ABSTRACT
Solubility enhancers are one way to get around the difficulties with medication absorption and dissolution in oral drug delivery, since many novel active pharmaceutical components are not well soluble in water. The efficacy of a novel solubility-enhancing excipient (Soluplus) to enhance intestinal medication absorption. Improved drug release from poorly soluble pharmaceuticals can be achieved via polymer-based solid dispersions, such as Amorphous Solid Dispersions (ASD). Drug release and the rate of precipitation are directly influenced by the polymer concentration in dissolving fluid as well as the polymer and drug release occurring simultaneously. Investigating the polymer’s release in addition to the drug’s release is crucial, in addition to emphasizing the simultaneous measurement of both polymer and drug in the dissolution media, the goal of this critical systematic review was to provide an overview of the existing quantification techniques for commonly used water-soluble polymers, such as Soluplus®.
INTRODUCTION
Soluplus is a trademarked name for a graft copolymer composed of Polyvinyl Acetate (PVAc), Polyethylene Glycol (PEG) and Polycaprolactone (PCL). This copolymer is primarily used as a carrier polymer in pharmaceutical formulations due to its unique properties, including its solubility in water, compatibility with a wide range of drugs and ability to enhance drug solubility and bioavailability. A commercial graft copolymer that belongs to the amphiphilic polymer class is called Soluplus. Because of its special qualities, it is frequently employed in biological and pharmacological applications. Vinyl caprolactam, vinyl acetate and Polyethylene Glycol (PEG) combine to form Soluplus, a copolymer. These monomers are copolymerized to produce a polymer that is both lipophilic (attractive to fat) and hydrophilic (attractive to water). A growing number of recently developed Active Pharmaceutical Ingredients (APIs) have strong lipophilic characteristics, which limits their oral bioavailability. The breakdown of medications that are not very water soluble in gastrointestinal media is the limiting stage for the drug’s penetration and absorption through the intestinal system, according to the Biopharmaceutical Classification System (BCS). Consequently, the goal of many formulation attempts is to use surface-active excipients, which can function as both wetting agents and solubilizers, to increase drug solubility in physiological fluids. Utilizing cyclodextrin complexes or nanosizing are two further techniques for increasing oral medication solubility (BASF, 2010).
Structure
Soluplus is synthesized by grafting PEG and PCL chains onto the PVAc backbone through covalent bonding, resulting in a copolymer with both hydrophilic and hydrophobic segments (Figure 1). This unique structure imparts amphiphilic properties to Soluplus, allowing it to interact with both water and lipids, which is advantageous for drug delivery applications.2 See the polymer profile in Table 1.
Soluplus is an appealing carrier polymer for pharmaceutical formulations because of several of its attributes: (Figure 2). It is easier to formulate aqueous-based drug delivery systems, such as oral solutions, suspensions and emulsions, because Soluplus is soluble in water. This feature facilitates better patient compliance and ease of administration (BASF, 2010; Paaveret al, 2014).
Applications
Soluplus has been extensively investigated and utilized in various pharmaceutical applications (Figure 3).
Significance of SOL in improving the dissolution rate and bioavailability of poorly water-soluble drugs
Lipophilic APIs are more orally bioavailable when formulated with surface-active excipients such as cyclodextrin complexes or nanosizing. Solid solutions are made possible by hot melt extrusion with Soluplus, which enhances solubility in polymer matrices. This technique increases drug flow by dispersing medication molecularly, which upon disintegration forms supersaturated solutions. Soluplus considerably increases solubility in aqueous media, which may increase the oral bioavailability of poorly soluble medications. It is especially effective for BCS class II substances. Soluplus (SOL) plays a crucial role in improving the dissolution rate and bioavailability of poorly water-soluble drugs, addressing a significant challenge in pharmaceutical development. The incorporation of SOL into drug formulations enhances the solubility and dissolution kinetics of hydrophobic compounds, thereby improving their absorption and therapeutic efficacy (Kumariet al., 2023).
Several factors contribute to the significance of SOL (Figure 4):
SOL – Based Formulation Strategies
Solid Dispersions (SDs)
Solid dispersion enhances drug solubility and dissolution, crucial for improving bioavailability. However, its main drawback is the thermodynamic instability of amorphous pharmaceuticals, prone to recrystallization during storage, especially if residual crystalline drugs are present. Complete transformation of crystalline drugs into amorphous form is vital for stability and dissolution improvement. Modern solid dispersion focuses on glass solutions of poorly soluble compounds using amorphous carriers with high glass transition temperatures, ensuring enhanced physical stability and dissolution capacity.
Resveratrol is a very promising antioxidant drug candidate with low oral bioavailability due to its intrinsic poor water solubility, intestinal effiux and metabolization mechanisms. Resveratrol solubility high-throughput screening with different carriers was performed showing an enhancement above 2000-fold with Soluplus® and Tween® 80 (Figure 5). The former was selected as a carrier at the ratio of resveratrol: Soluplus® (1:2) (Kumariet al., 2023).
Mahbubur Rahman et al. (2020) examined the effects of Sodium Dodecyl Sulfate (SDS), Hydroxypropyl Cellulose (HPC) and Soluplus (Sol) on the release of Griseofulvin (GF) from Amorphous Solid Dispersions (ASDs). The ASDs included 2.5% GF, different HPC/Sol (2.5%-12.5%) and either 0.125% SDS or none. Due to their better miscibility, Sol-based ASDs displayed XRPD-amorphous GF, whereas HPC-based ASDs displayed crystalline GF. While HPC caused GF recrystallization, Sol alone showed only moderate supersaturation. Retaining Sol’s inhibitory effect on recrystallization, SDS enhanced the wettability of Sol-based ASD without causing recrystallization. In GF-Sol-SDS ASDs, low SDS concentrations improved wettability without causing recrystallization. Higher supersaturation was correlated with increasing Sol loading; for example, after 30 min with 0.83% SDS, a 1:5:0.05 GF:Sol:SDS ASD retained ~500% GF supersaturation (~430%) (Rahmanet al., 2020).
Liu et al. (2015) sought to improve a poorly water-soluble adjuvant’s physicochemical characteristics and oral bioavailability using solid dispersion formulations. They considerably increased the rate of aprepitant dissolving by using Soluplus®. The persistence of the amorphous form was confirmed by XRD, DSC and SEM and FTIR revealed intermolecular hydrogen bonding between the aprepitant and Soluplus®. AUC0-t increased by 2.4 times as compared to aprepitant alone in rat pharmacokinetic trials, indicating improved solubility and bioavailability and absorption comparable to Emend®. With a potentially improved medication solubility and absorption, this effective method highlights the effectiveness of Soluplus® in solid dispersion formulation and characterization (Liuet al., 2015).
Shuyu Jia et al. (2022) investigated the usage of Soluplus (SOL) to improve Erlotinib (ERL) Solid Dispersions (SDs) and Microparticles (MPs) for drug dissolution. SOL inhibited crystallization while promoting ERL solubility. DSC, PXRD, SEM and FT-IR indicated that ERL MPs, which were created via bottom-up and solvent evaporation techniques, displayed metastable crystal form A, but ERL SDs stayed amorphous. More stability in ERL MPs was found by accelerated testing, which provided insight into drug solubilization and post-recrystallization stability. The solubility of both formulations was greatly increased. Because SOL is hygroscopic, stability was affected differently in MPs and SDs, requiring specific tactics. Because of its ability to stop crystallization, SOL may be used as an MP stabilizer under the direction of its molecular interactions with the medication. The understanding of stabilizing amorphous and metastable drug states is improved by this work (Jiaet al., 2022).
Parameter | Description |
---|---|
Structural Formula | PEG 6000/vinylcaprolactam/vinyl acetate 13/57/30. |
Appearance | White to yellowish free flowing granules. |
Molecular weight | Nominally in the range of 90 000-140 000 g/mol. |
Critical Micelle Concentration | Micelles are typically 70-100 nm in diameter (pH 7 buffer). |
HLB | Approximately ~14. |
Solubility | It is soluble in water. Furthermore, it is soluble in acetone (up to 50%), methanol (up to 45%), ethanol (up to 25%), dimethylformamide (up to 50%) and in mixtures of (1:1 m/m) methanol/acetone (up to 50%) and (1:1 m/m) ethanol/acetone (up to 45%). |
Density | 1.082 g/cm³. |
Angle of Repose | 27.5º |
Particle Size | Approximately 340 microns in diameter. |
Nanosuspensions
Drugs in pharmaceutical nanosuspensions are insoluble drug particles dispersed in aqueous solutions at nanoscale, stabilized by surfactants. Nanoparticles, on the other hand, are lipid or polymeric colloidal drug carriers. The only choice available when a medication molecule has numerous drawbacks that prevent them from creating appropriate formulations, such as the inability to produce salt, huge molecular weight and dose, high log P and melting point, is the nanosuspension approach (Baumgartneret al., 2016).
Different manufacturing procedures generate homogeneous particle sizes, which are primarily responsible for the submicron particle stability attained in the nanosuspension. Throughout their shelf life, nanosuspensions’ particles must not fluctuate in size to prevent spontaneous crystal development. As a result, the Oswald ripening effect can prevent any crystal development by preventing the presence of variable saturation solubility by preserving the uniform particle size distribution (Patel and Agrawal, 2011).
Anjani, Qonita Kurnia et al. (2022) studied the use of Metronidazole (MTZ) Nanosuspensions (MTZ-NS) in dissolving Microarray Patches (MAPs) to treat Skin and Soft Tissue Infections (SSTIs) caused by Bacteroides fragilis (Figure 6). The 115 nm particle size and 0.27 PDI of MTZ-NS was made possible by Soluplus®. The optimized MTZ-NS loaded MAPs completely reduced B. fragilis and exhibited no toxicity, achieving 95% skin penetration through all layers. Dermatokinetic studies on swine skin exhibited 95% transport efficiency across all layers over a 24 hr period, whereas biocompatibility testing on 3T3L1 cells showed low toxicity. Total bacterial inhibition was verified by agar plating experiments. By treating deeply embedded SSTIs with this innovative delivery system, less intrusive treatment options may be available, possibly eliminating the need for intravenous or oral antibiotics. In vivo efficacy studies ought to be a part of future research to its ability to treat in animal models (Anjaniet al., 2022).
