ABSTRACT
Background
Rhinitis is a prevalent condition affecting individuals of all age groups, resulting from factors such as cold, nasal infections, allergies, inflammation, and sinusitis. The diverse compositions of bioactive essential oils, containing many chemical components, hold significant promise in preventing and treating numerous human diseases. Certain herbs exhibit favorable physiological and medicinal values when administered individually or in combination with others. Both eucalyptus oil and peppermint oil have been traditionally used to alleviate nasal congestion.
Aim
Considering the synergistic effects of bioactive oils, the present study aimed to develop nasal microemulsion systems using bioactive oils.
Materials and Methods
The microemulsion was prepared with eucalyptus oil and peppermint oil based on the pseudo-ternary phase diagram with different ratios of surfactant and co-surfactant. The microemulsions were evaluated for pH, electrical conductivity, viscosity, optical transparency, droplet sizes and stability studies.
Results
The microemulsion droplets were nano-sized and stable at different temperature conditions. The in vitro anti-inflammatory activity of the microemulsion was assessed by protein denaturation assay and showed a promising result compared to the standard drug.
Conclusion
Eucalyptus oil and Peppermint oil blend microemulsion formulations could be an effective platform for nasal drug delivery system for the treatment of rhinitis
INTRODUCTION
Rhinitis, also known as nasal congestion, is defined by the obstruction of nasal passages and decreased airflow through the nose, resulting in the release of mucus, sneezing, and itching. This is caused by inflammation of the inner lining of the nose. Nasal congestion occurs as a result of the dilation of blood vessels triggered by exercise, consumption of spicy food, exposure to cold air, and even tension. Nevertheless, the primary factors leading to rhinitis are cold, influenza, chronic sinusitis, upper respiratory virus infection, and hay fever.1,2 Infectious rhinitis can be viral, bacterial, or fungal. Viral rhinitis normally resolves within seven days, while bacterial and fungal infections persist for a long time. Symptoms associated with rhinitis are sinus pain, facial pain, vacuum headaches, ear-popping, poor speech perception, and pain around the nasal bridge between eyes.1 Although rhinitis is not life-threatening, it may impair natural nasal breathing, loss of productivity, daily activities, mild to severe sleep disturbances, and quality of life in adults and children. Sometimes, the situation worsens in case of pre-existing diseases like eczema, asthma, or allergies.3,4 Rhinitis is commonly treated with medications, including intranasal anticholinergics, antihistamines, and decongestants.5 Oral and topical decongestants, intranasal steroids, and vasoconstrictors are frequently used for the treatment of rhinitis.6 Despite their large use, local and systemic adverse reactions are frequent.
Essential bioactive oils are part of medicinal plants frequently employed as formulation additives in pharmaceuticals, foods, and cosmetic products.7 Natural oils are extremely complex mixtures containing various chemical compounds used as an anti-inflammatory, antioxidant, analgesic, antibacterial, antiviral, and spasmolytic.8,9 The naturally occurring bioactive oils revealed potential pharmacological activity as an alternative to synthetic drugs when used alone or in combination. Eucalyptus Oil (EO) from Eucalyptus species (Eucalyptus globulus Labill) is commercially available for the treatment of the common cold and other respiratory infections. The active constituents’ citronellal and 1,8- cineole and other bioactive like p-cymene, citronellol, limonene, α-phelladrene, β-phelladrene, α-pinene, β-pinene, trans-pinocarveol, terpineol of EO have been reported for antibacterial, antifungal, anti-inflammatory and antitumor activity.10,11 The steam vapors of EO are traditionally used to remove the stuffiness in the nose and as aromatherapy for the treatment of migraine.12 Peppermint Oil (PO), an essential bioactive oil derived from M. piperitae aetheroleum L., is used to treat different human diseases like headaches, mild bacterial and fungal infections, and herpes simplex virus infections.12,13 Menthol and carvone are the major components of PO responsible for strong antifungal and antibacterial activity.14,15 Other bioactive components present in PO are α-pinene, β-pinene, sabinene, α-terpinene, γ-terpinene, p-cymene, limonene, terpinolene, linalool, menthone, menthofurane, isomenthone, neo-isomenthol, menthol, α-terpineol, piperitone, menthyl acetate.16,17 Terpenes have a crucial role in the prevention of inflammation.17 The lipophilic and high volatility characteristics of bioactive oils result in poor stability with lower efficacy and bioavailability.18 The therapeutic effectiveness of EO and PO for the treatment of rhinitis may be improved by blending them with suitable nasal delivery systems.
Nasal administration of drugs has become increasingly prominent due to its fast absorption, ability to bypass liver metabolism, and capacity to transport drugs to the brain through the olfactory area.19 The nasal administration of active pharmaceutical ingredients is a non-invasive route for local, systemic, and CNS action. Intranasal delivery of the drug may provide a rapid onset of action and improved bioavailability compared to oral administration.20 Therefore, it is expected that intranasal delivery of EO and PO blend (1:1) may attain the rapid onset of action in the management of rhinitis and nasal decongestion. Despite the potential of nasal drug delivery, it has several limitations like mucociliary clearance, poor permeability, and enzymatic degradation.21
Microemulsion seems to be a convincing approach for the administration of bioactive components to the nasal cavity. The microemulsion is a thermodynamically stable transient dispersion of oil, surfactant, co-surfactant, and aqueous phase.22 Their stability, solubilization properties, ability to protect labile drugs, control the drug release, and improved solubility have drawn attention as a unique vehicle for drug delivery. Microemulsion can incorporate a large number of natural oils in the continuous or dispersed phase, which is otherwise difficult to formulate.23 The advantages of microemulsion are enhancement of drug solubilization and better absorption across the mucosal membrane due to smaller globule size.24 The presence of co-surfactants in the microemulsion formulations additionally reduces the surface tension, which can expand the area of existence of a microemulsion system.25 Hence, as a co-surfactant ethanol, 1-hexanol, and propylene glycol were used to prepare microemulsion in the study.
Therefore, the purpose of this work was to prepare and evaluate a new nasal microemulsion containing bioactive EO and PO blend for the treatment of rhinitis. Also, the stability of the microemulsion was evaluated.
MATERIALS AND METHODS
Materials
Eucalyptus oil and Peppermint oil were purchased from Sigma Aldrich, India. Tween 80, propylene glycol, and Glycerin were purchased from Loba Chemicals, India. 1-Hexanol and Ethanol were purchased from Prism Sales, Raipur, India. All other chemicals were used in analytical grade and procured commercially. Distilled water was used throughout the study.
Construction of pseudo-ternary phase diagram
The pseudo-ternary phase diagrams were constructed using the water titration method with a slight modification to obtain the appropriate ratio of surfactant and co-surfactant that may result in the large existence of microemulsion area.22 Briefly, the surfactant Tween 80 and different co-surfactants were mixed (Smix) in weight ratios of 1:1, 1:2, and 1:3, respectively to prepare the phase diagrams. For each diagram, aliquots of Smix were mixed individually with oil (EO and PO blend 1:1) in glass vials in the ratios of 0.5:9.5, 1:9, 1.5:8.5, 2:8, 2.5:7.5, 3:7, 3.5:6.5, 4:6, 4.5:5.5, 5:5, 5.5:4.5, 6:4, 6.5:3.5, 7:3, 7.5:2.5, 8:2, 8.5:1.5, 9:1 and 9.5:0.5. One system was prepared by using only oil and surfactant without adding co-surfactant. Then, mixtures of oil and Smix at certain weight ratios were diluted dropwise with distilled water under gentle magnetic stirring. All the processes were carried out at 25°C temperature. After being equilibrated, the systems were characterized visually and determined as being microemulsion, crude emulsion, or microemulsion gel. The isotropic nature of the formulations was confirmed after being observed under polarizing light. The transparent/translucent appearance of the systems was considered a monophasic microemulsion and the turbid appearance with phase separation was considered a crude emulsion. The viscous systems that did not exhibit any change in the meniscus after being tilted to the right angle were considered gel.26 The phase diagrams were constructed using Triplot software.
