ABSTRACT
Background
Antibiotics play a crucial role in the treatment of infectious diseases in humans and animals. However, their incomplete metabolism in the body often results in their release as active compounds into aquatic systems via domestic wastewater treatment plants. This release poses potential risks of chronic toxicity and significant health and environmental hazards. This study examines the efficacy of Polypyrrole/Titanium Dioxide Composite (PTDC) as an innovative adsorbent for removing Cephalexin (CFX) from aqueous solutions.
Materials and Methods
In the batch adsorption experiments, the researchers inspected the influence of solution pH, contact time, adsorbent dosage, and initial CFX concentration. Thermodynamic parameters were calculated, which include standard Gibbs free energy (ΔG°), standard enthalpy (ΔH°), standard entropy (ΔS°), and activation energy (A).
Results
The biosorption processes are considered spontaneous when the values of ΔG° are negative. The endothermic character of the biosorption processes is suggested by ΔH°>O for the biosorption of CFX. The biosorption capacity of the PTDC increases as the temperature rises, which also supports the endothermic nature of the biosorption processes. The activation energy for the biosorption of every CFX ion was less than 42 kJ/mol at all temperatures, suggesting that each process was diffusion-controlled.
Conclusion
The outcomes indicated that PTDC exhibits capability as an adsorbent for removing CFX from water samples to safeguard the environment.
INTRODUCTION
Antibiotics have a broad range of uses in both human and veterinary medicine as they are effective against various bacterial infections.1,2 They are also widely used in animal farming and aquaculture to prevent diseases and promote growth.3 When administered, a significant portion of the antibiotics (30-90%) is excreted without being metabolized, resulting in active antibiotic residues in the environment, such as soil, surface water, and groundwater.4,5 This occurs due to runoff from domestic, agricultural, and industrial effluents. Some commonly used antibiotics have long half-lives and are persistent, posing potential unfavorable outcomes for water quality and aquatic life.6 The wide-ranging implementation of antibiotics can lead to the alteration of microbial ecosystems and the exertion of selective pressure on susceptible bacteria, fostering the endurance of resistant strains and the emergence of antimicrobial resistance.7,8 This can lead to the ineffectiveness of existing antibiotics in treating newly emerging infectious diseases.9 Antibiotics can also elicit allergic responses in particular individuals and disrupt the native microbial system when they enter the human body through the food chain and drinking water.10,11
Antibiotics present in aquatic systems have spurred the development of antibiotic-resistant strains and genes.12 CFX, found at concentrations ranging from 10 to 100 μg/L, is a prime example of an emerging antibiotic-based contaminant causing depletion of algae and nematodes in inland aquatic systems.13 Various methods have been proposed to address the challenges posed by antibiotics in aqueous systems, including electrochemical, biological, adsorption, membrane processes, and chemical oxidation methods.14,15 However, the high cost associated with most of these methods remains a notable issue.
Adsorption methods stand out for their advantages, including low costs and high efficiencies.16,17 There has been a significant increase in publications addressing this problem from 2010 to 2021, indicating a growing interest in research on the removal of antibiotics and emerging contaminants.18
Nanotechnology is currently a prominent trend in the field of materials science.19 It pertains to materials with at least one attribute (length, width, or thickness) within the nanometer range (1-100 nm).19 Nanomaterials possess unique characteristics, such as significant specific surface area, high reactivity, and remarkable adsorption and desorption capacities.20 As a result, nanomaterials are increasingly being employed in environmental applications.21 This study explored the efficacy of a polypyrrole/titanium dioxide nanocomposite as a promising nano-adsorbent for eliminating CFX from aqueous solutions. The investigation examined the influence of four parameters-temperatures, initial concentration, and time-on the efficiency of CFX adsorption. Furthermore, thermodynamic studies were conducted to analyze the adsorption of CFX on the polypyrrole/titanium dioxide nanocomposite.
MATERIALS AND METHODS
The research utilized chemicals of analytical reagent grade, and no extra purification was required. The adsorbate, Cephalexin, [Molecular Weight=347.39 g/mol; Chemical Formula=C16H17N3O4S, and λmax=280 nm] was supplied by Sigma Aldrich Co without any purification. To prepare a stock solution of CFX, the appropriate amount of CFX was dissolved in double distilled water, resulting in a 1000 mg/L solution. The desired concentration levels of the solutions required for experiments were achieved through the dilution of the stock solution with double distilled water.
In this procedure, 50 mL of distilled water was mixed with titanium dioxide nanoparticles (0.1 g). Then, polyvinyl alcohol (0.3 g) and pyrrole monomer (1 mL), serving as a stabilizer, were incorporated into the solution. The above solution was subjected to an oxidizing process by adding ferric chloride drop by drop after a period of 30 min. The addition of oxidant caused the solution to turn from green to black, indicating the conversion of pyrrole monomer to polypyrrole. The polymerization process was allowed to proceed for a duration of 5 hr under ambient conditions. The resultant polymer was subjected to filtration and multiple washings with distilled water to eliminate oligomers and impurities. Finally, the product was subjected to drying in an oven at 45°C for 48 hr. Finally, the resulting polymer was milled to obtain a powder. Additionally, pure polypyrrole and polypyrrole/ titanium dioxide composite (without stabilizer) were synthesized for comparison.