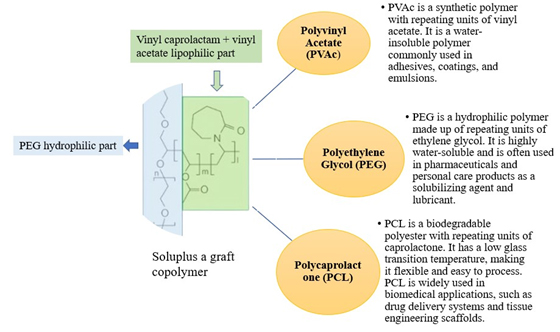
Figure 1:
Structure of Soluplus with components.
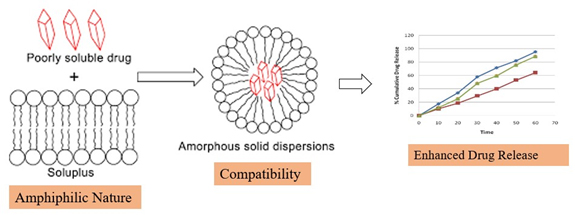
Figure 2:
Soluplus feature.
Itraconazole (ITZ) extrudates and nanosuspensions were compared in vitro and in vivo profiles by Keru Zhang et al. (2013). ITZ that was both amorphous and nanocrystalline was verified. In vitro release using amorphous ITZ/Soluplus demonstrated 100% release, whereas ITZ nanocrystals only achieved 40%. With a relative bioavailability of 98.3%, ITZ/Soluplus exhibited 6.9- and 11.6-fold greater AUC(0-t) and Cmax in vivo when compared to pure ITZ. Extrudates demonstrated a relative bioavailability of 101.3% and AUC(0-t) that were comparable to Sporanox®. There was stability for a minimum of three months. The study highlights increased amorphous ITZ/Soluplus solubility and bioavailability, with extrudates performing comparably to the commercial product and indicating potential as a stable substitute for improved ITZ oral delivery (Zhanget al., 2013).
Xing you Ye et al. (2016) presented a unique technique for creating Efavirenz (EFZ) Nanocrystal Solid Dispersions (NCSDs) by combining Hot-Melt Extrusion (HME) with High-Pressure Homogenization (HPH) (Figure 7). HPH first created a Nanosuspension (NS) using Kollidon® 30 and Sodium Lauryl Sulfate (SLS). The extruder barrel was then filled with Soluplus® and water was allowed to evaporate. By decreasing the size of EFZ particles, as demonstrated by zeta particle size analysis and scanning electron microscopy, this method increased the rate of dissolution. For six months, EFZ NCSD stability was guaranteed. This new strategy solves problems with traditional approaches and offers great opportunities to improve drug delivery systems (Yeet al., 2016).
Ramona Baumgartner et al., (2014) This paper introduces NANEX, a novel continuous process converting stabilized aqueous nano-suspensions into solid oral formulations in a single step, aiming to enhance drug solubility. Phenytoin nano-suspensions, stabilized with Tween® 80, were prepared via media milling. Using a hot melt extruder, the suspension was mixed with Soluplus® polymer, instantly removing water and dispersing nanocrystals in the molten polymer matrix. Transmission electron microscopy confirmed de-aggregated nanocrystals evenly dispersed in the extrudates, with no crystallinity changes observed. Dissolution studies demonstrated increased solubility of nano-crystalline phenytoin, regardless of the polymer used. This innovative approach offers a promising platform for designing advanced drug delivery systems to address challenges associated with drug stability, solubility and biological barrier crossing (Khinastet al., 2013).
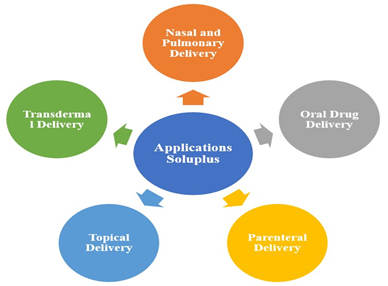
Figure 3:
Pharmaceutical applications of Soluplus.
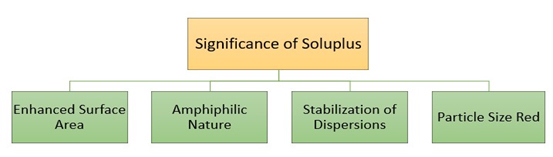
Figure 4:
Significance of Soluplus.
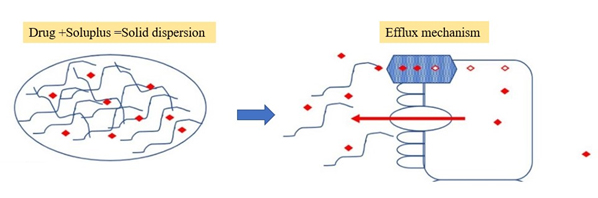
Figure 5:
solid dispersion combining Soluplus enhances the oral bioavailability of resveratrol.
Soluplus Mixed Nano micelles
The FDA’s 2007 clearance of the paclitaxel micelle formulation Genexol®PM for the treatment of breast, ovarian and lung cancer highlights the growing interest in nanomicelles for a variety of diseases. Nanomicelles, which are made of amphiphilic polymers, self-assemble in water to create hydrophobic core-hydrophilic shell nanostructures that are larger than 20-200 nm. By insulating the drug from plasma components, this structure improves solubility, controlled drug release and in vivo circulation. Small particle size promotes barrier traversal and cellular absorption, extending circulation and preventing filtration. Together, these characteristics increase the bioavailability of drugs, indicating the potential of nanomicelles for improvements in diagnostics and therapy (Hwanget al., 2020).
The study conducted by Ezequiel Bernabeu et al. (2016) aimed to increase the solubility of Paclitaxel (PTX) by creating mixed micelles using Soluplus® and TPGS. The results showed an increase of 38,000-fold in mixed micelles and 60,000-fold in pure Soluplus®. Stable release of PTX was shown by in vitro release investigations. In comparison to the PTX solution and single Soluplus® micelles, mixed micelles showed better anti-tumor activity against the cancer cell lines SKOV-3, MCF-7 and MDA-MB-231, along with increased cellular uptake. Via the induction of apoptosis by TPGS, blank mixed micelles produced cytotoxicity. Emphasizing their therapeutic potential, these results point to the potential of Soluplus®:TPGS mixed micelles as a promising nano-drug delivery method for cancer treatment (Bernabeuet al., 2016).
Pervez et al. (2023) used Microneedles (MNs) and nanomicelles to improve the solubility and bioavailability of Glimepiride (GM) by Transdermal Drug Delivery (TDD) (Figure 8). The zeta potential of the 10% Soluplus® and 40% GM nanomicelles was -16.2 mV, resulting in a 250-fold improvement in solubility. Dissolving Microneedles (DMNs) loaded with GM demonstrated concentration-independent release by non-Fickian transport, guaranteeing mechanical stability and drug release. When compared to oral tablets, pharmacokinetic tests in healthy volunteers revealed better values (Cmax: 1.56 μg/mL, Tmax: 4 hr, MRT: 40.04 hr). Microneedle shape, drug distribution and excipient compatibility were verified by SEM and FTIR. Studies conducted in vivo revealed sustained transdermal administration, which offers a novel approach to treating diabetes and promises improved glycemic control without side effects (Pervezet al., 2023).
Paclitaxel hybrid nanomicelles were developed by Ling-Hui Dian et al. (2018) as an oral chemotherapy target for resistant breast cancer. Soluplus, TPGS1000 and DQA (molar ratio 1/0.8/0.4) were combined to create nanomicelles, which have a diameter of around 65 nm, through solvent evaporation and hydration (Figure 9). In drug-resistant breast cancer cells, they demonstrated improved cellular uptake and strong anticancer activity, causing death via protein regulation and mitochondrial co-localization. In mice with resistant breast cancer, oral delivery equalled intravenous taxol effectiveness, underscoring the critical function of soluplus. These results indicate that paclitaxel hybrid nanomicelles represent a viable oral chemotherapy option for patients with drug-resistant breast cancer, providing enhanced therapeutic results and convenience (Khong, 2011).
Yoshiki Kojo et al. (2017) investigated the use of Soluplus®-based Self-Micellizing Solid Dispersion (SMSD) in hypochlorhydric environments to improve oral absorption and Itraconazole (ITZ) dissolution. SMSD showed an amorphous shape, better dissolving at neutral and acidic pH and insignificant degradation under rapid storage; however, exposure to light increased degradation by around 33%, emphasizing the necessity of light protection during storage. Pharmacokinetic analyses showed that SMSD/ITZ had a far higher oral bioavailability than crystalline ITZ, with absolute bioavailability values in the hypochlorhydric model being 6.3% and in normal rats being 2.9%. The potential of SMSD to enhance drug absorption is highlighted in this study, which is especially helpful for those with hypochlorhydria (Kojoet al., 2017).
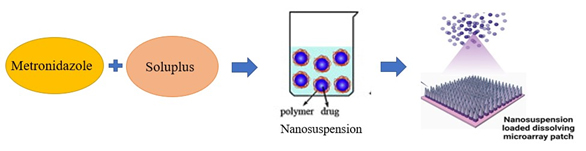
Figure 6:
Metronidazole nanosuspensions in dissolving Microarray Patches (MAPs).
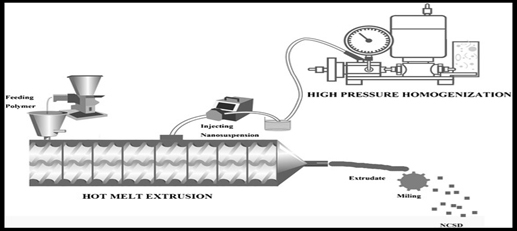
Figure 7:
Hot-Melt Extrusion (HME) with high-pressure homogenization.
