ABSTRACT
Background
The serine/threonine kinase p38 Mitogen-Activated Protein (MAP) kinase is one of the most well studied kinases in the inflammatory process. The goal of this study was to see how a p38 MAP Kinase inhibitor (PDB ID- 1A9U) affected the activity of a p38 MAP kinase implicated in inflammation. “Inflammation is a common feature of age-related neurodegenerative diseases in the Central Nervous System (CNS).” The p38 Mitogen-Activated Protein Kinase (MAPK) pathway regulates the synthesis of IL-1 and TNF.
Materials and Methods
The study focused on molecular docking and ADME of the relationships that exist between [4-amino-3-(1H-indol-1-yl) phenyl] (4-hydroxyphenyl) methanone derivatives and p38 Mitogen-Activated Protein (MAP) kinase. Inhibition of p38 Mitogen-Activated Protein (MAP) kinase pathway, a series of [4-amino- 3-(1 H-indol-1-yl) phenyl] (4-hydroxyphenyl) methanone derivations were developed and evaluated in silico. The designed composites’ medicine-likeness packets were predicted.
Results
According to molecular docking experiments, all composites had improved interactions with the target protein and may be powerful drugs. The developed [4-amino-3-(1H-indol-1-yl) phenyl] (4-hydroxyphenyl) methanone analogues may be more effective and safer anti-inflammatory medicines.
Conclusion
According to the findings of this investigation, p38 MAPK inhibitors alone are a unique therapeutic target for inflammatory disorders. p38 Mitogen-Activated Protein (MAP) Kinase is a critical serine/threonine kinase that has been well studied in the inflammatory process.
INTRODUCTION
The p38 Mitogen-Activated Protein Kinase (MAPK) is a key modulator of inflammation as well as inflammatory and neuropathic pain. Docking-groove dependent interactions are critical for p38 MAPK-mediated signal transduction, as we recently demonstrated. As a result, virtual screening was used to discover potential docking groove-targeted p38 MAPK inhibitors.1–3 Several indole family compounds were found to have low micromolar inhibitory activity in a p38 MAPK activity assay and in THP-1 human monocytes functioning as inhibitors of LPS-induced TNF release.4 Because of their powerful in vivo effects, p38 MAPK docking-site specific inhibitors have been proposed as a potential innovative method for the treatment of inflammatory pain. Inflammation is a chain reaction caused by innate immune responses to microbial infection. Non-infectious triggers, such as cell damage and tissue injury, can also activate these responses and cause local inflammation.5,6 Uncontrolled acute inflammation gradually destroys tissues and organs, impairs their activities, and progresses to chronic inflammation and degenerative disorders including osteoarthritis, rheumatoid arthritis, and even cancer. When exposed to a chemoattractant, tissue monocytes transform into macrophages, which engulf and destroy bacteria in phagolysosomes utilising a variety of hydrolytic enzymes.7 The Mitogen-Activated Protein Kinase (MAPK) p38 is an important signalling protein that regulates the synthesis of numerous inflammatory mediators. As a result, p38 MAPK has been the subject of extensive research in academic institutions as well as numerous pharmaceutical businesses.8,9 p38 belongs to the MAPK family of serine/threonine kinases, which have a similar general structure and manner of activation by phosphorylation at the activation loop by upstream activators such as MKK3 and MKK6 for p38 MAPK. Of the four p38 MAPK isoforms, p38 and are ubiquitously expressed, with p38 being the most prevalent in inflammatory cells. Many extracellular stimuli, including inflammatory cytokines, Lipopolysaccharide (LPS) or other bacterial products, and other stressors like as thermal, mechanical, or hypoxic insults, activate p38 MAPK.10,11 p38 MAPK then phosphorylates key transcription factors such as Myocyte Enhancer Factor 2 (MEF-2) and Activating Transcription Factor 2 (ATF-2). The activation state of downstream kinases such as MAPK-activated protein kinases 2 and 3 (MK2 and 3) that phosphorylate heat shock protein-27 (Hsp-27) and other cellular proteins is similarly controlled by p38 MAPK. A p38-dependent pathway affects the stability and expression of cytokine mRNAs such as TNF, IL-1, IL-6, and IL-8. In the setting of pain, p38 