) | Numeric (log mL/ min / kg) | Categorical (yes/no) | ||||||
Predicted values | |||||||||||||
Duartin | 91.013 | 0.059 | -0.525 | -2.987 | NO | Yes | Yes | Yes | Yes | No | Yes | 0.084 | No/No |
ADME and Tox characteristics for Duartin are predicted in silico.
Ligand | Structure | Pa | Pi | Activity |
---|---|---|---|---|
Duartin | 0,756 | 0,012 | JAK2 expression inhibitor. | |
0,743 | 0,011 | Apoptosis agonist. | ||
0,551 | 0,015 | Anticarcinogenic. | ||
0,531 | 0,062 | Antineoplastic. | ||
0,375 | 0,027 | Antineoplastic (lung cancer). | ||
0,392 | 0,070 | Kinase inhibitor. | ||
0,316 | 0,201 | Antineoplastic (non-Hodgkin’s lymphoma). | ||
0,289 | 0,030 | Antineoplastic (brain cancer) | ||
0,284 | 0,027 | Antineoplastic (lymphoma). | ||
0,289 | 0,052 | MAP kinase kinase 4 inhibitor. | ||
0,223 | 0,025 | Antineoplastic (carcinoma). | ||
0,202 | 0,061 | Antineoplastic (glioblastoma multiforme). | ||
0,188 | 0,040 | Antineoplastic (glioma). | ||
0,180 | 0,088 | Antileukemic. | ||
0,175 | 0,071 | Antineoplastic (melanoma). | ||
0,142 | 0,002 | Antimitotic, Podophyllotoxin-like. | ||
0,108 | 0,106 | MAP-kinase-activated kinase inhibitor. |
Prediction of Activity Spectra for Substances examination of Duartin.
Concentration-dependent cytotoxic effect of Duartin on breast cancer cells (MDA-MB-231)
The outcomes of the concentration-dependent cell toxicity assay are presented in Figure 3. This assay demonstrated a significant impact of Duartin on the viability of MDA-MB-231 cells. Additionally, the IC50 value for Duartin was determined to be 13.42 µM in MDA-MB-231 cancer cells.
