ABSTRACT
Background
Nipah virus is a deadly infectious virus that was first isolated and identified from Malaysia. Since then, a number of Nipah virus outbreaks have been reported from Bangladesh and India. Transmission of the disease occurs through Pteropus genus fruit bats. The case fatality rate of this infection is very high when compared with other viral zoonoses. At present, there are no approved vaccines or drugs available to prevent or treat this infection. A number of studies are ongoing to develop an efficient vaccine candidate to combat this deadly virus. The majority of them concentrate on the structural and non-structural proteins, which are the main targets of neutralizing antibodies.
Materials and Methods
Here, we analyzed the genome sequence identity of two Nipah virus strains, Indian and Malaysian, and also the amino acid identity between the two structural proteins (Attachment glycoprotein G and Fusion protein F) and one non-structural protein (W protein) of those two strains.
Results and Discussion
It was found that there is a considerable amount of nucleotide sequence homology between the initial strain that originated from Malaysia and the strain that is now found in India. Furthermore, the Structural and Non-structural proteins of these two strains exhibit a high degree of similarity.
Conclusion
Hence, a vaccine candidate designed using either NiV M or NiV B can be effectively used as a potent vaccine.
INTRODUCTION
Viral diseases contribute significantly to both morbidity and mortality across various global communities. A substantial portion of these viruses are zoonotic, originating in animals before transmitting to humans.1 Nipah virus (NiV) infection is a zoonosis. Fruit bats or flying foxes of the Pteropodidae family serve as the primary reservoir for NiV. Recently, the World Health Organization (WHO) has classified NiV as a highly preferred pathogen of global concern due to its emergence as a zoonotic threat.2 NiV is recognized for its ability to induce illness in both pigs and humans. Contracting NiV can lead to encephalitis, characterized by inflammation of the brain, resulting in a spectrum of symptoms ranging from mild to severe, potentially culminating in fatality.3
NiV derived its name from a village in Malaysia, Kampung Sungai Nipah; it was initially discovered in 1998. It then extended to Singapore in 1999 through exported pigs, resulting in infections among abattoir workers.4 Since 2001, NiV infection in humans has been independently identified in both India and Bangladesh. Subsequently, annual occurrences of infections have been consistently reported in Bangladesh.5 As of now, South Asia and Southeast Asia have reported more than 600 human NiV infection cases (Figure 1). The fatality rates have varied between 40% and 75%. Consequently, it represents a significant threat to human health.6 Because of its high degree of pathogenicity and the absence of successful treatments or vaccines, NiV is categorized as a BSL-4 pathogen (Biosafety Level-4).7
NiV transmission from bats to humans occurs via two primary pathways. Firstly, it can spread via intermediate hosts such as pigs and horses. Secondly, there is a food-borne transmission route, where date palm sap or fruits like guava or rambutan contaminated by the fruit bats urine or saliva serve as the vehicle for infection (Figure 2).8
The initial outbreak took place in 2001 in Siliguri district of West Bengal in India. Subsequently, an outbreak was documented in Nadia district, also located in West Bengal, in 2007.9 A more recent incident occurred in 2023, which was reported from the Kozhikode district of Kerala, a state in southern India. Individuals involved in this case reportedly acquired NiV from fruit bats.10 All of these outbreaks have exhibited high mortality rates, with the recent Kerala outbreak recording a staggering 91% fatality rate over time. In humans, the infection rapidly progresses to severe illness, leading to significant respiratory complications and profound encephalitis.11 The lack of antibodies or effective therapeutics to combat this illness underscores a critical need, driving scientists worldwide to pursue the development of a robust NiV vaccination and therapy strategy.
Top of Form
The NIV genome is comprised of a single-stranded RNA with a negative polarity of approximately 18.2 kb in length. Six structural proteins are encoded by it: Nucleoprotein (N), Phosphoprotein (P), Matrix protein (M), Fusion protein (F), Attachment glycoprotein (G), and the large protein also known as RNA polymerase protein (L). Additionally, the P gene holds responsibility for encoding three non-structural proteins through RNA editing (V and W proteins) or an alternative open reading frame (C protein) (Figure 3).12
F and G proteins play a vital role in facilitating the virion’s adherence to cellular receptors and subsequent entry into the host cell. Antibodies targeting the attachment glycoprotein G are crucial for neutralizing NiV infection. M protein is involved in budding and morphogenesis, while the N protein serves as an indispensable component of the viral life cycle by acting as a template for RNA dependant RNA Polymerase.13
The objective of the study is to examine both the nucleotide and amino acid sequence similarities among the structural and non-structural proteins (Attachment Glycoprotein (G), Fusion protein (F), and W protein) of Nipah virus strains from India and Malaysia. Therefore, the research can conclude that vaccine candidates utilizing proteins from either the Indian or Malaysian Nipah virus strain can be employed successfully for vaccine development against Nipah virus infection.
