ABSTRACT
Background
Anti-retroviral drugs are challenging formulation scientists due to their poor solubility problem. This criticality increases when the drug has to be delivered by a vaginal route where the availability of fluid to solubilize the drug is less.
Purpose
The Darunavir Ethanolate nano gel was prepared to aim to increase its solubility and improve the dissolution profile to be delivered in the vaginal route for prophylaxis treatment of HIV.
Materials and Methods
Phase solubility study of Darunavir Ethanolate: Hydroxypropyl-β-cyclodextrin was performed (simulated vaginal fluid). Inclusion complex formation was confirmed by FTIR studies. Nanogel was synthesized using the emulsion solvent evaporation method and evaluated for particle size, polydispersity index, zeta potential and entrapment efficiency. Solubility enhancement study of optimized batch in simulated vaginal fluid at different pH conditions was compared with pure drug.
Results
The phase solubility diagram showed the AL type of curve indicating 1:1 complex formation with a stability constant of 618.071 M-1. The formulated nanogel batch F8 with particle size 338.8 nm, PDI; 0.344, zeta potential; -40.3 mV and % entrapment efficiency; 93.52 was selected as the optimized batch. Nanogel showed a 40.9753, 56.9891 and 100.91-fold increase in drug solubility compared to the pure drug in simulated vaginal fluid at pH 3.5, 4.5 and 5.5 respectively. (%) Cumulative drug release of 83% at 22 hr, drug flux at 0.4421 µg/cm2/hr was observed. The best-fit model was the Korsmeyer-Peppas model followed by the Higuchi and the Zero-order models.
Conclusion
The formulated nano gel showed increasing solubility and dissolution profile of Darunavir Ethanolate to be administered in the vaginal cavity for prophylaxis treatment of HIV.
INTRODUCTION
As an effort to fight against HIV infection, microbicides are considered a valuable means to combat HIV at its early entry stage. Microbicides are substances that are given inside to the vagina or rectum to guard against HIV and other STDs. Topical microbicides are products that target viral or cellular targets in order to stop the virus from infecting target cells or from replicating, therefore reducing the spread of the virus. Acquisition of HIV (Cutler and Justman, 2008). Clinical trials study with Tenofovir vaginal gel, showing some promising results, new topical products are under development, including vaginal rings, vaginal and rectal gels and films (Coelhoet al., 2019). In 2021, the WHO recommended a monthly Dapivirine intravaginal ring for HIV prevention in cis-gender women, based on the results of two, phase-III randomized placebo-controlled clinical trials showing a 27% and 35% relative reduction in HIV incidence (Nelet al., 2016; Baetenet al., 2016). For successful microbicides requires a more careful selection of promising microbicides candidates and their formulations in early development stage. In regards to specific microbicides candidates (nucleoside reverse transcriptase inhibitors, nucleotide reverse transcriptase inhibitors, non-nucleoside reverse transcriptase inhibitors, protease inhibitors, entry and fusion inhibitors, integrase inhibitors) apart from their characterization concerning their intrinsic antiviral activity and cervicovaginal toxicity, biopharmaceutical properties of microbicides candidates i.e. solubility and mucosal penetration, should also be given importance, as the in vivo efficiency of potential microbicides will not only depend on its intrinsic anti-HIV activity but also on its biopharmaceutical availability, i.e. the ability to reach the primary target cells for HIV infection upon vaginal application (Garget al., 2003). One of the factors that affect microbicide availability after application in the vagina is its solubilized amount in the vaginal lumen, which in turn depends upon the intrinsic solubility capacity of the candidate compound in the microbicide vehicles or in the vaginal fluid. Achieving this may be quite challenging considering the limited aqueous solubility of many microbicide candidates, especially the small molecular and often rather lipophilic nature of HIV reverse transcriptase, integrase, or protease. Hence, it is of crucial importance that potential solubility issues of microbicide candidates are identified in early development or solved for such existing candidates to assist in candidate selection and guide formulation strategies. Many anti-retroviral drugs pose a challenge to formulation scientists due to their non-favorable properties like poor solubility and extensive first-pass metabolism by Cytochrome (CYP) mediate metabolism and Poly-glycoprotein (Pgp) efflux. To overcome these drawbacks modification of older ones, search for new chemical entities, use of alternate routes for drug delivery, formulation and development of novel drug delivery systems that can improve the solubility, bioavailability and hence efficacy of existing antiretroviral chemical entities are approaches presently developed and looked for.
