ABSTRACT
This review explores the role of additive manufacturing, particularly 3D printing, as an innovative approach in targeted drug delivery. It effectively addresses the limitations of traditional methods, such as high costs, complex geometrics and difficulties in individualized medications. The advent of 3D printing offers a modern solution, enabling the manufacturing of customized 3D objects from digital models. The approval of Spritam® (levetiracetam) by the FDA, the first additive manufacturing tablet, is a clear indication of the demand for 3D printing. This technique, which creates products layer-by-layer through a two-step process of data transfer and print head movement in all three dimensions, is gaining popularity. The review explores the diverse additive manufacturing methods applicable to drug delivery systems, including Fused Deposition Modeling (FDM), Stereo Lithography (SLA) and others. FDM, in particular, stands out for its creativity and cost-effectiveness. Beyond drug delivery, additive manufacturing has found applications in tissue engineering, manufacturing of complicated geometries, controlled-release systems and individualized medication for specific patient needs. The technology empowers the creation of intricate, customized structures loaded with drugs, demonstrating great potential for targeted therapies and personalized medicine.
INTRODUCTION
The pharmaceutical field is constantly exploring new approaches to drug delivery, with a particular focus on targeted delivery methods. Targeted delivery enhances patient safety and medication compliance. However, the conventional method is unable to fulfill individual patient needs due to their focus on mass production (Gaurkhedeet al., 2021). Traditional methods, including encapsulation and compression, are expensive and struggle to produce highly complex medications. Additionally, they are ineffective in manufacturing personalized products (Tanet al., 2019). For this purpose, 3D printing, a rapidly expanding and revolutionary technology, can be employed to make additive items from electronic models (Glukhovaet al., 2022). The 3D printing process involves two key steps: (1) transferring data from software to the 3D printer and (2) using the print head to repeatedly deposit material in layers, building the object one layer at a time (Ramya and Vanapalli, 2016). 3D printing technology gained significant attention in August 2015 when the FDA approved Spritam® (levetiracetam) as the first additive manufacturing tablet (Samiei, 2020). The pharmaceutical industry has recently shown a surge of interest in additive printing. This is due to 3D printing’s unique capabilities, such as creating complex drug release patterns, intricate drug shapes and customized medications. Additionally, it enhances the effectiveness of drug loading into pharmaceutical products (Senet al., 2020). 3D printing possesses several key features, including affordability, accessibility and the ability to produce objects in any conceivable shape. These potential positions 3D printing as a technology with significant probability for advancements in both the pharmaceutical and biomedical fields.2 Three-dimensional printing is a rapid prototyping technique that creates products layer by layer. This enables the production of complex internal structures, such as hollow channels, internal walls and areas with varying materials and porosities. Besides, additive manufacturing allows the dispensation of multiple drugs within a single object, a capacity beyond the reach of traditional pharmaceutical manufacturing methods (Liet al., 2018). Contemporary advancements in 3D printing technology have extended implementation in oral drug administration. This is notable because successful drug delivery requires leveraging modern technologies like 3D printing and nanotechnology while ensuring safe medication delivery (Pandeyet al., 2020). In tissue engineering and drug delivery, additive printing enables the creation of intricate and personalized structures infused with medication, which is especially advantageous for pediatric and geriatric patients (Jamrózet al., 2018). 3D printing encompasses various techniques, such as Stereolithography (SLA), Fused Deposition Modeling (FDM), Powder Bed Fusion, Binder Jetting and Material Jetting. FDM is particularly notable for its versatility and affordability, making it the most commonly utilized method.
Several techniques are employed in the creation of 3D-printed objects, including Fused Deposition Modeling (FDM) (Noberet al., 2019), Binder jet 3D printers (Senet al., 2020) Stereolithography (SLA) (Wanget al., 2016), Extrusion-based modelling (Gaurkhedeet al., 2021). This review article examines how 3D printing has transformed the development of various Drug Delivery Systems (DDS), such as tablets, capsules, gels, novel dosage forms and transdermal patches. Additionally, 3D printing provides a unique capability to tackle the challenge of creating complex multi-drug tablets, where each drug is released according to a customized release profile (Khaledet al., 2015).
Current research aims to provide a comprehensive review of 3D printing technologies used in drug delivery systems. This review will cover various 3D printing methods, including Fused Deposition Modeling (FDM), Stereo Lithography (SLA) and Digital Light Processing (DLP), among others. It will delve into how these techniques are applied to develop a range of drug delivery systems, such as multi-layered tablets, hydrogels, nanoparticles, liposomes, niosomes and transdermal patches. Additionally, the review will discuss the specific functionalities and practical applications of each 3D printing method in this context.
MATERIALS AND METHODS
The study took place over four months, from February 10, 2024, to June 10, 2024. Ethical approval was granted by the Research Ethics Committee at the University of Biological and Applied Sciences (UBAS) in Lahore, Pakistan, under reference number RMEC/AM/09791, ensuring adherence to ethical standards and guidelines. This review primarily utilized PubMed and Cochrane databases. The study protocols followed the PRISMA flow statement guidelines. Research studies were identified using keywords such as ‘3D Printing,’ ‘Future Fabrication,’ 3D Printing Applications” and ‘2010-2024’. Additionally, various electronic databases and manual searches on Google Scholar were conducted to collect relevant studies for this review. The inclusion criteria focused on studies conducted in English and studies on 3d printing, modern dosage form fabrication and its applications between 2010 and 2024 in different world regions.
Only studies published in English were considered. The Prisma flow chart and appraisal tool for systematic review are given in Figure 1 and Table 1, respectively.
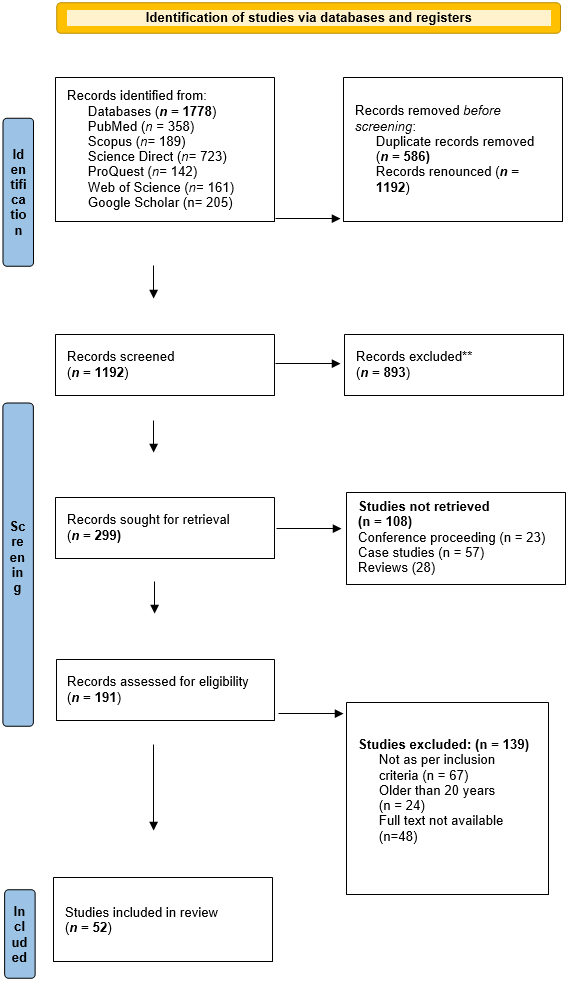
Figure 1:
PRISMA flow diagram for systematic review.