Hongxue Shen et al., (2018) A novel Genistein-Loaded Mixed Micelles (GEN-M) system composed of Soluplus® and TPGS was developed to address the poor solubility and bioavailability of genistein. The optimized GEN-M exhibited spherical morphology with a mean particle size of 184.7±2.8 nm and a narrow PDI of 0.162±0.002. It displayed high entrapment efficiency (97.12±2.11%) and drug loading (3.87±1.26%). GEN-M demonstrated sustained release behavior and increased the solubility of GEN in water to 1.53±0.04 mg/mL. Permeability across Caco-2 cell monolayers was enhanced and pharmacokinetic studies showed a 2.42-fold increase in relative oral bioavailability compared to free GEN. This novel nanomicelles system holds promise for delivering hydrophobic drugs efficiently (Shenet al., 2018).
Jaleh Varshosaz et.al (2017) aimed to develop polymeric micelles loaded with Docetaxel (DCT) and Fe3O4 Magnetic Nanoparticles (MNPs) for targeted delivery. MNPs were prepared with oleic acid coating. The micelles were characterized for size, zeta potential, drug loading and release. Fe loading was determined by atomic absorption. Characterization included FT-IR, TGA, TEM and VSM. The optimized formulation had 70% drug loading, zeta potential of -2.58 mV and a size of 144.3 nm. Drug release efficiency at pH 5.5 was 68.9% in 24 hr. MNPs were coated and showed superparamagnetic properties. DCT-loaded micelles exhibited higher cytotoxicity against breast cancer cells compared to free drug, with no significant cytotoxicity on fibroblast cells (Varshosazet al., 2017).
Self-Nanoemulsions
The study conducted by Tamer H. Hassan et al., (2014) examined semisolid Self-Nanoemulsifying Drug Delivery Systems (SNEDDS) that comprised PEG-30-dipolyhydroxystearate, also known as Cithrol® DPHS, Capmul® MCM and Kolliphor® HS 15. Mixtures showed a hydrodynamic diameter of less than 25 nm, solidifying at ambient temperature and melting at body temperature. The stability of the dispersion was greatly affected by the DPHS:MCM ratio, with a 2:1 ratio being ideal for the size, dispersibility and stability of the nanoemulsion. The mono-modal volume distributions of formulas F2 and F3 have an average diameter of less than 25 nm. For in vivo performance, the presence of excipients was established by 1H NMR. To evaluate the influence of biofate and digestibility, especially with medications that are poorly soluble in water, more research is necessary (Hassanet al., 2014).
Han et al., (2020) developed a precipitation inhibitor-based Self-Nanoemulsifying Drug Delivery System (PI-SNEDDS) using Soluplus and Poloxamer 407 to enhance the dissolution and oral bioavailability of pH-dependent soluble Carvedilol (CAR) (Figure 10). The PI-SNEDDS significantly increased CAR content in the oil phase of nanoemulsions in the stomach and inhibited subsequent precipitation in the intestine compared to CAR SNEDDS and tablets. Relative bioavailability of CAR PI-SNEDDS (397.41%) was 1.56-fold higher than CAR SNEDDS (254.09%). Preparation involved mixing the oil phase, surfactant and cosurfactant, dissolving CAR in the blank emulsion to form CAR SNEDDS, then adding precipitation inhibitors (PVP K30, PVP K90, HPMC E5, Poloxamer 407 and Soluplus) to obtain CAR PI-SNEDDS. This approach enhances drug dissolution and offers potential for improving oral bioavailability of poorly soluble drugs with pH-dependent solubility (Hanet al., 2020).
Hakan Nazlı et al. (2021) was to use Self-Nanoemulsifying Drug Delivery Systems (SNEDDS) to increase the water solubility of Aprepitant (APR). A number of formulations, including solid formulations using Soluplus® as a Polymeric Precipitation Inhibitor (PPI) and supersaturated (super-SNEDDS) and conventional SNEDDS, were developed. Neusilin® US2 was used as a porous carrier for solid formulations and optimization produced a combination of 20% Imwitor® 988, 60% Kolliphor® RH40 and 20% Transcutol® P. Improved solubility and dissolving rate of APR were found during characterization and Soluplus® played a critical role in avoiding drug precipitation. These SNEDDS formulations demonstrate the efficacy of lipid-based drug administration and show promise as a substitute for micronized APR or commercialized capsules (Nazlıet al., 2021).
Three different nanocarriers loaded with lidocaine were characterized by Aikaterini Lalatsa et al. (2016). Customized Franz cells were utilized for diffusion tests via cellulose membranes. Among the nanocarriers were Polymeric Micelles (PMs) (Soluplus), solid lipid nanoparticles (SLNs) (Tripalmitin: Lecithin: Labrasol: polysorbate-20: water; 3.33:1:40:1:4.67 w/w) and Self-Nanoemulsifying Drug Delivery Systems (SNEDDS) (Capryol-90: Transcutol: Labrasol; 1:3:6 w/w). Zeta-potential, size and form of the particles were all described. At a significance threshold of p<0.05, the study used one-way Analysis of Variance (ANOVA) in conjunction with post hoc Tukey’s tests to assess permeation differences. With an eye toward future eyelid surgery, the purpose of this work was to explore the viability of delivering lidocaine via nanotechnology across an artificial skin equivalent. Solid Lipid Nanoparticles (SLNs), Soluplus®-based Polymeric Micelles (PMs) and other lidocaine-loaded nanocarriers (Lalatsaet al., 2016).
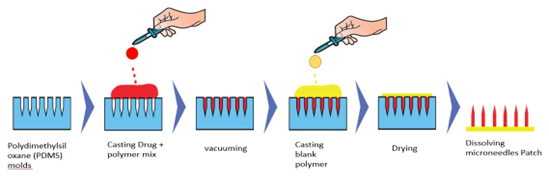
Figure 8:
Microneedles patch preparation.
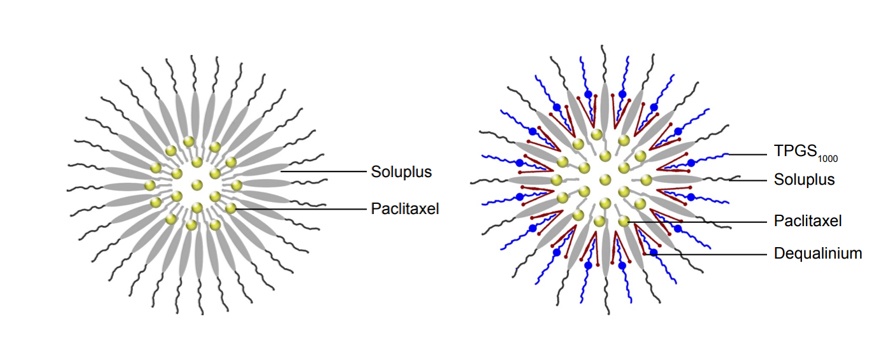
Figure 9:
Hybrid nanomicelles.
Drug Formulation Strategies with SOL
Hot-Melt Extrusion Technique
Hot-Melt Extrusion (HME) is a continuous manufacturing process used in the pharmaceutical industry for the preparation of solid dosage forms. In HME, (see Figure 11) a blend of drug, polymer (such as Soluplus), plasticizers and other excipients is fed into the extruder, where it is subjected to controlled heating and mixing. The heat softens the components, forming a molten mass that is forced through a die to obtain a desired shape, such as pellets, granules, or extrudates. As the material exits the die, it undergoes cooling and solidification, resulting in the formation of solid dosage forms with uniform drug distribution and controlled release properties. HME offers several advantages over conventional manufacturing methods, including improved product quality, reduced manufacturing costs, enhanced process efficiency and scalability (Patilet al., 2016; Repkaet al., 2008).
Advantages of Using Hot-Melt Extrusion in Soluplus-Based Formulations
There are benefits to using Soluplus in Hot-Melt Extrusion (HME) for pharmaceutical applications. It makes it possible to create Amorphous Solid Dispersions (ASDs) with medications that are not very soluble in water, which improves the drug’s solubility and rate of dissolution for increased bioavailability. HME ensures uniform dispersion within the polymer matrix by fostering close drug-polymer interactions. This technique makes it easier to incorporate Soluplus into different dosage forms, giving pharmacists exact control over the kinetics of drug release and loading in tablets, capsules and films. The continuous process of HME guarantees effective and consistent production at an industrial level, which is why pharmaceutical manufacture uses it. To summarize, HME offers a flexible and effective method for Soluplus-based formulations, resolving issues with poorly water-soluble medications and improving drug performance (Liet al., 2024).
In their 2015 study, Ritesh Fule et al. sought to improve the solubility and dissolution rates of Artesunate (ARS) by generating Solid Solutions (SSL) with Kollidon VA64 (VA64) or Soluplus (SOL) in addition to surfactants or plasticizers. The uniform distribution and amorphous drug states were validated by analytical methods. With F1 SSL (Soluplus-based) having 66.44 times higher AUC(0-72) and 16.60 times higher Cmax and K1 SSL (Kollidon VA64-based) having 62.20 times higher AUC(0-72) and 13.40 times higher Cmax than pure ARS, SSL formulations showed significantly quicker ARS release than pure ARS (Fuleet al., 2013).
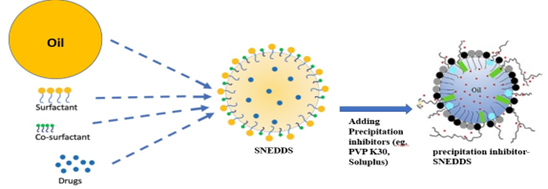
Figure 10:
Precipitation inhibitor-based Self-Nanoemulsifying Drug Delivery System (PI-SNEDDS).
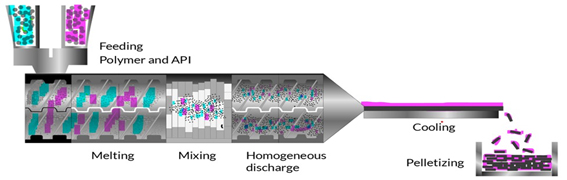
Figure 11:
Hot Melt Extrusion Process.
