ABSTRACT
Background
Tyrosinase is an enzyme involved in the production of L-DOPA. To look for novel tyrosinase inhibitors, we investigated the non-protein fraction from the sericin extract of the silk cocoon shell of Antheraea proylei J. for its ability to inhibit tyrosinase.
Materials and Methods
The non-protein fraction was subjected to GC-MS studies. The compounds identified from the GC-MS study were screened in silico for their ability to bind to tyrosinase enzyme. In vitro tyrosinase inhibitory assay of the non-protein extract was also studied.
Results and Discussion
Our results showed that the component, 1,3-bis(cinnamoyloxymethyl)adamantine showed stable binding with the tyrosinase enzyme. Molecular dynamics simulation of the complex of 1,3-bis(cinnamoyloxymethyl)adamantine and tyrosinase showed steady root mean square deviation values, Radius of gyration, root mean square fluctuation and solvent accessible surface area. Hydrogen bonding and van der Wals interaction were present between the ligand and the enzyme. In vitro tyrosinase assay showed that our extract had tyrosinase inhibitory effects.
Conclusion
The non-protein fraction from the sericin extract of Antheraea proylei J. can be further studied for their feasibility as a commercially available tyrosinase inhibitor.
INTRODUCTION
Tyrosinase (EC1.14.18.1) is a key enzyme in the process of melanogenesis. Tyrosinase catalyses the rate-limiting steps of hydroxylation of L-tyrosine to 3,4-dihydroxyphenylalanine (L-DOPA) and further oxidization of L-DOPA to produce melanin.1 Melanin is responsible for skin, hair and eye colour and plays a critical role in protecting the skin against ultraviolet radiation. Over accumulation of melanin is associated with disorders, such as freckles, melasma, solar lentigo, post-inflammatory hyperpigmentation and neurodegeneration like Parkinson’s disease.2 Tyrosinase inhibitors have found application in cosmetics and medicine. However, many known tyrosinase inhibitors are found to be cytotoxic, unstable and carcinogenic.3 Hence, there is a need for novel non-toxic, effective and stable tyrosinase inhibitory agents.
Silk cocoon shell is composed of two main proteins, sericin (20-30% w/w) and fibroin (70-80% w/w) and some trace amounts of non-protein components (1-5% w/w). The non-protein components include pigments, waxes, sugars, metabolites and certain inorganic compounds. The sericin-containing component has reported to have several valuable properties like antioxidant, antitumor and antimicrobial activities.4 However, the composition, preparation and biological activities of the non-protein component of the sericin solution haven’t been looked into yet.5 In the present investigation, the non-protein component of the sericin extract from the cocoon shells of Antheraea proylei J was determined using GC-MS chromatography. In silico and in vitro tyrosinase inhibition was performed to check the possibility of furthering the non-sericin component as a potential tyrosinase inhibitor.
MATERIALS AND METHODS
Materials
Cocoon shells of Antheraea proylei J. were acquired from tasar farm, Mortbung, Manipur, India. Ethanol from Jebsen and Jessen GmbH and Co. (Germany). Mushroom tyrosinase, kojic acid were from Sigma Chemical Co. (USA).3,4-dihydroxy-L-phenylalanine (L-DOPA) was from HiMedia (India).
Methods
Preparation of the non-protein component of sericin extract
The sericin extract from the cocoon shells of oak tasar silkworm was prepared using hot water extraction method.6 The cocoon shells were autoclaved and the aqueous solutions obtained were centrifuged at 8000 g for 15 min to remove any suspended insoluble materials. The resulting final aqueous solution was the sericin extract. The non-protein component in the sericin extract was fractionated using ethanol precipitation. Sericin extract and ethanol was mixed in the ratio of 1:8. The extract was fractionated using ethanol precipitation. The mixture was kept overnight at -20°C, followed by centrifugation at 15000 g for 30 min at 40°C. The resulting supernatant contained the non-protein component which was concentrated by rotary evaporation.