MAPK is activated in the spinal cord in various chronic pain models, and p38 MAPK inhibitors can temporarily limit pain hypersensitivity. These data suggest that p38 MAPK could be a promising target for developing therapeutic medicines to treat chronic inflammatory pain. However, human clinical trials with p38 MAPK inhibitors have so far failed to provide the desired results. The docking domain, which is located opposite the activation loop, allows p38 MAPK to selectively connect with upstream regulators as well as downstream substrates. The exact properties of these interactions have been well characterised, and the structure of p38 co-crystallized with peptides derived from the interacting motifs in MKK3b and MEF-2A has been determined.12 Surprisingly phosphorylation of either the substrate or p38 kinase can modulate the connections mediated by this docking region. This feature provides a plausible avenue for intervention into the p38 MAPK pathway by giving a potentially more selective protein target for therapeutic medicines than the currently most extensively used domain: the ATP binding site. The latter has a high degree of overall similarity with other protein kinases. As a result, the need for creative tactics utilising previously undiscovered interacting domains in p38 is an option that surely merits further investigation. We recently demonstrated that p38 MAPK can be phosphorylated in the docking groove, resulting in reduced binding of upstream kinases and downstream targets and decreased p38 MAPK activity. On this basis, we conducted a simulated screening of a chemical library for potential new p38 MAPK inhibitors targeting the docking groove.
Properties of p38 map kinase members
p38 was discovered as a 38-kDa protein that tyrosine phosphorylated rapidly in response to LPS stimulation, 3. p38 cDNA was also cloned as a molecule that binds indole derivatives, which have been shown to suppress the manufacture of inflammatory cytokines including Interleukin-1 (IL-1) and Tumour Necrosis Factor (TNF) in LPS-stimulated monocytes.13 To date, four p38 splice variants have been identified: p38, p38 5, p38 (ERK6, SAPK3) 6, 7, and p38 (SAPK4) 8. Among these, p38 and p38 are ubiquitously expressed, whereas p38 and p38 are expressed differently depending on tissue type. The serine/ threonine kinase p38 Mitogen-Activated Protein (MAP) kinase is one of the most well studied kinases in the inflammatory process. Among the four discovered p38 isoforms (p38, p38, p38, and p38), the form is the most thoroughly investigated and plays a critical role in the translational and transcriptional levels of the pro-inflammatory cytokines IL-1 and TNF. The inhibition of these pro-inflammatory cytokines has been identified as a promising avenue for the creation of novel therapeutic candidates. The pharmacological profile of the selective p38 inhibitor prototype was characterised, and its disease-modifying potential was demonstrated in an adjuvant-induced arthritis model. This strongly suggests that appropriately modulating the production of these cytokines can have a major impact on the treatment of chronic inflammatory disorders. In addition to its role in pro-inflammatory cytokine production, p38 is engaged in the activation of matrix metalloproteinase and the induction of COX-2 transcription, all of which are implicated in the process of tissue destruction and inflammation. Because of its several responsibilities in controlling the inflammatory response, p38 inhibitors are intended to address the underlying cause of chronic inflammatory illnesses and halt their progression.14 The indole is the quintessential small molecule p38 inhibitor, and these compounds served as the foundation for most of the early research. This article examines the indole heterocycles identified for p38 MAPK inhibition and discusses contemporary research in the creation of anti-inflammatory drugs.15
MATERIALS AND METHODS
Hardware and Software
It was used PC with Intel® CoreTM i9-10900 RAM 64.00 GB @ 2.80 GHz, 64-bit operating system at Windows 10. Also, the software Swiss Target Prediction (STP; www.swisstargetprediction.ch/)
Chem Draw
Professional 17.1, Chem3D 17.1, Discovery Studio® (www.3ds.com/), AutoDockTools® (http://autodock.scripps.edu/resources/adt) 22, and AutoDock Vina23.