Saad M. Alshahrani et al. (2015) investigated the use of a unique combination of Soluplus® and Hypromellose Acetate Succinate (HPMCAS-HF) polymers in Hot-Melt Extrusion (HME) to improve the solubility and stability of Carbamazepine (CBZ) in amorphous solid dispersions. A range of Soluplus® and HPMCAS-HF ratios were used to produce the best CBZ dissolving rates, which showed full miscibility and 40% drug loading. By using FTIR spectroscopy, DSC, XRD and dissolution investigations, characterization verified the production of an amorphous solid dispersion. With Soluplus® as the main matrix polymer, especially at 7-21% (w/w) HPMCAS-HF, favorable results, such as increased drug loading and release, were noted. A year of stability under severe conditions was demonstrated by the polymer mix, indicating its promise for stable formulations with enhanced release profiles and solubility through HME (Alshahraniet al., 2015).
Jelena Djuris et al. (2013) investigated the viability of using Hot-Melt Extrusion (HME) to create solid dispersions of Carbamazepine (CBZ) in the copolymer (Soluplus®) grafted with polyethylene glycol, polyvinyl caprolactam and polyvinyl acetate. Differential Scanning Calorimetry (DSC), ATR-FTIR spectroscopy, Thermogravimetric Analysis (TGA) and Hot Stage Microscopy (HSM) were used to evaluate miscibility. Soluplus® and plasticizer-free HME of CBZ were able to produce microcrystalline dispersion at greater levels of molecular dispersion, up to 5% w/w CBZ. The temperature at which the extrusion was carried out had an impact on dispersion; higher temperatures promoted both molecule dispersion and the creation of CBZ form I. These results provide credence to the optimization of HME processes by thermodynamic model use (Djuriset al., 2013).
Nano extrusion
Nanoextrusion, a variation of Hot-Melt Extrusion (HME), offers superior advantages in Soluplus (SOL)-based formulations due to its ability to produce nanoscale drug delivery systems. Nanoextrusion enables precise control over particle size, morphology and drug distribution, resulting in enhanced drug solubility, dissolution rate and bioavailability. By reducing drug particle size to the nanometer range, nanoextrusion maximizes drug surface area, facilitating rapid dissolution and absorption. Moreover, nanoextrusion promotes homogeneous drug-polymer interactions, ensuring uniform dispersion and stability of SOL-based nanoparticles. This enables tailored drug release profiles and improved therapeutic outcomes, making nanoextrusion a superior choice for SOL-based formulations (Khinast,et al., 2013).
Grizeofulvin (GF) extrudates were made by means of nanoextrusion with HPC and Soluplus® polymers: micro/nanocomposites with crystalline GF in HPC and amorphous GF in Soluplus® (ASD). The wet medium milling process’s GF particle size had an impact on composites’ dissolution. Since GF in HPC degrades more quickly and is enhanced in wettability more than GF nanocrystals, amorphous GF in Soluplus® dissolves more slowly than GF nanocrystals. ASD used high medication dosages to produce higher supersaturation. Fast-dissolving HPC-based nanocomposites and Soluplus®-based ASDs may enhance the solubility of low-dose BCS Class II drugs (Liet al., 2021).
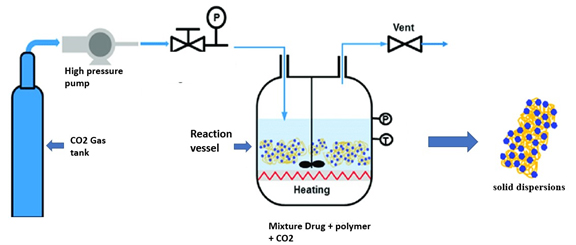
Figure 12:
Supercritical Fluid Processing.
Meng Li et al. (2017) looked at how the size of the polymeric matrix and the drug particle affected the drug’s inability to dissolve griseofulvin extrudates, a medication that is not very water soluble. Using Soluplus® stabilized wet-milled solutions and Hydroxypropyl Cellulose (HPC), nanoextrusion generated extrudates that were then dried and pulverized. In example, amorphous medicines with smaller extrudate particles showed delayed release from Soluplus®-based Amorphous Solid Dispersions (ASDs). Particle size effects were not as noticeable in nano/microcomposites using HPC. The investigation illustrated the impact of temperature-regulated regions with different polymer-drug ratios during the extrusion process. Increasing drug solubility using nanoextrusion is a promising approach that highlights the role of particle size and matrix composition on drug release kinetics (Liet al., 2017).
Stoja Milovanovic et al., (2022) This study specifically addresses the pharmaceutical sector and concentrates on producing novel materials using environmentally friendly methods. The potential application of supercritical CO2 (scCO2), both alone and in combination with the cardiovascular drug carvedilol, is being studied in the manufacturing of medical polymers such as Eudragit®, Soluplus® and hydroxypropyl methylcellulose acetate succinate. Solvent-free polymeric foams and Carvedilol solid dispersions with a controlled microstructure and pore width (101-257 μm) suitable for pharmaceutical applications were created in two hours at 30 MPa and 100ºC using a single-step static scCO2 technique. The scCO2 treatment had no effect on the polymer composition of the post-processing foams. Carvedilol dispersed molecularly and changed from a crystalline to an amorphous state in the solid dispersions because of hydrogen bonds it established with the polymers. Carvedilol dissolution from these solid dispersions was well-represented by the Korsmeyer-Peppas model, which demonstrated a markedly increased dissolution rate in acidic conditions. All things considered, the high-pressure technique that uses scCO2 appears to have potential for increasing drug dissolving rates and producing customized pharmaceutical materials (Milovanovicć and Lukić, 2022).
K. Eggenreich et al. sought to streamline the tablet production process in their 2016 work by employing Injection Molding (IM) straight from primary powder to create solid-dispersion-based tablets with fenofibrate as the model API. The graft co-polymer matrix approach and state-of-the-art IM equipment were employed to manufacture and characterize tablets both chemically and physically. The 10%, 20% and 30% (w/w) fenofibrate found in both powder- and pellet-based IM tablets showed consistent drug dispersion across formulations. Regardless of the processing method, a constant 60% drug release was seen after 120 min for both kinds of tablets. This work shows that it is possible to make tablets from compounded primary material that contain both fenofibrate and a solid dispersion base via IM, especially for formulations where the drug loading is less than 10%. These results are a first step in improving the efficiency of tablet production procedures (Eggenreichet al., 2016).
Supercritical Fluid Processing
Soluplus (SOL) improves the solubility and dispersion of weakly water-soluble pharmaceuticals, showing potential in supercritical fluid processing (Figure 12). Its ability to interact with supercritical fluids and medicinal molecules facilitates homogenous particle formation and drug solubilization. By adjusting parameters, Soluplus provides control over medication release kinetics, particle size and shape, enabling customized formulations. However, depending on the drug and processing circumstances, effectiveness may differ; therefore, optimization for the optimum drug loading and release profiles is required. Overall, Soluplus advances medication delivery applications by improving yield and efficiency in supercritical fluid-based processes such as Supercritical Antisolvent Precipitation (SAS) and Supercritical Fluid Extraction (SFE) (Misra and Pathak, 2020).
To accelerate the rate of dissolution of Simvastatin (SIM), Uttom Nandi et al. (2021) used Supercritical Carbon Dioxide (scCO2) to prepare SIM Solid Dispersions (SDs) with Soluplus® (SOL). Despite the crystallinity of SIM, amorphous SDs were revealed via physicochemical investigation (XRD, DSC, ATR-FTIR, SEM) when different SIM-SOL ratios (10%, 20% and 30%) were investigated. The results of the dissolution trials showed that SIM-SOL SDs displayed significantly higher rates of full release within 45 min when compared to physical mixtures. For medications that are sensitive to temperature, our solvent-free approach improved solubility without sacrificing stability, even at greater SIM loadings. An effective method for creating amorphous SDs is the scCO2-based approach, particularly when combined with supercritical fluid and Soluplus procedures (Nandiet al., 2021).
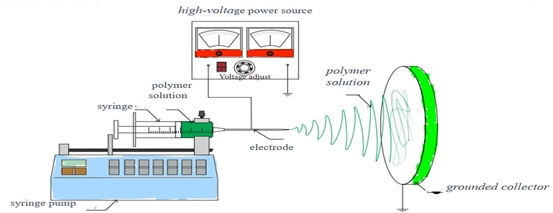
Figure 13:
illustrates the use of an electrospinning apparatus.
Rana M. Obaidat et al. (2017) looked at the use of Supercritical Fluid Technology (SFT) for solid dispersions to improve the solubility of tacrolimus, an immunosuppressant with low bioavailability. A variety of polymers were used, such as porous chitosan, PVP, Soluplus and HPMC. Chitosan, HPMC and PVP were added together with TPGS surfactant. The Loading Efficiency (LE) and dissolution of Soluplus dispersions were adjusted for processing parameters (time, temperature and pressure). In vitro release tests, DSC, XRD, FTIR and SEM were all used in the physicochemical analysis. After three months of stability testing, release profiles improved, with Soluplus achieving a cumulative release of 98.76% in just 24 hr. All dispersions, except for those based on chitosan, had amorphous drug precipitation. After a month, PVP formulations including TPGS displayed recrystallization, demonstrating the considerable impact of processing conditions on dispersion properties (Obaidatet al., 2017).
Catherine Potter et al., (2015) explores Supercritical Fluid Impregnation (SFI) and Hot Melt Extrusion (HME) for producing Amorphous Solid Dispersions (ASDs) of Indomethacin (INM). Both methods avoid toxic solvents and achieve lower temperatures than HME. INM dispersions at 50% w/w loading were amorphous via HME and 30% w/w via SFI. SFI showed γ-INM crystallinity, unlike HME. INM-PVP formulations exhibited higher dissolution rates, with PVP enhancing solubility 4-4.5 times over crystalline state compared to 2 times for Soluplus. HME produced more homogeneous dispersions, while SFI had challenges with high-viscosity polymers without surfactants. SFI formulations with Soluplus showed similar amorphous stability to HME. HME offers high throughput, but SFI is promising for thermally labile compounds or high Tg polymers. Different processing methods suit different drug formulations. Overall, considering multiple methods for poorly soluble drug formulations is essential for optimal outcomes (Potteret al., 2015).