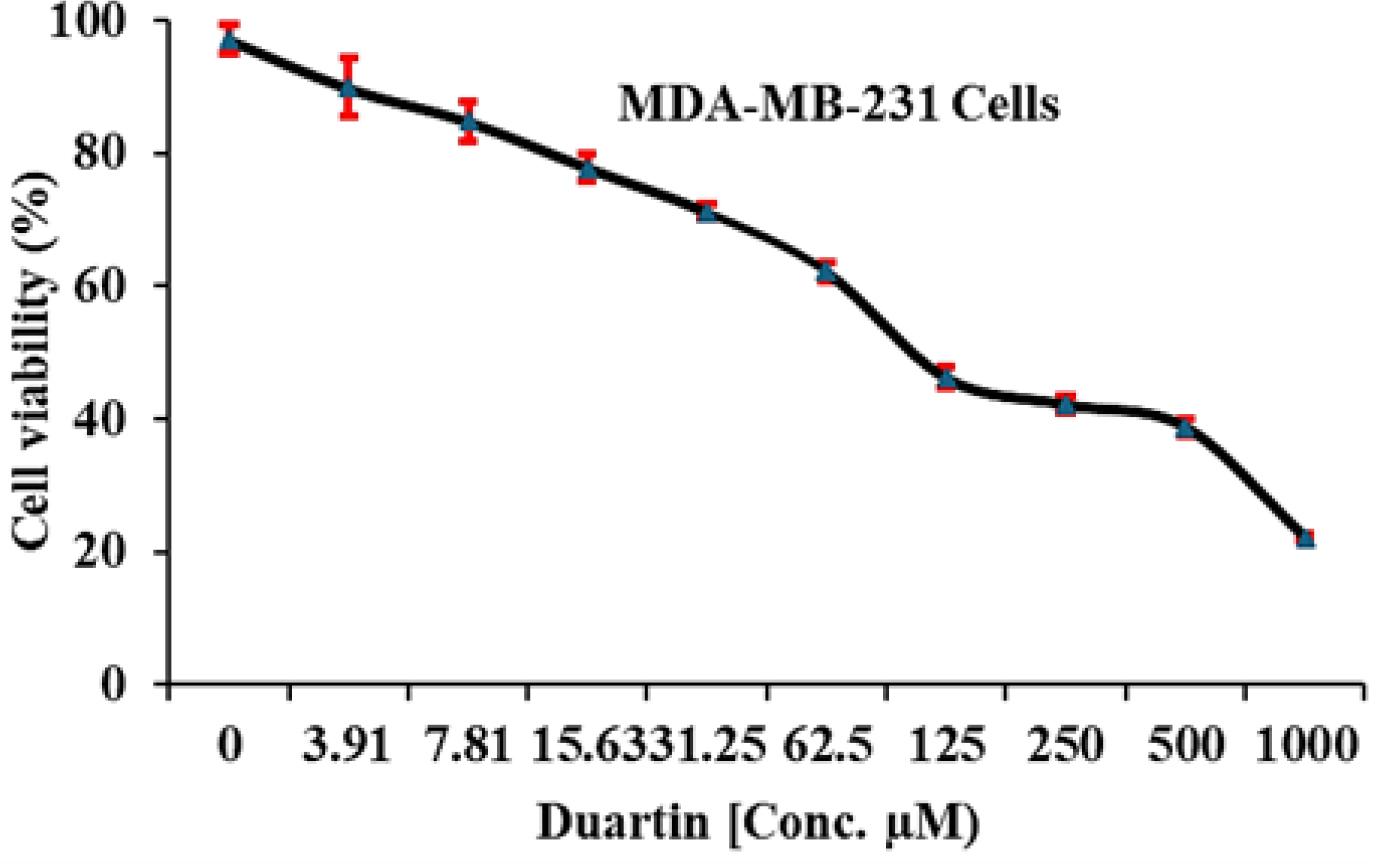
Figure 3:
Concentration dependent cell toxicity effect of Duartin on breast cancer cells (MDA-MB-231).
Time dependent cytotoxic effect of Duartin on breast cancer cells
The outcomes of the time-dependent cell toxicity assay are depicted in Figure 4. Increased exposure durations of breast cancer cells to the IC50 concentration of Duartin significantly impacted their viability for up to 48 hr.
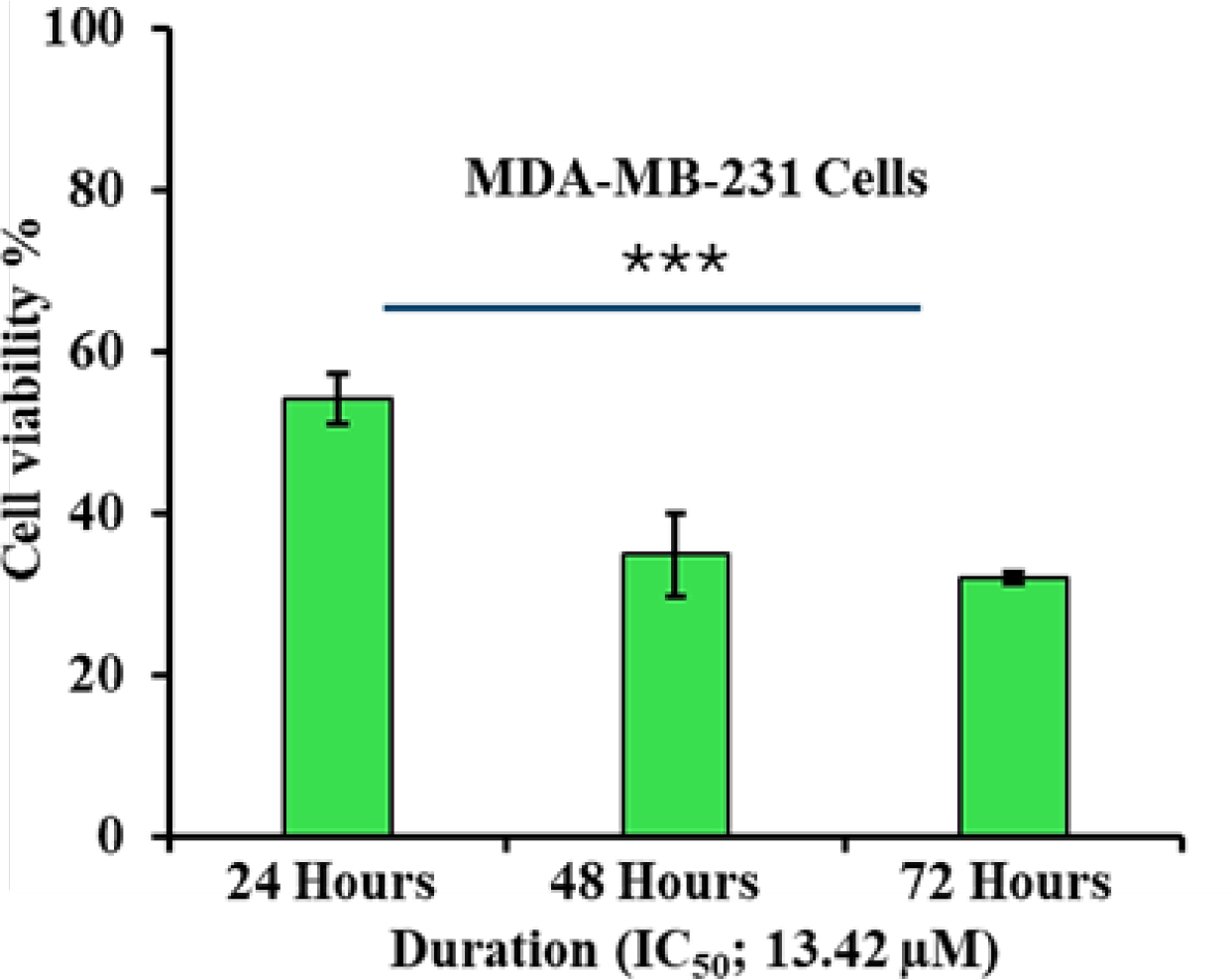
Figure 4:
Time dependent cytotoxic effect of Duartin on breast cancer cells.
Expression of Aurora A protein kinase in Duartin treated breast cancer cells
The outcomes represented in Figure 5 illustration a statistically significant effect of the IC50 concentration of Duartin on the mRNA expression of Aurora A protein kinase in breast cancer cells. Specifically, mRNA expression was significantly reduced (p<0.001) in Duartin-treated cells compared to untreated cells.
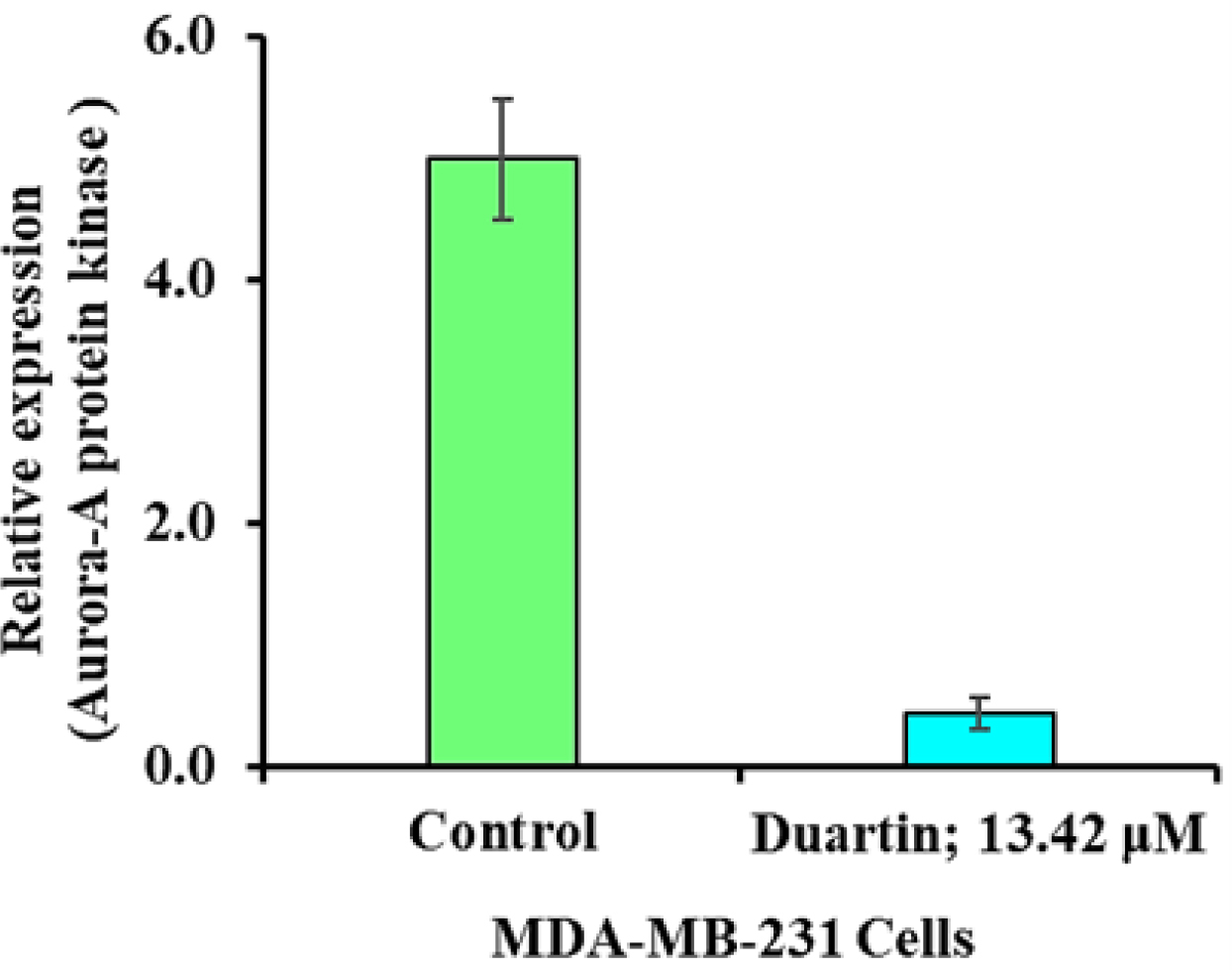
Figure 5:
Effect of IC50 dose of Duartin on the mRNA expression of Aurora A protein kinase.
DISCUSSION
Initially, the study aimed to investigate the binding affinity of various compounds with the Aurora A protein kinase, a crucial enzyme involved in cell cycle regulation and mitotic spindle assembly (Carmenaet al., 2009). The results demonstrated that Duartin exhibited the highest binding affinity with Aurora A protein kinase, suggesting its potential as a strong inhibitor of