Parameters | Study 1 (Gaurkhedeet al., 2021) | Study 2 (Tan and Maniruzzaman, 2019) | Study 3 (Glukhovaet al., 2022) | Study 4 (Liet al.,2018) | Study 5 (Senet al., 2020) | Study 6 (Nober et al., 2019) | Study 7 (Wanget al., 2016) | Study 8 (Khaledet al., 2015) | Study 9 (Erkuset al., 2023) | Study 10(Petrováet al., 2024) | Study 11(Yeunget al., 2019) | Study 12 (Chenet al., 2022) | Study 13 (Bressanet al., 2019) | Study 14(Valenciaet al., 2022) | Study 15 (Sharmaet al., 2022) | Study 16 (Goyaneset al., 2015) | Study 17 (Pereiraet al., 2019) | Study 18 (Finaet al., 2018) | Study 19 (Finaet al., 2017) | Study 20 (Cho and Jammalamadaka, 2018) | Study21 (Chanet al., 2024) | Study 22 (Heet al., 2023) | Study 23 (Ballacchinoet al., 2021) | Study 24 (Wanget al., 2016) | Study 25 (Arcaute and Mann, 2010) | Study 26 (Curti and Kirby, 2024) | Study 27 (Xuet al., 2020) | Study 28 (Linareset al., 2023) | Study 29 (Eosolyet al., 2010) | Study 30 (Pandav and Karanwad, 2024) |
---|---|---|---|---|---|---|---|---|---|---|---|---|---|---|---|---|---|---|---|---|---|---|---|---|---|---|---|---|---|---|
Are study objectives specific? | ✓ | ✓ | ✓ | ✓ | ✓ | ✓ | ✓ | ✓ | ✓ | ✓ | ✓ | ✓ | ✓ | ✓ | ✓ | ✓ | ✓ | ✓ | ✓ | ✓ | ✓ | ✓ | ✓ | ✓ | ✓ | ✓ | ✓ | ✓ | ✓ | ✓ |
Is the study design suitable for aims? | ✓ | ✓ | ✓ | ✓ | ✓ | ✓ | ✓ | ✓ | ✓ | ✓ | ✓ | ✓ | ✓ | ✓ | ✓ | ✓ | ✓ | ✓ | ✓ | ✓ | ✓ | ✓ | ✓ | ✓ | ✓ | ✓ | ✓ | ✓ | ✓ | ✓ |
Was the basic data adequately described? | ✓ | ✓ | ✓ | ✓ | ✓ | ✓ | ✓ | ✓ | ✓ | ✓ | ✓ | ✓ | ✓ | ✓ | ✓ | ✓ | ✓ | ✓ | ✓ | ✓ | ✓ | ✓ | ✓ | ✓ | ✓ | ✓ | ✓ | ✓ | ✓ | ✓ |
Were results internally consistent? | ✓ | ✓ | ✓ | ✓ | ✓ | ✓ | ✓ | ✓ | ✓ | ✓ | ✓ | ✓ | ✓ | ✓ | ✓ | ✓ | ✓ | ✓ | ✓ | ✓ | ✓ | ✓ | ✓ | ✓ | ✓ | ✓ | ✓ | ✓ | ✓ | ✓ |
Were the results presented for all the analyses described in the methods? | ✓ | ✓ | ✓ | ✓ | ✓ | ✓ | ✓ | ✓ | ✓ | ✓ | ✓ | ✓ | ✓ | ✓ | ✓ | ✓ | ✓ | ✓ | ✓ | ✓ | ✓ | ✓ | ✓ | ✓ | ✓ | ✓ | ✓ | ✓ | ✓ | ✓ |
Are discussion and conclusions justified by results? | ✓ | ✓ | ✓ | ✓ | ✓ | ✓ | ✓ | ✓ | ✓ | ✓ | ✓ | ✓ | ✓ | ✓ | ✓ | ✓ | ✓ | ✓ | ✓ | ✓ | ✓ | ✓ | ✓ | ✓ | ✓ | ✓ | ✓ | ✓ | ✓ | ✓ |
Were the limitations of the study discussed? | ✗ | ✓ | ✓ | ✓ | ✓ | ✗ | ✗ | ✗ | ✗ | ✗ | ✗ | ✗ | ✗ | ✗ | ✓ | ✗ | ✗ | ✗ | ✗ | ✗ | ✗ | ✗ | ✗ | ✓ | ✗ | ✗ | ✗ | ✗ | ✗ | ✗ |
Is there any conflict of interest that might affect study findings? | ✗ | ✗ | ✗ | ✗ | ✗ | ✗ | ✗ | ✗ | ✗ | ✗ | ✗ | ✗ | ✗ | ✗ | ✗ | ✗ | ✗ | ✗ | ✗ | ✗ | ✗ | ✗ | ✗ | ✗ | ✗ | ✗ | ✗ | ✗ | ✗ | ✗ |
Parameters | Study 31 (Finaet al., 2017) (Finaet al., 2017) | Study 32 (Lekurwale and Karanwad, 2022) | Study 33 (Finaet al., 2018) | Study 34 (Adamovet al., 2022) | Study 35(Kadryet al., 2019) | Study36 (Xuet al., 2021) | Study 37 (Wuet al., 2019) | Study 38 (Yanget al., 2020) | Study 39 (Madžarević, 2021) | Study 40 (Xuet al., 2021) | Study 41 (Liet al., 2022) | Study 42 (Papadimitriouet al., 2022) | Study 43 (Xenikakis and Tsongas, 2021) | Study 44 (Cristaldiet al., 2021) | Study 45 (Cristaldi, 2020) | Study 46 (De Grandiet al., 2022) | Study 47 (Heet al., 2023) | Study 48 (Tan and Maniruzzaman, 2018) | Study 49 (Cailleaux and Sanchez-Ballester, 2021) | Study 50 (Mathewet al., 2020) | Study51 (Pireset al., 2020) | Study 52(Khalid, 2022) |
---|---|---|---|---|---|---|---|---|---|---|---|---|---|---|---|---|---|---|---|---|---|---|
Are study objectives specific? | ✓ | ✓ | ✓ | ✓ | ✓ | ✓ | ✓ | ✓ | ✓ | ✓ | ✓ | ✓ | ✓ | ✓ | ✓ | ✓ | ✓ | ✓ | ✓ | ✓ | ✓ | ✓ |
Is the study design suitable for aims? | ✓ | ✓ | ✓ | ✓ | ✓ | ✓ | ✓ | ✓ | ✓ | ✓ | ✓ | ✓ | ✓ | ✓ | ✓ | ✓ | ✓ | ✓ | ✓ | ✓ | ✓ | ✓ |
Was the basic data adequately described? | ✓ | ✓ | ✓ | ✓ | ✓ | ✓ | ✓ | ✓ | ✓ | ✓ | ✓ | ✓ | ✓ | ✓ | ✓ | ✓ | ✓ | ✓ | ✓ | ✓ | ✓ | ✓ |
Were results internally consistent? | ✓ | ✓ | ✓ | ✓ | ✓ | ✓ | ✓ | ✓ | ✓ | ✓ | ✓ | ✓ | ✓ | ✓ | ✓ | ✓ | ✓ | ✓ | ✓ | ✓ | ✓ | ✓ |
Were the results presented for all the analyses described in the methods? | ✓ | ✓ | ✓ | ✓ | ✓ | ✓ | ✓ | ✓ | ✓ | ✓ | ✓ | ✓ | ✓ | ✓ | ✓ | ✓ | ✓ | ✓ | ✓ | ✓ | ✓ | ✓ |
Are discussion and conclusions justified by results? | ✓ | ✓ | ✓ | ✓ | ✓ | ✓ | ✓ | ✓ | ✓ | ✓ | ✓ | ✓ | ✓ | ✓ | ✓ | ✓ | ✓ | ✓ | ✓ | ✓ | ✓ | ✓ |
Were the limitations of the study discussed? | ✗ | ✗ | ✗ | ✗ | ✗ | ✗ | ✗ | ✗ | ✗ | ✗ | ✗ | ✗ | ✗ | ✗ | ✗ | ✗ | ✗ | ✗ | ✗ | ✗ | ✗ | ✗ |
Is there any conflict of interest that might affect study findings? | ✗ | ✗ | ✗ | ✗ | ✗ | ✗ | ✗ | ✗ | ✗ | ✗ | ✗ | ✗ | ✗ | ✗ | ✗ | ✗ | ✗ | ✗ | ✗ | ✗ | ✗ | ✗ |
Exclusion Criteria
The exclusion criteria included:
- Conventional dosage form design.
- Traditional methods of formulation development.
- Research articles written in languages other than English.
- Studies conducted before 2010.
Data Extraction
The extracted data from the included studies comprised author details, the year of the study, the 3D printing device, its history, construction, fabrication procedure and application.