Electrospinning
Electrospinning is a versatile technique used to produce nanofibrous materials from polymer solutions or melts. In this process, a high voltage is applied to a polymer solution or melts, causing the formation of a charged jet that is stretched and elongated as it travels towards a collector (Figure 13). The solvent evaporates during flight, leaving behind ultrafine fibers with diameters ranging from nanometers to micrometers. Electrospun nanofibers offer a high surface area-to-volume ratio and a porous structure, making them suitable for various applications such as drug delivery, tissue engineering, filtration and wound dressing. The morphology and properties of electrospun fibers can be tailored by adjusting parameters such as polymer concentration, solution viscosity, applied voltage and collector configuration. Despite its versatility, electrospinning has limitations such as low production rates, difficulty in scaling up and potential polymer degradation during processing (Xueet al., 2019).
Urve Paaver et al. (2014) used the synthetic graft copolymer Soluplus (PCL-PVAc-PEG) to study electrospun nanofibrous Drug Delivery Systems (DDSs) loaded with Piroxicam (PRX). Upon examination using Raman spectroscopy, X-ray powder diffraction, differential scanning calorimetry and scanning electron microscopy, circular nanofibers with diameters varying between 500 nm and 2 µm were identified. Inside the nanofibers, PRX stayed amorphous. These DDSs showed consistent, extended PRX release that was appropriate for wound healing treatment. To produce stable DDSs for poorly water-soluble drugs like PRX, the study proposes electrospinning using Soluplus. This technique may find use in advanced wound healing formulations (Paaveret al, 2014).
Using electrospinning, Olivera Kaljević et al. (2017) methodically produced Soluplus nanofibers laden with carvedilol. A miscibility investigation showed that carvedilol and Soluplus were compatible. Acetone: chloroform (90:10; w/w) as a solvent combination improved electrospinning. At lower drug concentrations, smooth nanofibers with fewer beads were observed using scanning electron microscopy. Through differential scanning calorimetry, it was determined that carvingilol had taken on an amorphous state within the nanofibers. Carvedilol and Soluplus interacted, as seen by the infrared spectra. In contrast to 20% from pure material, almost 85% of the drug was released in 15 min during dissolution studies. This technique demonstrates how studies of miscibility and solubility deal with weakly water-soluble drug dissolution (Kaljevićet al., 2017).
Ahmad Salawi et al. (2018) used solvent casting to examine the effect of vitamin E as a plasticizer on Soluplus® films. Various amounts of vitamin E (0-75% w/w) were used to cast the films. Reduced tensile strength and Young’s modulus, together with greater flexibility and higher elongation, were the results of the texture examination. Vitamin E improved Soluplus® compatibility by decreasing film crystallinity. Increased water contact angle and reduced swelling were indicative of improved hydrophobicity and adhesion with higher vitamin E concentration. While films with ≥65% may function as pressure-sensitive adhesive films, those with 30-50% vitamin E demonstrated great pliability. Using mixes of vitamin E and Soluplus®, this study suggests customized mechanical and adhesive qualities for flexible film compositions (Salawi and Nazzal, 2018).
The process of electrospinning nanofibrous DDSs. Figure 13 illustrates the electrospinning apparatus and experimental conditions utilized in the creation of the nanofibrous DDSs.
Suyeong Nam et al., (2017) An electrospinning technique was used to create a Poly (Vinyl Alcohol) (PVA) and Soluplus (SP)- based Nanofiber (NF) mat that would be used to administer Angelica Gigas Nakai (AGN) extract (ext) to oral malignancies. Electrospinning was used to construct AGN/SP NF (mean diameter: 75±26 nm; entrapment efficiency: 84.6±18.6%) and AGN/PVA/SP NF (mean diameter: 170±35 nm; entrapment efficiency: 81.0±10.1%). X-ray Diffractometry (XRD) analysis was used to confirm that AGN EtOH ext had become amorphous throughout the electrospinning procedure used to create NF structures. When compared to the AGN/PVA NF group, the AGN/PVA/SP NF group demonstrated faster disintegration (within 3 min) and quick wetting (within 2 sec), ensuring the successful and traditional administration of AGN/PVA/SP NF film in the oral cavity without the need for beverage consumption (Namet al., 2017).
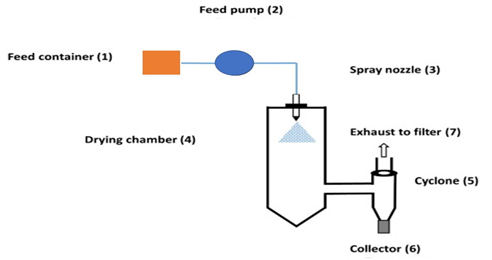
Figure 14:
Spray-drying.
Zsombor K Nagy et al. (2012) improved the solubility of spironolactone by using Soluplus® as a carrier and solubilizer. To make solid solutions, extrusion and Electrospinning (ES) techniques were used. Particularly in ES-generated fibers, Raman microscopy showed negligible crystalline spironolactone. Better dissolving and a larger surface area were produced by ES as opposed to extrusion. Effective amorphization and solid solution production were enhanced by ES, especially once the process was refined. These results demonstrate the potential of ES and extrusion to enhance the solubility of pharmaceuticals that are not very soluble in water, providing pharmaceutical formulations with promising solutions (Nagyet al., 2012).
In their 2018 study, Eniko Borbas et al. investigated size-exclusion membranes as a potential lipid membrane replacement for Amorphous Solid Dispersions (ASDs). Soluplus and Vinylpyrrolidone-vinyl acetate copolymer (PVPVA64) ASDs loaded with carvedilol were electrospun. The study assessed the load-dependent effects of additives on cellulose membrane regeneration and permeation using a μFLUX diffusion cell. The Supersaturation Ratio (SSR) drove membrane transit by representing the dissolution of medicine in relation to its solubility. Polymer additives demonstrated comparable membrane transport behavior despite different methods. This proposes size-exclusion membranes as workable substitutes for lipid membranes in formulations for ASD, helping to improve medication solubility and bioavailability (Borbáset al., 2018).
Polyvinylpyrrolidone (PVP) was used in the Formulations of Fenofibrate (FF) Electrospun (ES) nanofiber compositions by Peter Belton et al. (2021) to improve physical stability. High humidity did not stabilize FF-PVP ES dispersions, despite their propensity to increase drug solubility. PVP was used in conjunction with Eudragit E, Soluplus and Hypromellose Acetate Succinate (HPMCAS) to address moisture sorption problems. According to miscibility tests, phase separation is influenced by FF’s compatibility with Eudragit E and Soluplus over PVP and HPMCAS. While PVP-Eudragit E maintained its ability to increase disintegration, PVP-Soluplus reduced the release of the medication. Phase separation led to formulation deformation in polymer blends, but it also decreased water absorption. With a focus on phase separation and polymer miscibility’s roles in ES nanofiber formulation stability and dissolution enhancement, our findings inform the creation of balanced drug delivery systems (Tipduangtaet al., 2021).
The solubility and dissolution enhancement of lovastatin were studied by Sasa Kajdic et al. (2020) using poloxamer 188/poly (ethylene oxide) and Soluplus/poly(ethylene oxide) nanofibers. Lower starting concentrations and different nanofiber morphologies were the results of lovastatin’s breakdown during electrospinning. To increase lovastatin’s chemical stability within the nanofibers, antioxidants were added. With no crystalline lovastatin visible, Soluplus-based nanofibers had the best rate of lovastatin dissolving and solubility, suggesting enhanced drug release. In an expedited stability examination, poloxamer-based nanofibers including antioxidants showed improved lovastatin stability, while soluplus-based nanofibers showed only marginal lovastatin retention. For formulation stability, appropriate packaging and storage conditions are essential. Antioxidant-enriched Soluplus-based nanofibers present a viable method for improving the solubility and dissolution rates of chemically unstable and poorly soluble medications such as lovastatin (Kajdičet al., 2020).
Borbas E et al. (2016) used solvent-based electrospinning to assess the effects of formulation additives on meloxicam dissolution, supersaturation and membrane transport. Soluplus, 2-hydroxypropyl)-β-cyclodextrin and Polyvinylpyrrolidone (PVP) derivatives (K30, K90, VA 64) were used as solubilizing agents to generate five Amorphous Solid Dispersions (ASDs). While the dissolving characteristics of the formulations were similar, the formulations that included Soluplus showed better flow. The study created a mathematical model based on Fick’s first rule and showed how important it is to take supersaturation gradients into account when optimizing drug delivery methods for APIs that are poorly soluble in water (Borbáset al., 2016).
Spray-Drying
Spray-drying is a widely used technique for converting liquid formulations into dry powders by atomizing the feed solution into droplets and drying them rapidly in a heated chamber. The droplets are dispersed in a hot gas stream, where solvent evaporation occurs, resulting in the formation of solid particles (Figure 14). Spray-drying offers several advantages, including high efficiency, reproducibility and scalability. It is commonly employed in the pharmaceutical industry to produce inhalable powders, solid dispersions and amorphous formulations. The process parameters such as inlet temperature, feed rate and atomization pressure can be adjusted to control particle size, morphology and drug encapsulation efficiency. Despite its widespread use, spray-drying may lead to issues such as particle aggregation, loss of bioactivity and residual solvent content in the final product (Santoset al., 2017).
Lucas et al. (2022) explored the use of Spray Freeze Drying (SFD) to create amorphous celecoxib spheres that would improve solubility. Stable porous particles were produced by freeze-drying tertiary butyl alcohol solutions of celecoxib with excipients (povidone, HPMC-AS, Soluplus®). The amorphous form of celecoxib was verified by XRPD research. Kollidon 25 and HPMC-AS combinations demonstrated better drug concentrations and rates of dissolution; lower Tmax values suggested faster in vivo drug absorption. AUC values continued to be like formulations that were amorphous. SFD particles’ high porosity allowed for quick disintegration and absorption, providing a viable method to improve the oral bioavailability of weakly water-soluble drugs like celecoxib (Lucaset al., 2022).