Ligands
The reference drugs were set of Drug Bank Online (https:// go.drugbank.com/); minocycline (DB01017), dasatinib (DB01254), fostamatinib (DB12010) 24, and SPs were designed using Chem Draw 17.1.
Receptors
MAPK14 (p38) bound to SR348 was employed as the molecular target, and it was downloaded from the protein data bank (https:// www.rcsb.org/) as PDB code 6ZWP25.
In silico ADME (Absorption, Distribution, Metabolism and Excretion Studies)
The pharmacokinetics of the motes inside an organism’s body is described by ADME or IDME. The ADME assesses the danger of administering a pharmaceutical emulsion to a mortal body or other living things. By means of an online tool comparable to, new derivations of indole pharmacokinetic packages are linked in silico Swiss ADME (http//www.swissadme.ch/). We study Lipinski’s rule. According to the Lipinski’s rule of 5, the two or further violation makes the motes orally inactive. It includes hydrophobicity, electronic distribution, and the presence of several pharmacophore features.
Molecular docking Study
To prognosticate the list commerce of designed indole derivations molecular docking was carried out with the targeted protein. The p38 Mitogen-Activated Protein (MAP) kinase is the targeted protein. We study molecular docking with software i.e. Auto dock Vina software. The protein is downloaded from PDB and the unwanted tittles like hetero tittles unwanted chains, cofactors and water motes are removed and also protein get ready for commerce. The designed indole derivations are optimized by using 3 D software and 2 D software. The designed indole nucleus derivations are given below Figure 1.
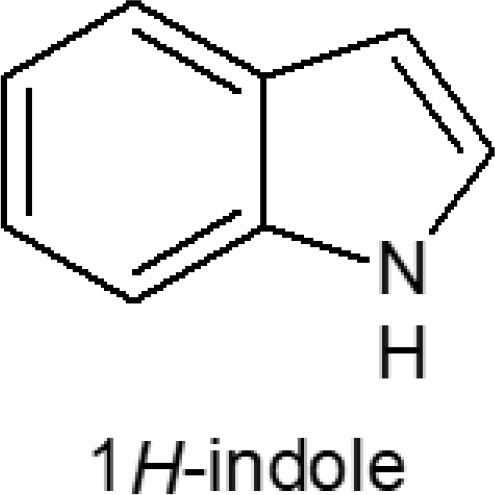
Figure 1:
Designed indole nucleus.
RESULTS AND DISCUSSION
Chemistry
The synthetic protocol to obtain [4-amino-3-(1H-indol-1-yl) phenyl] (4-hydroxyphenyl) methanone is outlined in Scheme 1 (Figure 2). [4-amino-3-(1H-indol-1-yl) phenyl] (4-hydroxyphenyl) methanone was prepared by refluxing 4-chloro-2-(1H-indol-1-yl) aniline and 4-hydroxy benzaldehyde in ethanol. 4-chloro-2-(1H-indol-1-yl) aniline was prepared by refluxing 2,5-dichloroaniline and 1H-indole. The 1 H NMR spectra of [4-amino-3-(1H-indol-1-yl) phenyl] (4-hydroxyphenyl) methanone displayed broad singlet signals corresponding to D2O exchangeable NH2 protons at δ 10.98 ppm. Intermolecular cyclization of [4-amino-3-(1H-indol-1-yl) phenyl] (4-hydroxyphenyl) methanone with substituted reagents acids (S3a–S3h) in presence of catalyst and various solvents.