The PRISMA 2020 statement: an updated guideline for reporting systematic reviews.
For more information, visit: http://www.prisma-statement.org/
3D printing devices
Fused Deposition Modeling (FDM)
In 1988, Steven Scott Crump developed the Fused Deposition Modeling (FDM) method, later founding Stratasys and commercializing the process in the following year (Günaydın and Süleyman Türkmen, 2018). FDM has since become a widely used 3D printing technique for drug delivery systems (Goyaneset al., 2015).
FDM facilitates the creation of hollow objects and dosage forms with varied drug release profiles, offering high-resolution and precise dosage control through computer settings (Goyaneset al., 2015). The components of fused deposition modeling include a Filament roll from where the filament holds drug and polymer passes towards heated rollers. The heated material is released from the nozzle to a moveable base/platform, where 3D objects are prepared. The graphical representation of fused deposition modeling is shown in Figure 2.
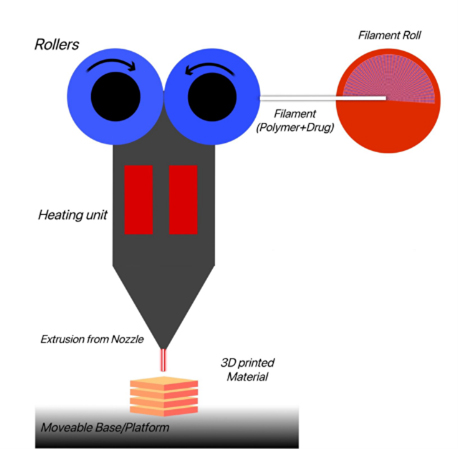
Figure 2:
Fused Deposition Modelling.
Fused Deposition Modeling (FDM) operates by passing an extruded polymer filament through a heated coil, melting the polymer, which is then deposited onto a platform where it solidifies. By relying on these solidified layers, a 3D object is formed. FDM enables the creation of hollow objects and dosage forms with varying drug release profiles by adjusting the formulation’s infill density or surface area-to-volume ratio. This technique, known as Hot-Melt Extrusion (HME), is commonly used in the pharmaceutical industry to integrate drugs within a carrier matrix at the molecular level, allowing for the development of solid solutions for drug delivery systems with higher drug-loading capacity (Goyaneset al., 2015).
Microfluidics is used for nanoparticles, with reactors essentially made from conventional materials. 3D printing is being used, with fused deposition modeling being a less expensive option (Bressanet al., 2019). Fused deposition modeling, in which an extruded polymer crosses through a heating coil. The polymer softens by heat and then is placed onto a plate to solidify. Then, layers of hardened polymer are deposited and form a 3D object. This printing method can form hollow objects and dosage forms in different drug release profiles. The final step involves adjusting the surface area-to-volume ratio or infill density of the formulation. Compared to earlier printing methods, FDM 3D printing provides higher resolution and allows for improved dosage precision, which can be readily adjusted by modifying computer settings (Goyaneset al., 2015).
Selective Laser Sintering technique (SLS)
SLS, a 3D printing technique, is utilized for the development of innovative solid dosage forms (Finaet al., 2018). At room temperature, this method is advantageous for producing objects with good resolution (Finaet al., 2017).
The study’s objective is to show that SLS is easy to use to form novel solid dosage forms with instant drug release qualities to make the oral disintegrating formulations (Finaet al., 2018). The components of SLS are a Laser, scanner, recoated blade, power bed, power tank and building plate as shown in Figure 3 (Finaet al., 2017).
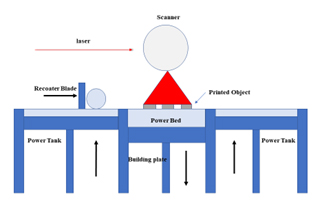
Figure 3:
Selective Laser Sintering.
Copolymers and polymers were combined individually with colorant and 5% active ingredient. The powder mixtures were then printed using SLS technology to form printlet. Printlets hold active ingredients, which have recently been produced by using various 3-D printing processes called stereolithography. Drug-containing photopolymerizable polymer solution is solidified using a laser with SLA technology. At room temperature, this method has the advantage of forming objects with good resolution (Finaet al., 2017).
Stereolithography (SLA)
Stereolithography is a fast, economical, one-step method for producing multi-scale aspects and combining them with microfluidic-microneedle devices at the same time (Yeunget al., 2019). Among the various forms of 3D printing, Stereo Lithography (SLA) stands out for its capability to create objects through the formation of interconnected polymer matrices via resin cross-linking (Choet al., 2018).
3D printing has emerged as a powerful technique for fabricating 3D nanogels. Nanogels are hydrogels formed by cross-linking nanoscopic micelles dispersed in a liquid medium. Most nanogels are produced by incorporating drug(s) or photo-initiator-loaded nanoparticles, liposomes, or nano-emulsions into hydrogels using 3D printing methods (Choet al., 2018). To enhance the capabilities of Stereo Lithography (SLA), components typically include a resin vat, UV chamber, biocompatible resins and composite materials, as illustrated in Figure 4 (Yeunget al., 2019).
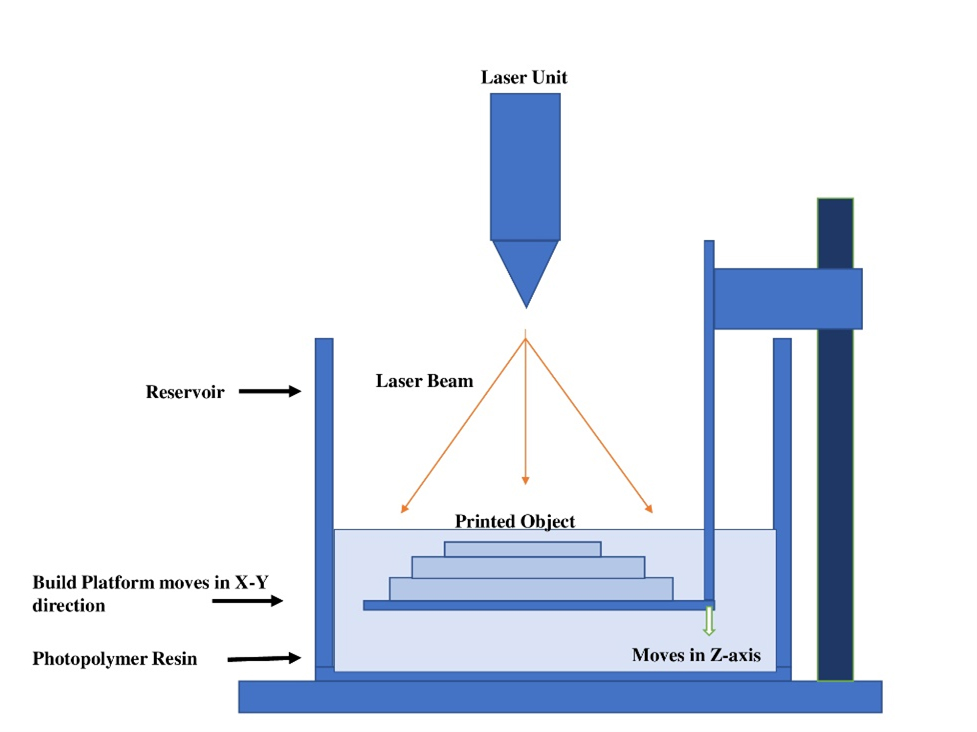
Figure 4:
Stereo Lithography (SLA).
Stereolithography exhibits a vat polymerization process. In resin vat, the laser is directed to a certain depth, resulting in localized polymerization and consequent solidification. The intensity of the light source, scanning speed, exposure time, quantity of polymer and photo-initiator employed and various other factors collectively determine the energy imparted by the laser during SLA printing. Layer-by-layer solidification proceeds until a complete, three-dimensional object is produced (Wanget al., 2016). A new composite substance with added metal is necessary to enhance the SLA application and mechanical properties. Ag nanoparticles are a promising class of nanofillers for obtaining composites with optical and electrical properties, hence expanding the range of applications for SLA into domains such as biomedical or electronic.