Role of Attachment Glycoprotein (G), Fusion protein (F), and W protein in Virulence
A type II protein is the attachment Glycoprotein (G), having a tetrameric structure and it binds with the receptors on the host cell ephrin-B2 and ephrin-B3,13 which have been well preserved among numerous species. Recently, certain vaccines containing NiV-G have been demonstrated to prevent potentially fatal challenge infections during preliminary clinical trials. Some of the vaccine candidates utilize Recombinant viruses such as Vesicular Stomatitis Virus (VSV),14 Canarypox, Vaccinia virus, Measles virus, Rabies virus, and Adenovirus platforms have been developed.15 Thus far, the only licensed and approved Henipavirus vaccine for equine use is the Equivac® HeV, an equine vaccine against HeV and NiV, which was in Australia in 2012. This subunit vaccine contains the soluble form of HeV-G (HeVsG) and has demonstrated efficacy in protecting cats, ferrets, and non-human primates,16 but not pigs from NiV. The efficacy of HeVsG in safeguarding from NiV infection in certain animal models underscores the necessity for assessing a vaccine incorporating NiV glycoprotein G.17 The antibodies targeting the NiV- G glycoprotein are acknowledged for their pivotal role in eradicating NiV infection and in inducing vaccine-triggered protective immunity.18
A vaccine for NiV has entered into a human clinical trial for the first time, which includes a DNA Vaccine (DNA-G) and a Recombinant Chimpanzee Adenovirus vaccine (AdC68-G). Both express NiV-G and their effectiveness and immunogenicity were evaluated in mice and hamsters. The findings indicate that both vaccinations activated the formation of enduring neutralizing antibodies and a strong T-cell response against the G protein post-vaccination in BALB/c mice, as well as provided defense against fatal infections with NiV-M and NiV-B in Syrian golden hamsters.19
Following cellular attachment, the virus utilizes a class I trimeric Fusion protein (F) to facilitate the merging of membranes of viruses and cells. It is accomplished through a structural shift of the F protein from an unstable prefusion state to an extremely stable post-fusion state, promoting membrane fusion and mediating viral entry. Studies on NiV have demonstrated that the prefusion form of F protein is most significant for the design and development of vaccines since F protein in this form is the primary target of neutralizing antibodies. Therefore, several iterations have been performed to stabilize the prefusion conformation of F proteins using a design based on structure and the addition of a fold on or trimerization domain GCN4.20
Upon infection, viruses encounter a variety of antiviral host responses, with Interferon (IFN) reactions holding crucial functions in the early stages of innate immunity and subsequent acquired immunity modulation.21 By attaching to receptors on target cells, type 1 IFNs carry out biological activities by initiating the autophosphorylation and activation of Janus Kinases (JAKs), specifically JAK1 and tyrosine kinase 2. This activation leads to the phosphorylation of signaling molecules STAT1 and STAT2. Phosphorylated STATs then associate with Interferon Regulatory Factor 9 (IRF-9), forming an Interferon-Stimulated Gene Factor 3 (ISGF3), that triggers transcription of numerous genes involved in establishing the cell’s resilience to viruses. In NiV, the V and/or C proteins have demonstrated IFN-antagonist activity. Additionally, the four P gene products in NiV have been shown to possess IFN antagonist action. Through their interaction with STAT1 and STAT2, the V and W proteins influence IFN signalling, while the TLR3 pathway is likewise inhibited by the W protein. There have been hints that the C protein exhibits partial inhibition of IFN. Even so, its target remains ambiguous. Often, proteins that block viral IFN are recognized as significant virulence factors.12 Consequently, investigations into the role of these NiV accessory proteins are expected to yield new perspectives on pathogenesis and provide crucial data for the development of vaccines.
Protein W of NiV possesses a signal for nuclear localization within its distinct domain at the end, enabling it to sequester STAT1 in the nucleus. This action results in the creation of both nuclear and cytoplasmic inhibition of STAT1. Acting as antagonists for Interferon (IFN) responses, proteins P, V and W sequester STAT proteins, while W protein exhibits the highest efficiency and protein P displays the lowest. Furthermore, V and W proteins of NiV have the capability to inhibit the IFN-b promoter and IRF3-responsive promoter (ISG54 promoter) which are activated by viruses. Notably, the W protein alone demonstrates robust suppression of promoter activation in reaction to stimulation of Toll-like receptor 3.22
MATERIALS AND METHODS
Retrieval of Nucleotide sequence of NiV M and NiV B
The nucleotide sequences of both Malaysian and Indian Nipah virus isolates were obtained from the NCBI database (https://www.ncbi.nlm.nih.gov/). Subsequently, these sequences were utilized for BLAST analysis. Nipah Virus M strain genome length is18246 nucleotides,22 whereas the Nipah virus B strain has a genome of 18242 nucleotides. The Nipah virus exhibited a high mortality rate and is notably more lethal compared to other strains.23 In many instances, the infection leads to severe respiratory illness and fatal encephalitis in humans.24 The Nipah virus shows a strong tendency for human-to-human transmission.25 These factors have led to the choice of studying the Nipah virus. The protein sequences were saved in FASTA format for further sequential analysis.