In 1998 the pharmaceutical company Tibotec Therapeutics Inc. developed synthetic Protease Inhibitor (PI) Darunavir (DRVE) as monotherapy for treating wild type of HIV. Darunavir shows poor oral bioavailability at 37% due to poor aqueous solubility (Darunavir Ethanolate has a solubility of approximately 0.15mg/mL in water at 20ºC) and CYP metabolism and Pgp efflux. A combination with Ritonavir has been used to increase its oral bioavailability up to 80% but this combination is not recommended in patients with severe hepatic impairment. Also, co-administration of Darunavir with Ritonavir is contraindicated with drugs that are highly dependent on CYP3A for clearance, as high levels of plasma concentration are associated with serious and life-threatening events such as hypotension and cardiac arrhythmias. Thus, solubility and in turn bioavailability enhancement have been the bottleneck in the development of Darunavir. This problem becomes more critical when the drug has to be developed as a microbicide which is to be administered in the vaginal cavity where the volume of fluid to solubilize the drug is less (United States Food and Drug Administration, 2008; Adeoye and Cabral-Marques, 2017; Corrêaet al., 2012). Joachim B et al., 2012, demonstrated the solubility and permeability properties critical for microbicide development for 4 model microbicides: tenofovir, dapivirine, darunavir and saquinavir mesylate. Also, the effect of formulation excipients on both solubility and permeability was investigated. The report showed poor solubility (0.51 mM) of Darunavir at normal vaginal pH. Solubility of Darunavir was enhanced as compared to the pure drug by the addition of cyclodextrins but showed a poor level of solubility when a gel formulation of Darunavir was accessed in simulated vaginal fluid even in the presence of solubilizing agent; cyclodextrins. In terms of enabling permeation (HEC-1A cells) of microbicides, cyclodextrin-hydroxyethyl cellulose gels outperform alternative formulations, including emulsions and silicone elastomers. Potentials of Darunavir being incorporated into a microbicide vaginal ring formulation with Dapivirine-the current lead microbicide candidate have been reported. With the evidence of drug resistance mutation in HIV infection, Darunavir has been evaluated as a potential drug candidate in combination with microbicide strategies since these molecules have a relatively high barrier to the development of resistance (Diarmaid et al., 2018). Other formulation strategies to increase the solubility and dissolution profile of Darunavir ethanolate have been reported, notably, Mangesh B et al., 2017, formulated and developed the solid lipid nanoparticles of Darunavir to overcome the solubility and bioavailability associated problems of the drug. Also, the same group prepared the self-micro-emulsifying drug delivery system of Darunavir for lymphatic system uptake to improve bioavailability. B. Padmasriet al., 2016, formulated chewable release tablets of Darunavir for immediate release. Smeets Aet al., 2018, studied the feasibility of producing amorphous Darunavir solid dispersion by electro-spraying to improve its solubility. Darunavir’s oral bioavailability and dissolution could be enhanced by using solid self-nano emulsifying drug delivery systems, according to research conducted by Inugala S.et al. 2015.