Preparation of microemulsion
Microemulsion formulations were prepared according to the composition obtained from the constructed phase diagram, presented in Table 1. The concentrations of oil and water were kept the same in all formulations. The variables were types of co-surfactants and their presence or absence in the formulations. The EO and PO blend with 1:1 ratio was mixed with a surfactant or Smix and then water was added to the mixture dropwise under magnetic stirring. Glycerin was added in the formulations as a humectant and to avoid nasal irritation caused by excipients. All the formulations were kept separately in a well-closed container for further use.
Materials | ME1 | ME2 | ME3 | ME4 |
---|---|---|---|---|
EO + PO (1:1) | 15 | 15 | 15 | 15 |
Tween 80 | 70 | 35 | 35 | 35 |
Ethanol | – | 35 | – | – |
Propylene glycol | – | – | 35 | – |
1-Hexanol | – | – | – | 35 |
Water | 15 | 15 | 15 | 15 |
Composition of microemulsion formulations with various co-surfactants.
Evaluation of microemulsion
Determination of external appearance
Microemulsions were evaluated for colour, homogeneity, and odour. The optical transparency was investigated by keeping the sample in clear containers under a good illuminator and against a black-and-white background. The uniformity among the formulations was also studied using a trinocular polarizing microscope (Metz-888A, Metzer, India). A drop of microemulsion was placed on a glass slide covered with a coverslip and then observed under a microscope.
Determination of pH
The pH of microemulsion formulations was measured using a digital pH meter (S220, pH/Ion Meter, Mettler Toledo, Switzerland). The pH meter was calibrated using pH 4 and pH 7 buffers before measurement.27 The glass electrode of pH meter was immersed directly into the microemulsion formulation at room temperature and readings were recorded. Each measurement was carried out in triplicate.
Determination of electrical conductivity
The electrical conductivity of the prepared microemulsions was measured at 25±2°C using a digital conductivity meter (G-3001, Digital Conductivity Meter, HPG Systems and India). The process was followed as per the manufacturer’s instructions. The electrode was directly dipped into the microemulsion, and the reading was recorded once equilibrium was achieved. All the samples were measured in triplicate.
Determination of viscosity
The viscosity of microemulsion formulations was determined using a Brookfield dial reading viscometer (LVT, Brookfield Engineering Laboratories, USA) at 25°C temperature. The rotating LV spindle number 61 was used for all the measurements. The speed of the spindle was adjusted to 30 rpm, and the reading was recorded using the analog display of the viscometer.27 The viscosity was calculated by multiplying the reading with the corresponding factor value. All samples were measured in triplicate.
Determination of transmittance
The transmittance of microemulsions was measured using a UV-visible spectrophotometer (UV 3000, Labindia Analytical Instruments, Mumbai, India). Briefly, 1 mL of sample was placed inside the glass cuvette and the transmittance of light through the microemulsion was measured at the wavelength of 650 nm using distilled water as blank (with 100% transmittance).28 All measurements were carried out in triplicate.
Determination of thermodynamic stability
The thermodynamic stability of microemulsion formulations was evaluated by the three-step flowing process to find the stable formulations as per reported methods with slight modification.29
Heating-cooling cycle study
To study the effect of temperature, three glass vials filled with microemulsion and hermetically closed were placed vertically in a refrigerator (4°C) and then in a hot air oven (40°C) for a period of 48 hr each. The microemulsions were examined and any changes were recorded. This cooling and heating cycle was repeated four times.
Freeze-thaw cycle study
In this study, microemulsion was kept separately in three glass vials and sealed with a rubber closure. The glass vials were then exposed between -10°C and 25°C with storage at each temperature for 48 hr. The process was repeated four times and any changes observed were recorded.
Centrifugation study
Microemulsion formulations were centrifuged (RM-12C, REMI, India) at 4000 rpm for 30 min at room temperature to observe the changes for phase separation, aggregation, and creaming. The formation of two layers or hazy appearance will be considered the separation of oil and water in the formulations.
Determination of droplet size
The microemulsion droplet size was measured as per the reported method30 using Photon Correlation Spectroscopy (PCS) (Malvern Instruments, Malvern, UK). The fixed angle of 90° was used for the measurement of size. The sample was slightly sonicated before measurement. The droplet size was estimated by the average of three determinations under identical conditions and presented as mean diameter.
Atomic Force Microscopy (AFM) study
The morphology of microemulsion droplets was examined by atomic force microscopy. The microemulsion sample was placed on a clean flat glass surface and air dried. The examination was carried out with AFM (JPK instrument, Berlin, Germany) using a silicone probe having a force constant 0.2 N/m. The image was obtained with the scan speed of 2 Hz and 312 kHz resonant frequency.31
Stability study
The stability study of microemulsion formulation was carried out as a function of storage temperature, Relative Humidity (RH), and time. The samples were packed in borosilicate glass vials and stored in the stability study chamber (KI 220, Khera Instruments, India) maintaining constant environmental climatic conditions. The storage conditions and sample testing frequency were as per the protocol of ICH (International Conference on Harmonization) guidelines. EO and PO blend microemulsions were evaluated for physical appearance, pH, conductivity, and viscosity over six months at predetermined time intervals. All studies were performed in triplicate.30
In vitro anti-inflammatory activity study
Heat- induced egg albumin denaturation assay
The anti-inflammatory activity of EO and PO blend microemulsion was carried out as per the reported method with little modification.32,33 The various reaction mixtures consisted of 2 mL microemulsion, 1% w/v egg albumin (fresh hen’s egg), and Phosphate-Buffered Saline (PBS, pH 6.8) were prepared to make final concentrations of 100, 200, 300, and 500 μg/mL. Similar mixtures were also prepared using a reference drug sample of acetylsalicylic acid (aspirin) without adding microemulsion. An equal volume of PBS was used as a control. The above reaction mixtures were incubated at 37°C for 15 min and then heated at 70°C for 5 min. After cooling, the absorbance of all mixtures was analyzed at 660 nm using a UV-visible spectrophotometer (UV 3000, Labindia Analytical Instruments, Mumbai, India). The control group is considered as 100% protein denaturation. The inhibition of albumin denaturation was determined using the following formula:
Where, A1=absorbance of the control and A2=absorbance of the test.
Statistical analysis
Data were expressed as mean value±SD (standard deviation). For statistical evaluation, experimental data was tested by One-way Analysis of Variance (ANOVA). In all cases, p<0.05 (*) was indicated as a significant difference.