Adsorption Studies
The PTDC composite containing polypyrrole and titanium dioxide was shaken at 180 rpm with 100 mL of an aqueous solution of CFX in a 200 mL flask at various temperatures ranging from 0-40 C. After a predetermined time interval, the reaction mixtures were filtered and analyzed for CFX concentration using a UV-vis spectrophotometer. To estimate the maximum adsorption, adsorption experiments were conducted by varying the solution pH of CFX to 7, adsorbent dosage from 0.1-1 g, time from 10-120 min, and initial concentrations from 10-100 mg/L. To ensure consistency in experimental results, all investigations were carried out in triplicate. The percentage of CFX adsorbed onto PTDC was calculated using equation 1:22
The initial and equilibrium concentrations of CFX (mg/L) in the solution are represented by C0 and Ce, respectively. To calculate the adsorption capacity, the mass balance equation 2 was utilized:23
The adsorption capacity (measured in mg/g) is denoted as “qe”. The volume of the CFX solution (measured in L) is represented by “V”. Finally, the weight of the adsorbent (measured in g) is denoted as “M”.
The adsorption of CFX onto PTDC solid surfaces is affected by changes in temperature. An increase in temperature favors the endothermic reaction while the opposite is true for the exothermic reaction. Equation 3 was utilized to gauge the corresponding change in free energy.24
The absolute temperature is denoted by T (K). The calculation of the equilibrium constant (K) is based on the relationship outlined in equation 4.25
Ce and Ca stand for the equilibrium concentrations of CFX (mg/L) in solution and on the biosorbent, respectively.
The study also analyzed the thermodynamic properties of biosorption of CFX onto PTDC by measuring the change in free energy (ΔG°), enthalpy (ΔH°), and entropy (ΔS°). ΔH° and ΔS° were determined using the van’t Hoff equation. Equations 5 and 6 demonstrate the relationship between the ΔG° and other thermodynamic properties.26
T represents the absolute temperature measured in Kelvin. R denotes the gas constant, which has a value of 8.314 joules per mole per Kelvin.
The calculation of A was carried out using the following equation.27
RESULTS
The removal efficiency of CFX was investigated by analyzing the influence of contact time with varying durations ranging from 10 to 120 min. The experiment was conducted under specific conditions (CFX concentration of 100 mg/L, pH of 3, and 0.6 g/L PTDC). The obtained results are depicted in Figure 1, which demonstrates an increase in removal efficiency as the contact time increases.
Figure 2 demonstrates the impact of pH, an important experimental variable, on the adsorption process within the pH range of 3 to 11 on the adsorption of CFX by PTDC. It is evident from Figure 2 that the removal efficiency decreased as the pH increased.
Figure 1 exhibits the outcomes of the impact of initial CFX concentrations of 10, 25, 50, and 100 mg/L, at a pH of 3, 75 min contact time, and PTDC dosage of 0.6 g/L. As shown in Figure 1, as the initial quantities of CFX are heightened, the removal efficiency lessened.
The influence of adsorbent dosages on the adsorption isotherms was also determined. Figure 3 displays the results of the adsorbent dosage effect, which includes 0.1, 0.2, 0.4, 0.6, 0.8, and 1 g/L at pH of 3, 75 min contact time, and CFX concentration of 100 mg/L. As illustrated in Figure 3, the removal efficiency increased as the adsorbent dosage increased.