Desai et al. (2023) investigated the use of Soluplus or Kollicoat IR in solid dispersion to increase the solubility and bioavailability of Arteether (ART), a BCS class II antimalarial with limited oral bioavailability. Three solvent-based methods were used: aqueous freeze-drying, hydro-alcoholic spray drying and ethanol rotary evaporation. The ART-polymer miscibility was enhanced by a 4-6% increase in polymer content. The maximum ART saturation solubility (476.01 mg/L with Soluplus) was obtained by spray drying. The formulations that were spray-dried demonstrated exceptional flow properties (Carr index: 21.27) and accomplished 88.88% drug release in a pH 6.8 buffer. As a carrier, Soluplus fared better than Kollicoat IR, producing a stable, somewhat amorphous ART solid dispersion with improved oral bioavailability (Desai and Chatterjee, 2023).
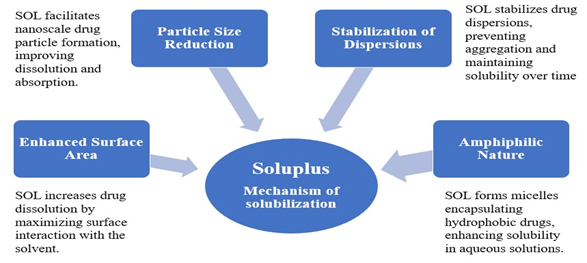
Figure 15:
Soluplus-Mechanism of solubilization.
Koch et al. (2020) used three methods to increase the solubility of Cannabidiol (CBD). While amorphous CBD was more soluble and dissolved more quickly than its crystalline counterpart, it was also more prone to recrystallization. Using water-soluble polymers (Kollidon® VA64, Kollidon® 12PF, Soluplus®), mesoporous silicas (Silsol®, Syloid® AL-1FP) and cyclodextrins (CH3αCD, HPβCD, HPγCD) by freeze-drying, spray-drying, subcritical CO2 impregnation, or hot-melt extrusion, full amorphous CBD was obtained. Formulations including cyclodextrin and CBD showed notable improvements in solubility, especially when freeze- or spray-dried. Among water-soluble polymers, Kollidon® VA64 demonstrated the largest solubility increase. For at least two months, the majority of formulations remained stable, providing important information on the potential medical uses of CBD (Kochet al., 2020).
Freeze-Drying
Freeze-drying, also known as lyophilization, is a low-temperature dehydration technique that involves freezing and then vacuum-removing the solvent. It maintains the structure and activity of meals, medications and biomolecules by stabilizing them. Superior stability, extended shelf life and preserved bioactivity are among the benefits. It consists of desorption (secondary drying), freezing and primary drying (sublimation). Ice crystals are directly sublimated during primary drying and any remaining moisture is removed during secondary drying. The end products include low density, good reconstitution characteristics and have a porous structure. Notwithstanding its advantages, freeze-drying is an expensive and time-consuming process that requires specialized equipment and optimization to ensure consistency and quality (Roy and Gupta, 2004).
Rehab Shamma et al. (2014) used solvent-casting to improve the solubility of Spirolactone (SL) for usage in pediatric applications utilizing freeze-dried oral thin films. Maximum desirability (0.836) was attained by the improved formulation (0.33:0.66 Soluplus:PVA ratio, 30% PEG 400, 10% polymer concentration). Improved porosity structure in the freeze-dried films facilitated the dissolving of SL. User satisfaction and residue-free dissolution were validated by in vivo studies. After five and fifteen min, this formulation demonstrated the best mechanical qualities and maximum drug release, making it appropriate for freeze-drying. The research highlights the potential of freeze-dried Soluplus/PVA-based oral thin films as a means of improving the oral dispersion of poorly soluble medications, particularly in the case of young patients (Shamma and Elkasabgy, 2016).
Pozzoli et al. (2017) used Soluplus® to create an Amorphous Solid Dispersion/solution (ASD) through freeze-drying to improve Budesonide (BUD) absorption and dissolution via nasal delivery. The porosity structure and specific surface area of the freeze-dried Soluplus-BUD formulation (LYO) demonstrated enhanced solubility and X-ray diffraction verified the drug’s amorphous nature. Comparing LYO to commercial goods that were based on water, such as dry powder and suspension, LYO showed faster release rates. With a greater transfer in permeability tests using RPMI 2650 nasal cells than commercial BUD solution, LYO-Ca, bulked with calcium carbonate, demonstrated appropriate aerodynamic qualities for nasal distribution. The nasal administration of poorly soluble medications appears to be improved by Soluplus (Pozzoliet al., 2017).
Soluplus(®) nanomicelles were created by Fernando Alvarez-Rivera et al. (2016) to improve the solubility, stability and ocular permeability of α-Lipoic Acid (ALA). Soluplus increased ALA solubility by over 10 times and formed micelles that were smaller (70-80 nm) than those produced by conventional surfactants. After being freeze-dried, membrane-sterilized and diluted in lachrymal fluid, these nanomicelles showed stability. They demonstrated the ability to gel in situ at doses of 1 or 2 mM, which may extend the corneal residence period. Soluplus nanomicelles markedly enhanced ALA build-up and flow toward the receptor chamber in cow ocular permeability experiments. Comparing Soluplus nanomicelles to traditional eye drops, the results point to them as potentially effective ALA carriers due to their superior stability, solubility and corneal permeability (Alvarez-Riveraet al., 2016).
Improvements in Physical Stability and Solubility
The lipophilic qualities of a growing number of newly developed Active Pharmaceutical Ingredients (APIs) cause them to have low oral bioavailability. The breakdown of weakly water-soluble medications in gastrointestinal medium is the limiting stage for their penetration and absorption through the intestinal system, according to the Biopharmaceutical Categorization System (BCS).
Hua Yang (2014) investigated the stabilization of Fenofibrate (FBT) nanosuspensions using Soluplus® and HPMC. The study found that nanosuspensions stabilized by Soluplus® (344 nm) and HPMC (642 nm) significantly reduced particle size compared to coarse FBT. Soluplus® provided superior stability by minimizing Ostwald ripening due to its micelle formation, which slowed the diffusion of dissolved FBT. This stabilization resulted in better dissolution and bioavailability. The in vivo study showed higher AUC, Cmax and shorter Tmax for the nanosuspensions (Yanget al., 2014).
Jian Guan et al. (2019) explored how hyaluronic acid and Soluplus® work together to preserve the supersaturation and enhance the physical stability of lovastatin. As a crystal growth inhibitor, HA was included into the structure of Soluplus® to provide steric and electrostatic stability, improving performance both in vitro and in vivo. A potential strategy for supersaturated drug delivery systems, the combination of Soluplus® and hyaluronic acid effectively preserved supersaturation while enhancing moisture uptake and physical stability (Guanet al., 2019).
Sumit Kumar Saha (2023) applied the Amorphous Solid Dispersion (ASD) technique to study the solubility and dissolution enhancement of Alectinib hydrochloride (ALB). Solubility was greatly improved by using Soluplus in a 1:5 ratio with ALB and it was further enhanced by adding 5% P407 in the third-generation ASD. The formulation maintained stability for a full year and demonstrated a two-fold increase in FaSSIF dissolution (Sahaet al., 2023).
Rosario Pignatello (2022) demonstrated that Soluplus® nanomicelles, with a size of 60-70 nm, effectively enhance the solubility of BCS class II drugs like ibuprofen, idebenone and miconazole. Solubility increased linearly with Soluplus® concentration. The nanomicelles remained stable after freeze-drying and reconstitution, showing potential for bioadhesive applications like ocular drug delivery (Pignatelloet al., 2022).
Applicant | Application Number and Status | Outcome |
---|---|---|
Zhejiang University of Technology ZJUT. | CN201610243442. 8A Granted (Active). | Temperature-sensitive gel matrix using Soluplus, providing extended drug slow release for 7 days, superior to Poloxamer 407. |
Allergan Inc. | EP12808637. 8A Granted (Active). | Ophthalmic compositions using Soluplus for enhancing solubility and bioavailability of therapeutic agents. |
Sanovel Ilac Sanayi Ve Ticaret Anonim Sirketi. | WO2013191668A1 Published. | Compositions using Soluplus for hypertension, enhancing solubility in solid formulations. |
Allergan Inc. | EP2790673B1 Granted (Active). | Ophthalmic compositions with Soluplus graft copolymers, enhancing drug delivery for eye applications. |
European Patent Office. | 20902732.5 Published. | Solid oral composition of Olaparib with enhanced solubility and bioavailability. |
Memoona Ishtiaq et al. (2024) created Ternary Solid Dispersions (TSDs) of curcumin with Soluplus® (20%), which considerably improved its solubility and dissolution. The modified formulation, F3, improved curcumin solubility to 186 μg/mL (3100-fold) and dissolution to 91% (9-fold). FTIR, SEM, XRD and DSC corroborated the increased solubility. When compared to pure curcumin, F3 demonstrated improved biological activities such as antibacterial, antioxidant (93%) and anti-inflammatory properties (Ishtiaqet al., 2024).
Iskra Z. Koleva et al. (2024) significantly improved eugenol’s water solubility by encapsulating it with Soluplus® and Lutrol F 127 using spray drying. The optimized formulations achieved high encapsulation efficiencies of 97.9-98.2% for 5% eugenol and around 90% for 15% eugenol. The addition of Lutrol F 127 improved particle shape and flowability, enhancing the solubility and usability in various dosage forms (Koleva and Tzachev, 2024).
Soluplus-Mechanism of solubilization
Soluplus® improves drugs solubility through various vital mechanisms (Figure 15). It decreases drugs particle size to the nanoscale, resulting in better solubility and absorption. Soluplus stabilizes dispersions by preventing particle aggregation, resulting in sustained solubility. Its amphiphilic nature enables it to create micelles that encapsulate hydrophobic drugs, improving their solubility in aqueous solutions. Furthermore, Soluplus improves the drug’s surface area by optimizing interaction with the solvent, resulting in faster dissolving. These mechanisms work together to greatly increase the solubility and bioavailability of medicines that are poorly water soluble (Figure 15).