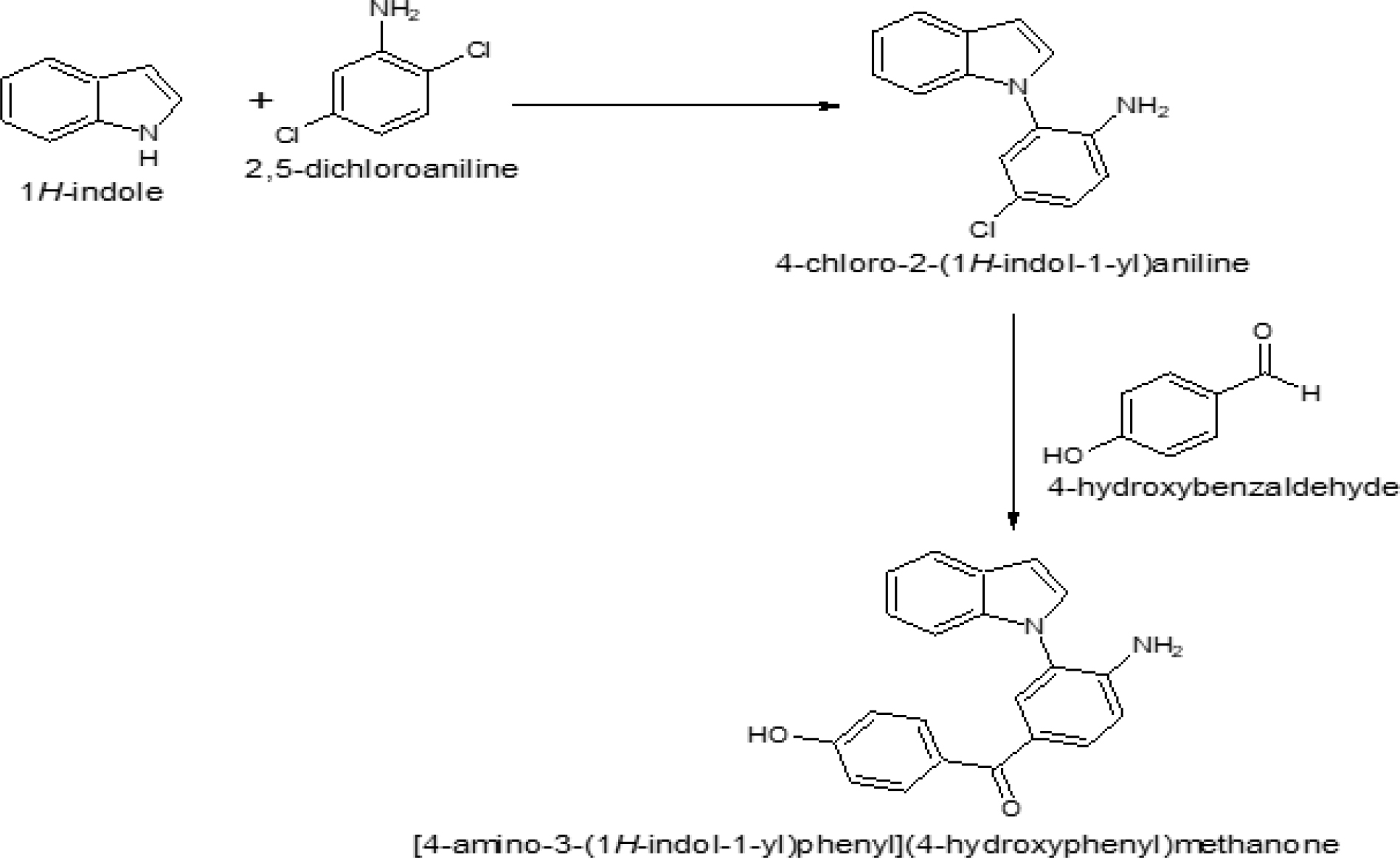
Figure 2:
Synthetic scheme of [4-amino-3-(1H-indol-1-yl) phenyl] (4-hydroxyphenyl) methanone.
Drug likeness analysis
The 4-amino-3-(1H-indol-1-yl) phenyl] (4-hydroxyphenyl) methanone, which was listed in Table 1 for its anti-inflammatory efficacy for the new derivatives. Drugs or chemicals with high biological activity at low concentrations and low toxicity or adverse effects generally qualify as successful new medications. Numerous reports on the use of the heterocyclic 4-amino- 3-(1H-indol-1-yl) phenyl] (4-hydroxyphenyl) methanone nucleus for treating bacterial and fungal infections are available. For its anti-fungal efficacy, new compounds of 4-amino-3- (1H-indol-1-yl) phenyl] (4-hydroxyphenyl) methanone are being developed. It serves as an inhibitor of ergo sterol production. In Figure 2, all the proposed compounds are listed. The proposed chemical was discovered to exhibit ergo sterol biosynthesis inhibitor efficacy with a low risk of adverse medication reactions.