Figure 4 illustrates the impact of temperature, an important experimental variable, on the adsorption process within the temperature range of 20 to 50, on the adsorption of CFX by PTDC. It is evident from Figure 4 that the removal efficiency amplified as the temperature increased.
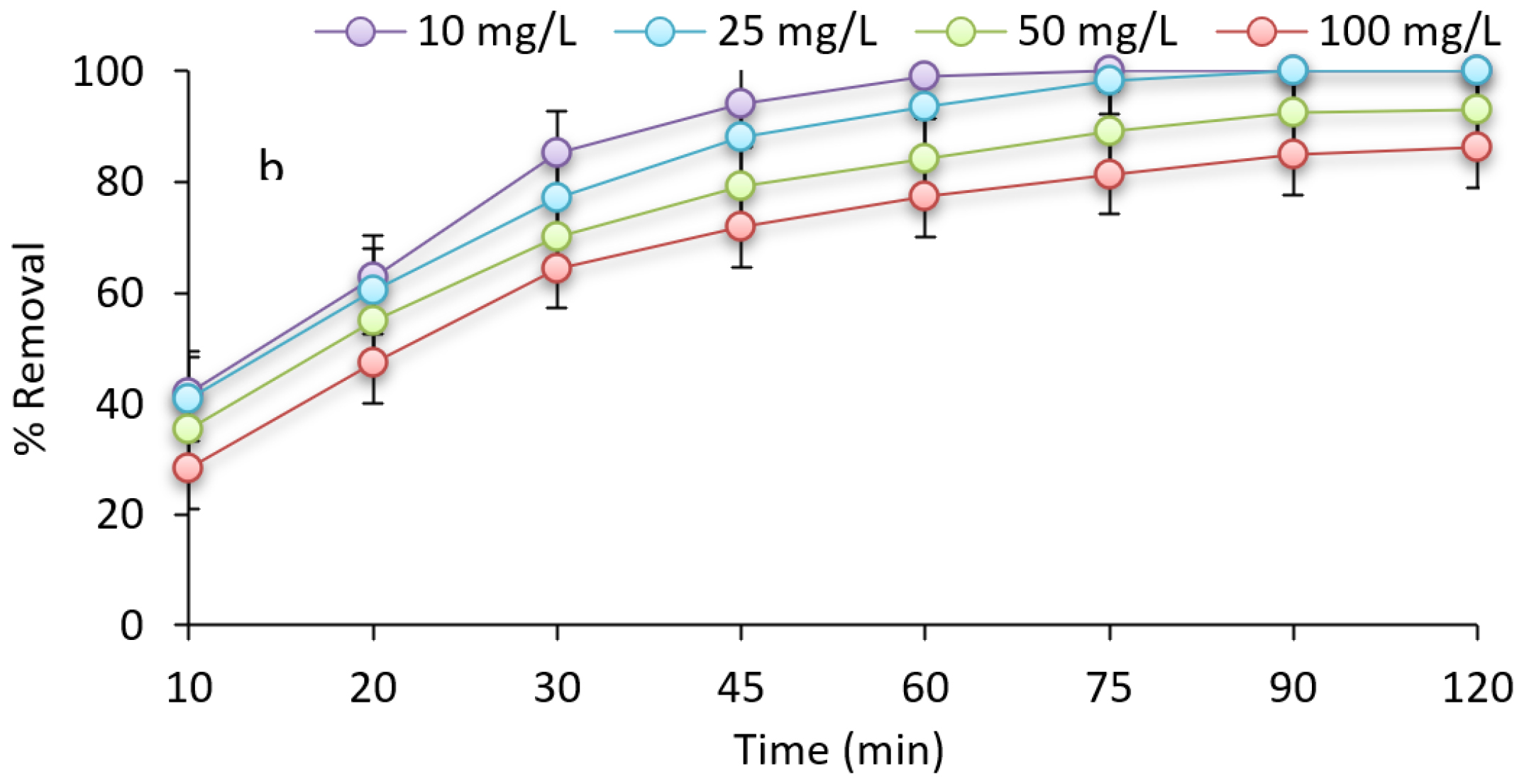
Figure 1:
The relationship between contact time and concentration for CFX adsorption (Con=100 mg/L, pH=3, dose: 0.6 g/L and temperature 30°C).
The ΔH° and ΔS° were derived via the intercept and slope of the plot of T versus ΔG° (Table 1). Typically, the ΔG° for physisorption is in the range of -20 to 0 kJ/mol and for chemisorption varies between -80 and -400 kJ/mol. The study conducted revealed that the range of the overall ΔG° values was between -2.25 and -0.84 kJ/mol. These findings indicated that the physical adsorption of the CFX was spontaneous, meaning that the system did not require any external energy resources. The enhanced biosorption efficacy at elevated temperatures is evidenced by the decrease in ΔG°. Additionally, the upsurge in temperature correlated with an increase in the biosorption capacity.
Tem (K) | ΔG° (kJ/mol) | A (kJ/mol) | ΔH° (kJ/mol) | ΔS° (kJ/mol K) |
---|---|---|---|---|
293 | -2.55 | 4.65 | 45.65 | 0.431 |
303 | -1.84 | 6.72 | ||
313 | -1.39 | 8.94 | ||
323 | 0.84- | 10.28 |
Thermodynamic parameters of adsorption of CFX onto PTDC.
Moreover, the A magnitude provides insight into the nature of the adsorption process, which can be either diffusion-controlled or chemically-based. Adsorption processes A<42 kJ/mol are diffusion-controlled, while those with higher energies are chemically-based. Table 1 presents the A values at four different temperatures. In this study, the A values were <42 KJ/mol, demonstrating diffusion-controlled adsorption processes.
DISCUSSION
In the beginning, CFX was quickly adsorbed. The first 30 min saw a removal of 89% of the CFX, after which the PTDC’s removal was gradual until it became constant at 30 min. The rapid adsorption during this contact time is due to the highly active sites present on the surface of the PTDC, which strongly attract the active sites of the adsorbent and adsorbate.28,29 It is probable that the sluggish adsorption rate is a result of the electrostatic hindrance or repulsion that occurs between the adsorbed adsorbate species, which are positively charged, on the PTDC surface and the available adsorbate species in the solution.30 Additionally, the slow diffusion of solute ions into the bulk of the adsorbent may also contribute to the delay in pore diffusion. At 60 min, the maximum CFX adsorption onto PTDC was achieved, and equilibrium was reached.
This study investigated the efficiency of PTDC in removing the CFX from aquatic environments. As the results indicate, a rise in pH led to a decline in CFX removal efficiency. Increasing the contact time and the amount of adsorbent enhances the adsorption efficiency, influenced by the ionization degree of substances in the solution, the separation of functional groups on the active adsorption sites, and the chemistry of the solution.31 These factors contribute to the electrostatic attraction between the adsorbate and adsorbent.