In the manufacturing of patches, the solidification of liquid resin to obtain the desired object in the SLA method relies on photopolymerization. Solidification is continued until solid; the 3D object is manufactured (Wanget al., 2016). An SLA printer and Class IIa biocompatible resin were utilized to create a microfluidic-enabled microneedle device. The product underwent preprocessing using 3D printer preparation software and was designed using Computer-Aided Design (CAD) software. To enhance productivity and quality, the object was positioned at a 45º angle. To optimize the stiffness and strength of the microneedle patch, SLA-printed components were cleaned in isopropyl alcohol for 5 min and then cured in a UV chamber with a 405nm wavelength at 80ºC for 20 min. A single-piece, three-dimensional microfluidic device with a multi-inlet and integrated hollow microneedle array was produced using SLA 3D printing. Underneath the construction platform is an optical window through which the printer in use applies UV light, enabling additive manufacturing of the intended model through the layer-after-layer curing of photopolymer resin inside the resin tank (Yeunget al., 2019).
Digital Light Processing (DLP)
It is a 3D printing technique based on photopolymerization. By this technique, irradiation photopolymer hardens layer by layer as the sections of the model are stick out onto the liquid photopolymer’s surface by the digital micromirror element (Erkuset al., 2023).
Digital light processing is a helpful technique for producing a digital workflow for personalized medicine. There is evidence of its effectiveness in applications in biomedical areas such as dental prostheses and tissue engineering (Yanget al., 2020). The initial functional component is a digital micromirror device made of several adjustable mirror sizes of microns, as shown in Figure 5.
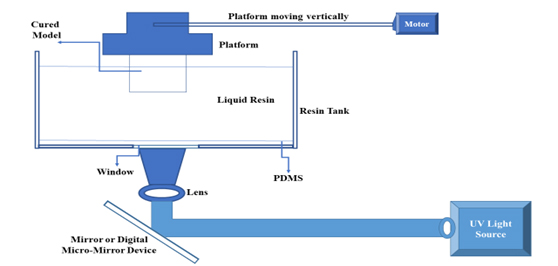
Figure 5:
Digital Light Processing.
This method employs light sources that direct light onto photosensitive material using an array of chipsets based on optical microelectromechanical technology. A rotating mirror controls the path of light, projecting it onto photosensitive resins. The resolution of digital light processing-based 3D printing is determined by the projection plane, which is modified by the DMD (Digital Micromirror Device) and lens. Ultimately, digital light processing achieves high-resolution (Zhanget al., 2020). Microneedles were prepared with the help of a computer-aided program named Tinker CAD. After printing, every patch consisted of 36 conical microneedles measuring 1mm in diameter and 1.5 mm in height. After being changed to STL format, the design was connected to the Lumen X 3D printer. In the printing process, a solvent and resin are utilized. Following printing, the patch was gently removed, rinsed in 70% ethanol and then exposed to a UV chamber for 1 min (Petrováet al., 2024). In contrast to other techniques, DLP is less susceptible to oxygen inhibition. As compared to the rest of the 3D printing techniques, DLP provides higher resolution and faster printing (Erkuset al., 2023). Rapid modifications to the final patch’s size and shape are another merit of employing DLP printing, contrary to the conventional method (Petrováet al., 2024).
LCD-printed microfluidic devices
LCD 3D printing, combined with specially formulated ink, paves the way for anyone, anywhere, to access high-resolution formulating of ready-to-use microfluidic and organ-on-a-chip devices. This technology even holds promise for the preparation of liposomes (Heet al., 2023).
The liposomes made with microfluidics have better encapsulation efficiency than other techniques (Ballacchinoet al., 2021). Printer platform, printed object, resin vat, power switch, USB port, LED source, touch screen and platform securing knob as shown in Figure 6 (Ballacchinoet al., 2021).
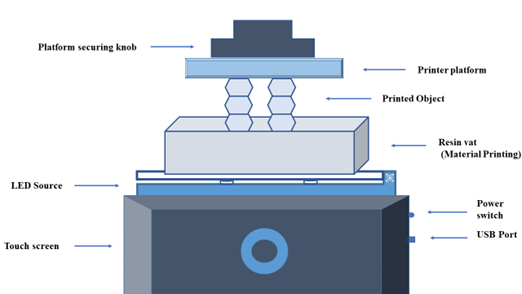
Figure 6:
LCD Printed Microfluidic Device.
The designed microfluidic devices were used in this work to manufacture liposomes. It entails the fabrication of various microfluidic structures, particularly Y-shaped mixers, featuring different configurations with two inlets and one outlet. A continuous flow of lipids in an alcohol solution and another in a wet solution pass through separate channels. Liposome production is achieved by merging these two channels where the streams converge. Therefore, the mixing is determined by the diffusion process occurring at the boundary between liquids, resulting in the formation of micelles as the lipids precipitate in the wet solutions, followed by the formation of liposomes (Ballacchinoet al., 2021).
3D-printed Reactor-in-a-Centrifuge (RIAC) device
RIAC is a simple, pump-free technique that requires a 3D-printed flow-through reactor and is powered by a standard centrifuge (Andreaet al., 2021).
The RIAC device is used to formulate liposomes and silver nanospheres, which are used in the production of nanoparticles (Andreaet al., 2021). The components used in the formulation of liposomes are the reservoir, centrifuge tube, polymer stabilizer and test tube, as shown in Figure 7 (Heet al., 2023).
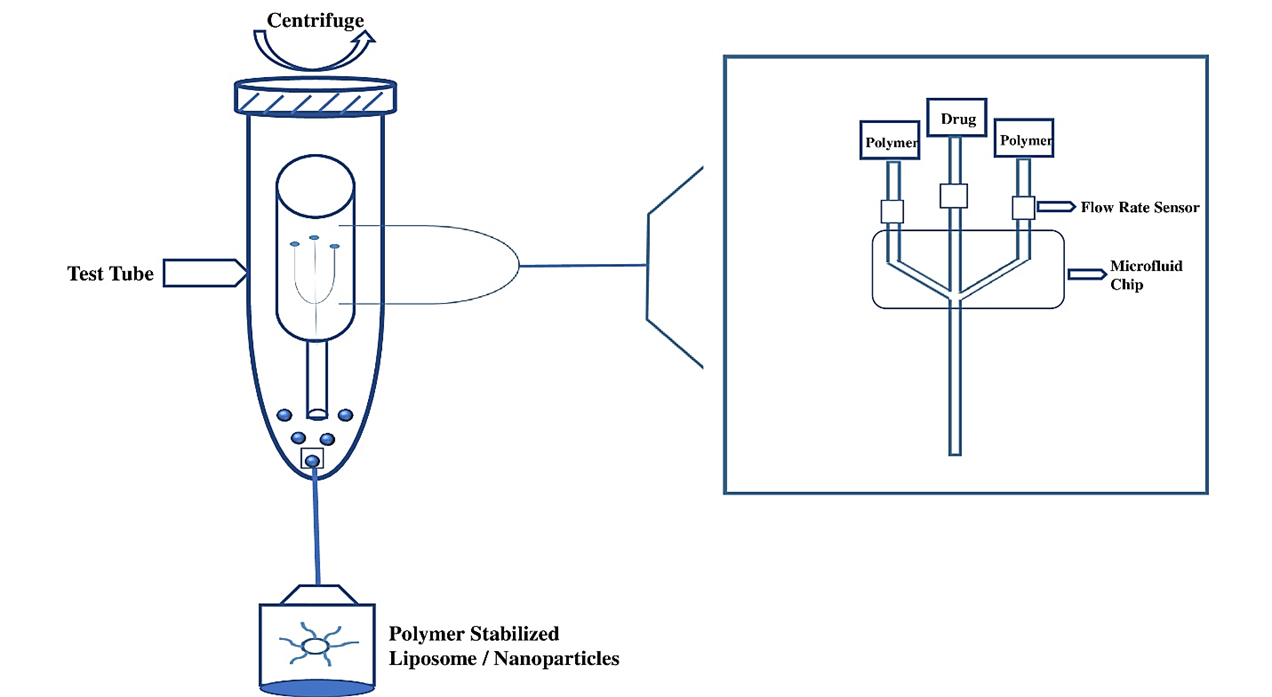
Figure 7:
Reactor in a centrifuge (RIAC) Device.