Label | Structure | Label | Structure |
---|---|---|---|
S3a | S3e | ||
S3b | S3f | ||
S3c | S3g | ||
S3d | S3h |
Derivatives of designed compound of indole.
Absorption, Distribution, Metabolism, Excretion, and Toxicity (ADMET)
4-amino-3-(1H-indol-1-yl) phenyl] (4-hydroxyphenyl) methanone molecules, the proposed compounds, all have oral activity. Tables 1 and 2 present the findings of ADME research. Based on research, we projected that the molecules would have a good oral bioavailability since the proposed compounds adhere to the rule of five and have an octanol-water partition coefficient (mol log P) that is not larger than 5 (Table 2). The expression for the water solubility is the logarithm of the molar concentration. The normal water solubility range for proposed compounds is between -5.00 and -6.00, and it is anticipated that the molecules will have a high oral bioavailability (Table 3). The calculation of the derivatives’ percent absorption revealed that, due to the similarity between intestinal epithelial cells and Caco2 permeability (log Papp in 10-6 cm/s) cells from the human colon, the majority of an oral medication’s absorption happens through the gastrointestinal system. The complete proposed derivative demonstrated significant Caco-2 permeability and intestinal absorption (Table 3). The blood-brain barrier permeability, % unbound, and Volume of Distribution (VD) were used to forecast how the chemicals will be distributed throughout the body. A higher VD value indicates that the tissues are receiving the medication more effectively than the plasma. In tissues, all of the chemicals exhibit a moderate distribution. According to the plasma protein binding model, a drug will firmly bind to blood carrier proteins. The percentage PPB for compounds containing 4-amino-3-(1H-indol-1-yl) phenyl] (4-hydroxyphenyl) methanone ranges from 93 to 100%. The developed compound gives a high possibility that these compounds can easily reach the intended targets as a result of ADMT and Drug likeness study. We determined the blood-brain barrier’s (BBB) permeability using Swiss ADME and pre ADMET technologies. All 4-amino- 3-(1H-indol-1-yl) phenyl] (4-hydroxyphenyl) methanone derivatives engage in substrate- or inhibitor-mediated interactions with cytochrome. All of the proposed substances have favourable ADME and toxicity characteristics and can be regarded as potential lead molecules.
Comp. | Molecular weight (g/ mol) | CMC rule violation | Lipinski’s rule violation | Mol Log P | H bond donor | H bond acceptor | No. of rotatable bonds | TPSA (Å2) |
---|---|---|---|---|---|---|---|---|
S3a | 448.47 g/mol | 3 | Yes | 2.94 | 2 | 3 | 6 | 105.87 Å2 |
S3b | 448.47 g/mol | 3 | Yes | 2.94 | 2 | 3 | 6 | 105.87 Å2 |
S3c | 418.49 g/mol | 3 | Yes | 3.83 | 1 | 2 | 6 | 57.25 Å2 |
S3d | 370.40 g/mol | 3 | Yes | 2.93 | 1 | 3 | 5 | 74.32 Å2 |
S3e | 356.37 g/mol | 3 | Yes | 2.71 | 2 | 3 | 4 | 85.32 Å2 |
S3f | 403.48 g/mol | 3 | Yes | 3.90 | 2 | 1 | 5 | 60.05 Å2 |
S3g | 448.47 g/mol | 3 | Yes | 2.94 | 2 | 3 | 6 | 105.87 Å2 |
S3h | 432.47 g/mol | 3 | Yes | 3.75 | 1 | 3 | 6 | 74.32 Å2 |
Drug likeness analysis of designed 4-amino-3-(1H-indol-1-yl) phenyl] (4-hydroxyphenyl) methanone with Lipinski’s rule.