The study also sought to understand how pH influences within the range of 3 to 11, revealing that the highest percentage of CFX removal occurred at lower pH values. With a rise in pH from 3 to 11, the percentage of CFX removal decreased from 98.8% to 47.4%. The reason for the higher removal percentage at acidic pH levels may be enlightened by the following explanation: in acidic pH environments, active sites on the adsorbent surface become protonated, leading to an increase in the density of charge on the adsorbent surface.32 Considering the molecular structure of CFX and its hydroxide and amine bonds, CFX tends to adsorb positively charged species. Therefore, when the pH decreases, the percentage removal increases due to the electrostatic attraction between the adsorbent and the adsorbate.33
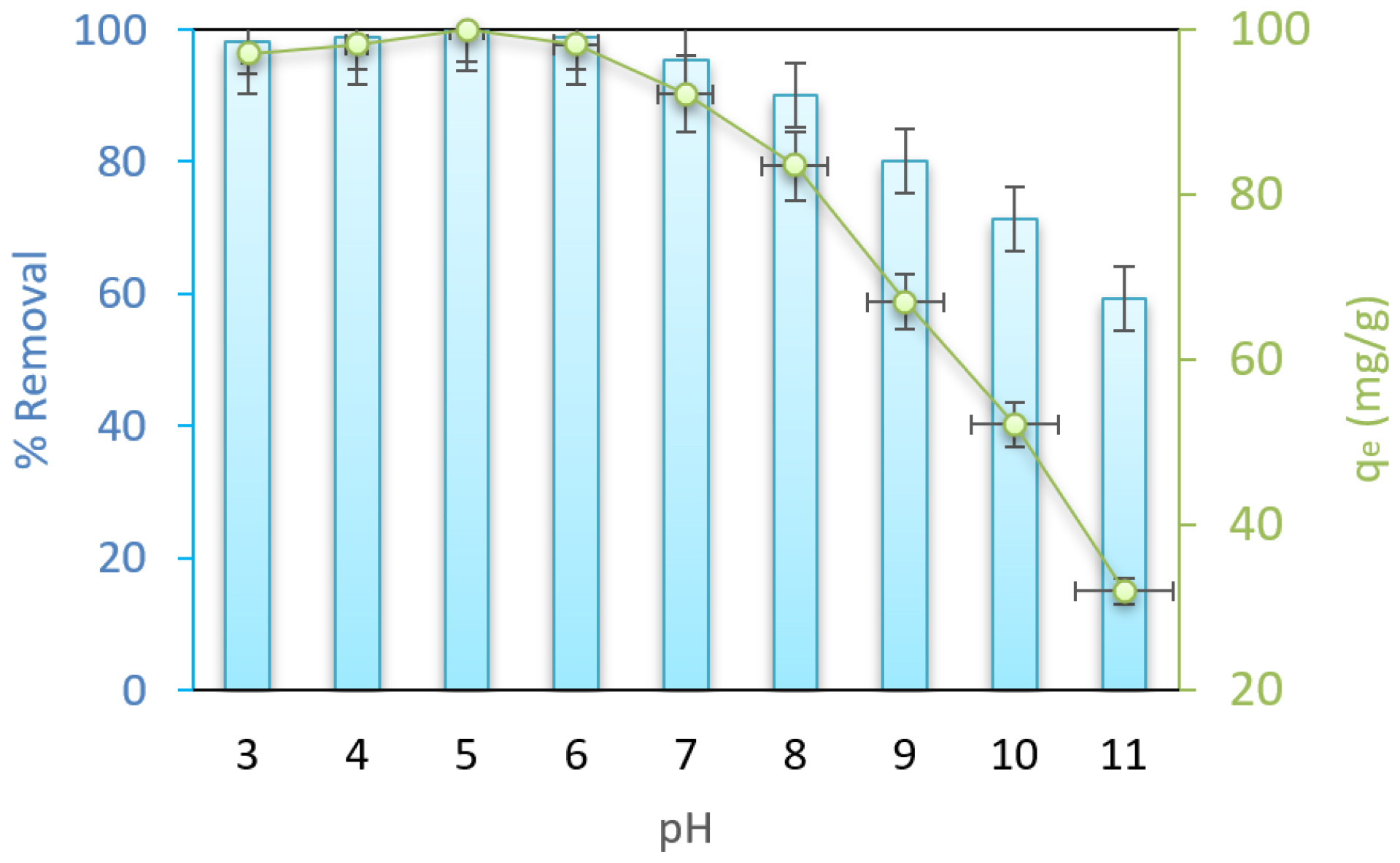
Figure 2:
Effect of pH for CFX adsorption (Con=100 mg/L, dose: 0.6 g/L and temperature 30°C).