this kinase. The binding affinity indicated that Duartin forms multiple stable interactions with key residues of Aurora A protein kinase. Specifically, Duartin establishes conventional hydrogen bonds with the LYS41 and ALA213 residues, which are critical for the stabilization of the ligand-protein complex (Panigrahi and Desiraju, 2007). The presence of these hydrogen bonds likely contributes significantly to the high binding affinity observed (Chenet al., 2016). Moreover, Duartin also engaged in a diversity of other non-covalent interactions, as well as van der Waals forces, carbon hydrogen bonds, Pi-sigma interactions, alkyl bonds, and Pi-alkyl bonds. These interactions further stabilize the Duartin-Aurora A complex, enhancing the overall binding affinity. The interaction pattern of Duartin with Aurora A protein kinase, emphasize the unique and robust interaction network formed by Duartin. The diverse array of bonds and interactions not only highlights the strong binding capability of Duartin but also highlights its specificity towards Aurora A protein kinase (Pitsawonget al., 2018; Zorbaet al., 2014). This specificity is crucial for the development of targeted inhibitors that can effectively disrupt Aurora A protein kinase activity without affecting other kinases, thereby minimizing potential off-target effects (Zorbaet al., 2014).
The findings of this study provide a comprehensive evaluation of the drug-like properties of Duartin, supported by ADMET calculations, PASS examination, and Swiss Target Prediction. The ADMET properties direct that Duartin keeps promising features in terms of absorption, distribution, metabolism, and excretion, while also exhibiting a lack of toxicity. These properties are crucial for any potential therapeutic agent, as they ensure that the compound can be efficiently absorbed, distributed to the target tissues, metabolized effectively and excreted without causing adverse effects (Ononamaduet al., 2021). The PASS analysis further corroborates the potential of Duartin as a therapeutic agent by predicting a range of biological activities. PASS analysis employs a robust algorithm to envisage the activity (biological) spectrum of a compound and the results for Duartin indicate its potential efficacy in various pharmacological domains (Poroikovet al., 2000). This predictive capability is essential for early-stage drug discovery, as it helps in identifying promising candidates for further investigation. SwissTargetPrediction analysis supports the findings of the PASS analysis by providing insights into the potential molecular targets of Duartin (Dainaet al., 2019). Together; these computational predictions affirm the drug-like properties of Duartin and highlights its potential as a good therapeutic agent, targeting Aurora A protein kinase in breast cancer. The convergence of favourable ADMET characteristics, a broad spectrum of predicted biological activities and multiple potential molecular targets positions Duartin as a promising candidate for further preclinical and clinical studies.