Retrieval of protein sequences
The amino acid sequences of Structural proteins, such as the attachment glycoprotein G and Fusion glycoprotein F, as well as the Non-Structural protein W, were retrieved from the NCBI Database. These sequences were then employed for BLAST Analysis.26
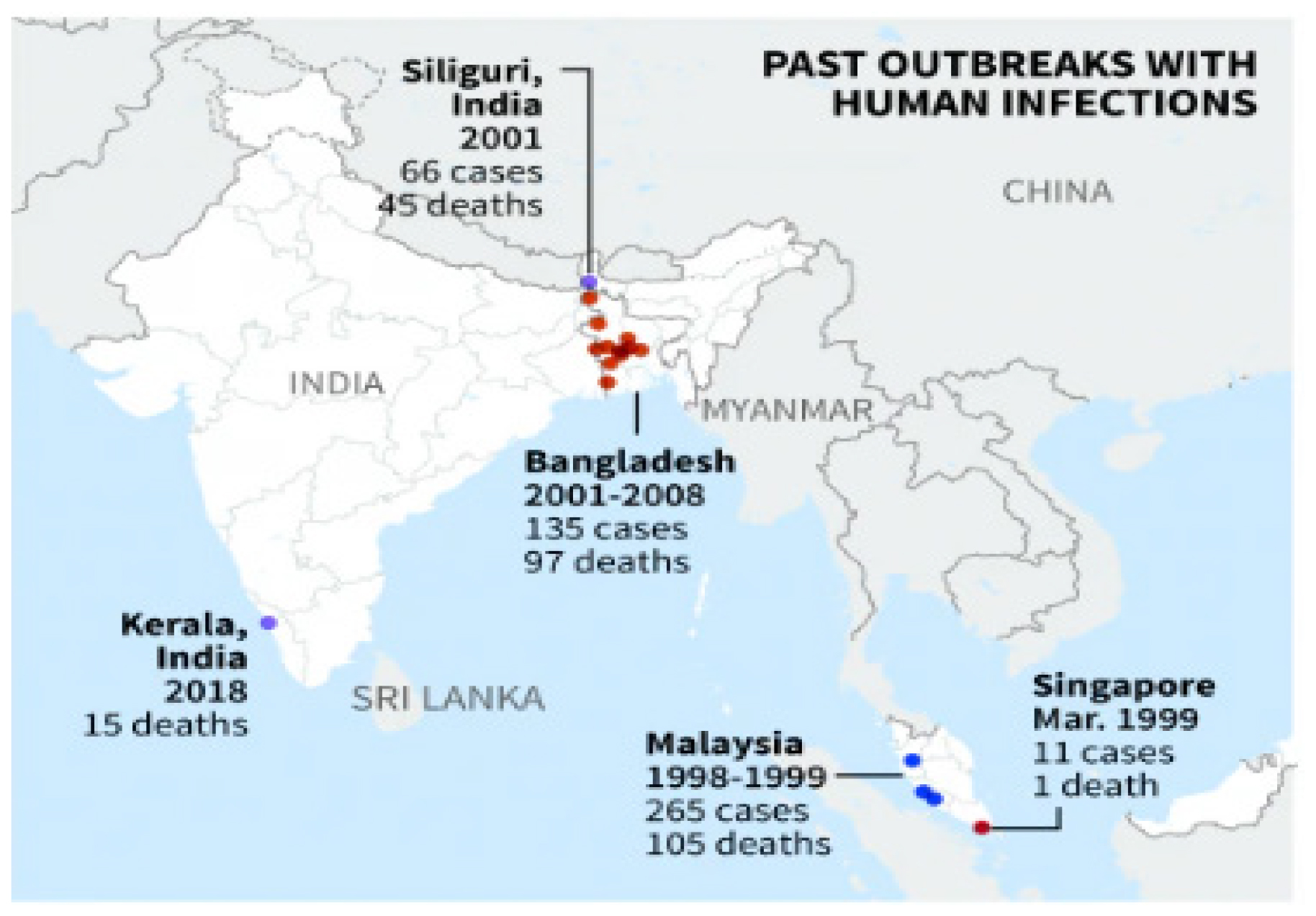
Figure 1:
The outbreaks of the Nipah virus in geographical regions ranged from the initial outbreak in Malaysia to India.
RESULTS
Nucleotide sequences retrieval and BLAST analysis
The nucleotide sequences of NiV Malaysia and NiV India (GenBank accession numbers AJ564623.1 and MH523642.1 respectively) were obtained from NCBI database. These sequences were then utilised for BLAST analysis (Table 1).
Sl. No. | Isolate | Strain | Location | GenBank | Length (bp) | Homology % |
---|---|---|---|---|---|---|
1 | NV/MY/99/UM-0128 | NiV M | Malaysia | AJ564623.1 | 18246 | 91.47 |
2 | MCL-18-H-1088 | NiV B | India | MH523642.1 | 18242 |
Nucleotide sequences and their Homology.
Protein Sequences Retrieval and BLAST Analysis
The amino acid sequences of the three proteins: Attachment glycoprotein G, Fusion Protein F (Structural protein) and W Protein (Non-structural protein) of the Nipah virus strain isolated from India and Malaysia were retrieved from NCBI Database (Tables 2, 3 and Figure 4). The same was employed for BLAST analysis. The genomes of NiV M (GenBank Accession: AJ564623.1) and NiV B (GenBank Accession: MH523642.1) have 91.47 percent nucleotide sequence identity. The F, G and W proteins of NiV M and NiV B have amino acid sequence similarities of 95.85%, 98.53%, and 84.06%, respectively.