Patent
Table 2 summarizes key patents involving Soluplus® formulations, highlighting innovative applications.
CONCLUSION
In conclusion, the use of solubility enhancers like Soluplus is a viable solution to the problems posed by the insufficient solubility of Active Pharmaceutical Ingredients (APIs) in oral drug delivery. By using methods such as solid dispersions and especially Amorphous Solid Dispersions (ASD), Soluplus improves drug absorption and release in the intestines, increasing bioavailability. The thorough analysis highlights the significance of measuring drug release and polymer simultaneously in dissolving media, highlighting the need for reliable quantification methods tailored to water-soluble polymers like Soluplus®. Several manufacturing approaches have been investigated to successfully incorporate Soluplus into therapeutic formulations, including as hot-melt extrusion, nanoextrusion, supercritical fluid processing, electrospinning, spray-drying and freeze-drying. Improved physical stability, regulated release and increased drug solubility are some benefits of these techniques. Also, Soluplus amphiphilic nature, increased surface area, particle size reduction and stabilization of dispersions all contribute to its capacity to improve the physical stability, dissolving rate and bioavailability of poorly water-soluble medications. The performance of poorly soluble medications can be greatly improved by Soluplus, which also advances pharmaceutical formulations for better therapeutic outcomes. All things considered, Soluplus proves to be a flexible and effective solubility enhancer. Leveraging the full potential of Soluplus in medication delivery applications requires ongoing study and development of formulation and processing procedures.
Cite this article:
Shinde DT, Patil KR, Mali KD. Review on Soluplus®: Pharmaceuticals Revolutionizing Drug Delivery and Formulation Strategies. Int. J. Pharm. Investigation. 2025;15(2):10-8.
ACKNOWLEDGEMENT
We thank R. C. Patel Institute of Pharmaceutical Education and Research (RCPIPER) and R. C. Patel Institute of Pharmacy (RCPIOP), Shirpur, for their support and resources in facilitating this work.
ABBREVIATIONS
SOL | Soluplus |
---|---|
SD | Solid Dispersion |
HME | Hot Melt Extrusion |
ASD | Amorphous Solid Dispersion |
BCS | Biopharmaceutics Classification System |
FDT | Fast Disintegrating Tablet |
ART | Arteether |
SFD | Spray freeze drying |
NCSDs | Nanocrystal solid dispersions |
HME | Hot-melt extrusion |
HPH | High-pressure homogenization |
MTZ-NS | Metronidazole nanosuspensions |
SMSD | Self-micellizing solid dispersion |
TGA | Thermogravimetric analysis |
CBD | Cannabidiol |
HSM | Hot stage microscopy |
SFD | Spray Freeze Drying |
BUD | Budesonide |
References
- Alshahrani S. M., Lu W., Park J.-B., Morott J. T., Alsulays B. B., Majumdar S., Langley N., Kolter K., Gryczke A., Repka M. A., et al. (2015) Stability-enhanced hot-melt extruded amorphous solid dispersions via combinations of Soluplus and HPMCAS-HF. AAPS PharmSciTech 16: 824-834 Google Scholar
- Alvarez-Rivera F., Fernández-Villanueva D., Concheiro A., Alvarez-Lorenzo C.. (2016) α-lipoic acid in Soluplus Polymeric nanomicelles for Ocular Treatment of Diabetes-Associated Corneal Diseases. Journal of Pharmaceutical Sciences [Internet] 105: 2855-2863 Google Scholar
- Anjani Q. K., Sabri A. H. B., Domínguez-Robles J., Moreno-Castellanos N., Utomo E., Wardoyo L. A. H., Larrañeta E., Donnelly R. F., et al. (2022) Metronidazole nanosuspension loaded dissolving microarray patches: An engineered composite pharmaceutical system for the treatment of skin and soft tissue infection. Biomaterials Advances 140: Article 213073 Google Scholar
- . (2010) Technical information soluplus. BASF, pharma ingredients serv : 1-8 Google Scholar
- Baumgartner R., Teubl B. J., Tetyczka C., Roblegg E.. (2016) Rational design and characterization of a nanosuspension for intraoral administration considering physiological conditions. Journal of Pharmaceutical Sciences 105: 257-267 Google Scholar
- Bernabeu E., Gonzalez L., Cagel M., Gergic E. P., Moretton M. A., Chiappetta D. A., et al. (2016) Novel Soluplus—TPGS mixed micelles for encapsulation of paclitaxel with enhanced in vitro cytotoxicity on breast and ovarian cancer cell lines. Colloids and Surfaces. B, Biointerfaces [Internet] 140: 403-411 Google Scholar
- Borbás E., Sinkó B., Tsinman O., Tsinman K., Kiserdei É., Démuth B., Balogh A., Bodák B., Domokos A., Dargó G., Balogh G. T., Nagy Z. K., et al. (2016) Investigation and mathematical description of the real driving force of passive transport of drug molecules from supersaturated solutions. Molecular Pharmaceutics 13: 3816-3826 Google Scholar
- Borbás E., Tőzsér P., Tsinman K., Tsinman O., Takács-Novák K., Völgyi G., Sinkó B., Nagy Z. K., et al. (2018) Effect of formulation additives on drug transport through size-exclusion membranes. Molecular Pharmaceutics 15: 3308-3317 Google Scholar
- Desai P., Chatterjee B.. (2023) Comparison of two grafted copolymers, soluplus and Kollicoat IR, as solid dispersion carriers of arteether for oral delivery prepared by different solvent-based methods. ACS Omega [Internet] 8: 45337-45347 Google Scholar
- Djuris J., Nikolakakis I., Ibric S., Djuric Z., Kachrimanis K.. (2013) Preparation of carbamazepine-Soluplus solid dispersions by hot-melt extrusion and prediction of drug-polymer miscibility by thermodynamic model fitting. European Journal of Pharmaceutics and Biopharmaceutics [Internet] 84: 228-237 Google Scholar
- (2018) by Dove Press published : 719-731 Google Scholar
- Eggenreich K., Windhab S., Schrank S., Treffer D., Juster H., Steinbichler G., Laske S., Koscher G., Roblegg E., Khinast J. G., et al. (2016) Injection molding as a one-step process for the direct production of pharmaceutical dosage forms from primary powders. International Journal of Pharmaceutics 505: 341-351 Google Scholar
- Fule R. A., Meer T. S., Sav A. R., Amin P. D.. (2013) Artemether-soluplus hot-melt extrudate solid dispersion systems for solubility and dissolution rate enhancement with amorphous state characteristics. Journal of Pharmaceutics 2013: Article 151432 Google Scholar
- Guan J., Liu Q., Jin L., Xu H., Wu H., Zhang X., Mao S., et al. (2019) Synergistic effect of Soluplus and hyaluronic acid on the supersaturation maintenance of lovastatin: The facilitated in vitro-in vivo performance and improved physical stability. Carbohydrate Polymers [Internet] 222: Article 114978 Google Scholar
- Han H., Li Y., Peng Z., Long K., Zheng C., Wang W., Webster T. J., Ge L., et al. (2020) A Soluplus/Poloxamer 407-based self-nanoemulsifying drug delivery system for the weakly basic drug carvedilol to improve its bioavailability. Nanomedicine: Nanotechnology, Biology, and Medicine 27: Article 102199 Google Scholar
- Hassan T. H., Metz H., Mäder K.. (2014) Novel semisolid SNEDDS based on PEG-30-dipolyhydroxystearate: Development and characterization. International Journal of Pharmaceutics [Internet] 477: 506-518 Google Scholar
- Hwang D., Ramsey J. D., Kabanov A. V.. (2020) Polymeric micelles for the delivery of poorly soluble drugs: From nanoformulation to clinical approval. Advanced Drug Delivery Reviews [Internet] 156: 80-118 Google Scholar
- Ishtiaq M., Manzoor H., Khan I. U., Asghar S., Irfan M., Albekairi N. A., Alshammari A., Alqahtani A. F., Alotaibi S., Munir R., Shah P. A., Hussain L., Saleem M. A., Razzaq F. A., Khalid S. H., et al. (2024) Curcumin-loaded soluplus and #xae; based ternary solid dispersions with enhanced solubility, dissolution and antibacterial, antioxidant, anti-inflammatory activities. Heliyon [Internet] 10: Article e34636 Google Scholar
- Jia S., Ning S., Leng Y., Jing Q., Xu Z., Ren F., et al. (2022) Stabilizing effect of soluplus on erlotinib metastable crystal form in microparticles and amorphous solid dispersions. Polymers 14: Article 1241 Google Scholar
- Kajdič S., Zupančič Š., Roškar R., Kocbek P.. (2020) The potential of nanofibers to increase solubility and dissolution rate of the poorly soluble and chemically unstable drug lovastatin. International Journal of Pharmaceutics [Internet] 573: Article 118809 Google Scholar
- Kaljević O., Djuris J., Čalija B., Lavrič Z., Kristl J., Ibrić S., et al. (2017) Application of miscibility analysis and determination of Soluplus solubility map for development of carvedilol-loaded nanofibers. International Journal of Pharmaceutics 533: 445-454 Google Scholar
- Khinast J., Baumgartner R., Roblegg E.. (2013) Nano-extrusion: A one-step process for manufacturing of solid nanoparticle formulations directly from the liquid phase. AAPS PharmSciTech 14: 601-604 Google Scholar
- Koch N., Jennotte O., Gasparrini Y., Vandenbroucke F., Lechanteur A., Evrard B., et al. (2020) Cannabidiol aqueous solubility enhancement: Comparison of three amorphous formulations strategies using different type of polymers. International Journal of Pharmaceutics 589: Article 119812 Google Scholar