Another device is a 3D-printed RIAC device in which a single reservoir was pipetted with a lipid solution of 2 mL, which contained different molar ratios of stabilizer, solubilizer, surfactant, polymer stabilizer and membrane stabilizer to produce liposomes and optimize the formulation parameters. After being preheated to the appropriate temperature, Deionized water was transferred into the second reservoir. Additionally, 6 mL of water was added to the bottom of a 50 mL centrifuge tube containing the RIAC. This water addition was aimed at reducing the tendency of liposomes to aggregate. Increasing this volume beyond 6 mL would not significantly alter the properties of the liposomes. However, modifying production parameters such as time (between 1000 and 2000 cf) and centrifugal force would further decrease the concentration of liposomes in the final product (Heet al., 2023).
Applications of 3d Printed Devices
Fused Deposition Modelling (FDM)
FDM provides cost-effectiveness, design flexibility and exceptional reproducibility in pharmaceutical production procedures (Tanet al., 2018). This technology aids the production of complicated geometries swiftly from digital design without any necessity of moulds or other traditional industrial procedures (Cailleauxet al., 2021). FDM printing technology can be utilized in the development of Controlled-release and floating gastroretentive tablets. Due to its accuracy, versatility and ease of use in producing various dosage forms, including implants, capsules, films and adhesives, 3D printing by fused deposition modeling contains great potential for therapeutic customization (Pireset al., 2020). Solid dispersions can enhance the solubility of APIs by utilizing suitable polymers. Combining solid dispersions with FDM offers new technological possibilities that address the challenge of low API solubility (Khalid and Billa, 2022).
Stereolithography (SLA)
SLA is used for the development of controlled-release drug delivery (Xuet al., 2020). It finds applications in the field of tissue engineering. SLA is used to develop multi-material 3-D structures with specified characteristics (Arcaute and Mann, 2010). SLA is also used for thermolabile drugs and avoids flowability issues (Arcauteet al., 2010). SLA can form printable resins (Linareset al., 2023).
Selective Laser Sintering (SLS)
SLS printers are capable of producing bilayer objects. In industries, SLS is used for different manufacturing processes, such as plastic, metallic and ceramic products. The necessity of excipients and solvents can be minimized. Also, it recycles and reprocesses feedstock. SLS is used to form porous scaffolds with intricate geometries both internally and externally. SLS devices produce SLS-mediated hollow capsular shells (Linareset al., 2023).
Digital Light Processing (DLP)
DLP is effective for establishing a digital workflow in personalized medicine, as evidenced by its applications in biomedical fields such as dental prostheses and tissue engineering. Hydrogel, microneedles and dental models can be manufactured (Pandavet al., 2024). Drug delivery systems such as nose patches, intravaginal rings, oral dosage forms, bladder devices, microneedles and dental implants can be produced with the help of DLP (Adamovet al., 2022). It is also used in computational imaging, multiplexing and hyperspectral imaging (Xuet al., 2021). Designing acrylate-based photosensitive resin through 4D printing can be done through DLP (Kadryet al., 2019).
LCD 3D Printer
Liquid Crystal Display (LCD) technology is utilized to manufacture solid oral dosage forms, where active substances can be blended with photopolymer before printing and encapsulated within solidified matrices (Wuet al., 2019). In mobile-based pharmaceutical supply systems it is clearly advantageous for patients who live far away, as it improves access to healthcare by allowing them to receive their medications directly (Madžarević and Ibrić, 2021). Liquid crystal display 3D printing helps in the formulation of different placebo moulds of VOR tablets (Xuet al., 2021). Microneedle arrays are successfully constructed employing 3D printing methods such as liquid crystal display (Papadimitriouet al., 2022). By using a liquid crystal display, the human microneedles were fabricated, which provides the accuracy that is essential for microstructures like human Microneedles (Papadimitriouet al., 2022).
Reactor in a Centrifuge (RIAC)
The efficacy and accuracy of the reactors were demonstrated through the production of inorganic and organic nanoparticles. It is used in the formulation of nanoscale liposomes within the required size range, that is, diameter (80-300 nm). It is also used to produce silver nanospheres at selected operational settings, which have significance in drug delivery for infectious and cancerous disease treatment and imaging techniques. Cationic liposomes have become a necessary DDS due to the advancement in the fields of gene therapy and mRNA-based vaccines. Due to their compatibility and effectual transfection, results have led to the development of functionalized liposomal formulation through RIAC. RIAC is used to formulate liposomes and silver nanospheres (Andreaet al., 2021). The graphical representation of the 3d printing application is illustrated in Figure 8.
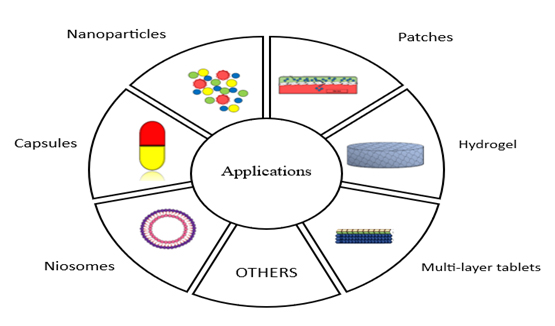
Figure 8:
Application of 3D Printing Devices.
CONCLUSION
This review comprehensively explored the development procedures and diverse applications of 3D printing technologies in fabricating a range of drug delivery systems. From FDM-printed controlled-release tablets to DLP-manufactured microneedles, 3D printing offers unparalleled flexibility and customization compared to traditional methods. Notably, 3D printing empowers the creation of personalized medicine, a prospect previously limited by conventional manufacturing. While challenges like high costs and complex material requirements remain, 3D printing undoubtedly presents a transformative platform for the pharmaceutical industry, paving the way for more precise, patient-centric and on-demand drug delivery systems.
Cite this article:
Mustafa MA, Malik A, Javed E, Amjad A, Iqbal S, Mustafa E. A Comparative Review of 3D Printing Technologies and their Applications: A Systematic Review for Future of Medicine Fabrication. Int. J. Pharm. Investigation. 2025;15(2):10-8.
ACKNOWLEDGEMENT
We want to extend our sincere gratitude to the Deanship of the Faculty of Pharmaceutical Sciences at Lahore University of Biological and Applied Sciences (UBAS) for providing the necessary resources and support that made this research possible. Their commitment to fostering academic excellence has been invaluable.