Sl. No. | Name of Protein | Strain | NCBI Accession No. | No. of Amino Acids |
---|---|---|---|---|
1 | Attachment Glycoprotein G | NiV M | CBM41034.1 | 602 |
2 | Fusion Glycoprotein F | CBM41033.1 | 546 | |
3 | W Protein | QHR78997.1 | 450 | |
4 | Attachment Glycoprotein G | NiV B | QBQ56723.1 | 602 |
5 | Fusion Glycoprotein F | QBQ56722.1 | 546 | |
6 | W Protein | QBQ56719.1 | 450 |
Protein sequences retrieved from NCBI.
Name of Protein | Country (Outbreak/ Isolated) | Homology % |
---|---|---|
G Glycoprotein | Malaysia and India | 98.53 |
F Glycoprotein | 95.85 | |
W Protein | 84.06 |
BLAST Analysis of structural and Non-structural protein.
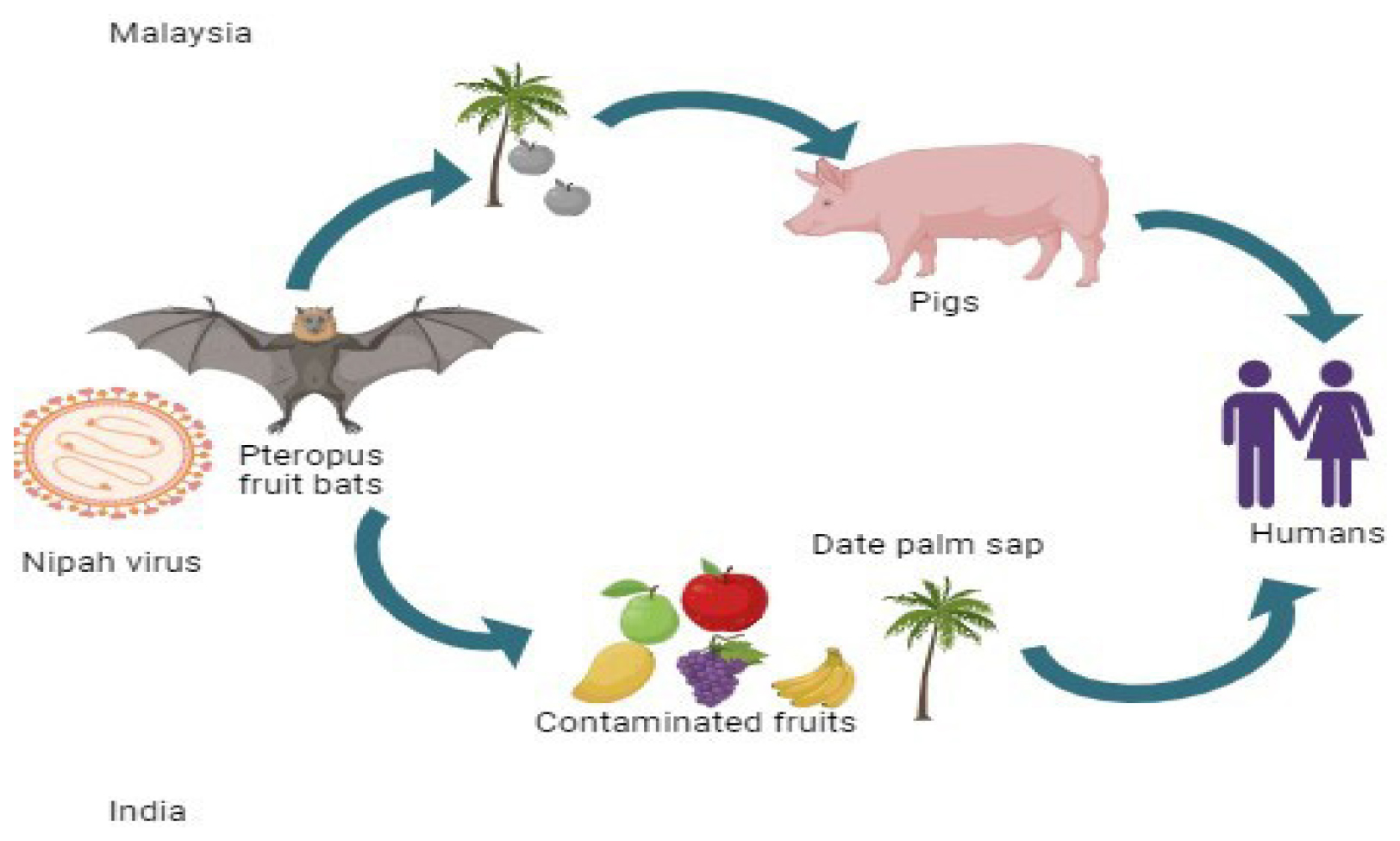
Figure 2:
Transmission of Nipah virus from Fruit bats to humans: Malaysia and India.