GC-MS analysis
Gas Chromatography-Mass Spectrometry (GC-MS) analysis of the ethanolic extract was carried out using the GC-MS (Shimadzu QP-2010 Plus with Thermal Desorption System TD 20). RTx-5MS column (30 m in length, 0.25 mm internal diameter and 0.25 μm film thickness) was used. The analysis was done by injecting 1 μL of the sample with a split ratio of 10.0. The column oven temperature was set at 80°C for 0.5 min then increased from 80°C to 300°C at a rate of 10°C/min. Helium gas was used as the carrier gas. The column flow rate was maintained at 1.2 mL/min and the injection temperature was 260°C. Compounds were identified by comparing the spectral data of peaks with the corresponding standard mass spectra from the library database.7
In silico analysis for tyrosinase inhibition
Preparation of ligands and protein for docking
Screening of compounds for binding with tyrosinase
Molecular dynamics simulation studies
The 1,3-Bis(cinnamoyloxymethyl)adamantine-tyrosinase complex was subjected to molecular dynamics simulation to check for stability in docking. Molecular dynamics simulation was performed by GROMACS (Groningen MAchine for Chemical Simulations) version 2022.3. The initial structure is prepared using pdb2gmxby adding hydrogens. CHARMM36 force field is used to describe the interactions and ligand topology.11 Trajectory analysis is performed using the inbuilt GROMACS programs. All visual analysis is performed using Visual Molecular Dynamics (VMD) software.12
Binding free energy analysis by MM/PBSA
g_mmpbsa program is used to evaluate the binding energy profile of protein and ligand.13 Residue-wise free energy decomposition is derived using the g_mmpbsa associated python program, MmPbSaDecomp.py script.
In vitro antityrosinase assay
The antityrosinase activity of the ethanolic extract of the non-protein component was determined according to Kim et al., 2005.14 The reaction mixture was constituted by mixing 2 U of mushroom tyrosinase, 8 mM DOPA and the sample in 100 mM phosphate buffer, pH 6.8, followed by incubation for 5 min at room temperature. The amount of dopachrome produced was measured at 480 nm. Kojic acid was taken as a positive control. The enzyme activity was expressed as per cent enzyme activity.
IC50determination
The concentration of the sample required for 50% inhibition of the enzyme (IC50) was determined using OriginPro 8. The results were expressed as Mean±Standard Deviation (SD) of three independent experiments.