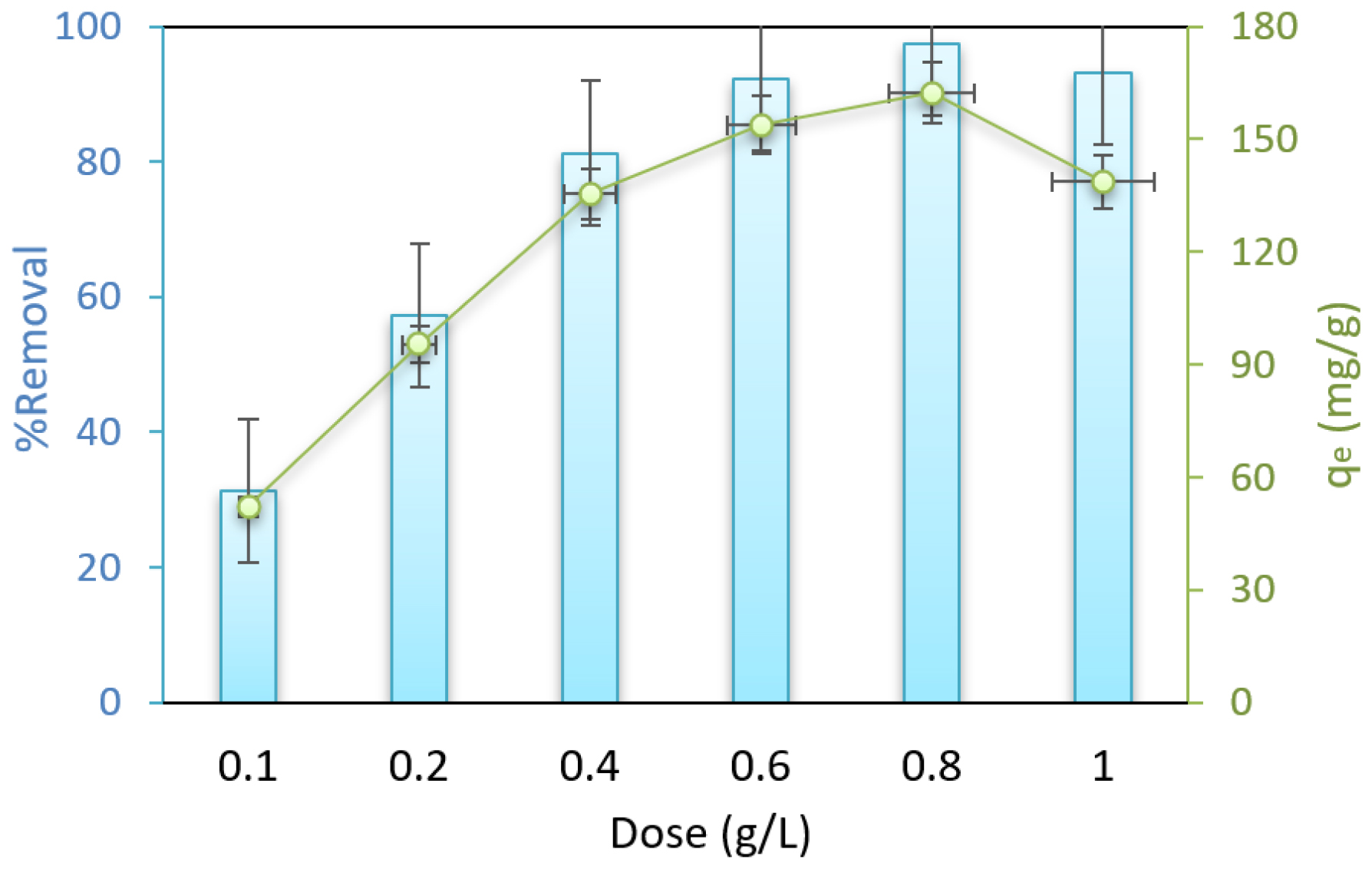
Figure 3:
Effect of adsorbent dose for CFX adsorption (Con=100 mg/L, pH=3 and temperature 30°C).
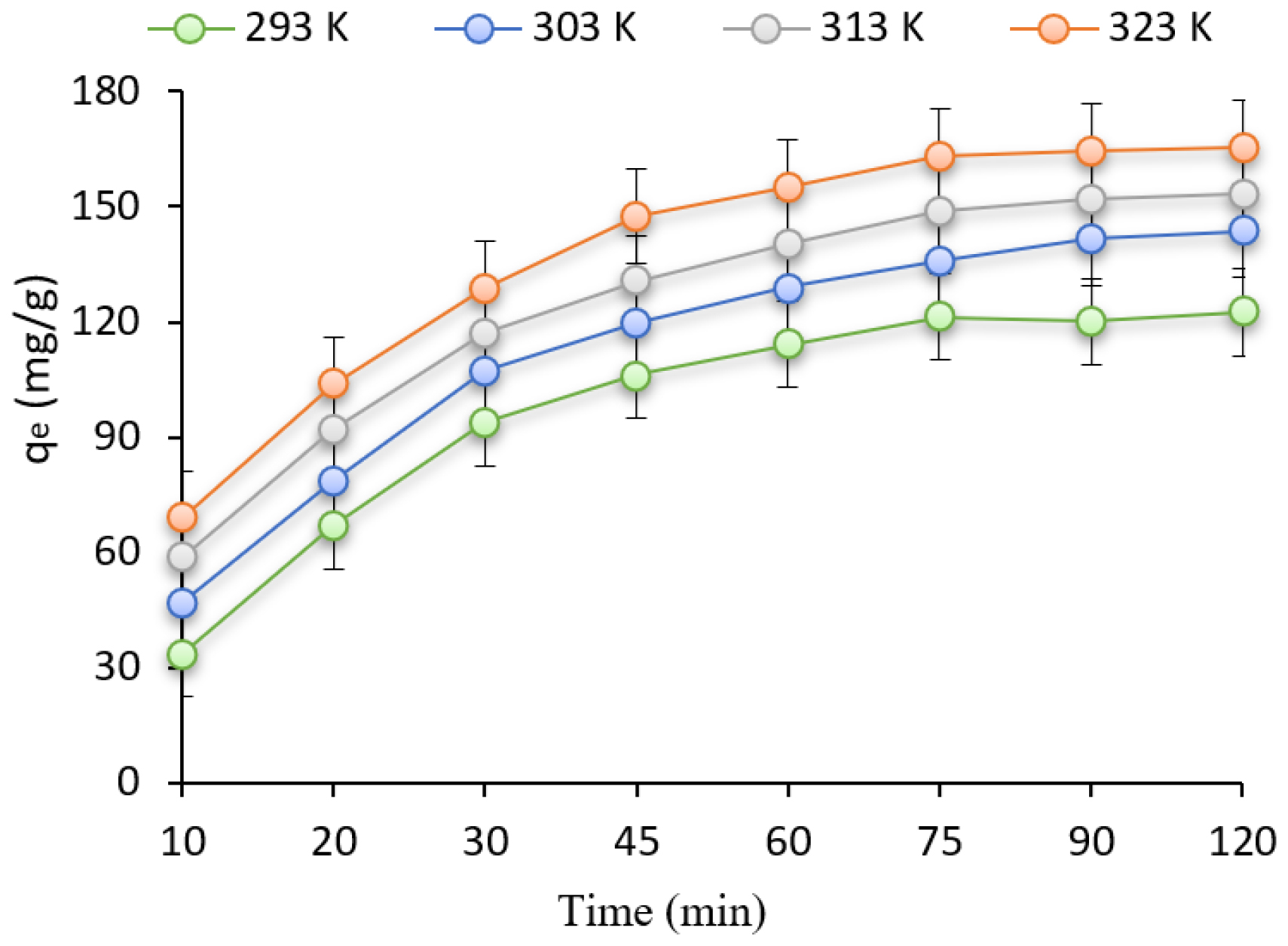
Figure 4:
Effect of temperature for CFX adsorption (Con=100 mg/L, pH=3, dose: 0.8 g/L).
Time plays a fundamental character in modeling and designing adsorption processes. In this study, the correlation between contact time and adsorption was analyzed over periods ranging from 10 to 120 min, with adsorbent amounts ranging from 0.1 to 0.4 g. According to the results, as the contact time and adsorbent amount increased, the percentage of CFX removal also increased. With 0.2 g of adsorbent, the removal efficiency of CFX increased from 81.82% in one hour to 83.90% in 2 hr. Additionally, with increasing time, the qe increased until reaching equilibrium after occupying all active sites on the adsorbent, at which point the adsorption rate decreased.34
The biosorption processes are spontaneous when the ΔG° values are negative. Biosorption of CFX is an endothermic process as ΔH° has a positive value. The biosorption capacity increases with temperature, which supports the endothermic nature of the process.35,36 The increase in ΔS° values during the biosorption of CFX implies a heightened disorder at the solid/solution interface.37 PTDC adsorbent is effective in low dosages due to its binding sites and surface area. Increasing the dosage decreases the ratio of CFX to adsorbent.38