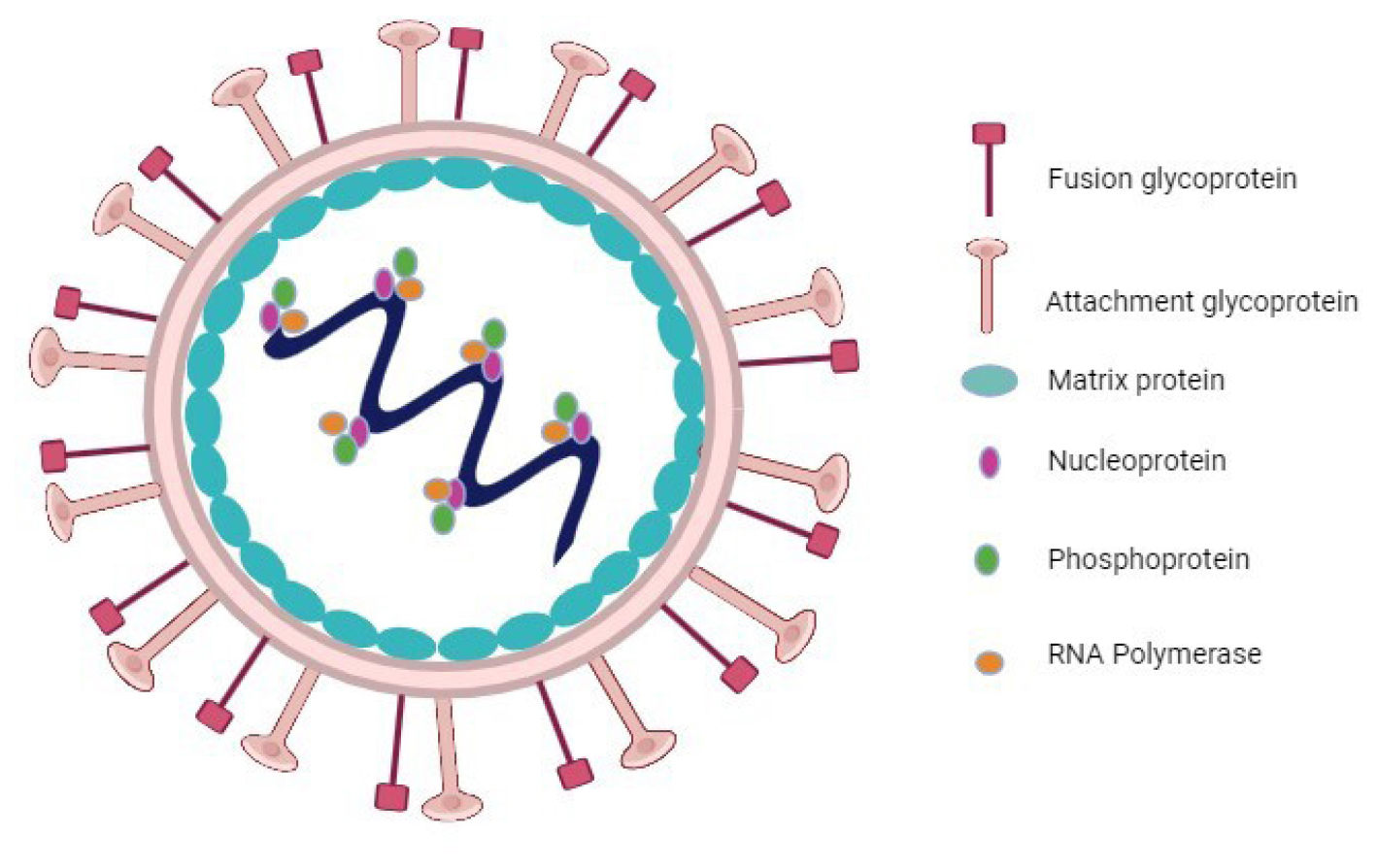
Figure 3:
Structure of the Nipah virus.
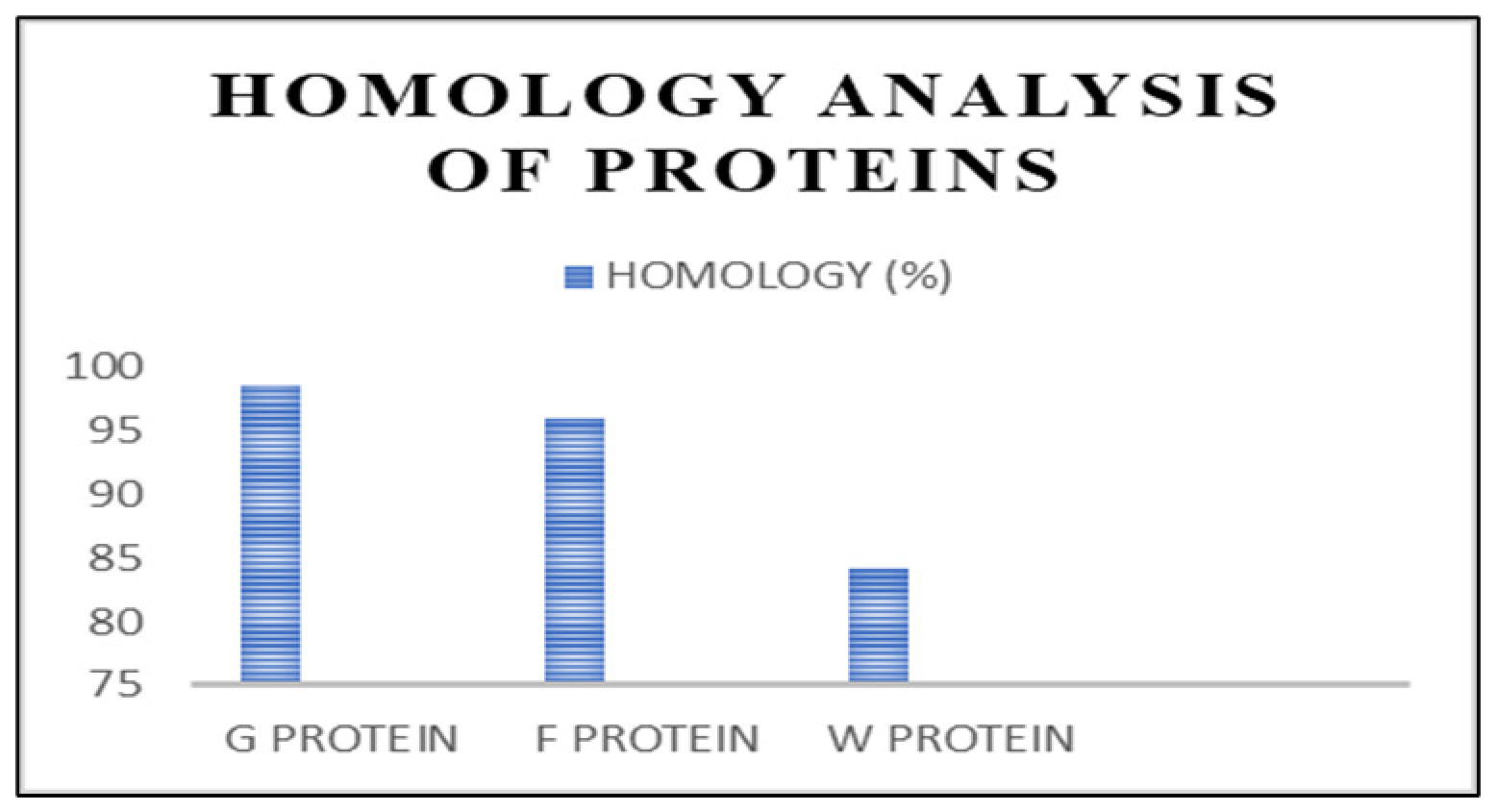
Figure 4:
Graphical representation of the homological analysis.
DISCUSSION
Nipah virus infections have resulted in a mortality rate ranging from 72% to 86% among those infected in Bangladesh and India. The virus spreads through the bodily secretions of bats, pigs, and other infected individuals. Despite the fact that it was initially identified in humans in 1998, there are currently no authorized drugs or vaccines available for its treatment.27 Examination of NiV genome sequences has delineated two distinct clades: NiV M comprising isolates from Malaysia and NiV B encompassing NiV isolates from Bangladesh and India. These clades have been implicated in outbreaks across various geographical areas. Clinical observations have unveiled notable distinctions between the outbreaks of NiV M and NiV B. NiV B exhibits a shorter time for incubation in comparison to NiV M. Additionally, a significant proportion of NiV B infections manifest symptoms related to the respiratory system alongside deadly encephalitis, while NiV M primarily induces encephalitis with fewer respiratory manifestations. Moreover, NiV B infections are associated with a higher death rate than NiV M.
The nucleotide sequence identity between the genomes of NiV M (GenBank Accession: AJ564623.1) and NiV B (GenBank Accession: MH523642.1) is 91.47%. The amino acid sequence similarity between the F, G and W proteins of NiV M and NiV B is 95.85%, 98.53%, and 84.06%, respectively. As NiV originated in Malaysia in 1998 and still the episodes occur in Southeast Asia annually, the proteins of NiV M and NiV B remain highly conserved for 2.5 decades without any variations. Hence the Structural and Non-structural proteins of Malaysian and Indian NiV strains can be employed for the designing of NiV vaccine