ABBREVIATIONS
FDM | Fused Deposition Modeling |
---|---|
RIAC | Reactor in a centrifuge |
SLA | Stereo lithography |
DLP | Digital Light Processing |
HME | Hot-melt extrusion |
DDS | Drug delivery system |
LCD | Liquid Crystal Display |
CAD | Computer-Aided Design |
DMD | Digital Micromirror Device |
FDA | Food and drug administration |
Ag | Silver |
References
- . (Array) Elsevier [Internet]. undefined. Formulation and characterization of immediate-release oral dosage forms with zolpidem tartrate fabricated by digital light processing (DLP) 3D printing technique. ElsevierI Adamov, G Stanojević, D Medarević, B Ivković, D Kočović, D Mirković, S IbrićInternational journal of pharmaceutics
- . (Array) Elsevier [Internet]. undefined. Formulation and characterization of immediate-release oral dosage forms with zolpidem tartrate fabricated by digital light processing (DLP) 3D printing technique. ElsevierI Adamov, G Stanojević, D Medarević, B Ivković, D Kočović, D Mirković, S IbrićInternational journal of pharmaceutics
- Andrea Cristaldi D., Labanca A., Donal Pottinger T., Owen J., Stulz E., Zhang X., Carugo D., et al. (2021) 3D printed reactor-in-a-centrifuge (RIAC): Making flow-synthesis of nanoparticles pump-free and cost-effective. Chemical Engineering Journal 425: 3D Google Scholar
- Arcaute K., Mann B.. (Array) Elsevier [Internet]. biomaterialia RWA, 2010 undefined. Stereolithography of spatially controlled multi-material bioactive poly (ethylene glycol) scaffolds. ElsevierK Arcaute, B Mann, R WickerActa biomaterialia Google Scholar
- Arcaute K., Mann B., Wicker R.. (2010) Stereolithography of spatially controlled multi-material bioactive poly(ethylene glycol) scaffolds. Acta Biomaterialia 6: 1047-1054 Google Scholar
- . Manufacturing of 3D-printed microfluidic devices for the synthesis of drug-loaded liposomal formulations p. 2021. mdpi.comG Ballacchino, E Weaver, E Mathew, R Dorati, I Genta, B Conti, DA LamprouInternational Journal of Molecular Sciences, 2021•mdpi.com [Internet] Google Scholar
- Ballacchino G., Weaver E., Mathew E., Dorati R., Genta I., Conti B., Lamprou D. A., et al. (2021) Manufacturing of 3d-printed microfluidic devices for the synthesis of drug-loaded liposomal formulations. International Journal of Molecular Sciences 22 Google Scholar
- Bressan L., Robles-Najar J., Adamo C.. (Array) Elsevier [Internet]. 2019 undefined. 3D-printed microfluidic device for the synthesis of silver and gold nanoparticles. ElsevierLP Bressan, J Robles-Najar, CB Adamo, RF Quero. BMC, DP de Jesus, JAF da SilvaMicrochemical journal Google Scholar
- Bressan L. P., Robles-Najar J., Adamo C. B., Quero R. F., Costa B. M. C., de Jesus D. P., da Silva J. A. F., et al. (2019) 3D-printed microfluidic device for the synthesis of silver and gold nanoparticles. Microchemical Journal 146: 1083-1089 Google Scholar
- . (Array) Elsevier [Internet]. undefined. Fused deposition modeling (FDM), the new asset for the production of tailored medicines. ElsevierS Cailleaux, NM Sanchez-Ballester, YA Gueche, B Bataille, I SoulairolJournal of controlled release Google Scholar
- Cailleaux S., Sanchez-Ballester N. M., Gueche Y. A., Bataille B., Soulairol I.. (2021) Fused deposition modeling (FDM), the new asset for the production of tailored medicines. Journal of Controlled Release 330: 821-841 Google Scholar
- Chan A. K. C., Ranjitham Gopalakrishnan N., Traore Y. L., Ho E. A.. (2024) Formulating biopharmaceuticals using three-dimensional printing. Journal of Pharmacy and Pharmaceutical Sciences 27: 12797 Google Scholar
- Chen Z., Wu H., Zhao S., Chen X., Wei T., Peng H., Chen Z., et al. (2022) 3D-printed integrated ultrasonic microneedle array for rapid transdermal drug delivery. Molecular Pharmaceutics 19: 3314-3322 Google Scholar
- Cho H., Jammalamadaka U.. (Array) Materials KT, 2018 undefined. Nanogels for pharmaceutical and biomedical applications and their fabrication using 3D printing technologies. H Cho, U Jammalamadaka, K TappaMaterials, 2018. mdpi.com. mdpi. com [Internet] Google Scholar
- Cho H., Jammalamadaka U., Tappa K.. (2018) Nanogels for pharmaceutical and biomedical applications and their fabrication using 3D printing technologies. Materials 11 Google Scholar
- Cristaldi D.. (Array) Continuous-flow reactors for large-scale production of nanoparticles Google Scholar
- . (2021) undefined, 3d printed reactor-in-a-centrifuge (RIAC): Making flow-synthesis of nanoparticles pump-free and cost-effective. ElsevierDA Cristaldi, A Labanca, TD Pottinger, J Owen, E Stulz, X Zhang, D CarugoChemical Engineering Journal, 2021•Elsevier [Internet] Google Scholar
- Curti C., Kirby D.. (Array) Elsevier [Internet]. Pharmaceutics CRIJ of, 2024 undefined. Systematic screening of photopolymer resins for stereolithography (SLA) 3D printing of solid oral dosage forms: Investigation of formulation factors on. ElsevierC Curti, DJ Kirby, CA RussellInternational. Journal of Pharmaceutics Google Scholar
- Curti C., Kirby D. J., Russell C. A.. (2024) Systematic screening of photopolymer resins for stereolithography (SLA) 3D printing of solid oral dosage forms: Investigation of formulation factors on printability outcomes. International Journal of Pharmaceutics 653: 123862 Google Scholar
- De Grandi D., Meghdadi A., LuTheryn G.. (Array) undefined. Facile production of quercetin nanoparticles using 3D printed centrifugal flow reactors. D De Grandi, A Meghdadi, G LuTheryn, D CarugoRSC advances, 2022 p. 2022. pubs.rsc. org. pubs.rsc.org [Internet] Google Scholar
- Eosoly S., Brabazon D., Lohfeld S.. (Array) Elsevier [Internet]. biomaterialia LLA, 2010 undefined. Selective laser sintering of hydroxyapatite/polyε-caprolactone scaffolds. ElsevierS Eosoly, D Brabazon, S Lohfeld, L LooneyActa biomaterialia Google Scholar
- Erkus H., Bedir T., Kaya E., Tinaz G., Materialia OG. (Array) undefined. Innovative transdermal drug delivery system based on amoxicillin-loaded gelatin methacryloyl microneedles obtained by 3D printing. Elsevier [Internet] Google Scholar
- Erkus H., Bedir T., Kaya E., Tinaz G. B., Gunduz O., Chifiriuc M.-C., Ustundag C. B., et al. (2023) Innovative transdermal drug delivery system based on amoxicillin-loaded gelatin methacryloyl microneedles obtained by 3D printing. Materialia 27 Google Scholar
- Fina F., Goyanes A., Gaisford S.. (Array) Elsevier [Internet]. of ABI journal, 2017 undefined. Selective laser sintering (SLS) 3D printing of medicines. ElsevierF Fina, A Goyanes, S Gaisford, AW BasitInternational journal of pharmaceutics Google Scholar
- Fina F., Goyanes A., Gaisford S., Basit A. W.. (2017) Selective laser sintering (SLS) 3D printing of medicines. International Journal of Pharmacy 529 Google Scholar
- . (2018) undefined. 3D printing of drug-loaded gyroid lattices using selective laser sintering. ElsevierF Fina, A Goyanes, CM Madla, A Awad, SJ Trenfield, JM Kuek, P Patel, S Gaisford, AW BasitInternational journal of pharmaceutics, 2018•Elsevier [Internet] Google Scholar
- . (2018) undefined. Fabricating 3D printed orally disintegrating printlets using selective laser sintering. ElsevierF Fina, CM Madla, A Goyanes, J Zhang, S Gaisford, AW BasitInternational journal of pharmaceutics, 2018•Elsevier [Internet] Google Scholar
- Fina F., Madla C. M., Goyanes A., Zhang J., Gaisford S., Basit A. W., et al. (2018) Fabricating 3D printed orally disintegrating printlets using selective laser sintering. International Journal of Pharmaceutics 541: 101-107 Google Scholar
- Gaurkhede S. G., Osipitan O. O., Dromgoole G., Spencer S. A., Pasqua A. J. D., Deng J., et al. (2021) 3D printing and dissolution testing of novel capsule shells for use in delivering acetaminophen. Journal of Pharmaceutical Sciences 110: 3829-3837 Google Scholar