The rapid adsorption of CFX in the initial stages may stem from the abundance of available adsorption sites at the outset of the process, facilitating the easy uptake of contaminants.39 However, as time progresses, the changes in CFX removal percentages show little variation. This could be the outcome of the saturation of external active sites on the adsorbent or the challenging access of CFX molecules to active sites on the adsorbent’s surface, necessitating additional time for adsorption to occur via internal active sites.40 Investigating the impact of CFX concentration under optimal conditions, solutions ranging from 10 to 100 mg/L of CFX were examined. It was observed that the CFX elimination percentage decreased with rising levels of pollutants. This phenomenon arises because, at higher concentrations, the accessibility of the adsorbent material to adsorption sites diminishes.37
CONCLUSION
Within this study, the elimination of CFX from an aqueous environment was accomplished through the use of PTDC. The research delved into the impact of diverse factors such as contact time, temperature, and CFX concentration on the adsorbent’s efficiency. The most effective adsorption occurred within a 60 min period, yielding a high monolayer adsorption capacity of 198.4 mg/g. The consistently negative ΔG° values (>0 KJmol-1) indicated the spontaneity of the process. Thermodynamic analysis conclusively demonstrated that the adsorption process of CFX onto magnetic PTDC nanoparticles was spontaneous and endothermic.
Cite this article:
Balarak D, Moein H, Chandrika K. Adsorption of Cephalexin Antibiotics from Aqueous Solution Using a Polypyrrole/Titanium Dioxide Nanocomposite. Int. J. Pharm. Investigation. 2024;14(4):1253-9.
ACKNOWLEDGEMENT
The authors are grateful to Zahedan University of Medical Sciences, because of supporting of this research (IR.ZAUMS. REC.1400.379).