The concentration-dependent cell toxicity assay revealed that Duartin exerts a pronounced effect on the viability of breast cancer cells. Duartin, 13.42 µM, indicates that Duartin has a potent cytotoxic effect on these cells. This finding is crucial as it proposes that Duartin could potentially be developed as a therapeutic agent for breast cancer, specifically targeting MDA-MB-231 cells, which are known for their aggressive behaviour and resistance to certain treatments. Furthermore, the time-dependent cell toxicity assay provided additional insights into the cytotoxic dynamics of Duartin. The outcomes presented that prolonged exposure of MDA-MB-231 cells to the Duartin significantly reduced cell viability over a 48 hr period. This time-dependent decrease in viability highlights the sustained action of Duartin, suggesting that continuous exposure could be necessary for achieving optimal therapeutic effects. The significant reduction in cell viability with both concentration and time indicates that Duartin acts effectively at low micromolar concentrations and continues to impact cell survival over extended periods (Hashemniaet al., 2016; Pancholiet al., 2022). These findings are promising for the possible expansion of Duartin as a therapeutic agent.
Further, the results of this study also highlight the critical influence of Duartin on the regulation of Aurora A protein kinase mRNA expression in breast cancer cells (MDA-MB-231). The marked decrease in Aurora A mRNA levels following treatment with Duartin, suggests a potent inhibitory effect of Duartin. This finding is particularly significant with the well-established role of Aurora A kinase in the regulation of mitotic entry and progression, as well as its association with poor prognosis in various cancers, including breast cancer (Duet al., 2021; Nikhil and Shah, 2024). Furthermore, the impact of Duartin on Aurora A protein kinase mRNA expression may provide insights into its broader antitumor mechanisms. Aurora A kinase is a key player in the regulation of mitosis and its overexpression has been linked to chromosomal instability and tumorigenesis (Katayama et al., 2023). By significantly reducing the expression of this kinase, Duartin may help to restore normal cell cycle control and reduce the propagation of cancer cells.
CONCLUSION
The study aimed to examine the binding affinity of various compounds with Aurora A protein kinase, a crucial enzyme in cell cycle regulation. Duartin exhibited the highest binding affinity, forming stable interactions with Aurora A protein kinase. ADMET properties, PASS and SwissTargetPrediction analyses affirm its drug-like properties. Duartin significantly reduced cell viability in breast cancer cells, portentous it’s probable as a effective therapeutic agent. Its ability to decrease Aurora A mRNA levels indicates a strong inhibitory effect, potentially restoring normal cell cycle control and reducing cancer cell proliferation. Overall, the findings from this study indicate that the Duartin may emerge as a good therapeutic agent for further preclinical and clinical studies targeting breast cancer.
Cite this article:
Aloqbi AA. Evaluating the Therapeutic Potential of Duartin against Aurora A Protein Kinase in Breast Cancer Cells. Int. J. Pharm. Investigation. 2025;15(3):313-24.