RESULTS
Pseudo-ternary phase diagram study
The correlation between the phase behavior of a mixture and the compositions present inside the mixture can be captured by constructing a phase diagram. The pseudo-ternary phase diagrams of oil (EO and PO blend), Tween 80, and water were constructed separately by water titration method with or without adding co-surfactant (Figure 1). The surfactant was mixed with a co-surfactant (ethanol) (Smix) in a % weight ratio of 1:1, 1:2, and 1:3. The surfactant, Tween 80 was able to form microemulsion without co-surfactant over a range of oil-surfactant-water ratios and the microemulsion zone of about 25% was observed from the plotted pseudo-ternary phase diagram (Figure 1a). The maximum water of 50% was solubilized in an oil-surfactant blend with the least oil concentration and with increasing concentration of oil, the amount of water progressively reduced. The fluidity of the microemulsion was reduced with increasing concentration of water inside the microemulsion zone. However, with increasing concentration of water with high surfactant content the system changed into gel outside the microemulsion zone, and with further dilution, the gel structure transformed into a coarse emulsion.
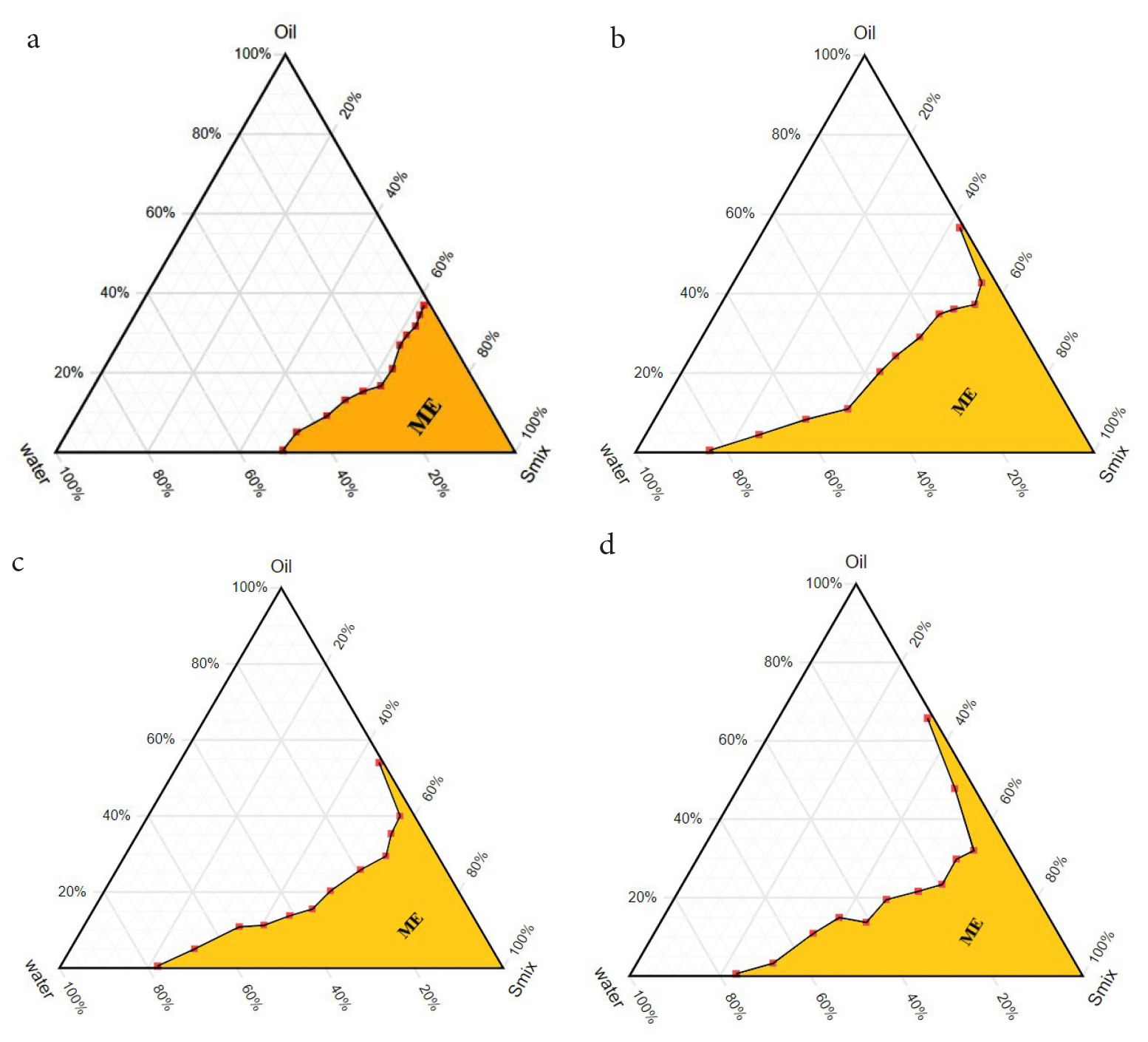
Figure 1:
Pseudo-ternary phase diagrams of oil (EO and PO blend), Tween 80 and water system with or without co-surfactant. a. Phase diagram without co-surfactant, b. Surfactant/Co-surfactant (1:1), c. Surfactant/Co-surfactant (1:2) and d. Surfactant/ Co-surfactant (1:3).
The addition of co-surfactant in the oil-surfactant system increased the amount of incorporated water in the microemulsion zone as compared to the co-surfactant-free system (Figure 1). The microemulsions prepared with Tween 80 and ethanol (Smix) in the weight ratio of 1:1, 1:2, and 1:3 showed an increased amount of water in their respective microemulsion zone. The incorporation of ethanol with the ratio of 1:1 increased the water content to a maximum of 84% occupying about 45% of the total area in the phase diagram (Figure 1b). The increasing concentration of co-surfactant further decreased the area of the microemulsion zone as compared to Smix ratio 1:1 (Figure 1c and 1d). The system containing the Smix ratio of 1:2 and 1:3 showed water content of 78% and 72% with a microemulsion zone of 34% and 40% respectively at low oil concentrations.
Preparation of microemulsion
Four microemulsion formulations were prepared based on the results obtained from the pseudo-ternary phase diagram by keeping oil and water concentrations the same (Table 1). Three different co-surfactants viz. ethanol, propylene glycol, and 1-hexanol with Smix ratio 1:1 were used to develop the Microemulsion formulations (ME2, ME3, and ME4), and one formulation was developed without adding co-surfactant (ME1). The microemulsions containing three different co-surfactants increased the microemulsion zone with the maximum amount of water in the systems. At a constant oil and water ratio, the microemulsion zone of ethanol containing system occupied almost 50% of the total area (Figure 2b) in the pseudo-ternary phase diagram, whereas propylene glycol and 1-hexanol occupied about 45% and 42% of the total area (Figure 2c and 2d) respectively. This area was only 32% in the case of co-surfactant- free microemulsion (Figure 2a). The maximum water content of 86% for ethanol and 87% for propylene glycol was observed with very little oil content. At the same time, 74% of the water content was observed in the case of 1-hexanol. The water content was reduced sharply in the phase diagram with increasing oil concentration and the microemulsion zone was also reduced in all cases. The presence of co-surfactant abolished the region of gel from the oil-water system in the phase diagram compared to co-surfactant-free systems.26,34
Microemulsion Formulations | pH | Electrical conductivity (mS/cm) | Viscosity (cp) | Transmittance (%) |
---|---|---|---|---|
ME1 | 5.08±0.14 | 0.18±0.06 | 64.26±3.43 | 83.77±0.55 |
ME2 | 5.71±0.09 | 0.27±0.05 | 12.58±0.52 | 96.85±0.21 |
ME3 | 5.43±0.17 | 0.21±0.02 | 31.79±1.43 | 90.92±0.99 |
ME4 | 6.54±0.08 | 0.23±0.02 | 16.83±0.76 | 94.22±0.58 |
Results of pH, electrical conductivity viscosity and transmittance of EO and PO blend microemulsion formulations (data represents mean value±SD, n=3).