These studies reported are towards developing formulation techniques for oral delivery of Darunavir and not for vaginal delivery. (Kogawaet al., 2016; Kogawaet al., 2015; Kogawaet al., 2014). reported a β-cyclodextrin inclusion complex with pure Darunavir and Darunavir Ethanolate which showed an increase in the solubility of more than 28 times as compared to the free Darunavir in acetate buffer 0.05 M at pH 4.5, 23 times in purified water and 22 times in phosphate buffer 0.2 M at pH 6.8, they attributed such results to changes in the crystalline form of the drug. Darunavir-cyclodextrin amorphous form showed rapid aqueous dissolution since the molecules were randomly arranged, requiring less energy to separate each other, resulting in faster dissolution with higher absorption. Though the cyclodextrins inclusion complex showed increased solubility but its performance in vaginal fluid needs to be accessed as far as its vaginal administration is concerned. Most studies for vaginal formulation developments are aimed at antibiotics and anti-fungal drugs whereas antiviral drugs are rarely involved in these studies. László Jicsinszky et al. have published a full-length review on the use of cyclodextrin in antiviral therapy (Jicsinszkyet al., 2021). Nanogel drug delivery system has been showing promising results with regards to API having poor solubility and bioavailability administered through a non-oral route. The term nanogel is defined as aqueous dispersions of hydrogel particles formed by a physically or chemically cross-linked polymer network composed of either homopolymers or copolymers of nanoscale size. Nanogel formulations show high stability, API loading capacity and responsiveness to environmental factors like pH, temperature, etc. Large surface area and nano size range; favors drug targeting, solubility and bioavailability enhancement (Yallapuet al., 2007; Jóhannessonet al., 2016). Recently cyclodextrins have been the polymer of choice in nano-system/nanogel construction which adds more advantage over simple cyclodextrins inclusion complex by giving free hand of manipulating behaviors, targeting and loading of the API. Adeoye and Cabral-Marques, 2017, have reviewed the cyclodextrins nanosystem in brief for further information. Thus, the present work aims to prepare cyclodextrins-based nanogel microbicide of Darunavir Ethanolate to increase its solubility and dissolution profile in pre-exposure prophylaxis in HIV infection.
MATERIALS AND METHODS
Materials
Hydroxypropyl-β-cyclodextrinM.W=1541.54 was purchased from ANALAB Fine Chemicals (Mumbai, India) and Hydroxypropyl Methyl Cellulose was bought from Loba Chemie Pvt. Ltd., (Mumbai, India), Span80, Ethylene Glycol Diglycidyl Ether and Dichloromethane were purchased from TCI Chemicals Pvt. Ltd., (India), Darunavir Ethanolate was gifted by Lupin Research Park (Pune, India), HPLC grade Methanol, Acetonitrile was purchased from Sigma Aldrich Ltd. (Mumbai, India) All other chemicals were of A R grade and were used as received.
Methods
Solubility studies of Darunavir Ethanolate (DRVE) in various medium at different pH condition
The solubility study of pure DRVE in simulated vaginal fluid and water at various pH conditions was performed. pH of the prepared Simulated Vaginal Fluid (SVF) (Marqueset al., 2011) was adjusted by using 0.2M NaOH and 0.2M HCL. An excess amount of pure drug was added to 10 mL simulated vaginal fluid of pH ranging from 1.5, 2.5, 3.5, 4.5, 5.5, 6.5 to 7.5 and was taken into a conical flask. The mixture was kept in the mechanical shaker (REMI, Mumbai, India) for 48 hr. at 37ºC and 80 rpm to aid solubilization. Further, the samples were kept at room temperature for 24 hr. to reach equilibrium. The sample was then filtered through the Whatman filter (CAT no.10001 pore size 0.041) and the filtrate was analyzed by using HPLC (Nagendrakumaret al., 2014) (Agilent, 1120 Compact LC, Germany). Also, the solubility enhancement study of prepared DRVE nanogel at pH 3.5, 4.5, 5.5 (normal vaginal pH range) was performed using the same methodology as described above.
Phase solubility studies of DRVE in SVF
The phase solubility study of DRVE was performed as per the Higuchi and Corners method (Higuchi, 1965) in the SVF medium. An excess quantity of DRVE (300 mg) was added to increasing concentration (10 µg/mL to 160 µg/mL) of Hydroxypropyl-β-Cyclodextrin (HPβCD) in 10 mL simulated vaginal fluid at pH 4.5.