P38α MAPK assay results
All of the synthesised substances S3a to S3h were tested for inhibition of p38 MAPK mediated ATF-2 phosphorylation using an enzyme assay. In comparison to the standard chemical, the compounds demonstrated activity ranging from 80 to 95 PPB% (Table 3). The compounds S3h and S3f, which contained benzoyl chloride and acetyl chloride linked to position 4 of the phenyl ring of indole nucleus derivatives, had the highest enzyme inhibitory activity (94-95%). Substitution of their indole ring in the fourth position with alcohol, aniline, ether, and ester (S3a, S3c, S3b, S3d) resulted in a modest decrease in activity (83 and 90%, respectively).
Comp. | Absorption | Distribution | Metabolism | |||||||
---|---|---|---|---|---|---|---|---|---|---|
Caco2 permeability (log Papp in 10-6 cm/s) | GI absorption | BBB perm. (logBB) | BBB Per meant | PPB (%) | CYP3A4 substrate | CYP1A2 inhibitor | CYP2C9 inhibitor | CYP3A4 inhibitor | CYP2C19 inhibitor | |
S3a | 22.2815 | Low | 1.49973 | No | 91.54 | Non | No | No | Yes | Yes |
S3b | 22.2815 | Low | 1.49973 | No | 91.88 | Non | No | No | No | Yes |
S3c | 22.2815 | High | 1.49973 | No | 91.84 | Non | Yes | No | Yes | Yes |
S3d | 22.2815 | High | 1.49973 | Yes | 92.24 | Non | Yes | Yes | Yes | Yes |
S3e | 22.2815 | High | 1.49973 | No | 90.52 | Non | No | Yes | No | Yes |
S3f | 35.1695 | High | 4.28882 | No | 95.44 | Weakly | Yes | No | Yes | Yes |
S3g | 22.2815 | High | 1.49973 | No | 91.68 | Non | Yes | Yes | Yes | Yes |
S3h | 22.2815 | High | 1.49973 | No | 94.25 | Non | No | Yes | No | Yes |
In silico ADMET (Absorption, Distribution, Metabolism, Excretion, and Toxicity) properties of 4-amino-3-(1H-indol-1-yl) phenyl] (4-hydroxyphenyl) methanone.
Biological evaluation
Synthesized newer Indole derivatives were screened for Anti-inflammatory activity. Total 8 compounds were evaluated for their biological screening. The following section describes, in brief the Anti-inflammatory activity.
Anti-inflammatory activity
The carrageenan-induced rat paw oedema paradigm was used to assess the anti-inflammatory efficacy of all synthesised Indole derivatives. Wistar rats (100-200 g) were randomly assigned to one of three groups: control, test, or standard.16,17 Each group is made up of six creatures. During the experiment, animals were fasted overnight and only distilled water was given to them. Indomethacin was commonly used as a standard medicine. Both the test and control medications were suspended in 1% Carboxymethyl Cellulose (CMC) and given orally via Gavage needle. In the control group, 1% Carboxymethyl Cellulose (CMC) was supplied.18 We induced the carrageen a (1%) by the sub planner surface of the rats’ right hind paws 1 hr after administering the substance. % paw oedema inhibition was calculated for Indole derivatives. The anti-inflammatory activity of indole derivatives is shown in below Table 4.
Code | Dose Mg/Kg | Inhibition of paw oedema after 3 h (%) | Inhibition of paw oedema after 6 h (%) |
---|---|---|---|
S3a | 30 mg/Kg | 6.30±0.28 | 88.24 |
S3b | 30 mg/Kg | 2.48±0.23 | 68.48 |
S3c | 30 mg/Kg | 4.46±0.22 | 51.16 |
S3d | 30 mg/Kg | 6.69±0.27 | 79.98 |
S3e | 30 mg/Kg | 6.26±0.241 | 69.48 |
S3f | 30 mg/Kg | 3.22±0.281 | 53.98 |
S3g | 30 mg/Kg | 6.52±0.271 | 79.54 |
S3h | 30 mg/Kg | 7.69±0.27 | 79.98 |
Control | – | 0 .36±0.28 | |
Indomethacin | 40 mg/Kg | 2.78±0.340 | 67.44 |
Anti-inflammatory activities of compounds MD1 to MD8 by carrageenan-induced rat paw oedema model.