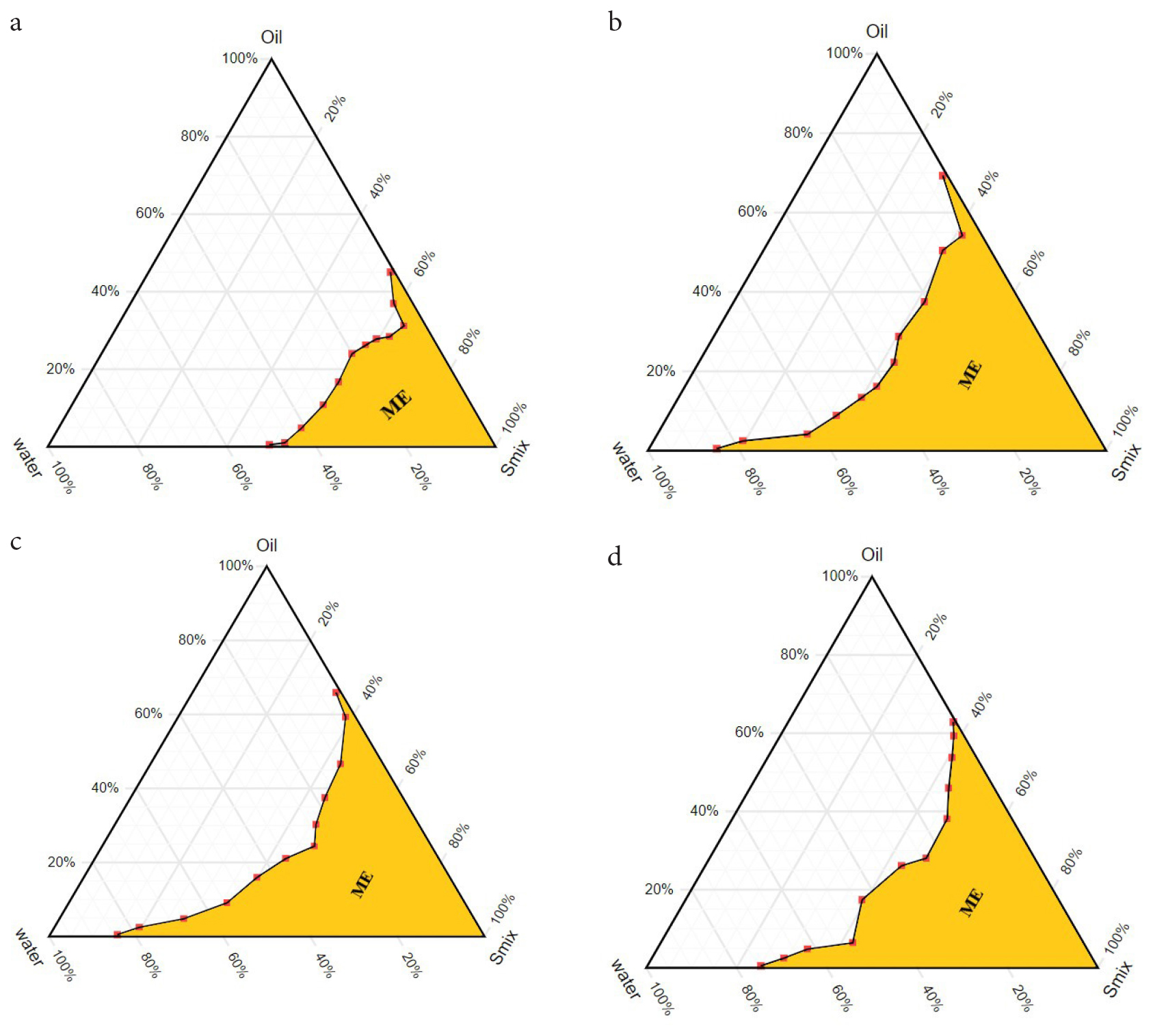
Figure 2:
Pseudo-ternary phase diagrams of Microemulsion formulations (ME1, ME2, ME3 and ME4). a. ME1 without co-surfactant, b. ME2 with co-surfactant ethanol, c. ME3 with co-surfactant propylene glycol and d. ME4 with co-surfactant 1-hexanol.
Evaluation of microemulsion
All the microemulsion formulations were transparent, optically homogenous, and isotropic liquid. The odour of the formulations was similar to the odour of the physical mixture of EO and PO. No birefringent texture was noted after the light microscopy study.
Determination of pH
The pH of all microemulsion formulations was measured in the range of 5.08 to 6.54 (Table 2), which is almost the usual pH range of nasal fluids. It is very important for a nasal preparation that could minimize the irritation inside the nasal cavity upon installation.22
Group | Storage conditions | Sample testing frequencies |
---|---|---|
Time 0 | – | 0 |
Long term | 25±2°C/60±5% RH | 1, 3, 6 and 9 months |
Accelerated | 40±2°C/75±5% RH | 1, 3 and 6 months |
Long term (Refrigerated) | 5±3°C | 1, 3, 6 and 9 months |
Different storage conditions included in the experiments as per ICH guidelines.
Determination of electrical conductivity
The structural characteristics of the microemulsion can be studied by measuring the changes in conductivity at an increasing weight fraction of water in the system. Electrical conductivity might be used to determine the type of microemulsion formed, as o/w type microemulsions show better electrical conductivity than w/o type. The higher conductivity is due to the water as a continuous external phase in the emulsion. The lower water content in microemulsion formulation could disperse in the oil phase with small droplets, where they separated each other by minimum interaction, developed w/o type microemulsion with low electrical conductivity.27,35 The dilution of w/o microemulsion with water increased the water fraction inside the system and showed increased electrical conductivity due to the external pseudo-aqueous phase.36 The electrical conductivity of four microemulsion formulations is given in Table 2. All the formulations showed low conductivity. However, slightly increased conductivity (0.27±0.05 mS/cm and 0.23±0.02 mS/cm) was observed with the microemulsion formulations containing an alcohol group. The microemulsion ME1 showed low conductivity due to less water content inside it.