RESULTS
The composition of the non-protein component of the sericin extract from the cocoon of Antheraea proylei J. was identified by GC-MS analysis as described in GC-MS analysis. The GC-MS spectra are shown in Figure 1. The compounds identified by the GC-MS analysis are mentioned in Table 1. Thirty-four metabolites were identified and it includes polyamines, phenolics, cholesterol derivatives, pyrazole, pyrazole derivatives, fatty acids and fatty acid derivatives.
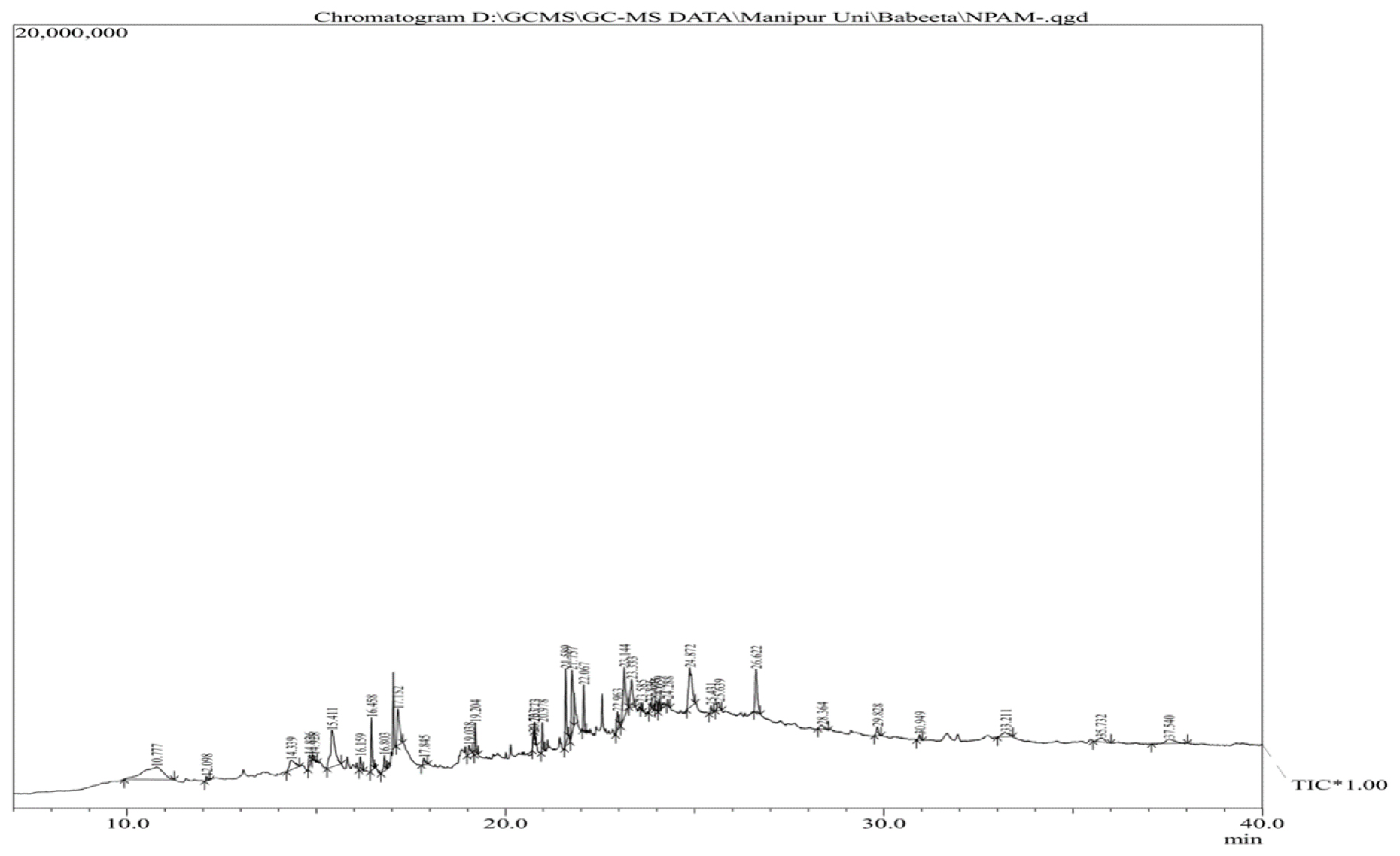
Figure 1:
GC-MS spectra of the ethanolic fraction from the sericin extract of the cocoon shells of Antheraea proylei J.
Sl. No. | Compound name | Binding energy (kcal/mol) |
---|---|---|
1 | 3,3’-Iminobispropylamine | -4.4 |
2 | Phenol, 2,4-Bis(1,1-Dimethylethyl)- | -6.7 |
3 | 3-Methyl-1,4-diazabicydo[4.3.0]nonan-2,5-dione, N-acetyl | -6.7 |
4 | 4-(1,1-Dioxidotetrahydro-3-thienyl)morpho | -5.4 |
5 | Pyrrolo[1,2-a]pyrazine-1,4-dione, hexahydro- | -7.1 |
6 | 8-Octadecanone | -5.4 |
7 | Pyrrolo[1,2-a]pyrazine-1,4-dione, hexahydro-3-(2-methylpr | -6.4 |
8 | n-Hexadecanoic acid | -5.1 |
9 | N,N’-Dibutylidene-hydrazine | -4.2 |
10 | 9-Octadecenoic acid (Z)- | -5.6 |
11 | Hexadecanamide | -5.6 |
12 | 9,12-Octadecadienoic Acid (Z,Z)- | -5.6 |
13 | 9-Octadecenamide, (Z)- | -5.4 |
14 | Octadecanamide | -5.1 |
15 | octadecanoic acid, 3-oxo-, ethyl ester | -5.8 |
16 | 2-Ethylbutyric acid, tridecyl ester | -5.2 |
17 | 9-t-Butyltricyclo[4.2.1.1(2,5)]decane-9,10-diol | -6.8 |
18 | 1-Cyclohexyldimethylsilyloxybutane | -4.6 |
19 | 4-(2-Tert-Butyl-5-oxo-1,3-Dioxolan-4-yl)butylformamide | -6.1 |
20 | Octadecanoic acid, 2,3-dihydroxypropyl ester | -5.2 |
21 | 4,7,10,13,16,19-Docosahexaenoic acid, methyl ester, (all-Z | -6.8 |
22 | 9-Octadecenamide | -5.4 |
23 | 1,3-Bis(cinnamoyloxymethyl)adamantine | -8.4 |
24 | 5,8,11,14-Eicosatetraenoic acid, ethyl ester | -6.7 |
25 | Cholesta-3,5-diene | -7.9 |
26 | 9-Octadecenamide | -4.8 |
27 | 1,37-Octatriacontadien | -5.1 |
28 | Cholesterol | -8.0 |
29 | 8-Nonenoic acid, 9-(1,3-nonadienyloxy)-, methyl ester | -5.6 |
30 | Z-11-Tetradecen-1-ol trifluoroacetate | -7.2 |
31 | Betulinaldehyde | -8.0 |
32 | Palmitic acid vinyl ester | -5.0 |
33 | 1-Pentatriacontanol | -5.2 |
34 | Tetrahydrosarsasapogenin | -8.1 |
Autodock Vina based screening results of the binding of the constituents of non-protein fraction of the sericin extract from Antheraea proylei J. with tyrosinase protein.