candidates. Recent studies by de Wit et al.20 and Lu et al.19 have demonstrated that the main vaccine candidates for the Nipah virus are the structural proteins G and F. As per the earlier studies conducted towards the identification of the immunodominant protein of NiV, the structural proteins F and G and non-structural protein Ware are in the scope, and our studies validated the same. Sen et al. analyzed the various Nipahvirus strains to ascertain the conservation patterns of amino acids within the proteins. Nine proteins from Nipah virus were considered as potential targets for therapy. Through computational methods, four peptide inhibitors targeting the G, F and M proteins were identified, along with 146 small molecule inhibitors targeting the F, G, M, N and P proteins. This investigation provides insights into potential target sites for drug development.27,28 Srivastava and Kolbe examined nine proteins, namely the C protein (gi-1859635642), glycoprotein (gi-253559848), fusion protein (gi-13559813), nucleoprotein (gi-1679387250), matrix protein (gi-13559811), phosphoprotein (gi-1802790259), W protein (gi-374256971), V protein (gi-1802790260) and RNA polymerase (gi-15487370). They retrieved up to 96 full-length protein sequences from various strains and sources of NiV to aid in the development of multipath vaccines.28,29
CONCLUSION
The persistent risk of abrupt NiV outbreaks, notably in India and Bangladesh, underscores the necessity for promptly deployable countermeasure strategies within public health responses. Currently, there are no authorized NiV vaccines that are accessible for human use. Analyzing the structural and functional aspects of viral proteins could aid in designing possible vaccine strategies. This study specifically assessed the viability of utilizing the Structural and Non-Structural proteins G, F, and W proteins of Nipah virus as a promising vaccine candidate.