Determination of viscosity
The viscosity of developed microemulsions was determined. The results are shown in Table 2. It was observed that the viscosity of co-surfactant free Microemulsion (ME1) was high (64.26±3.43 cp) and showed pseudo-plastic flow behavior. The addition of co-surfactant into the formulations reduced the viscosity remarkably. The formulation containing propylene glycol (ME3) produced the highest viscosity of 31.79±1.43 cp comparing other co-surfactant-containing formulations. No noticeable difference in the viscosity was observed between ethanol and 1-hexanol- containing microemulsions. The presence of short-chain alcohol as a co-surfactant highly reduced the viscosity of the formulation (ME2). The result was consistent with the previous findings.34
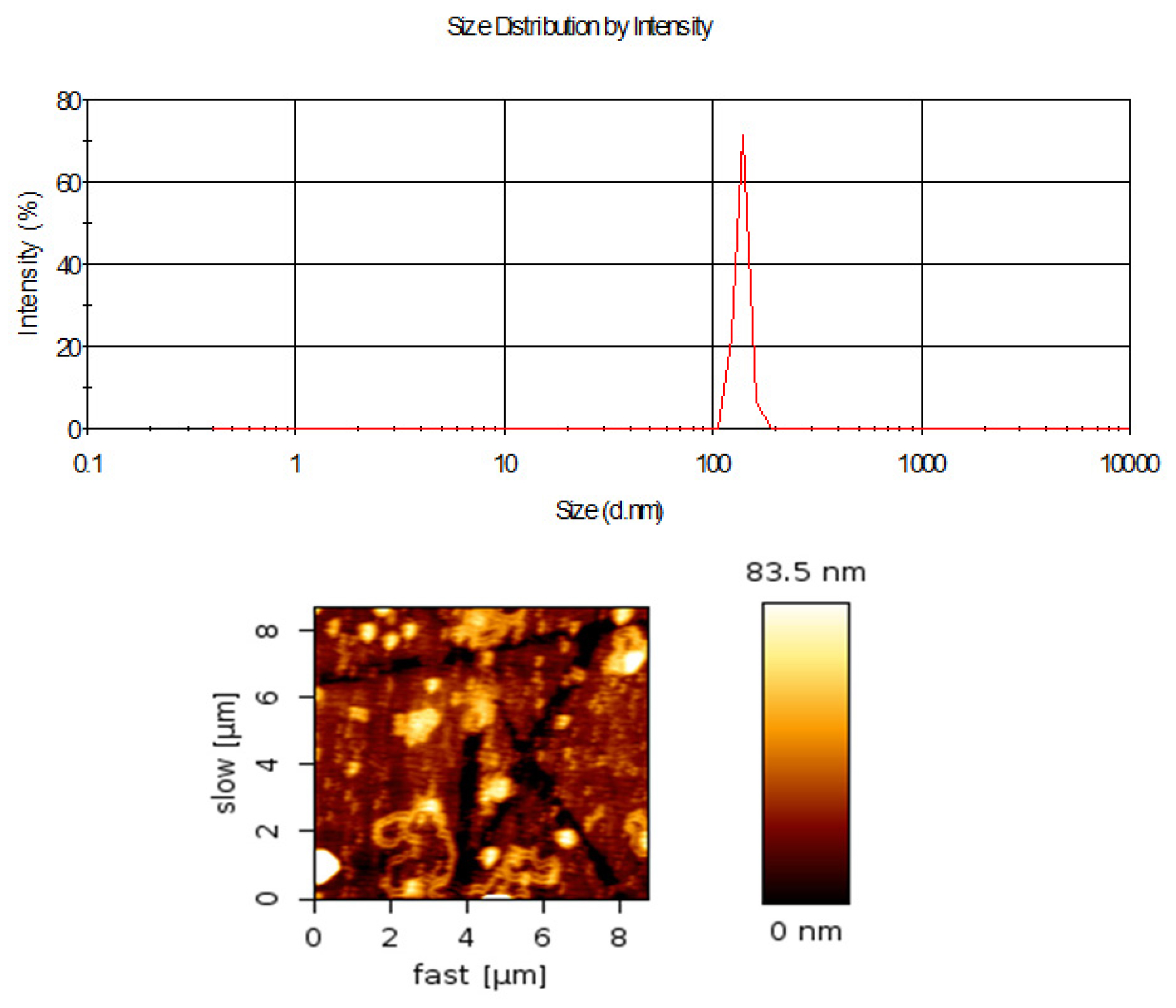
Figure 3:
Size distribution and morphological analysis (a) Mean droplet size distribution of microemulsion formulation ME2 (data as mean±SD; n=5), (b) Atomic force Microscopy image of microemulsion.
Determination of transmittance
All microemulsion formulations showed a high % transmittance after spectroscopic determination, which was the indication of good emulsification of oil by Smix. The higher transmittance value suggested the homogeneity, optical clarity and transparency of the formulations. However, microemulsion formulation ME1 showed less % transmittance as compared to other formulations (Table 2). The absence of a co-surfactant may be the reason for decreased transparency.
Thermodynamic stability study
Microemulsion formulations were exposed to different thermodynamic stress conditions using a heating-cooling cycle, freeze-thaw cycle, and centrifugation tests. It was observed that no formulation showed turbidity or phase separation after thermodynamic stress tests, indicating the physical stability of microemulsions.
Droplet size and surface morphology analysis
The mean droplet sizes of the microemulsion formulation were measured by Photon Correlation Spectroscopy (PCS). The sizes of droplets were in the nano range (Figure 3a) and size distribution was mono-dispersed. The AFM experimentation showed the droplet sizes were spherical (Figure 3b). The droplet sizes obtained by AFM were relatively smaller than the droplet sizes measured by the particle size analyzer.
Stability study
The accelerated stability study was carried out to establish the stability of EO and PO blend microemulsion for six months of storage in different temperature conditions (Table 3). The microemulsion ME2 was selected for the stability study based on its larger microemulsion zone in the pseudo-ternary phase diagram. The physical appearance of ME2 microemulsion formulation stored at 40°C/75% RH for 6 months was slightly hazy as compared to the microemulsion stored at 25°C/60% RH. The hazy appearance might be the reason for the removal of volatile content from oil globules into the water phase. The physical appearance was consistent when stored at 5°C temperature. After six months, the pH and electrical conductivity of the sample were not varied at the storage of 25°C/60% RH and 5°C respectively (Figure 4a and 4b). However, a slight increase in pH and electrical conductivity was observed for the formulation stored at 40°C/75% RH. There were no noticeable changes observed in the viscosity of the formulation stored at 25°C/60% RH and 5°C, but a slight increase and then decrease in viscosity were noticed at the storage of 40°C/75% RH (Figure 4c).
In vitro anti-inflammatory activity study
The anti-inflammatory activity of EO and PO blend microemulsion was evaluated by performing an egg albumin denaturation assay. Considering the higher microemulsion zone and better stability, the formulation ME2 was selected for the study. The anti-inflammatory effect of microemulsion was compared with the standard drug aspirin. From the result, it was observed that microemulsion could show anti-inflammatory activity (Figure 5).
DISCUSSION
The formation of the microemulsion zone depends on the physicochemical properties of a surfactant, an oil phase, and an aqueous phase. Besides this, the low interfacial tension between oil-water interfaces, surfactant film on the oil globules, and surfactant-oil miscibility also play an important role in microemulsion formation.26 The possibility of microemulsion formation will be greater when the surfactant contains a short lipophilic chain and a double bond-containing group.25 Hence, Tween 80 could form microemulsions with EO and PO blend. The addition of co-surfactant in the system not only reduces the surface tension but also fluidizes the interfacial film between oil-water interfaces which can help the expansion of the area of existence of microemulsion. This can be attributed to the greater penetration of the oil phase towards the hydrophobic region, which leads to enhanced fluidity of the interface by decreasing interfacial tension. Co-surfactants containing medium and short-chain alcohols were successfully examined for developing microemulsion.25,35 The increasing area of the microemulsion zone from the phase diagram study with the Smix ratio of 1:1 was selected for further formulation development and analysis.