The mixture was kept in the mechanical shaker (REMI, Mumbai, India) for 48 hr at 37ºC and 80 rpm to aid solubilization. Further, the samples were kept at room temperature for 24 hr to reach equilibrium. The sample was then filtered through the Whatman filter 0.45 µm size and the filtrate was analyzed by using HPLC (Agilent, 1120 CompactLC, Germany). The type of inclusion complex and the stability constant (ks) were determined from the phase solubility diagram using the equation of Higuchi and Connors:
where ks is stability constant (M-1), So is intrinsic solubility of DRVE. Slope is obtained from the linear line equation of the phase diagram.
Fourier Transforms Infrared (FTIR) studies of DRVE-HPβCD inclusion complex
FTIR analysis of the dried powder of the DRVE-HPβCD inclusion complex was done (Zidaneet al., 2016). DRVE-HPβCD inclusion complex was prepared using the same procedure as in the case of the phase solubility study described above. 2.5 mg of inclusion complex sample weighed on an analytical balance (Mettler Toledo, H51, Switzerland) were added to potassium bromide, which was previously dried to constant weight in an oven. Pellets were prepared each weighing 150 mg for FTIR analysis (Prestige-21, Shimadzu, Japan).
Preparation of nanogels
An emulsion-solvent evaporation method was used for the preparation of nano gel as described below (Moya-Ortegaet al., 2012; Moya-Ortegaet al., 2013).
Preparation of aqueous phase
To 10 mL (20%w/w) solution of HPβCD in 0.2 M NaOH, 4 mL of Ethylene Glycol Diglycidyl Ether (EGDE) was added and stirred for 5 min. To this 10 mL, HPMC solution in 0.2 M NaOH (1%, 2%w/w) was added under stirring, these batches were heated at 60ºC for 25 min.
Preparation of organic phase
The organic phase consists of solutions of Span 80 (0, 0.5, 1 and 2%w/v) in organic solvent dichloromethane.
Preparation of the w/o emulsions
14 mL of the aqueous phase was added to a 20 mL organic phase and homogenized using an ultra-turra T 25 (Janke and Kunkel, Ink-Labortechnik, Germany) at 4000 rpm for 8 min. The emulsion obtained was kept under a magnetic stirrer (100 rev./min) at 60ºC for 30 min. Immediately the emulsions were poured into 100 mL of distilled water and stirred at 60ºC for 3 hr for the complete evaporation of dichloromethane to form a colloidal dispersion. The residual content of the organic solvent was confirmed by gas chromatography.
Dialysis of the nanogels dispersion
50 mL of each colloidal system formed was placed into dialysis bags (MWCO 12-14 KDa) which were then placed into beakers containing water. The water in the beakers was replaced every 12 min to eliminate impurities. The colloidal dispersion was kept in a deep freezer at -40ºC and further lyophilized (Operon, FDB-5503, Korea) to form powder of unloaded HPβCD nanogel.
Evaluation of drug unloaded HPβCD nanogel
Determination of percentage yield
The percentage yield of freeze-dried nanogel was determined by gravimetry. Each batch of 0.5 g of freeze-dried nanogel was taken in 50 mL acetone (HPLC grade) and sonicated for 10 min at room temperature. Further separation of nanogel from the colloidal system was done by filtration. The Nanogel mass was further dried till consistent weight was achieved in the oven at 40ºC. The theoretical yield was taken as the sum of the weight of the HPβCD, HPMC and EDGE (as a cross-linker with a specific density of 1.114 g/mL) taken for each batch. The percentage yield of each batch of freeze-dried nanogel was determined by formula.
Determination of particle size and Polydispersity Index (PDI)
Determination of swelling ratio
The swelling ratio of the prepared nano gel was determined (Wonget al., 2015; Maliket al., 2017; Mohanraj and Chen, 2007). Approximately 0.2 g of freeze-dried nano gel was immersed in 100 mL of SVF and was removed periodically at 1, 3, 6 and 12 hr. reweighed after removing excess SVF carefully with a filter paper. The swelling ratio of the freeze-dried nano gel at each time was calculated using the formula: Given below
where, ws=weight of swollen sample and wd=weight of dry sample respectively.