The Autodock Vina-based screening results for the compounds against the tyrosinase enzyme showed that the compound 1,3-Bis(cinnamoyloxymethyl)adamantine showed the best binding affinity for the active site of tyrosinase (Table 1). This compound was subjected to Autodock 4.0 analysis. 1,3-Bis(cinnamoyloxymethyl)adamantine showed a binding energy of -7.18 kcal/mol (Table 2) and hydrogen bonding with THR268 amino acid residue (Figure 2a).
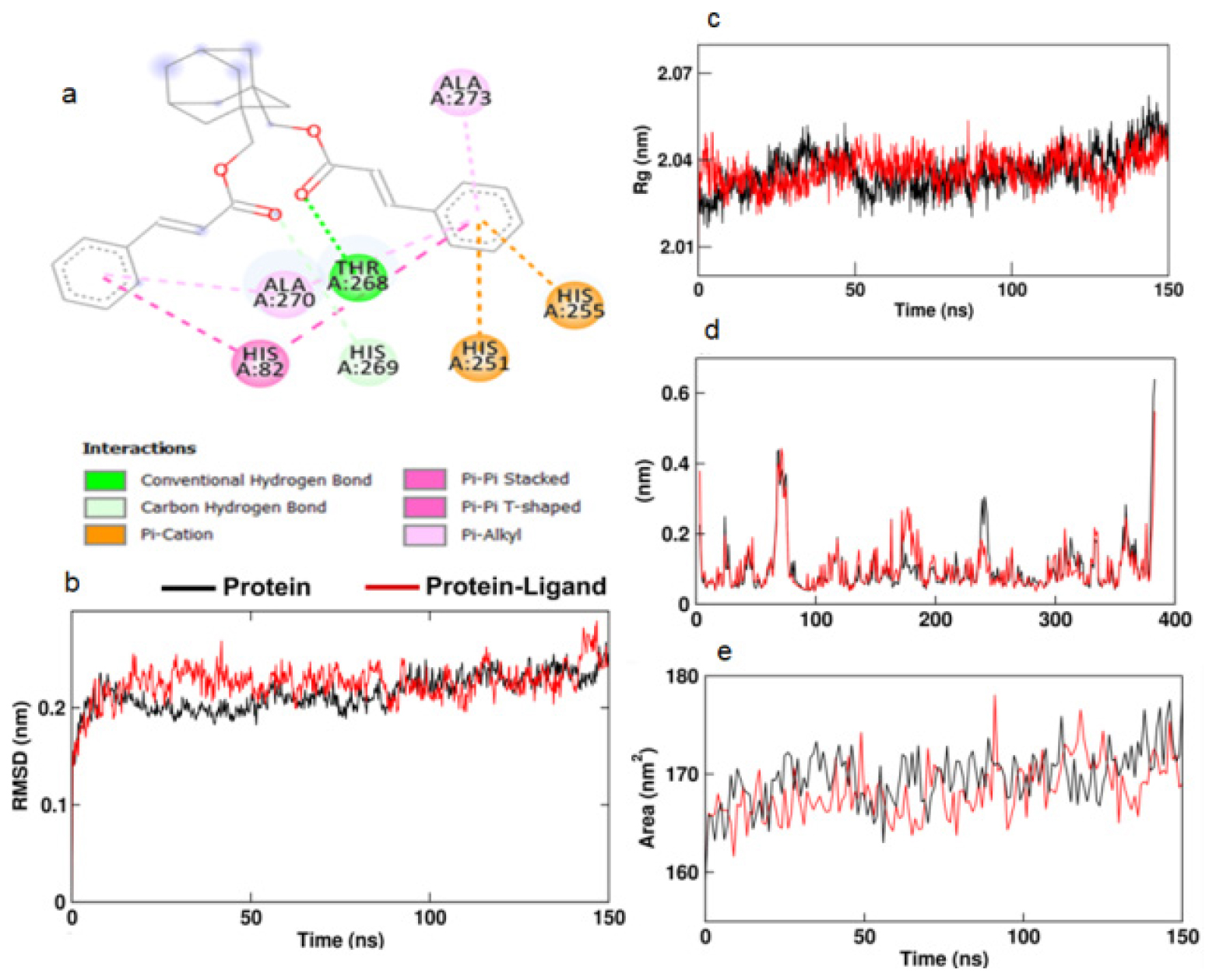
Figure 2:
a. 2D image of the docking of Bis(cinnamoyloxymethyl)adamantine with tyrosinase enzyme. b. RMSD, c. Rg, d. RMSF and e. SASA of the tyrosinase protein (black) and the complex of Bis(cinnamoyloxymethyl) adamantine-tyrosinase complex (red).