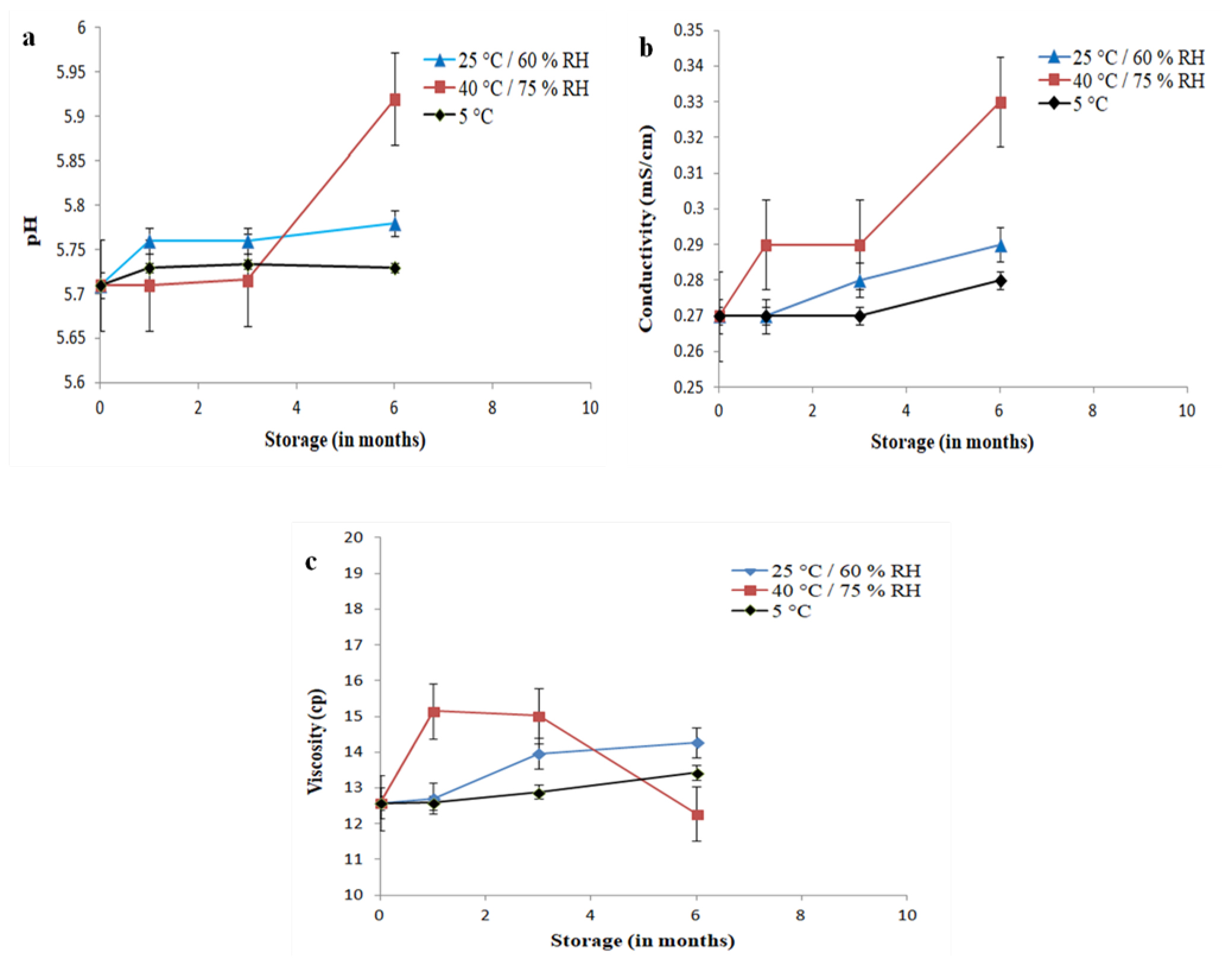
Figure 4:
pH, electrical conductivity and viscosity of EO and PO blend Microemulsion (ME2) stored at different conditions and during different times from 0 to 6 months. Error bars represent SD (n=3).
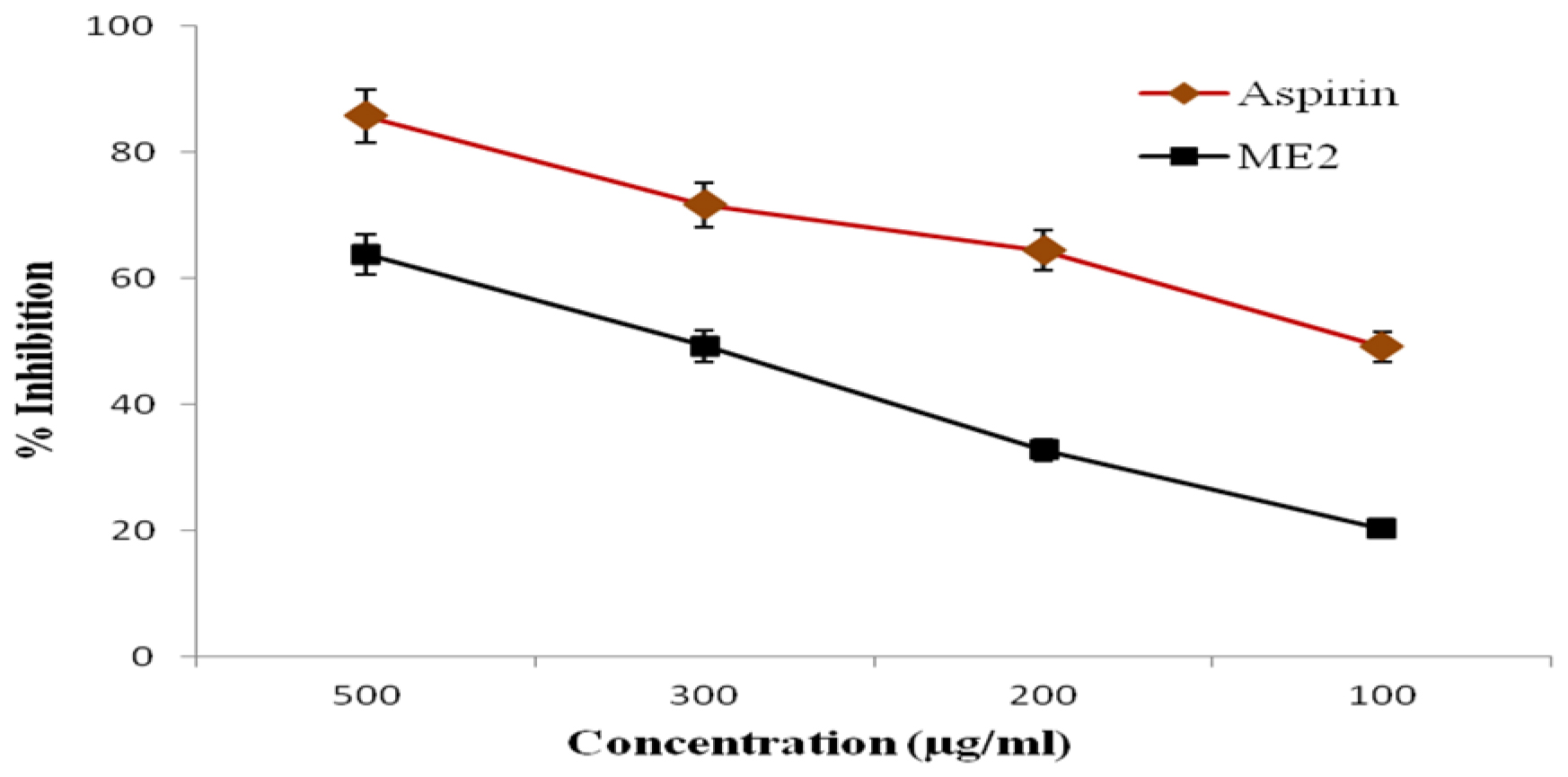
Figure 5:
Protein denaturation activity of microemulsion formulation and standard drug aspirin. The error bars represent SD (n=3). The value of p<0.05 was considered significant.