Differential Scanning Calorimetry studies of drug unloaded/loaded HPβCD nanogel
Differential Scanning Calorimetry (DSC) measurements were performed on a DSC-821 (Mettler Toledo DSC, Switzerland) with a thermal analyzer (Manchunet al., 2016). Accurately weighed samples; pure DRVE, HPMC, HPβCD, unloaded and loaded nanogel (5 mg each) were placed in sealed aluminum pans separately, before heating under nitrogen flow (20 mL/min.) at a scanning rate of 10ºC/min from 25-250º using empty aluminum pan as reference.
Method of drug loading onto freeze-dried nanogel
As per the result obtained by phase solubility study, which showed stable 1:1 molar ratio inclusion complex formation of DRVE: HPβCD and all freeze-dried nano gel batches were loaded with DRVE in 1:1 molar ratio, the molecular weight of DRVE and HPβCD being 593.74 g/mol and 1541.51 g/mol respectively.10mL acetone solution of Darunavir Ethanolate (300 mg) was prepared. The formed solution was treated in an ultrasonic bath at 25ºC for 60 min. Aqueous solution of freeze-dried nanogel equivalent to 778.93 mg HPβCD was added to the drug solution and the samples were treated in an ultrasonic bath at 25ºC for 60 min. Water was evaporated using a Rotavapor R-210 with Vacuum controller V-850 (Buchi, Switzerland) (Shenet al., 2016).
Evaluation DRVE-loaded HPβCD nanogel
Entrapment efficiency
The entrapment efficiency of the formed nano gel was determined (Saraogiet al., 2022; Yaoet al., 2016). 0.5 g of DRVE-loaded nano gel was weighed accurately and transferred to a 100 mL conical flask, followed by the addition of 50 mL of acetone (HPLC grade) and sonicated for 10 min. The following colloidal dispersion was filtered and analyzed by HPLC (Agilent, 1120 Compact LC, Germany) for the concentration of DRVE untrapped. The following equation were used to calculate drug content and entrapment efficiency:
Determination of particle size, Polydispersity Index (PDI) and zeta potential
Optimized formulation
Based on results of entrapment efficiency, particle size, PDI and zeta potential, optimized formulation was selected which were further tested for mucoadhesion force and drug release.
Mucoadhesion test
The mucoadhesion test of the optimized formulation was performed in triplicate with the help of a goat vaginal mucosal membrane from a slaughterhouse maintained under simulated vaginal fluid of pH 4.5. The Brookfield CT3 texture analyzer (made in the USA) with a suitable probe was used for the determination of the mucoadhesive force(g) (Mannaet al., 2016).
Drug Release
The drug release of the optimized batch was performed by using the Franz diffusion cell (SES GmbH, Analyze system, Germany) method (Kleinet al., 2016; Bergincet al., 2012). 2g of nanogel equivalent to 300 mg was placed on a semi-permeable membrane of 25 mm diameter, 0.45 micrometer pore size which in turn was mounted on the glass fiber of the donor compartment. In the receptor compartment, simulated vaginal fluid of pH 4.5 was placed. About 0.5 mL of the sample was withdrawn while maintaining sink condition at regular time intervals up to 22 hr. Each 0.5mL was diluted up to 5 mL with acetonitrile: methanol (90:10) mobile phase for HPLC determination of the drug at 271 λmax. Percent cumulative drug diffusion vs time graph was plotted.
Drug release mathematical modeling
Mathematical modeling of the dissolution profiles was performed using KinetDS 3 rev. (Poland) (Shaikhet al., 2015; Heet al., 2011; Menniniet al., 2016; Balaschet al., 1996). Various models were applied to verify which model better fits the dissolution data using R2 (correlation coefficient), AIC (Akaike information criterion) and RMSE (root mean squared error) as selection parameters. Also, drug flux (J) was determined by the slope of the line equation generated by plotting cumulative drug release vs. time.