ABBREVIATIONS
PDB | Protein Data Bank |
---|---|
ADMET | Absorption, Distribution, Metabolism, Excretion and Toxicity |
DMSO | Dimethyl sulfoxide |
PASS | Prediction of Activity Spectra for Substances. |
References
- Bhullar K. S., Lagarón N. O., McGowan E. M., Parmar I., Jha A., Hubbard B. P., Rupasinghe H. P. V., et al. (2018) Kinase-targeted cancer therapies: Progress, challenges and future directions. Molecular Cancer 17: 48 https://doi.org/10.1186/s12943-018-0804-2 | Google Scholar
- Carmena M., Ruchaud S., Earnshaw W. C.. (2009) Making the Auroras glow: Regulation of Aurora A and B kinase function by interacting proteins. Current Opinion in Cell Biology 21: 796-805 https://doi.org/10.1016/j.ceb.2009.09.008 | Google Scholar
- Chen D., Oezguen N., Urvil P., Ferguson C., Dann S. M., Savidge T. C., et al. (2016) Regulation of protein-ligand binding affinity by hydrogen bond pairing. Science Advances 2: Article e1501240 https://doi.org/10.1126/sciadv.1501240 | Google Scholar
- Daina A., Michielin O., Zoete V.. (2019) SwissTargetPrediction: Updated data and new features for efficient prediction of protein targets of small molecules. Nucleic Acids Research 47: W357-W364 https://doi.org/10.1093/nar/gkz382 | Google Scholar
- Du R., Huang C., Liu K., Li X., Dong Z.. (2021) Targeting AURKA in Cancer: Molecular mechanisms and opportunities for Cancer therapy. Molecular Cancer 20: 15 https://doi.org/10.1186/s12943-020-01305-3 | Google Scholar
- Fu J., Bian M., Jiang Q., Zhang C.. (2007) Roles of Aurora kinases in mitosis and tumorigenesis. Molecular Cancer Research: MCR 5: 1-10 https://doi.org/10.1158/1541-7786.MCR-06-0208 | Google Scholar
- Goldenson B., Crispino J. D.. (2015) The aurora kinases in cell cycle and leukemia. Oncogene 34: 537-545 https://doi.org/10.1038/onc.2014.14 | Google Scholar
- Hashemnia S. M. R., Atari-Hajipirloo S., Roshan-Milani S., Valizadeh N., Mahabadi S., Kheradmand F., et al. (2016) Imatinib alters cell viability but not growth factors levels in TM4 Sertoli cells. International Journal of Reproductive Biomedicine 14: 577-582 https://doi.org/10.29252/ijrm.14.9.577 | Google Scholar
- Ingebriktsen L. M., Humlevik R. O. C., Svanøe A. A., Sæle A. K. M., Winge I., Toska K., Kalvenes M. B., Davidsen B., Heie A., Knutsvik G., Askeland C., Stefansson I. M., Hoivik E. A., Akslen L. A., Wik E., et al. (2024) Elevated expression of Aurora-A/AURKA in breast cancer associates with younger age and aggressive features. Breast Cancer Research: BCR 26: 126 https://doi.org/10.1186/s13058-024-01882-x | Google Scholar
- Jeng Y.-M., Peng S.-Y., Lin C.-Y., Hsu H.-C.. (2004) Overexpression and amplification of Aurora-A in hepatocellular carcinoma. Clinical Cancer Research: An Official Journal of the American Association for Cancer Research 10: 2065-2071 https://doi.org/10.1158/1078-0432.ccr-1057-03 | Google Scholar
- Katayama H., Brinkley W. R., Sen S.. (2003) The Aurora kinases: Role in cell transformation and tumorigenesis. Cancer Metastasis Reviews 22: 451-464 https://doi.org/10.1023/a:1023789416385 | Google Scholar
- Katayama H., Wang J., Treekitkarnmongkol W., Kawai H., Sasai K., Zhang H., Wang H., Adams H. P., Jiang S., Chakraborty S. N., Suzuki F., Arlinghaus R. B., Liu J., Mobley J. A., Grizzle W. E., Wang H., Sen S., et al. (2012) Aurora kinase-A inactivates DNA damage-induced apoptosis and spindle assembly checkpoint response functions of p73. Cancer Cell 21: 196-211 https://doi.org/10.1016/j.ccr.2011.12.025 | Google Scholar
- Keen N., Taylor S.. (2004) Aurora-kinase inhibitors as anticancer agents. Nature Reviews. Cancer 4: 927-936 https://doi.org/10.1038/nrc1502 | Google Scholar