The microemulsion formation depends on the lowering of surface tension of the oil-water interface by surfactants and changes in dispersion entropy. In the event of a large surface tension drop, a favorable entropic change may occur due to the negative free energy of formation, which makes the microemulsion thermodynamically stable.25,29,37 Therefore, instead of using a surfactant, the Smix with minimum concentration was able to reduce surface tension effectively by lowering the energy of the systems, resulting in a stable microemulsion. Thermodynamically stable microemulsions are developed at a certain concentration of oil, water, and surfactant without creaming, cracking, or phase separation. Thermal stability can distinguish microemulsion from kinetically stable emulsion. The normal emulsion separates into two phases under stress, whereas microemulsions remain in a single phase.38
Allergic rhinitis is a type I hypersensitivity reaction that occurs by exposure to a specific type of allergen. Like native proteins, heat-denatured proteins provoke the delaying of a hypersensitivity reaction.39 It was also reported that conventional NSAID’s not only acted by reducing prostaglandin synthesis but also acted by preventing protein denaturation.33 Anti-denaturation activity is a convenient way for the preliminary investigation of anti-inflammatory activity. It is suggested that bioactive EO and PO blend microemulsion will be able to control the production of antigens by inhibiting protein denaturation, which may reduce nasal congestion during allergic rhinitis.40,41 However, further in vivo experimentation is necessary to explore the microemulsion formulation for nasal delivery.
CONCLUSION
Microemulsion is a promising nasal drug delivery carrier. Eucalyptus oil and Peppermint oil blend microemulsion formulations were successfully prepared. The incorporation of co-surfactant into microemulsion formulation will not only affect the physical characteristics but will also influence the nasal drug delivery efficacy which will be beneficial for the management of rhinitis. The presence of different co-surfactants showed the variation of microemulsion zone with varying viscosity and pH. The overall physicochemical effects depended on the nature of the co-surfactant used and ethanol was the efficient one, among other co-surfactants. The stability study suggested that microemulsion was stable in different temperature conditions. The result of in vitro anti-inflammatory activity suggested further in vivo experimentation for nasal inflammation associated with rhinitis. Thus, EO and PO blend microemulsions may provide an effective platform for nasal drug delivery systems for the treatment of rhinitis.
Cite this article:
Pendharkar T, Soni A, Dua JS, Jana U. Bioactive Eucalyptus and Peppermint Oil Blend Microemulsion Preparation, Characterization and Evaluation. Int. J. Pharm. Investigation. 2024;14(4):1181-91.
ACKNOWLEDGEMENT
The authors express deep gratitude to the Department of Pharmaceutics, School of Pharmacy, Chouksey Engineering College, for providing support and facilities for the completion of the study.
ABBREVIATIONS
ANOVA | Analysis of Variance |
---|---|
AFM | Atomic Force Microscopy |
CNS | Central Nervous System |
EO | Eucalyptus Oil |
ICH | International Conference on Harmonization |
ME | Microemulsion |
NSAID | Nonsteroidal Anti-Inflammatory Drug |
PBS | Phosphate-Buffered Saline |
PCS | Photon Correlation Spectroscopy |
PO | Peppermint Oil |
RH | Relative Humidity |
SD | Standard Deviation |
Smix | Surfactant/Co-surfactant |
UV | Ultraviolet |
References
- Klimek L. Potential of levocetirizine in the relief of nasal congestion. Int J Clin Pract. 2005;59(6):721-9. [PubMed] | [CrossRef] | [Google Scholar]
- Corey JP, Houser SM, Ng BA. Nasal congestion: a review of its etiology, evaluation and treatment. Ear Nose Throat J. 2000;79(9):690-704. [PubMed] | [CrossRef] | [Google Scholar]
- Mansfield LE, Diaz G, Posey CR, Flores-Neder J. Sleep disordered breathing and daytime quality of life in children with allergic rhinitis during treatment with intranasal budesonide. Ann Allergy Asthma Immunol. 2004;92(2):240-4. [PubMed] | [CrossRef] | [Google Scholar]
- Kakumanu S, Glass C, Craig T. Poor sleep and daytime somnolence in allergic rhinitis: significance of nasal congestion. Am J Respir Med. 2002;1(3):195-200. [PubMed] | [CrossRef] | [Google Scholar]
- Chang MT, Song S, Hwang PH. Cryosurgical ablation for treatment of rhinitis: A prospective multicenter study. Laryngoscope. 2020;130(8):1877-84. [PubMed] | [CrossRef] | [Google Scholar]
- Jaume F, Valls-Mateus M, Mullol J. Common cold and acute rhinosinusitis: up-to-date management in 2020. Curr Allergy Asthma Rep. 2020;20(7):28 [PubMed] | [CrossRef] | [Google Scholar]
- Dhakad AK, Pandey VV, Beg S, Rawat JM, Singh A. Biological, medicinal and toxicological significance of Eucalyptus leaf essential oil: a review. J Sci Food Agric. 2018;98(3):833-48. [PubMed] | [CrossRef] | [Google Scholar]
- Bassolé IH, Juliani HR. Essential oils in combination and their antimicrobial properties. Molecules. 2012;17(4):3989-4006. [PubMed] | [CrossRef] | [Google Scholar]
- Adorjan B, Buchbauer G. Biological properties of essential oils: an updated review. Flavour Fragr J. 2010;25(6):407-26. [CrossRef] | [Google Scholar]
- Aziz ZA, Nasir HM, Ahmad A, Setapar SH, Ahmad H, Noor MH, et al. Enrichment of Eucalyptus oil nanoemulsion by micellar nanotechnology: transdermal analgesic activity using hot plate test in rats’ assay. Sci Rep. 2019;9(1):13678 [PubMed] | [CrossRef] | [Google Scholar]
- Silva J, Abebe W, Sousa SM, Duarte VG, Machado MI, Matos FJ, et al. Analgesic and anti-inflammatory effects of essential oils of Eucalyptus. J Ethnopharmacol. 2003;89(2-3):277-83. [PubMed] | [CrossRef] | [Google Scholar]
- Göbel H, Schmidt G, Dworschak M, Stolze H, Heuss D. Essential plant oils and headache mechanisms. Phytomedicine. 1995;2(2):93-102. [PubMed] | [CrossRef] | [Google Scholar]
- Davies SJ, Harding LM, Baranowski AP. A novel treatment of postherpetic neuralgia using peppermint oil. Clin J Pain. 2002;18(3):200-2. [PubMed] | [CrossRef] | [Google Scholar]