Compound | Binding energy (kcal/mol) | Inhibition constant (μM) | Ligand efficiency | Number of Hydrogen bonds |
---|---|---|---|---|
1,3-Bis(cinnamoyloxymethyl)adamantine | -7.18 | 5.42 | -0.27 | 1 |
Binding energy of 1,3-Bis(cinnamoyloxymethyl)adamantine against tyrosinase.
Molecular dynamics simulation of the 1,3-Bis(cinnamoyloxymethyl)adamantine and tyrosinase complex showed an increase in the Root Mean Square Deviation (RMSD) value compared to the protein structure alone (Figure 2b). The Radius of gyration (Rg) stayed steady for tyrosinase both in the presence and absence of the ligand (Figure 2c). The Root Mean Square Fluctuation (RMSF) study of the amino acids in the tyrosinase enzyme shows a restriction in the motion of the amino acids between positions 75 to 85 and 250 to 270. The RMSF values (Figure 2d) correspond with the 2D image (Figure 2a) of the interaction between the ligand and HIS82 and the amino acids between positions 250 to 273 of the protein. Solvent Accessible Surface Area (SASA) of the protein alone and in the presence of the ligand didn’t show any difference (Figure 2e). The number of hydrogen bonds between the protein and ligand stayed at a maximum of 2 (Figure 3a). The total number of pairs of hydrogen bonds in the entire structure has significantly reduced in the protein in the presence of ligand (Figure 3b).
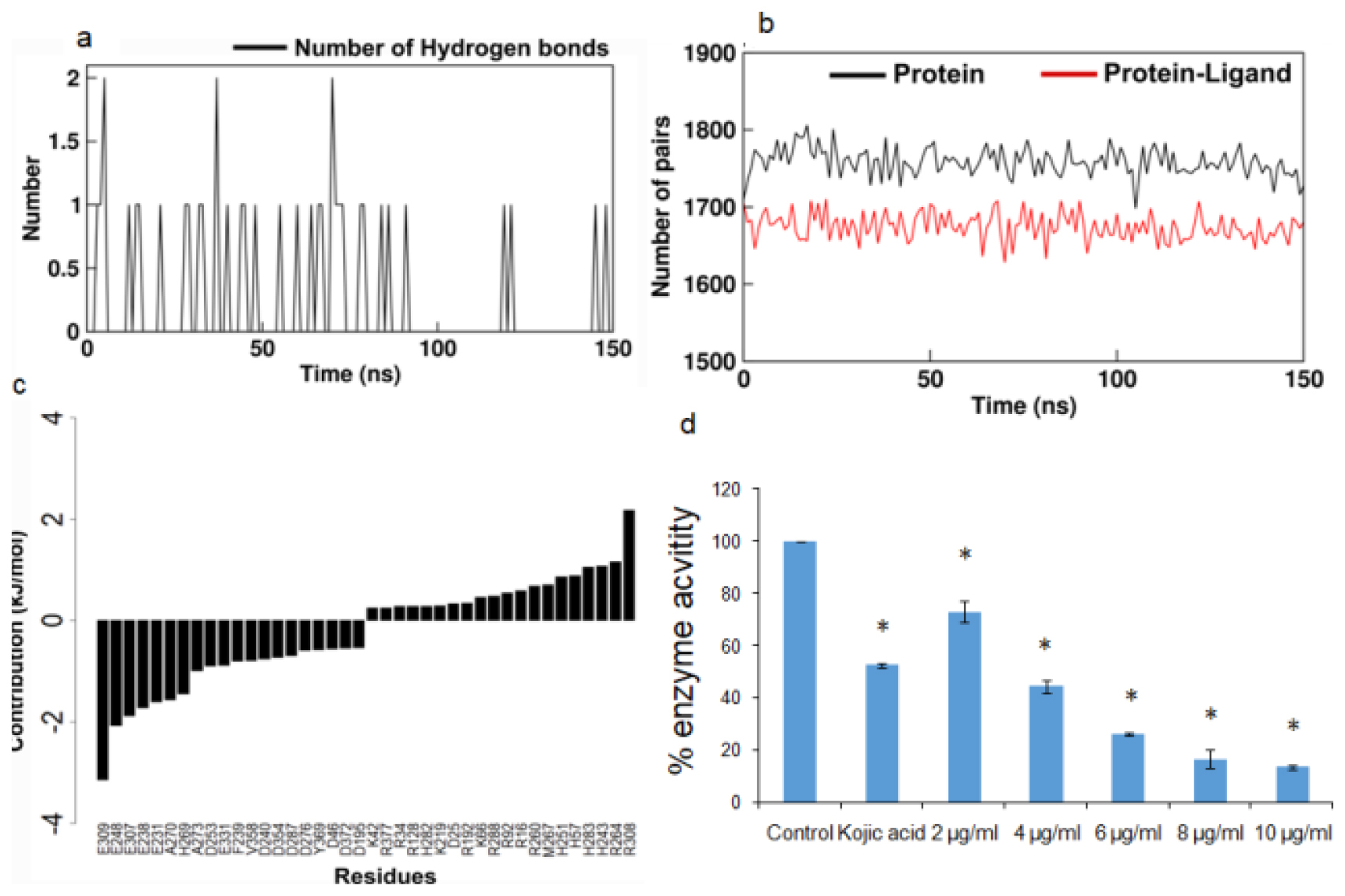
Figure 3:
a. Number of hydrogen bonds between Bis(cinnamoyloxymethyl)adamantine and tyrosinase. b. Total number of hydrogen bonding pairs in the tyrosinase structure calculated in presence (red) and absence (black) of Bis(cinnamoyloxymethyl)adamantine. c. Residue wise decomposition of free energy of twenty residues each for contributing most and least to protein-ligand interactions. d. Bar graph showing the % enzyme activity of tyrosinase under exposure to kojic acid and varying concentrations of ethanolic extract of the non-sericin component. Values are expressed as mean±standard deviation. *p<0.01 of the respective treated groups versus control.
The Molecular Mechanics/Poisson-Boltzmann Surface Area (MM/PBSA) analysis reveals that van der Waals non-bonded interactions have contributed most to the ligand-protein interactions (Table 3). From Figure 3c we observed that the most dominant contributions are residues GLU309, GLU248, GLU307 and the residues in the positions of 75 to 85 and 250 to 270.
Energy components | Energy (kJ/mol) |
---|---|
van der Waal energy (ΔEvdw) | -78.370±64.666 kJ/mol |
Electrostatic energy (ΔEvdw) | -20.103±27.626 |
Polar solvation energy (ΔGsol) | 49.750± 67.177 |
SASA energy (ΔGSASA) | -9.691±7.989 |
Binding energy (ΔGbinding) | -58.415±43.331 |
Binding free energy and the contribution of energy components (kJ/mol) for the protein-ligand complex.
The ethanolic extract also caused a gradual reduction in the tyrosinase activity with increasing doses of the extract (Figure 3d). The extract showedin vitro antityrosinase activity with an IC50 value of 3.808±0.920 μg/mL (Table 4).
Sample | IC50 (μg/mL) |
---|---|
Kojic acid | 0.006±0.002 |
Ethanolic extract | 3.808±0.920 |
IC50values for tyrosinase inhibition.