- Kitzen J. J., de Jonge M. J., Verweij J.. (2010) Aurora kinase inhibitors. Critical Reviews in Oncology/Hematology 73: 99-110 https://doi.org/10.1016/j.critrevonc.2009.03.009 | Google Scholar
- Lin X., Xiang X., Hao L., Wang T., Lai Y., Abudoureyimu M., Zhou H., Feng B., Chu X., Wang R., et al. (2020) The role of Aurora-A in human cancers and future therapeutics. American Journal of Cancer Research 10: 2705-2729 https://doi.org/10.1016/j.critrevonc.2009.03.009 | Google Scholar
- Liu B., Zhou H., Tan L., Siu K. T. H., Guan X.-Y.. (2024) Exploring treatment options in cancer: Tumor treatment strategies. Signal Transduction and Targeted Therapy 9: 175 https://doi.org/10.1038/s41392-024-01856-7 | Google Scholar
- Magnaghi-Jaulin L., Eot-Houllier G., Gallaud E., Giet R.. (2019) Aurora A protein kinase: To the centrosome and beyond. Biomolecules 9: 28 https://doi.org/10.3390/biom9010028 | Google Scholar
- Mehra R., Serebriiskii I. G., Burtness B., Astsaturov I., Golemis E. A.. (2013) Aurora kinases in head and neck cancer. The Lancet. Oncology 14: e425-e435 https://doi.org/10.1016/S1470-2045(13)70128-1 | Google Scholar
- Nikhil K., Shah K.. (2024) The significant others of aurora kinase a in cancer: Combination is the key. Biomarker Research 12: 109 https://doi.org/10.1186/s40364-024-00651-4 | Google Scholar
- Nowakowski J., Cronin C. N., McRee D. E., Knuth M. W., Nelson C. G., Pavletich N. P., Rogers J., Sang B.-C., Scheibe D. N., Swanson R. V., Thompson D. A., et al. (2002) Structures of the cancer-related Aurora-A, FAK and EphA2 protein kinases from nanovolume crystallography. Structure 10: 1659-1667 https://doi.org/10.1016/s0969-2126(02)00907-3 | Google Scholar
- Ononamadu C. J., Ibrahim A.. (2021) Molecular docking and prediction of ADME/drug-likeness properties of potentially active antidiabetic compounds isolated from aqueous-methanol extracts of Gymnema sylvestre and Combretum micranthum. Biotechnologia 102: 85-99 https://doi.org/10.5114/bta.2021.103765 | Google Scholar
- Pancholi S., Simigdala N., Ribas R., Schuster E., Leal M. F., Nikitorowicz-Buniak J., Rega C., Bihani T., Patel H., Johnston S. R., Dowsett M., Martin L.-A., et al. (2022) Elacestrant demonstrates strong anti-estrogenic activity in PDX models of estrogen-receptor positive endocrine-resistant and fulvestrant-resistant breast cancer. npj Breast Cancer 8: 125 https://doi.org/10.1038/s41523-022-00483-1 | Google Scholar
- Panigrahi S. K., Desiraju G. R.. (2007) Strong and weak hydrogen bonds in the protein-ligand interface. Proteins 67: 128-141 https://doi.org/10.1002/prot.21253 | Google Scholar
- Pitsawong W., Buosi V., Otten R., Agafonov R. V., Zorba A., Kern N., Kutter S., Kern G., Pádua R. A., Meniche X., Kern D., et al. (2018) Dynamics of human protein kinase Aurora A linked to drug selectivity. eLife 7: Article e36656 https://doi.org/10.7554/eLife.36656 | Google Scholar
- Poroikov V. V., Filimonov D. A., Borodina Y. V., Lagunin A. A., Kos A.. (2000) Robustness of biological activity spectra predicting by computer program PASS for noncongeneric sets of chemical compounds. Journal of Chemical Information and Computer Sciences 40: 1349-1355 https://doi.org/10.1021/ci000383k | Google Scholar
- Roshan P., Kuppa S., Mattice J. R., Kaushik V., Chadda R., Pokhrel N., Tumala B. R., Biswas A., Bothner B., Antony E., Origanti S., et al. (2023) An Aurora B-RPA signaling axis secures chromosome segregation fidelity. Nature Communications 14: 3008 https://doi.org/10.1038/s41467-023-38711-2 | Google Scholar