- Botschuijver S, Welting O, Levin E, Maria-Ferreira D, Koch E, Montijn RC, et al. Reversal of visceral hypersensitivity in rat by Menthacarin®, a proprietary combination of essential oils from peppermint and caraway, coincides with mycobiome modulation. Neurogastroenterol Motil. 2018;30(6) [PubMed] | [CrossRef] | [Google Scholar]
- Spisni E, Petrocelli G, Imbesi V, Spigarelli R, Azzinnari D, Donati Sarti MD, et al. Antioxidant, anti-inflammatory and microbial-modulating activities of essential oils: implications in colonic pathophysiology. Int J Mol Sci. 2020;21(11):1-27. [PubMed] | [CrossRef] | [Google Scholar]
- Kehili S, Boukhatem MN, Belkadi A, Ferhat MA. Peppermint ( L.) essential oil as a potent anti-inflammatory, wound healing and anti-nociceptive drug. Eur J Biol Res. 2020;10(2):132-49. [PubMed] | [CrossRef] | [Google Scholar]
- Yadav VR, Prasad S, Sung B, Kannappan R, Aggarwal BB. Targeting inflammatory pathways by triterpenoids for prevention and treatment of cancer. Toxins (Basel). 2010;2(10):2428-66. [PubMed] | [CrossRef] | [Google Scholar]
- El Asbahani A, Miladi K, Badri W, Sala M, Aït Addi EH, Casabianca H, et al. Essential oils: from extraction to encapsulation. Int J Pharm. 2015;483(1-2):220-43. [PubMed] | [CrossRef] | [Google Scholar]
- Illum L. Transport of drugs from the nasal cavity to the central nervous system. Eur J Pharm Sci. 2000;11(1):1-18. [PubMed] | [CrossRef] | [Google Scholar]
- Costantino HR, Illum L, Brandt G, Johnson PH, Quay SC. Intranasal delivery: physicochemical and therapeutic aspects. Int J Pharm. 2007;337(1-2):1-24. [PubMed] | [CrossRef] | [Google Scholar]
- Erdő F, Bors LA, Farkas D, Bajza Á, Gizurarson S. Evaluation of intranasal delivery route of drug administration for brain targeting. Brain Res Bull. 2018;143:155-70. [PubMed] | [CrossRef] | [Google Scholar]
- Acharya SP, Pundarikakshudu K, Panchal A, Lalwani A. Preparation and evaluation of transnasal microemulsion of carbamazepine. Asian J Pharm Sci. 2013;8(1):64-70. [CrossRef] | [Google Scholar]
- Xavier-Junior FH, Vauthier C, Morais AR, Alencar EN, Egito ES. Microemulsion systems containing bioactive natural oils: an overview on the state of the art. Drug Dev Ind Pharm. 2017;43(5):700-14. [PubMed] | [CrossRef] | [Google Scholar]
- Monton C, Settharaksa S, Suksaeree J, Chusut T. The preparation, characterization and stability evaluation of a microemulsion-based oral spray containing clove oil for the treatment of oral candidiasis. J Drug Deliv Sci Technol. 2020;57:101735 [CrossRef] | [Google Scholar]
- Lawrence MJ. Surfactant systems: microemulsions and vesicles as vehicles for drug delivery. Eur J Drug Metab Pharmacokinet. 1994;19(3):257-69. [PubMed] | [CrossRef] | [Google Scholar]
- El Maghraby GM. Transdermal delivery of hydrocortisone from eucalyptus oil microemulsion: effects of cosurfactants. Int J Pharm. 2008;355(1-2):285-92. [PubMed] | [CrossRef] | [Google Scholar]
- Ayoub AM, Ibrahim MM, Abdallah MH, Mahdy MA. Sulpiride microemulsions as antipsychotic nasal drug delivery systems: in vitro and pharmacodynamic study. J Drug Deliv Sci Technol. 2016;36:10-22. [CrossRef] | [Google Scholar]
- Ferreira MR, Santiago RR, De Souza TP, Egito ES, Oliveira EE, Soares LA, et al. Development and evaluation of emulsions from (Andiroba) oil. AAPS PharmSciTech. 2010;11(3):1383-90. [PubMed] | [CrossRef] | [Google Scholar]
- Shafiq S, Shakeel F, Talegaonkar S, Ahmad FJ, Khar RK, Ali M, et al. Development and bioavailability assessment of ramipril nanoemulsion formulation. Eur J Pharm Biopharm. 2007;66(2):227-43. [PubMed] | [CrossRef] | [Google Scholar]
- Jana U, Mohanty AK, Manna PK, Mohanta GP. Preparation and characterization of nebivolol nanoparticles using Eudragit® RS100. Colloids Surf B Biointerfaces. 2014;113:269-75. [PubMed] | [CrossRef] | [Google Scholar]
- Jana U, Mohanty AK, Pal SL, Manna PK, Mohanta GP. Felodipine loaded PLGA nanoparticles: preparation, physicochemical characterization and toxicity study. Nano Converg. 2014;1(1):1 [CrossRef] | [Google Scholar]
- Anyasor GN, Okanlawon AA, Ogunbiyi B. Evaluation of anti-inflammatory activity of Vahl leaf extract using and inflammation models. Clin Phytosci. 2019;5(1) [CrossRef] | [Google Scholar]
- Arif Ullah HM, Zaman S, Juhara F, Akter L, Tareq SM. Emranul Haque Masum RB. Anti-inflammatory activity of ethanolic extract of rhizome. BMC Complement Altern Med. 2014:1-12. [CrossRef] | [Google Scholar]
- Alany RG, Rades T, Agatonovic-Kustrin S, Davies NM, Tucker IG. Effects of alcohols and diols on the phase behaviour of quaternary systems. Int J Pharm. 2000;196(2):141-5. [PubMed] | [CrossRef] | [Google Scholar]
- Zhang H, Feng F, Li J, Zhan X, Wei H, Li H, et al. Formulation of food-grade microemulsions with glycerol monolaurate: effects of short-chain alcohols, polyols, salts and nonionic surfactants. Eur Food Res Technol. 2008;226(3):613-9. [CrossRef] | [Google Scholar]
- Roohinejad S, Oey I, Wen J, Lee SJ, Everett DW, Burritt DJ, et al. Formulation of oil-in-water β-carotene microemulsions: effect of oil type and fatty acid chain length. Food Chem. 2015;174:270-8. [PubMed] | [CrossRef] | [Google Scholar]
- Yuan Y, Li SM, Mo FK, Zhong DF. Investigation of microemulsion system for transdermal delivery of meloxicam. Int J Pharm. 2006;321(1-2):117-23. [PubMed] | [CrossRef] | [Google Scholar]
- Lawrence MJ, Rees GD. Microemulsion-based media as novel drug delivery systems. Adv Drug Deliv Rev. 2012;64:175-93. [CrossRef] | [Google Scholar]
- Gell PG, Benacerraf B. Studies on hypersensitivity. II. Delayed hypersensitivity to denatured proteins in guinea pigs. Immunology. 1959;2(1):64-70. [PubMed] | [Google Scholar]
- Mirke NB, Shelke PS, Malavdkar PR, Jagtap PN. Array. Innov Pharm Pharmacother. 2020;8(2):28-31. [CrossRef] | [Google Scholar]
- Raphael GD, Igarashi Y, White MV, Kaliner MA. The pathophysiology of rhinitis. V. Sources of protein in allergen-induced nasal secretions. J Allergy Clin Immunol. 1991;88(1):33-42. [PubMed] | [CrossRef] | [Google Scholar]