DISCUSSION
The identity and composition of these secondary metabolites found associated with the cocoon shell depend on the nature of the leaf of the food plant that the silkworm ingests.15 Hence different types of silk cocoon shells exhibited different metabolite composition. These metabolites are derived in the body of the silkworm. Hence the cocoon shell contained metabolites not present in the food plants which could be a source of finding novel bioactive agents.16
The non-protein fraction from the cocoon shell of Antheraea proylei J. was found to exhibit in vitro antityrosinase activity (IC50=3.808±0.920 μg/mL) (Table 4). An attempt to identify metabolites contributing to in vitro antityrosinase activity was made through Autodock Vina screening. Out of all the metabolites, the compound 1,3-Bis(cinnamoyloxymethyl) adamantine was found to exhibit the best binding affinity for the active site of the enzyme tyrosinase (Table 1). 1,3-Bis(cinnamoyloxymethyl)adamantine formed bonds with conserved residues like HIS57 and HIS255.17 Molecular dynamics simulation of the 1,3-Bis(cinnamoyloxymethyl)adamantine and tyrosinase complex showed an increase in the Root Mean Square Deviation (RMSD) value compared to the protein structure alone (Figure 2b). The Radius of gyration (Rg) stayed steady for tyrosinase both in the presence and absence of the ligand (Figure 2c). The increase in the RMSD value indicates the increased interaction between the protein and the ligand. Rg values showed the overall compactness of the structure.18 The lowering of RMSF values of the active site amino acids (Figure 2a and 2d) in proteins corresponded with the decreased protein activity.19 The total number of pairs of hydrogen bonds in the entire structure has significantly reduced in the protein in the presence of ligand (Figure 3a and 3b) which shows reduced structural stability of the protein induced by the presence of ligand.20 The in vitro results lend further credence to our in silico analysis of the potential of the non-protein component of silkworm extract to act as a tyrosinase inhibitor.
CONCLUSION
Our results demonstrated that the ethanolic extract of the non-protein component of the sericin extract was composed of several metabolites. Of those components, 1,3-Bis(cinnamoyloxymethyl)adamantine showed the best binding with the conserved amino acids of the tyrosinase active site. The stability of the binding was further demonstrated by the molecular dynamics simulation studies. In vitro inhibition of the activities of the tyrosinase enzyme by the ethanolic extracts of the non-sericin component showed further validation of the in silico studies.
Cite this article:
Chanu SB, Singh LR, Devi SK, Yaiphabi S, Ingti B, Baruah TJ. In silico and in vitro Antityrosinase Activity of the Non-Protein Fraction from the Sericin Extract of Antheraea proylei J. Int. J. Pharm. Investigation. 2024;14(3):851-6.
ACKNOWLEDGEMENT
The authors would like to acknowledge the support provided by the Department of Biochemistry, Manipur University, Central Instrument Facility, Jawaharlal Nehru University, Delhi and Guwahati Biotechnology Park.
ABBREVIATIONS
GC-MS | Gas Chromatography-Mass Spectrometry |
---|---|
L-DOPA | 3,4-dihydroxy-L-phenylalanine |
PDBQT | Protein Data Bank, Partial Charge (Q) and Atom Type (T) |
CHARMM36 | Chemistry at Harvard Macromolecular Mechanics |
GROMACS | Groningen MAchine for Chemical Simulations |
MM/PBSA | Molecular Mechanics/Poisson-Boltzmann Surface Area |
VMD | Visual Molecular Dynamics |
RMSD | Root mean square deviation |
RMSF | Root mean square fluctuation |
SASA | Solvent accessible surface area |
min | Minutes |
References
- Hou J, Vázquez-González M, Fadeev M, Liu X, Lavi R, Willner I, et al. Catalyzed and electrocatalyzed oxidation of L-tyrosine and L-phenylalanine to dopachrome by nanozymes. Nano Lett. 2018;18(6):4015-22. [PubMed] | [CrossRef] | [Google Scholar]