- . (2022) undefined. Green nanocomposite gels based on binary network of sodium alginate and percolating halloysite clay nanotubes for 3D printing. ElsevierSA Glukhova, VS Molchanov, YM Chesnokov, BV Lokshin, EP Kharitonova, OE PhilippovaCarbohydrate Polymers, 2022•Elsevier [Internet] Google Scholar
- Glukhova S. A., Molchanov V. S., Chesnokov Y. M., Lokshin B. V., Kharitonova E. P., Philippova O. E., et al. (2022) Green nanocomposite gels based on binary network of sodium alginate and percolating halloysite clay nanotubes for 3D printing. Carbohydrate Polymers 282: 119106 Google Scholar
- . (Array) Elsevier [Internet]. undefined. Fabrication of controlled-release budesonide tablets via desktop (FDM) 3D printing. ElsevierA Goyanes, H Chang, D Sedough, GB Hatton, J Wang. A Buanz, S Gaisford, AW BasitInternational journal of pharmaceutics Google Scholar
- Goyanes A., Chang H., Sedough D., Hatton G. B., Wang J., Buanz A., Gaisford S., Basit A. W., et al. (2015) Fabrication of controlled-release budesonide tablets via desktop (FDM) 3D printing. International Journal of Pharmaceutics 496: 414-420 Google Scholar
- He Y., De Grandi D. D., Chandradoss S., Lutheryn G., Cidonio G., Nunes Bastos R., Pereno V., Carugo D., et al. (2023) mdpi.com [Internet] Cited July 29, 2024. Rapid production of nanoscale liposomes using a 3D-printed reactor-in-A-centrifuge: Formulation, characterisation and super-resolution imaging. mdpi.com. Micromachines 14: 1763 Google Scholar
- He Y., Grandi D., Chandradoss S., Micromachines G. L.. (Array) undefined. Rapid production of nanoscale liposomes using a 3D-printed reactor-in-A-centrifuge: Formulation, characterisation and super-resolution imaging. Y He, DD Grandi, S Chandradoss, G LuTheryn, G cidonio, R Nunes Bastos, V PerenoMicromachines. mdpi.com. mdpi.com [Internet] Google Scholar
- Jamróz W., Szafraniec J., Kurek M., Jachowicz R.. (2018) 3D printing in pharmaceutical and medical applications – Recent achievements and challenges. Pharmaceutical Research 35: 176 Google Scholar
- Kadry H., Wadnap S., Xu C.. (Array) Elsevier [Internet]. Pharmaceutical FAEJ of, 2019 undefined. Digital light processing (DLP) 3D-printing technology and photoreactive polymers in fabrication of modified-release tablets. ElsevierH Kadry, S Wadnap, C Xu, F AhsanEuropean. Journal of Pharmaceutical Sciences Google Scholar
- Kadry H., Wadnap S., Xu C., Ahsan F.. (2019) Digital light processing (DLP)3D-printing technology and photoreactive polymers in fabrication of modified-release tablets. European Journal of Pharmaceutical Sciences 135: 60-67 Google Scholar
- . (Array) Elsevier [Internet]. undefined. 3D printing of tablets containing multiple drugs with defined release profiles. ElsevierSA Khaled. JC, MR Alexander, J Yang, CJ RobertsInternational journal of pharmaceutics Google Scholar
- Khaled S. A., Burley J. C., Alexander M. R., Yang J., Roberts C. J.. (2015) 3D printing of tablets containing multiple drugs with defined release profiles. International Journal of Pharmaceutics 494: 643-650 Google Scholar
- Khalid G.. (Array) Pharmaceutics NB, 2022 undefined. Solid dispersion formulations by FDM 3D printing—A review. GM Khalid, N BillaPharmaceutics, 2022. mdpi.com. mdpi. com [Internet] Google Scholar
- Khalid G. M., Billa N.. (2022) Solid dispersion formulations by FDM 3D printing—A review. Pharmaceutics 14 Google Scholar
- Lekurwale S., Karanwad T.. (Array) Elsevier [Internet]. Medicine SBA of 3D P, 2022 undefined. Selective laser sintering (SLS) of 3D printlets using a 3D printer comprised of IR/red-diode laser. ElsevierS Lekurwale, T Karanwad, S BanerjeeAnnals of 3D printed medicine Google Scholar
- Li Q., Guan X., Cui M., Zhu Z., Chen K., Wen H., Jia D., Hou J., Xu W., Yang X., Pan W., et al. (2018) Preparation and investigation of novel gastro-floating tablets with 3D extrusion-based printing. International Journal of Pharmaceutics 535: 325-332 Google Scholar
- . (2022) A novel technology for preparing the placebos of vortioxetine hydrobromide tablets using LCD 3D printing. ElsevierS. European Journal of Pharmaceutics and Biopharmaceutics 178: 159-167 Google Scholar
- Li J Pang, Hong S, Chen X, Shao S, Wang H, Lao H, Xiong L, Wu H, Yang W, et al. F YangEuropean Journal of Pharmaceutics and Biopharmaceutics, 2022•Elsevier [Internet] Google Scholar
- . (Array) undefined. Stereolithographic 3D printing: Formulation design based on percolation thresholds. Elsevier [Internet] Google Scholar
- Linares V., Casas M., Huwyler J., Caraballo I.. (2023) Stereolithographic 3D printing: Formulation design based on percolation thresholds. Journal of Drug Delivery Science and Technology 90 Google Scholar
- Madžarević M.. (Array) Elsevier [Internet]. Sciences SIEJ of P, 2021 undefined. Evaluation of exposure time and visible light irradiation in LCD 3D printing of ibuprofen extended release tablets. ElsevierM Madžarević, S IbrićEuropean. Journal of Pharmaceutical Sciences Google Scholar
- Madžarević M., Ibrić S.. (2021) Evaluation of exposure time and visible light irradiation in LCD 3D printing of ibuprofen extended release tablets. European Journal of Pharmaceutical Sciences 158: 105688 Google Scholar
- Mathew E., Pitzanti G., Larrañeta E.. (2024) Pharmaceutics. 3D printing of pharmaceuticals and drug delivery devices, DL p. 2020 undefined. mdpi.com [Internet] Google Scholar
- . (2019) undefined. Feasibility study into the potential use of fused-deposition modeling to manufacture 3D-printed enteric capsules in compounding pharmacies. ElsevierC Nober, G Manini, E Carlier, JM Raquez, S Benali, P Dubois, K Amighi, J GooleInternational journal of pharmaceutics, 2019•Elsevier [Internet] Google Scholar
- Nober C., Manini G., Carlier E., Raquez J.-M., Benali S., Dubois P., Amighi K., Goole J., et al. (2019) Feasibility study into the potential use of fused-deposition modeling to manufacture 3D-printed enteric capsules in compounding pharmacies. International Journal of Pharmaceutics 569: 118581 Google Scholar
- Pandav G., Karanwad T.. (Array) Elsevier [Internet]. of SBJ of the MB, 2024 undefined. Sketching feasibility of additively manufactured different size gradient conventional hollow capsular shells (HCSs) by selective laser sintering (SLS): From design to. ElsevierG Pandav, T Karanwad, S BanerjeeJournal of the mechanical behavior of biomedical materials Google Scholar
- Pandav G., Karanwad T., Banerjee S.. (2024) Sketching feasibility of additively manufactured different size gradient conventional hollow capsular shells (HCSs) by selective laser sintering (SLS): From design to applications. Journal of the Mechanical Behavior of Biomedical Materials 151: 106393 Google Scholar
- Pandey M., Choudhury H., Fern J. L. C., Kee A. T. K., Kou J., Jing J. L. J., Her H. C., Yong H. S., Ming H. C., Bhattamisra S. K., Gorain B., et al. (2020) 3D printing for oral drug delivery: A new tool to customize drug delivery. Drug Delivery and Translational Research 10: 986-1001 Google Scholar
- Papadimitriou P., Andriotis E., Fatouros D., Micromachines D. T.. (Array) 2022 undefined. Design and prototype fabrication of a cost-effective microneedle drug delivery apparatus using fused filament fabrication, liquid crystal display and semisolid. EG Andriotis, D Fatouros, D TzetzisMicromachines. mdpi.comP Papadimitriou. mdpi.com [Internet] Google Scholar
- Papadimitriou P., Andriotis E. G., Fatouros D., Tzetzis D.. (2022) Design and prototype fabrication of a cost-effective microneedle drug delivery apparatus using fused filament fabrication, liquid crystal display and semi-solid extrusion 3D printing technologies. Micromachines 13 Google Scholar