Several experimental NiV vaccines have undergone testing in various preclinical animal models. These studies primarily aimed to assess the immunogenicity and efficacy against NiV challenge infection. Efficacy in these investigations has largely been linked to the production of NiV-specific antibodies, with immunological surveillance that is based mostly on the identification of antibodies that neutralize viruses. Previous studies focused on identifying the immunodominant protein of NiV, particularly examining the structural proteins G and F as well as the Non-Structural protein W confirms the findings.
Cite this article:
Pariyapurath NK, Jagannathan S, Mathanmohun M, Pillai SB, Dhandapani K, Pachamuthu RG, et al. Targeted Immunization Strategies and Designing Vaccine against Indian Nipah Virus Strain (NiV B) and Malaysian Variant (NiV M). Int. J. Pharm. Investigation. 2024;14(4):1201-7.
ACKNOWLEDGEMENT
The authors would like to thank Ms. Reshma and Mr. S. Raju, Department of Microbiology, Pasteur Institute of India, Coonoor, Tamil Nadu, India, Dr. Vijayakumar Rajendran, IISC, Bangalore, India, Dr. Paramasivan Rajaiah, ICMR-Vector Control Research Centre, Madurai, Tamil Nadu, India for their continuous support and guidance.
ABBREVIATIONS
NiV | Nipah virus |
---|---|
WHO | World Health Organization |
BSL-4 | Biosafety Level-4 |
N | Nucleoprotein |
P | Phosphoprotein |
M | Matrix protein |
F | Fusion protein |
G | Attachment glycoprotein |
L | Large protein |
V | V protein |
W | W protein |
C | C protein |
RNA | Ribonucleic acid |
NCBI | National Center for Biotechnology Information |
BLAST | Basic Local Alignment Search Tool |
VSV | Vesicular Stomatitis Virus |
HeV | Hendra virus |
HeVsG | Hendra virus soluble glycoprotein |
IFN | Interferon |
JAK | Janus kinase |
STAT | Signal Transducer and Activator of Transcription |
IRF-9 | Interferon Regulatory Factor 9 |
ISGF3 | Interferon-Stimulated Gene Factor 3 |
TLR3 | Toll-like receptor 3 |
ISG54 | Interferon-Stimulated Gene 54 |
References
- Sachan A, Singh R, Gupta B, Kulesh R. Nipah virus and its zoonotic importance: a review. J Entomol Zool Stud. 2023;11(1):208-13. [CrossRef] | [Google Scholar]
- Talukdar P, Dutta D, Ghosh E, Bose I, Bhattacharjee S. Molecular pathogenesis of Nipah virus. Appl Biochem Biotechnol. 2023;195(4):2451-62. [PubMed] | [CrossRef] | [Google Scholar]
- European Centre for Disease Prevention and Control (ECDC). Factsheet on Nipah virus Disease. 2023 Available fromhttps://www.ecdc.europa.eu/en/infectious-disease-topics/z-disease-list/nipah-virus-disease/factsheet-nipah-virus-disease
- de Campos GM, Cella E, Kashima S, Alcântara LC, Sampaio SC, Elias MC, et al. Updated insights into the phylogenetics, Phylodynamics and genetic diversity of Nipah virus (NiV). Viruses. 2024;16(2):171 [PubMed] | [CrossRef] | [Google Scholar]
- Luby SP, Gurley ES, Hossain MJ. Transmission of human infection with Nipah virus. Clin Infect Dis. 2009;49(11):1743-8. [PubMed] | [CrossRef] | [Google Scholar]
- Gazal S, Sharma N, Gazal S, Tikoo M, Shikha D, Badroo GA, et al. Nipah and Hendra viruses: deadly zoonotic paramyxoviruses with the potential to cause the next pandemic. Pathogens. 2022;11(12) [PubMed] | [CrossRef] | [Google Scholar]
- Bruno L, Nappo MA, Ferrari L, Di Lecce R, Guarnieri C, Cantoni AM, et al. Nipah virus disease: epidemiological, clinical, diagnostic and legislative aspects of this unpredictable emerging zoonosis. Animals (Basel). 2022;13(1) [PubMed] | [CrossRef] | [Google Scholar]
- Hassan D, Ravindran R, Hossain A. Nipah virus Mystery: insight into Transmission and Mechanism of Disease Progression. J Pure Appl Microbiol. 2022;16(1):26-34. [CrossRef] | [Google Scholar]
- World Health Organization (WHO). Disease outbreak news, Nipah virus infection India October. 2023 [CrossRef] | [Google Scholar]
- Mohandas S, Shete A, Sarkale P, Kumar A, Mote C, Yadav P, et al. Genomic characterization, transcriptome analysis and pathogenicity of the Nipah virus (Indian isolate). Virulence. 2023;14(1):2224642 [PubMed] | [CrossRef] | [Google Scholar]
- Singh RK, Dhama K, Chakraborty S, Tiwari R, Natesan S, Khandia R, et al. Nipah virus: epidemiology, pathology, immunobiology and advances in diagnosis, vaccine designing and control strategies-a comprehensive review. Vet Q. 2019;39(1):26-55. [PubMed] | [CrossRef] | [Google Scholar]