ABBREVIATIONS
PTDC | Polypyrrole/titanium dioxide composite |
---|---|
CFX | Cephalexin |
References
- Acosta R, Fierro V, Martinez de Yuso AM, Nabarlatz D, Celzard A. Tetracycline adsorption onto activated carbons produced by KOH activation of tyre pyrolysis char. Chemosphere. 2016;149:168-76. [PubMed] | [CrossRef] | [Google Scholar]
- Ahmadi S, Banach A, Mostafapour FK, Balarak D. Study survey of cupric oxide nanoparticles in removal efficiency of ciprofloxacin antibiotic from aqueous solution: adsorption isotherm study. Desalin Water Treat. 2017;89:297-303. [CrossRef] | [Google Scholar]
- Ahmed MB, Zhou JL, Ngo HH, Guo W. Adsorptive removal of antibiotics from water and wastewater: progress and challenges. Sci Total Environ. 2015;532:112-26. [PubMed] | [CrossRef] | [Google Scholar]
- Asif Z, Chen Z. Removal of arsenic from drinking water using rice husk. Appl Water Sci. 2017;7(3):1449-58. [CrossRef] | [Google Scholar]
- Azarpira H, Mahdavi Y, Khaleghi O. Thermodynamic studies on the removal of metronidazole antibiotic by multi-walled carbon nanotubes. Pharm Lett. 2016;8(11):107-13. [CrossRef] | [Google Scholar]
- Balarak D, Baniasadi M, Bazzi M. Adsorption equilibrium and thermodynamic studies of ciprofloxacin from aqueous solutions by magnetic bentonite nanocomposites. Int J Pharm Investig. 2020;10(3):339-43. [CrossRef] | [Google Scholar]
- Balarak D, Chandrika K. Batch Studies on biosorption of ciprofloxacin on Freshwater Lemna minor. Int J Pharm Investig. 2019;9(3):117-21. [CrossRef] | [Google Scholar]
- Balarak D, Ganji F, Chandrika K, Haseeb S. Montmorillonite nanoparticles effectiveness in removal of amoxicillin from water solutions. Int J Pharm Investig. 2020;10(2):122-6. [CrossRef] | [Google Scholar]
- Balarak D, Khatibi AD, Chandrika K. Antibiotics removal from aqueous solution and pharmaceutical wastewater by adsorption process: a review. Int J Pharm Investig. 2020;10(2):106-11. [CrossRef] | [Google Scholar]
- Bazi M, Balarak D, Khatibi AD, Siddiqui SH, Mostafapour FK. Investigation of isotherm, kinetics and thermodynamics of ciprofloxacin adsorption by molecularly imprinted polymer from aqueous solutions. Int J Pharm Investig. 2021;11(3):269-73. [CrossRef] | [Google Scholar]
- Cao J, Xiong Z, Lai B. Effect of initial pH on the tetracycline (TC) removal by zero-valent iron: adsorption, oxidation and reduction. Chem Eng J. 2018;343:492-9. [CrossRef] | [Google Scholar]
- Chen H, Gao B, Li H. Removal of sulfamethoxazole and ciprofloxacin from aqueous solutions by graphene oxide. J Hazard Mater. 2015;282:201-7. [PubMed] | [CrossRef] | [Google Scholar]
- Hazard Mater. 2015;282:201-7. [PubMed] | [CrossRef] | [Google Scholar]
- Darweesh TM, Ahmed MJ. Adsorption of ciprofloxacin and norfloxacin from aqueous solution onto granular activated carbon in fixed bed column. Ecotoxicol Environ Saf. 2017;138:139-45. [PubMed] | [CrossRef] | [Google Scholar]
- Filipinas JQ, Rivera KK, Ong DC, Pingul-Ong SM, Abarca RR, de Luna MD, et al. Removal of sodium diclofenac from aqueous solutions by rice hull biochar. Biochar. 2021;3(2):189-200. [CrossRef] | [Google Scholar]
- Guler UA, Sarioglu M. Removal of tetracycline from wastewater using pumice stone: equilibrium, kinetic and thermodynamic studies. J Environ Health Sci Eng. 2014;12:79 [PubMed] | [CrossRef] | [Google Scholar]
- Han H, Cui Y, Gao R, Huang Y, Luo Y, Shen S, et al. Study on nitrogen removal from rice paddy field drainage by interaction of plant species and hydraulic conditions in eco-ditches. Environ Sci Pollut Res Int. 2019;26(7):6492-502. [PubMed] | [CrossRef] | [Google Scholar]
- Hu D, Wang L. Adsorption of amoxicillin onto quaternized cellulose from flax noil: kinetic, equilibrium and thermodynamic study. J Taiwan Inst Chem Eng. 2016;64:227-34. [CrossRef] | [Google Scholar]
- Ji L, Chen W, Duan L, Zhu D. Mechanisms for strong adsorption of tetracycline to carbon nanotubes: a comparative study using activated carbon and graphite as adsorbents. Environ Sci Technol. 2009;43(7):2322-7. [PubMed] | [CrossRef] | [Google Scholar]
- Ji L, Shao Y, Xu Z, Zheng S, Zhu D. Adsorption of monoaromatic compounds and pharmaceutical antibiotics on carbon nanotubes activated by KOH etching. Environ Sci Technol. 2010;44(16):6429-36. [PubMed] | [CrossRef] | [Google Scholar]
- Kerkez-Kuyumcu Ö, Bayazit ŞS, Salam MA. Antibiotic amoxicillin removal from aqueous solution using magnetically modified graphene nanoplatelets. J Ind Eng Chem. 2016;36:198-205. [CrossRef] | [Google Scholar]