RESULTS AND DISCUSSION
Solubility studies of DRVE in various medium at different pH condition
As shown in Figure 1, solubility of pure DRVE in water was found in the range of 0.26 to 0.04 mg/mL, while solubility in SVF was found in the range of 0.06 to 0.009 mg/mL. The trend shows a sharp decline in the solubility of DRVE at higher pH. DRVE solubility was significantly enhanced in nanogel formulation in SVF medium as compared to pure DRVE (40.9753, 56.9891 and 100.91 fold at pH 3.5, 4.5 and 5.5 respectively).
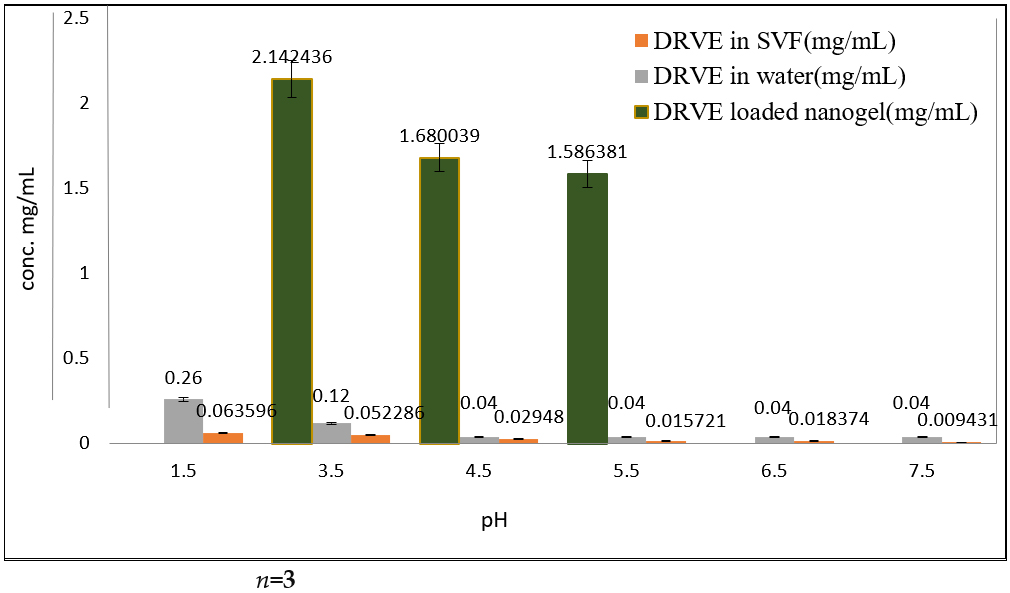
Figure 1:
Solubility study of pure and nanogel loaded DRVE in various mediums at different pH conditions.
Phase solubility studies
The equilibrium phase solubility study diagram for DRVE with HPβCD in SVF, as the chosen derivative of cyclodextrin for nanogel formulation, is presented in Figure 2. The solubility of DRVE increases as the concentration of HPβCD increases, indicating a linear relationship. As per the graph obtained it can be concluded formation of a 1:1 stoichiometric inclusion complex. Similar results were reported by Ana Carolina Kogawaet al., 2014, who reported a phase solubility study of Darunavir Ethanolate:βCD complex in purified water, indicating 1:1 complex formation. Comparing these two studies, it can be seen that Darunavir Ethanolate solubility was increased 16 to18 times by HPβCD complex as compared to βCD complex(though in different experimental conditions like pH and solvent e.g pH 4.5 and SVF) also stability constant stand at 618.071 m-1 for DRVE: HPβCD complex as compared to 526.48 m-1 for DRVE:βCD complex. This means DRVE stays for an average of 600 times longer close to the HPβCD cavity than when solvated freely in the solvent. Such a result can be attributed to the high hydrophilicity and high degree of substitution of HPβCD as compared to βCD which leads to higher solubilization capacity and good complex formation.