Molecular docking results
A fascinating method for predicting the major list mode of a ligand with a target protein with a known three-dimensional structure is molecular docking. It’s a crucial tool in the design of structure-based, computer-supported medicine. The intended 4-amino-3-(1H-indol-1-yl) phenyl] (4-hydroxyphenyl) methanone derivations bind successfully with the target protein’s active site p38 Mitogen-Activated Protein Kinase (MAPK) by using auto dock software. The designed derivatives like S3h and S3f shows good list via hydrophobic and hydrogen bonds, respectively, to the target protein, whereas S3a, S3c, S3b, S3d shows hydrophobic interaction.
In this exploration; all composites are active and S3h, S3b and S3f is the most active emulsion with minimal list affinity are named as potent impediments. Hydrophobic commerce of S3a, S3c, S3d with LEU168; LEU168; PHE142; LEU168; LEU138; MET175; PHE142; PHE172; LEU168; LEU138 a distinct group. The hydrogen bond conformation between the motes PHE and LEU is another factor is completely honored as indicated which have observed Tables 2 and 3. Docking investigations showed that the planned emulsion and target protein were the list mode of the most active composites. 2 D and 3 D Structure of designed indole derivations of S3b, S3f and S3h are given below (Table 5).
2D-docking poses | 3D-docking poses |
---|---|
Compound: S3b | |
S3f | |
S3h |
2 D and 3 D Structure of designed indole derivatives.
CONCLUSION
The 4-amino-3-(1H-indol-1-yl) phenyl] (4-hydroxyphenyl) methanone derivatives were designed and it’s in silico parameter was studied. According to docking score, Drug likeness analysis of derivatives of designed compound of 4-amino-3- (1H-indol-1-yl) phenyl] (4-hydroxyphenyl) methanone and ADME studies the designed mixes can be considered as super eminent molecules. Among the derivatives, S3h, S3f and S3b show the most potent asset according to a molecular docking study. They interact with LEU168; LEU168; PHE142; LEU168; LEU138; MET175; PHE142; PHE172; LEU168; LEU138 to form hydrophobic commerce and with PHE and MET form hydrogen cleave. These mixes pass the ADME test, indicating that they are eligible for drug-likeness. These compounds have strong intestinal and PPB absorption capacities.
Cite this article:
Bhor RJ, Agale AC, Sitaram GM, Navnath MD, Sunil WS. Docking Sites of Indole Derivative on Mitogen-Activated Protein Kinase (MAPK) Inhibitor (PDB ID- 1A9U) Against Inflammation. Int. J. Pharm. Investigation. 2024;14(1):172-9.
ACKNOWLEDGEMENT
The authors are thankful to Dr. S.B. Bhawar, Pravara Rural College of Pharmacy, Pravaranagar.