- Li G, Zhang D, Wang M, Huang J, Huang L. Preparation of activated carbons from Iris tectorum employing ferric nitrate as dopant for removal of tetracycline from aqueous solutions. Ecotoxicol Environ Saf. 2013;98:273-82. [PubMed] | [CrossRef] | [Google Scholar]
- Li M, Liu Y, Liu S, Shu D, Zeng G, Hu X, et al. Cu(II)-influenced adsorption of ciprofloxacin from aqueous solutions by magnetic graphene oxide/nitrilotriacetic acid nanocomposite: competition and enhancement mechanisms. Chem Eng J. 2017;319:219-28. [CrossRef] | [Google Scholar]
- Li S, Zhang X, Huang Y. Zeolitic imidazolate framework-8 derived nanoporous carbon as an effective and recyclable adsorbent for removal of ciprofloxacin antibiotics from water. J Hazard Mater. 2017;321:711-19. [PubMed] | [CrossRef] | [Google Scholar]
- Lv JM, Ma YL, Chang X, Fan SB. Removal and removing mechanism of tetracycline residue from aqueous solution by using Cu-13X. Chem Eng J. 2015;273:247-53. [CrossRef] | [Google Scholar]
- Mahvi AH, Mostafapour FK. Biosorption of tetracycline from aqueous solution by azolla filiculoides: equilibrium kinetic and thermodynamics studies. Fresenius Environ Bull. 2018;27(8):5759-67. [CrossRef] | [Google Scholar]
- Marzbali MH, Esmaieli M, Abolghasemi H, Marzbali MH. Tetracycline adsorption by H3PO4-activated carbon produced from apricot nut shells: A batch study. Process Saf Environ Prot. 2016;102:700-9. [CrossRef] | [Google Scholar]
- Moein H, Balarak D, Meshkinain A, Chandrika K, Yazdani N. Effects of operational parameters on the removal of tetracycline from aqueous solutions by electrocoagulation. Int J Pharm Investig. 2021;11(1):23-6. [CrossRef] | [Google Scholar]
- Mostafapour FK, Bazi M, Siddiqui SH, Bagheri H, Balarak D. Highly efficient adsorption and removal of amoxicillin from aqueous solution by magnetic graphene oxide nanocomposite. Int J Pharm Investig. 2021;11(4):384-8. [CrossRef] | [Google Scholar]
- Mostafapour FK, Haseeb S, Balarak D, Moein H, Sajadi AA, Jalalzaei Z, et al. Thermodynamic study of amoxicillin and naphthalene adsorption on activated carbon derived from Salvadora persica. Int J Pharm Investig. 2021;11(1):41-5. [CrossRef] | [Google Scholar]
- Naghsh N, Barnoos S, Zisti F, Chandrika K, Balarak D. Thermodynamic study of adsorption of amoxicillin on synthesized NiO of pharmaceutical wastewater. Int J Pharm Investigation. 2024;14(2):365-70. [CrossRef] | [Google Scholar]
- Peng H, Pan B, Wu M, Liu Y, Zhang D, Xing B, et al. Adsorption of ofloxacin and norfloxacin on carbon nanotubes: hydrophobicity- and structure-controlled process. J Hazard Mater. 2012;233-234:89-96. [PubMed] | [CrossRef] | [Google Scholar]
- Peng X, Hu F, Dai H, Xiong Q, Xu C. Study of the adsorption mechanisms of ciprofloxacin antibiotics onto graphitic ordered mesoporous carbons. J Taiwan Inst Chem Eng. 2016;65:472-81. [CrossRef] | [Google Scholar]
- Peterson JW, Petrasky LJ, Seymour MD, Burkhart RS, Schuiling AB. Adsorption and breakdown of penicillin antibiotic in the presence of titanium oxide nanoparticles in water. Chemosphere. 2012;87(8):911-17. [PubMed] | [CrossRef] | [Google Scholar]
- Rostamian R, Behnejad H. A comparative adsorption study of sulfamethoxazole onto graphene and graphene oxide nanosheets through equilibrium, kinetic and thermodynamic modeling. Process Saf Environ Prot. 2016;102:20-9. [CrossRef] | [Google Scholar]
- Sarker N, Fakhruddin AN. Removal of phenol from aqueous solution using rice straw as adsorbent. Appl Water Sci. 2017;7(3):1459-65. [CrossRef] | [Google Scholar]
- Wang F, Yang B, Wang H, Song Q, Tan F, Cao Y, et al. Removal of ciprofloxacin from aqueous solution by a magnetic chitosan grafted graphene oxide composite. J Mol Liq. 2016;222:188-94. [CrossRef] | [Google Scholar]
- Yu F, Li Y, Han S, Ma J. Adsorptive removal of antibiotics from aqueous solution using carbon Materials. Chemosphere. 2016;153:365-85. [PubMed] | [CrossRef] | [Google Scholar]
- Zhang Y, Jiao Z, Hu Y, Lv S, Fan H, Zeng Y, et al. Removal of tetracycline and oxytetracycline from water by magnetic Fe3O4@graphene. Environ Sci Pollut Res Int. 2017;24(3):2987-95. [PubMed] | [CrossRef] | [Google Scholar]
- Zhou Y, Jin X, Chen Z. The removal of amoxicillin from wastewater using organobentonite. Zha Sx. J Environ Manag. 2013;129:569-76. [PubMed] | [CrossRef] | [Google Scholar]
- Zisti F, Chandrika K, Balarak D. Effective adsorption of ciprofloxacin antibiotic using of novel magnetic Fe3O4/Activated carbon nanocomposite from aqueous solution. Int J Pharm Investigation. 2023;13(4):778-83. [CrossRef] | [Google Scholar]