- Lambert MW, Maddukuri S, Karanfilian KM, Elias ML, Lambert WC. The physiology of melanin deposition in health and disease. Clin Dermatol. 2019;37(5):402-17. [PubMed] | [CrossRef] | [Google Scholar]
- Cho K, Ryu CS, Jeong S, Kim Y. Potential adverse effect of tyrosinase inhibitors on teleosts: a review. Comp Biochem Physiol C Toxicol Pharmacol. 2020;228:108655 [PubMed] | [CrossRef] | [Google Scholar]
- Kunz RI, Brancalhão RM, Ribeiro LF, Natali MR, Res BM. Int. [PubMed] | [CrossRef] | [Google Scholar]
- Wang X, Tang J, Huang J, Hui M. Production and characterization of bacterial cellulose membranes with hyaluronic acid and silk sericin. Colloids Surf B Biointerfaces. 2020;195:111273 [PubMed] | [CrossRef] | [Google Scholar]
- Wang HY, Wang YJ, Zhou LX, Zhu L, Zhang YQ. Isolation and bioactivities of a non-sericin component from cocoon shell silk sericin of the silkworm
. Food Funct. 2012;3(2):150-8. [PubMed] | [CrossRef] | [Google Scholar] - Choudhary DC, Shekhawat JKS, Kataria K. GC-MS analysis of bioactive phytochemicals in methanol extract of aerial part and callus of decne. Pharmacogn J. 2019;11(5):1055-63. [CrossRef] | [Google Scholar]
- O’Boyle NM, Banck M, James CA, Morley C, Vandermeersch T, Hutchison GR, et al. Open Babel: an open chemical toolbox. J Cheminform. 2011;3(1):33 [PubMed] | [CrossRef] | [Google Scholar]
- Forli S, Huey R, Pique ME, Sanner MF, Goodsell DS, Olson AJ, et al. Computational proteinligand docking and virtual drug screening with the AutoDock suite. Nat Protoc. 2016;11(5):905-19. [PubMed] | [CrossRef] | [Google Scholar]
- Trott O, Olson AJ. J Comput Chem J Comput Chem. 2010;31:455461 [PubMed] | [CrossRef] | [Google Scholar]
- Vanommeslaeghe K, Hatcher E, Acharya C, Kundu S, Zhong S, Shim J, et al. CHARMM general force field: a force field for drug-like molecules compatible with the CHARMM all-atom additive biological force fields. J Comp Chem. 2010;31(4):671-90. [PubMed] | [CrossRef] | [Google Scholar]
- Abraham MJ, Murtola T, Schulz R, Páll S, Smith JC, Hess B, et al. GROMACS: high performance molecular simulations through multi-level parallelism from laptops to supercomputers. SoftwareX. 2015;1-2:19-25. [CrossRef] | [Google Scholar]
- Kumari R, Kumar R. Open source drug discovery, C; Lynn, A. g_mmpbsa-A GROMACS tool for high-throughput MM-PBSA calculations. J Chem Inf Model. 2014;54(7):1951-62. [CrossRef] | [Google Scholar]
- Kim YJ, Uyama H. Tyrosinase inhibitors from natural and synthetic sources: structure, inhibition mechanism and perspective for the future. Cell Mol Life Sci. 2005;62(15):1707-23. [PubMed] | [CrossRef] | [Google Scholar]
- Neog K, Das A, Unni BG, Ahmed GU, Rajan RK. Studies on secondary metabolites of som ( Kost), a primary host plant of Muga silkworm ( Helfer). Int J Pharm Sci Res. 2011;3(3):1441-7. [PubMed] | [CrossRef] | [Google Scholar]
- Hirayama C, Ono H, Tamura Y, Nakamura M. C-prolinylquercetins from the yellow cocoon shell of the silkworm,
. Phytochemistry. 2006;67(6):579-83. [PubMed] | [CrossRef] | [Google Scholar] - Mauracher SG, Molitor C, Al-Oweini R, Kortz U, Rompel A. Latent and active abPPO4 mushroom tyrosinase cocrystallized with hexatungstotellurate (VI). Acta Crystallogr D Biol Crystallogr. 2014;70(9):2301-15. [PubMed] | [CrossRef] | [Google Scholar]
- Guterres H, Im W. Improving protein-ligand docking results with high-throughput molecular dynamics simulations. J Chem Inf Model. 2020;60(4):2189-98. [PubMed] | [CrossRef] | [Google Scholar]
- De Vita S, Chini MG, Bifulco G, Lauro G. Insights into the ligand binding to bromodomain-containing protein 9 (BRD9): a guide to the selection of potential binders by computational methods. Molecules. 2021;26(23):7192 [PubMed] | [CrossRef] | [Google Scholar]
- Schiebel J, Gaspari R, Wulsdorf T, Ngo K, Sohn C, Schrader TE, et al. Intriguing role of water in protein-ligand binding studied by neutron crystallography on trypsin complexes. Nat Commun. 2018;9(1):3559 [PubMed] | [CrossRef] | [Google Scholar]