ABBREVIATIONS
mg/kg | Milligram/kilograms |
---|---|
sec | Seconds kcal kilocalorie |
Mol.Wt | Molecular Weight |
gm | Gram |
LEU | Leucine |
THR | Threonine |
ALA | Alanine |
MET | Methionine |
PHE | Phenylalanine |
MAPK | Mitogen-activated protein kinase |
WHO | World health association |
Log P | Partition coefficient |
References
- Bachstetter AD, Van Eldik LJV. The p38 MAP kinase family as regulators of proinflammatory cytokine production in degenerative diseases of the CNS. Aging Dis. 2010;1(3):199-211. [PubMed] | [Google Scholar]
- Cuadrado A, Nebreda AR. Mechanisms and functions of p38 MAPK signalling. Biochem J. 2010;429(3):403-17. [PubMed] | [CrossRef] | [Google Scholar]
- Rathod R, Khaire A, Kale A, Joshi S. A combined supplementation of vitamin B12 and n-3 polyunsaturated fatty acids across two generations improves nerve growth factor and vascular endothelial growth factor levels in the rat hippocampus. Neuroscience. 2016;339:376-84. [PubMed] | [CrossRef] | [Google Scholar]
- Field MS, Kamynina E, Chon J, Stover PJ. Nuclear folate metabolism. Annu Rev Nutr. 2018;38(38):219-43. [PubMed] | [CrossRef] | [Google Scholar]
- Gröber U, Kisters K. Schmidt J. Neuroenhancement with vitamin B12 underestimated neurological significance. Nutrients. 2013;5(12):5031-45. [PubMed] | [CrossRef] | [Google Scholar]
- Hobbenaghi R, Javanbakht J, Hosseini E, Mohammadi S, Rajabian M, Moayeri P, et al. Neuropathological and neuroprotective features of vitamin B12 on the dorsal spinal ganglion of rats after the experimental crush of sciatic nerve: an experimental study. Diagn Pathol. 2016;11:123 [PubMed] | [CrossRef] | [Google Scholar]
- Chan W, Almasieh M, Catrinescu MM, Levin LA. Cobalamin-associated superoxide scavenging in neuronal cells is a potential mechanism for vitamin B12–deprivation optic neuropathy. Am J Pathol. 2018;188(1):160-72. [PubMed] | [CrossRef] | [Google Scholar]
- Horiuchi K, Amizuka N, Takeshita S, Takamatsu H, Katsuura M, Ozawa H, et al. Identification and characterization of a novel protein, periostin, with restricted expression to periosteum and periodontal ligament and increased expression by transforming growth factor β. J Bone Miner Res. 1999;14(7):1239-49. [PubMed] | [CrossRef] | [Google Scholar]
- Boehm JC, Smietana JM, Sorenson ME, Garigipati RS, Gallagher TF, Sheldrake PL, et al. 1-substituted 4-aryl-5-pyridinylimidazoles: A new class of cytokine suppressive drugs with low 5-lipoxygenase and cyclooxygenase inhibitory potency. J Med Chem. 1996;39(20):3929-37. [PubMed] | [CrossRef] | [Google Scholar]
- Ben-Levy R, Hooper S, Wilson R, Paterson HF, Marshall CJ. Nuclear export of the stress-activated protein kinase p38 mediated by its substrate MAPKAP kinase-2. Curr Biol. 1998;8(19):1049-57. [PubMed] | [CrossRef] | [Google Scholar]
- Lee JC, Young PR. Role of CSBP/p38/RK stress response kinase in LPS and cytokine signaling mechanisms. J Leukoc Biol. 1996;59(2):152-7. [PubMed] | [CrossRef] | [Google Scholar]
- Cuadrado A, Nebreda AR. Mechanisms and functions of p38 MAPK signalling. Biochem J. 2010;429(3):403-17. [PubMed] | [CrossRef] | [Google Scholar]
- Ono K, Han J. The p38 signal transduction pathway activation and function. Cell Signal. 2000;12(1):1-13. [PubMed] | [CrossRef] | [Google Scholar]
- Kumar S, Boehm J, Lee JC. p38 MAP kinases: key signalling molecules as therapeutic targets for inflammatory diseases. Nat Rev Drug Discov. 2003;2(9):717-26. [PubMed] | [CrossRef] | [Google Scholar]
- Ramesh G. Novel therapeutic targets in neuroinflammation and neuropathic pain. Inflam Cell Signal. 2014;1(3) [PubMed] | [CrossRef] | [Google Scholar]
- Streit WJ, Mrak RE, Griffin WS. Microglia and neuroinflammation: A pathological perspective. J Neuroinflammation. 2004;1(1):14 [PubMed] | [CrossRef] | [Google Scholar]
- Chao CC, Hu S, Peterson PK. Glia, cytokines and neurotoxicity. Crit Rev Neurobiol. 1995;9(2-3):189-205. [PubMed] | [Google Scholar]
- Skaper SD. The brain as a target for inflammatory processes and neuroprotective strategies. Ann N Y Acad Sci. 2007;1122(1):23-34. [PubMed] | [CrossRef] | [Google Scholar]