- Liew YJ, Ibrahim PA, Ong HM, Chong CN, Tan CT, Schee JP, et al. The immunobiology of Nipah virus. Microorganisms. 2022;10(6) [PubMed] | [CrossRef] | [Google Scholar]
- Ortega V, Zamora JL, Monreal IA, Hoffman DT, Ezzatpour S, Johnston GP, et al. Novel roles of the Nipah virus attachment glycoprotein and its mobility in early and late membrane fusion steps. mBio. 2022;13(3) [PubMed] | [CrossRef] | [Google Scholar]
- Woolsey C, Borisevich V, Fears AC, Agans KN, Deer DJ, Prasad AN, et al. Recombinant vesicular stomatitis virus-vectored vaccine induces long-lasting immunity against Nipah virus disease. J Clin Invest. 2023;133(3) [PubMed] | [CrossRef] | [Google Scholar]
- Gómez Román R, Tornieporth N, Cherian NG, Shurtleff AC, L’Azou Jackson M, Yeskey D, et al. Medical countermeasures against henipaviruses: a review and public health perspective. Lancet Infect Dis. 2022;22(1) [PubMed] | [CrossRef] | [Google Scholar]
- Geisbert TW, Bobb K, Borisevich V, Geisbert JB, Agans KN, Cross RW, et al. A single dose investigational subunit vaccine for human use against Nipah virus and Hendra virus. npj Vaccines. 2021;6(1):23 [PubMed] | [CrossRef] | [Google Scholar]
- Mishra G, Prajapat V, Nayak D. Advancements in Nipah virus treatment: analysis of current progress in vaccines, antivirals and therapeutics. Immunology. 2024;171(2):155-69. [PubMed] | [CrossRef] | [Google Scholar]
- Kalodimou G, Veit S, Jany S, Kalinke U, Broder CC, Sutter G, et al. A soluble version of Nipah virus glycoprotein G delivered by vaccinia virus MVA activates specific CD8 and CD4 T cells in mice. Viruses. 2019;12(1) [PubMed] | [CrossRef] | [Google Scholar]
- Lu M, Yao Y, Zhang X, Liu H, Gao G, Peng Y, et al. Both chimpanzee adenovirus-vectored and DNA vaccines induced long-term immunity against Nipah virus infection. npj Vaccines. 2023;8(1):170 [PubMed] | [CrossRef] | [Google Scholar]
- de Wit E, Feldmann F, Cronin J, Goldin K, Mercado-Hernandez R, Williamson BN, et al. Distinct VSV-based Nipah virus vaccines expressing either glycoprotein G or fusion protein F provide homologous and heterologous protection in a nonhuman primate model. EBiomedicine. 2023;87:104405 [PubMed] | [CrossRef] | [Google Scholar]
- Pelissier R, Iampietro M, Horvat B. F1000 Research Ltd. ‘Recent advances in the understanding of Nipah virus immunopathogenesis and antiviral approaches,’ F1000Research. F1000Res. 2019;8 [PubMed] | [CrossRef] | [Google Scholar]
- Keiffer TR, Ciancanelli MJ, Edwards MR, Basler CF. Interactions of the Nipah virus, P, V and W proteins across the STAT family of transcription factors. mSphere. 2020;5(6) [PubMed] | [CrossRef] | [Google Scholar]
- Baleanu D, Shekari P, Torkzadeh L, Ranjbar H, Jajarmi A, Nouri K, et al. Stability analysis and system properties of Nipah virus transmission: A fractional calculus case study. Chaos Solitons Fract. 2023;166:112990 [CrossRef] | [Google Scholar]
- Spiropoulou CF. Nipah virus outbreaks: still small but extremely lethal. J Infect Dis. 2019;219(12):1855-7. [PubMed] | [CrossRef] | [Google Scholar]
- Malabadi RB, Kolkar KP, Chalannavar RK, Acharya M, Mudigoudra BS. Cannabis sativa: 2023-Outbreak and Re-emergence of Nipah virus (NiV) in India: role of Hemp oil. GSC Biol Pharm Sci. 2023;25(1):63-77. [CrossRef] | [Google Scholar]
- Rodrigue V, Gravagna K, Yao J, Nafade V, Basta NE. Current progress towards prevention of Nipah and Hendra disease in humans: A scoping review of vaccine and monoclonal antibody candidates being evaluated in clinical trials. Trop Med Int Health. 2024;29(5):354-64. [PubMed] | [CrossRef] | [Google Scholar]
- Sharma SK, Srivastava S, Kumar A, Srivastava V. Anticipation of antigenic sites for the goal of vaccine designing against Nipah virus: an Immunoinformatics inquisitive quest. Int J Pept Res Ther. 2021;27(3):1899-911. [PubMed] | [CrossRef] | [Google Scholar]
- Sen N, Kanitkar TR, Roy AA, Soni N, Amritkar K, Supekar S, et al. Predicting and designing therapeutics against the Nipah virus. PLOS Negl Trop Dis. 2019;13(12) [PubMed] | [CrossRef] | [Google Scholar]
- Srivastava S, Kolbe M. Novel “GaEl antigenic patches” identified by a “reverse Epitomics” approach to design multipatch vaccines against NIPAH infection, a silent threat to global human health. ACS Omega. 2023;8(35):31698-713. [PubMed] | [CrossRef] | [Google Scholar]