- . (2019) undefined. Temporary plasticiser: A novel solution to fabricate 3D printed patient-centred cardiovascular ‘Polypill’architectures. ElsevierBC Pereira, A Isreb, RT Forbes, F Dores, R Habashy, JB Petit, MA Alhnan, EF OgaEuropean Journal of Pharmaceutics and Biopharmaceutics, 2019•Elsevier [Internet] Google Scholar
- Petrová E., Chvíla S., Štěpánek F., Zbytovská J., Lamprou D. A.. (2024) Imiquimod nanocrystal-loaded dissolving microneedles prepared by DLP printing. Drug Delivery and Translation Research Google Scholar
- . (Array) Elsevier [Internet]. undefined. Predictive models of FDM 3D printing using experimental design based on pharmaceutical requirements for tablet production. ElsevierFQ Pires, I Alves-Silva, LAG Pinho, JA Chaker. LL Sa-Barreto, GM Gelfuso, T GratieriInternational. Journal of Pharmaceutics p. 2020 Google Scholar
- Pires F. Q., Alves-Silva I., Pinho L. A. G., Chaker J. A., Sa-Barreto L. L., Gelfuso G. M., Gratieri T., Cunha-Filho M., et al. (2020) Predictive models of FDM 3D printing using experimental design based on pharmaceutical requirements for tablet production. International Journal of Pharmaceutics 588: 119728 Google Scholar
- Ramya A., Vanapalli S. L.. (2016) 3D printing technologies in various applications. International Journal of Mechanical Engineering and Technology 7 Google Scholar
- Samiei N.. (2020) Recent trends on applications of 3D printing technology on the design and manufacture of pharmaceutical oral formulation: A mini review. Beni-Suef University Journal of Basic and Applied Sciences 9 Google Scholar
- . (2020) undefined. Formulation design for inkjet-based 3D printed tablets. ElsevierK Sen, A Manchanda, T Mehta, AWK Ma, B ChaudhuriInternational journal of pharmaceutics, 2020•Elsevier [Internet] Google Scholar
- Sen K., Manchanda A., Mehta T., Ma A. W. K., Chaudhuri B.. (2020) Formulation design for inkjet-based 3D printed tablets. International Journal of Pharmaceutics 584: 119430 Google Scholar
- Sharma P. K., Choudhury D., Yadav V., Murty U. S. N., Banerjee S.. (2022) 3D printing of nanocomposite pills through desktop vat photopolymerization (stereolithography) for drug delivery reasons. 3D Printing in Medicine 8: 3 Google Scholar
- Tan D., Maniruzzaman M.. (Array) Pharmaceutics AN, 2018 undefined. Advanced pharmaceutical applications of hot-melt extrusion coupled with fused deposition modelling (FDM) 3D printing for personalised drug delivery. DKTan, M Maniruzzaman, A NokhodchiPharmaceutics. mdpi.com. mdpi.com [Internet] Google Scholar
- Tan D., Maniruzzaman M.. (Array) Polymers AN, 2019 undefined. Development and optimisation of novel polymeric compositions for sustained release theophylline caplets (PrintCap). ComDK Tan, M Maniruzzaman, A NokhodchiPolymers. MDPI. mdpi. com [Internet] Google Scholar
- Tan D. K., Maniruzzaman M., Nokhodchi A.. (2018) Advanced pharmaceutical applications of hot-melt extrusion coupled with fused deposition modelling (FDM) 3D printing for personalised drug delivery. Pharmaceutics 10 Google Scholar
- Tan D. K., Maniruzzaman M., Nokhodchi A.. (2019) Development and optimisation of novel polymeric compositions for sustained release theophylline caplets (PrintCap) via FDM 3D printing. Polymers 12 Google Scholar
- Valencia L., Herrera M., de la Mata M. (2024) Polymers A de L, 2022 undefined. Synthesis of silver nanocomposites for stereolithography: In situ formation of nanoparticles. mdpi.com [Internet] Google Scholar
- Wang J., Goyanes A., Gaisford S.. (Array) Elsevier [Internet]. of ABI journal, 2016 undefined. Stereolithographic (SLA) 3D printing of oral modified-release dosage forms. ElsevierJ Wang, A Goyanes, S Gaisford, AW BasitInternational journal of pharmaceutics Google Scholar
- Wang J., Goyanes A., Gaisford S., Basit A. W.. (2016) Stereolithographic (SLA) 3D printing of oral modified-release dosage forms. International Journal of Pharmaceutics 503: 207-212 Google Scholar
- Wu H., Chen P., Yan C., Cai C.. Design YSMand, 2019 undefined. Four-dimensional printing of a novel acrylate-based shape memory polymer using digital light processing. ElsevierH Wu, P Chen, C Yan, C Cai, Y ShiMaterials, and Design, 2019•Elsevier [Internet] Google Scholar
- Wu H., Chen P., Yan C., Cai C., Shi Y.. (2019) Four-dimensional printing of a novel acrylate-based shape memory polymer using digital light processing. Materials and Design 171 Google Scholar
- . (Array) Elsevier [Internet]. undefined. Fabrication of hollow microneedles using liquid crystal display (LCD) vat polymerization 3D printing technology for transdermal macromolecular delivery. ElsevierI Xenikakis, K Tsongas, EK Tzimtzimis, CK Zacharis, N Theodoroula, EP KalogianniInternational. Journal of Pharmaceutics p. 2021 Google Scholar
- Xenikakis I., Tsongas K., Tzimtzimis E. K., Zacharis C. K., Theodoroula N., Kalogianni E. P., Demiri E., Vizirianakis I. S., Tzetzis D., Fatouros D. G., et al. (2021) Fabrication of hollow microneedles using liquid crystal display (LCD) vat polymerization 3D printing technology for transdermal macromolecular delivery. International Journal of Pharmaceutics 597: 120303 Google Scholar
- Xu X., Awwad S., Diaz-Gomez L.. Pharmaceutics CAL, 2021 undefined. 3D printed punctal plugs for controlled ocular drug delivery. mdpi.comX Xu, S Awwad, L Diaz-Gomez, C Alvarez-Lorenzo, S Brocchini, S Gaisford, A GoyanesPharmaceutics, 2021•mdpi.com [Internet] Google Scholar
- Xu X., Awwad S., Diaz-Gomez L., Alvarez-Lorenzo C., Brocchini S., Gaisford S., Goyanes A., Basit A. W., et al. (2021) 3d printed punctal plugs for controlled ocular drug delivery. Pharmaceutics 13 Google Scholar
- . (Array) Elsevier [Internet]. 2020 undefined. Stereolithography (SLA) 3D printing of an antihypertensive polyprintlet: Case study of an unexpected photopolymer-drug reaction. ElsevierX Xu, P Robles-Martinez, CM Madla, F Joubert. A Goyanes, AW Basit, S GaisfordAdditive manufacturing Google Scholar
- Xu X., Robles-Martinez P., Madla C. M., Joubert F., Goyanes A., Basit A. W., Gaisford S., et al. (2020) Stereolithography (SLA) 3D printing of an antihypertensive polyprintlet: Case study of an unexpected photopolymer-drug reaction. Additive Manufacturing 33 Google Scholar
- . (Array) Elsevier [Internet]. undefined. Smartphone-enabled 3D printing of medicines. ElsevierX Xu, A Seijo-Rabina, A Awad, C Rial. S Gaisford, AW Basit, A GoyanesInternational. Journal of Pharmaceutics p. 2021 Google Scholar
- Xu X., Seijo-Rabina A., Awad A., Rial C., Gaisford S., Basit A. W., Goyanes A., et al. (2021) Smartphone-enabled 3D printing of medicines. International Journal of Pharmaceutics 609: 121199 Google Scholar
- Yang Y., Zhou Y., Lin X., Yang Q.. (Array) Pharmaceutics GY, 2020 undefined. Printability of external and internal structures based on digital light processing 3D printing technique. Lin, Q Yang, G YangPharmaceutics, X. mdpi.comY Yang, Y Zhou. mdpi.com [Internet] Google Scholar
- Yang Y., Zhou Y., Lin X., Yang Q., Yang G.. (2020) Printability of external and internal structures based on digital light processing 3D printing technique. Pharmaceutics 12 Google Scholar
- Yeung C., Chen S., King B., Lin H., King K., Akhtar F., Diaz G., Wang B., Zhu J., Sun W., Khademhosseini A., Emaminejad S., et al. (Array) A 3D-printed microfluidic-enabled hollowmicroneedlearchitecturefortransdermaldrugdelivery. pubs.aip.org[Internet]. Biomicrofluidics 13: 064125 Google Scholar
- Zhang J., Hu Q., Wang S., Tao J., Gou M.. (2020) Digital light processing based three-dimensional printing for medical applications. International Journal of Bioprinting 6: 242 Google Scholar