- Kojo Y., Kobayashi K., Matsunaga S., Suzuki H., Seto Y., Sato H., Onoue S., et al. (2017) Avoidance of food effect on oral absorption profile of itraconazole by self-micellizing solid dispersion approach. Drug Metabolism and Pharmacokinetics [Internet] 32: 273-276 Google Scholar
- Koleva I. Z., Tzachev C. T.. (2024) Efficient improvement of eugenol water solubility by spray drying encapsulation in Soluplus and Lutrol F 127. Pharmaceuticals 17: Article 1156 Google Scholar
- Kumari L., Choudhari Y., Patel P., Gupta G. D., Singh D., Rosenholm J. M., Bansal K. K., Kurmi B. D., et al. (2023) Advancement in solubilization approaches: A step towards bioavailability enhancement of poorly soluble drugs. Life 13: Article 1099 Google Scholar
- Lalatsa A., Emeriewen K., Protopsalti V., Skelton G., Saleh G. M.. (2016) Developing transcutaneous nanoenabled anaesthetics for eyelid surgery. The British Journal of Ophthalmology 100: 871-876 Google Scholar
- Li M., Furey C., Skros J., Xu O., Rahman M., Azad M., Dave R., Bilgili E., et al. (2021) Impact of matrix surface area on griseofulvin release from extrudates prepared via nanoextrusion. Pharmaceutics 13: Article 1036 Google Scholar
- Li M., Ioannidis N., Gogos C., Bilgili E.. (2017) A comparative assessment of nanocomposites vs. amorphous solid dispersions prepared via nanoextrusion for drug dissolution enhancement. European Journal of Pharmaceutics and Biopharmaceutics [Internet] 119: 68-80 Google Scholar
- Li S., Zhang Z., Gu W., Gallas M., Jones D., Boulet P., Johnson L. M., de Margerie V., Andrews G. P., et al. (2024) Hot melt extruded high-dose amorphous solid dispersions containing lumefantrine and soluplus. International Journal of Pharmaceutics [Internet] 665: Article 124676 Google Scholar
- Liu J., Zou M., Piao H., Liu Y., Tang B., Gao Y., Ma N., Cheng G., et al. (2015) Characterization and pharmacokinetic study of aprepitant solid dispersions with soluplus. Molecules 20: 11345-11356 Google Scholar
- Lucas D., Kožák J., Rautenberg A., Chrétien C., Pellequer Y., Lamprecht A., et al. (2022) Designing highly porous amorphous celecoxib particles by spray freeze drying leads to accelerated drug absorption in-vivo. European Journal of Pharmaceutics and Biopharmaceutics 174: 20-28 Google Scholar
- . (2017) Chapter 24Santos D. IntechOpen Google Scholar
- Milovanović S., Lukić I.. (2022) An overview on the application of supercritical carbon dioxide for the processing of pharmaceuticals. Arhiv za Farmaciju (Belgr) 72: 566-590 Google Scholar
- Misra S. K., Pathak K.. (2020) Supercritical fluid technology for solubilization of poorly water soluble drugs via micro- and naonosized particle generation. ADMET and DMPK 8: 355-374 Google Scholar
- Nagy Z. K., Balogh A., Vajna B., Farkas A., Patyi G., Kramarics A., Marosi G., et al. (2012) Comparison of electrospun and extruded Soluplus-based solid dosage forms of improved dissolution. Journal of Pharmaceutical Sciences 101: 322-332 Google Scholar
- Nam S., Lee J.-J., Lee S. Y., Jeong J. Y., Kang W.-S., Cho H.-J., et al. (2017) Angelica gigas Nakai extract-loaded fast-dissolving nanofiber based on poly(vinyl alcohol) and Soluplus for oral cancer therapy. International Journal of Pharmaceutics 526: 225-234 Google Scholar
- Nandi U., Ajiboye A. L., Patel P., Douroumis D., Trivedi V.. (2021) Preparation of solid dispersions of simvastatin and soluplus using a single-step organic solvent-free supercritical fluid process for the drug solubility and dissolution rate enhancement. Pharmaceuticals 14: Article 846 Google Scholar
- Nazlı H., Mesut B., Özsoy Y.. (2021) In vitro evaluation of a solid supersaturated self nanoemulsifying drug delivery system (Super-snedds) of aprepitant for enhanced solubility. Pharmaceuticals 14: Article 1089 Google Scholar
- Obaidat R. M., Tashtoush B. M., Awad A. A., Al Bustami R. T.. (2017) Using supercritical fluid technology (SFT) in Preparation of Tacrolimus Solid Dispersions. AAPS PharmSciTech 18: 481-493 Google Scholar
- Paaver U., Tamm I., Laidmäe I., Lust A., Kirsimäe K., Veski P., Kogermann K., Heinämäki J., et al. (2014) Soluplus graft copolymer: Potential novel carrier polymer in electrospinning of nanofibrous drug delivery systems for wound therapy. BioMed Research International 2014: Article 789765 Google Scholar
- Patel V. R., Agrawal Y. K.. (2011) Nanosuspension: An approach to enhance solubility of drugs. Journal of Advanced Pharmaceutical Technology and Research 2: 81-87 Google Scholar
- Patil H., Tiwari R. V., Repka M. A.. (2016) Hot-melt extrusion: From theory to application in pharmaceutical formulation. AAPS PharmSciTech 17: 20-42 Google Scholar
- Pervez S., Nasir F., Hidayatullah T., Khattak M. A., Alasmari F., Zainab S. R., Gohar S., Tahir A., Maryam G. E., et al. (2023) Transdermal delivery of glimepiride: A novel approach using nanomicelle-embedded microneedles. Pharmaceutics 15: Article 2019 Google Scholar
- Pignatello R., Corsaro R., Bonaccorso A., Zingale E., Carbone C., Musumeci T., et al. (2022) Soluplus() polymeric nanomicelles improve solubility of BCS-class II drugs. Drug Delivery and Translational Research 12: 1991-2006 Google Scholar
- Potter C., Tian Y., Walker G., McCoy C., Hornsby P., Donnelly C., Jones D. S., Andrews G. P., et al. (2015) Novel supercritical carbon dioxide impregnation technique for the production of amorphous solid drug dispersions: A comparison to hot melt extrusion. Molecular Pharmaceutics 12: 1377-1390 Google Scholar
- Pozzoli M., Traini D., Young P. M., Sukkar M. B., Sonvico F.. (2017) Development of a Soluplus budesonide freeze-dried powder for nasal drug delivery. Drug Development and Industrial Pharmacy 43: 1510-1518 Google Scholar
- Rahman M., Ahmad S., Tarabokija J., Parker N., Bilgili E.. (2020) Spray-dried amorphous solid dispersions of griseofulvin in HPC/soluplus/SDS: Elucidating the multifaceted impact of SDS as a minor component. Pharmaceutics 12: Article 197 Google Scholar
- Repka M. A., Majumdar S., Kumar Battu S., Srirangam R., Upadhye S. B.. (2008) Applications of hot-melt extrusion for drug delivery. Expert Opinion on Drug Delivery 5: 1357-1376 Google Scholar
- Roy I., Gupta M. N.. (2004) Freeze-drying of proteins: Some emerging concerns. Biotechnology and Applied Biochemistry 39: 165-177 Google Scholar
- Saha S. K., Joshi A., Singh R., Jana S., Dubey K.. (2023) An investigation into solubility and dissolution improvement of alectinib hydrochloride as a third-generation amorphous solid dispersion. Journal of Drug Delivery Science and Technology [Internet] 81: Article 104259 Google Scholar
- Salawi A., Nazzal S.. (2018) The physiochemical, mechanical and adhesive properties of solvent-cast vitamin E/Soluplus films. International Journal of Pharmaceutics [Internet] 552: 378-387 Google Scholar
- Shamma R., Elkasabgy N.. (2016) Design of freeze-dried Soluplus/polyvinyl alcohol-based film for the oral delivery of an insoluble drug for the pediatric use. Drug Delivery 23: 489-499 Google Scholar
- Shen H., He D., Wang S., Ding P., Wang J., Ju J., et al. (2018) Preparation, characterization and pharmacokinetics study of a novel genistein-loaded mixed micelles system. Drug Development and Industrial Pharmacy 44: 1536-1542 Google Scholar
- Tipduangta P., Belton P., McAuley W. J., Qi S.. (2021) The use of polymer blends to improve stability and performance of electrospun solid dispersions: The role of miscibility and phase separation. International Journal of Pharmaceutics 602: Article 120637 Google Scholar
- Varshosaz J., Dehkordi A. J., Setayesh S.. (2017) Magnetic polyvinyl caprolactam-polyvinyl acetate-polyethylene glycol micelles for docetaxel delivery in breast cancer: An in vitro study on two cell lines of breast cancer. Pharmaceutical Development and Technology [Internet] 22: 659-668 Google Scholar
- Vasconcelos T., Prezotti F., Araújo F., Lopes C., Loureiro A., Marques S., Sarmento B., et al. (2021) Third-generation solid dispersion combining Soluplus and poloxamer 407 enhances the oral bioavailability of resveratrol. International Journal of Pharmaceutics [Internet] 595: Article 120245 Google Scholar
- Xue J., Wu T., Dai Y., Xia Y., States U., States U., et al. (2019) Electrospinning and electrospun nanofibers: Methods, materials and applications. Chemical Reviews 119: 5298-5415 Google Scholar
- Yang H., Teng F., Wang P., Tian B., Lin X., Hu X., Zhang L., Zhang K., Zhang Y., Tang X., et al. (2014) Investigation of a nanosuspension stabilized by Soluplus to improve bioavailability. International Journal of Pharmaceutics [Internet] 477: 88-95 Google Scholar
- Ye X., Patil H., Feng X., Tiwari R. V., Lu J., Gryczke A., Kolter K., Langley N., Majumdar S., Neupane D., Mishra S. R., Repka M. A., et al. (2016) Conjugation of hot-melt extrusion with high-pressure homogenization: A novel method of continuously preparing nanocrystal solid dispersions. AAPS PharmSciTech 17: 78-88 Google Scholar
- Zhang K., Yu H., Luo Q., Yang S., Lin X., Zhang Y., Tian B., Tang X., et al. (2013) Increased dissolution and oral absorption of itraconazole/Soluplus extrudate compared with itraconazole nanosuspension. European Journal of Pharmaceutics and Biopharmaceutics [Internet] Array: 1285-1292 Google Scholar