- Sasai K., Parant J. M., Brandt M. E., Carter J., Adams H. P., Stass S. A., Killary A. M., Katayama H., Sen S., et al. (2008) Targeted disruption of Aurora A causes abnormal mitotic spindle assembly, chromosome misalignment and embryonic lethality. Oncogene 2998 27: 4122-4127 https://doi.org/10.1038/onc.2008.47 | Google Scholar
- Sen S., Zhou H., White R. A.. (1997) A putative serine/threonine kinase encoding gene BTAK on chromosome 20q13 is amplified and overexpressed in human breast cancer cell lines. Oncogene 14: 2195-2200 https://doi.org/10.1038/sj.onc.1201065 | Google Scholar
- Sung H., Ferlay J., Siegel R. L., Laversanne M., Soerjomataram I., Jemal A., Bray F., et al. (2021) Global cancer statistics 2020: GLOBOCAN estimates of incidence and mortality worldwide for 36 cancers in 185 countries. CA: A Cancer Journal for Clinicians 71: 209-249 https://doi.org/10.3322/caac.21660 | Google Scholar
- Takeshita M., Koga T., Takayama K., Ijichi K., Yano T., Maehara Y., Nakanishi Y., Sueishi K., et al. (2013) Aurora-B overexpression is correlated with aneuploidy and poor prognosis in non-small cell lung cancer. Lung Cancer 80: 85-90 https://doi.org/10.1016/j.lungcan.2012.12.018 | Google Scholar
- Torchia E. C., Chen Y., Sheng H., Katayama H., Fitzpatrick J., Brinkley W. R., Caulin C., Sen S., Roop D. R., et al. (2009) A genetic variant of Aurora kinase A promotes genomic instability leading to highly malignant skin tumors. Cancer Research 69: 7207-7215 https://doi.org/10.1158/0008-5472.CAN-09-1059 | Google Scholar
- Trott O., Olson A. J.. (2010) AutoDock Vina: Improving the speed and accuracy of docking with a new scoring function, efficient optimization and multithreading. Journal of Computational Chemistry 31: 455-461 https://doi.org/10.1002/jcc.21334 | Google Scholar
- Wang P., Gong Y., Guo T., Li M., Fang L., Yin S., Kamran M., Liu Y., Xu J., Xu L., Peng F., Xue X., Yang M., Hung M.-C., Lam E. W.-F., Gu C., Wang C., Zhan Q., Liu Q., et al. (2019) Activation of aurora A kinase increases YAP stability via blockage of autophagy. Cell Death and Disease 10: 432 https://doi.org/10.1038/s41419-019-1664-4 | Google Scholar
- Wang X. X., Liu R., Jin S. Q., Fan F. Y., Zhan Q. M.. (2006) Overexpression of Aurora-A kinase promotes tumor cell proliferation and inhibits apoptosis in esophageal squamous cell carcinoma cell line. Cell Research 16: 356-366 https://doi.org/10.1038/sj.cr.7310046 | Google Scholar
- Willems E., Dedobbeleer M., Digregorio M., Lombard A., Lumapat P. N., Rogister B., et al. (2018) The functional diversity of Aurora kinases: A comprehensive review. Cell Division 13: 7 https://doi.org/10.1186/s13008-018-0040-6 | Google Scholar
- Zhang J., Ma J., Li Y., An Y., Du W., Yang Q., Huang M., Cai X., et al. (2024) Overexpression of Aurora kinase B is correlated with diagnosis and poor prognosis in hepatocellular carcinoma. International Journal of Molecular Sciences 25: 2199 https://doi.org/10.3390/ijms25042199 | Google Scholar
- Zhang Y., Ma Y., Wang Y., Mukhopadhyay D., Bi Y., Ji B., et al. (2022) Aurora kinase a inhibitor MLN8237 suppresses pancreatic cancer growth. Pancreatology 22: 619-625 https://doi.org/10.1016/j.pan.2022.03.019 | Google Scholar
- Zheng D., Li J., Yan H., Zhang G., Li W., Chu E., Wei N., et al. (2023) Emerging roles of Aurora-A kinase in cancer therapy resistance. Acta Pharmaceutica Sinica. B 13: 2826-2843 https://doi.org/10.1016/j.apsb.2023.03.013 | Google Scholar
- Zorba A., Buosi V., Kutter S., Kern N., Pontiggia F., Cho Y.-J., Kern D., et al. (2014) Molecular mechanism of aurora A kinase autophosphorylation and its allosteric activation by TPX2. eLife 3: Article e02667 https://doi.org/10.7554/eLife.02667 | Google Scholar