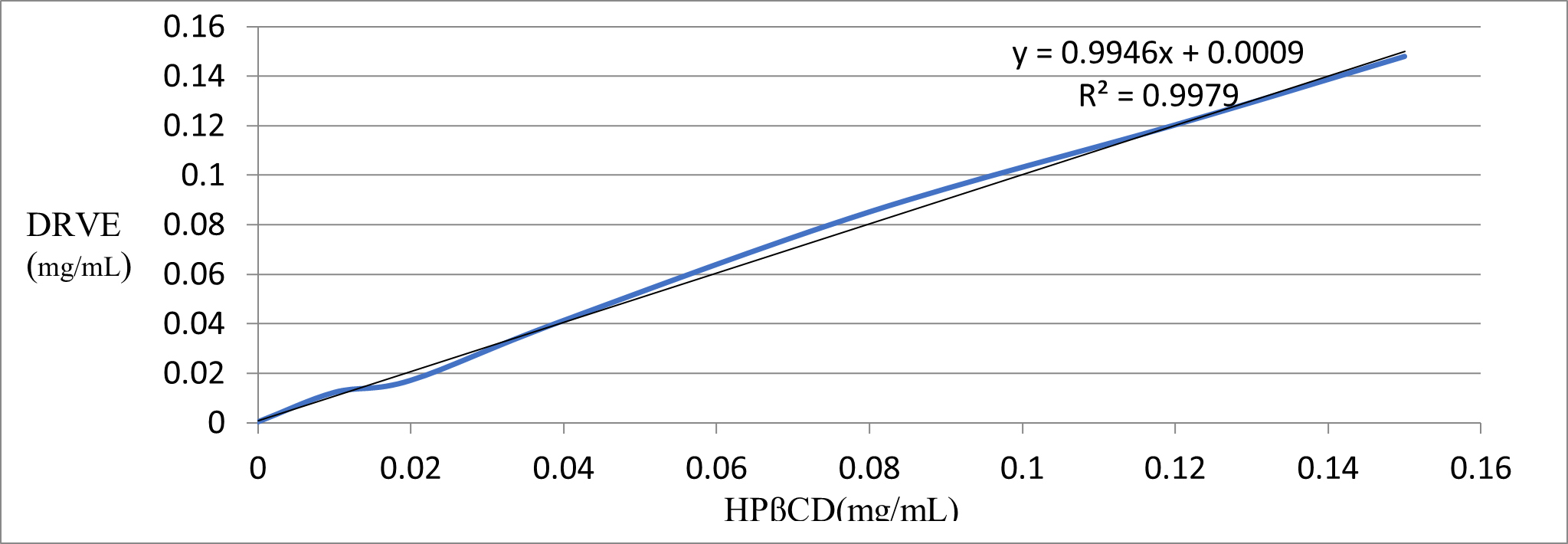
Figure 2:
Phase solubility diagram of DRVE with HPβCD in SVF (pH 4.5) at 37ºC.
FTIR studies of DRVE-HPβCD inclusion complex
FT-IR analysis of pure DRVE, HPβCD and lyophilized DRVE-HPβCD inclusion complex is shown in Figure 3. The characteristic peaks of pure DRVE-like primary amines (NH2 stretching) at 3456 cm-1, primary amines and alcohol at 3362 and 3258 cm-1 respectively, alkane and benzene ring at 3063 cm-1 and 2964 cm-1, amide and ester at 1707 and 1632 cm-1 respectively can be seen in the IR spectrum. In the lyophilized DRVE-HPβCD inclusion complex characteristic peaks of O-H stretching vibration at 3406 cm-1, =CH2 symmetrical stretching vibration, -CH stretching vibration at 2984 and 2930, H-O-H bending vibration at 1646 cm-1, symmetric C-O-C stretching vibration at 1083 cm-1 and 1033 cm-1 can be seen which are the characteristic peak of HPβCD alone. Characteristic peaks of pure drugs are not seen in the inclusion of complex products indicating its inclusion in the cavity of HPβCD.
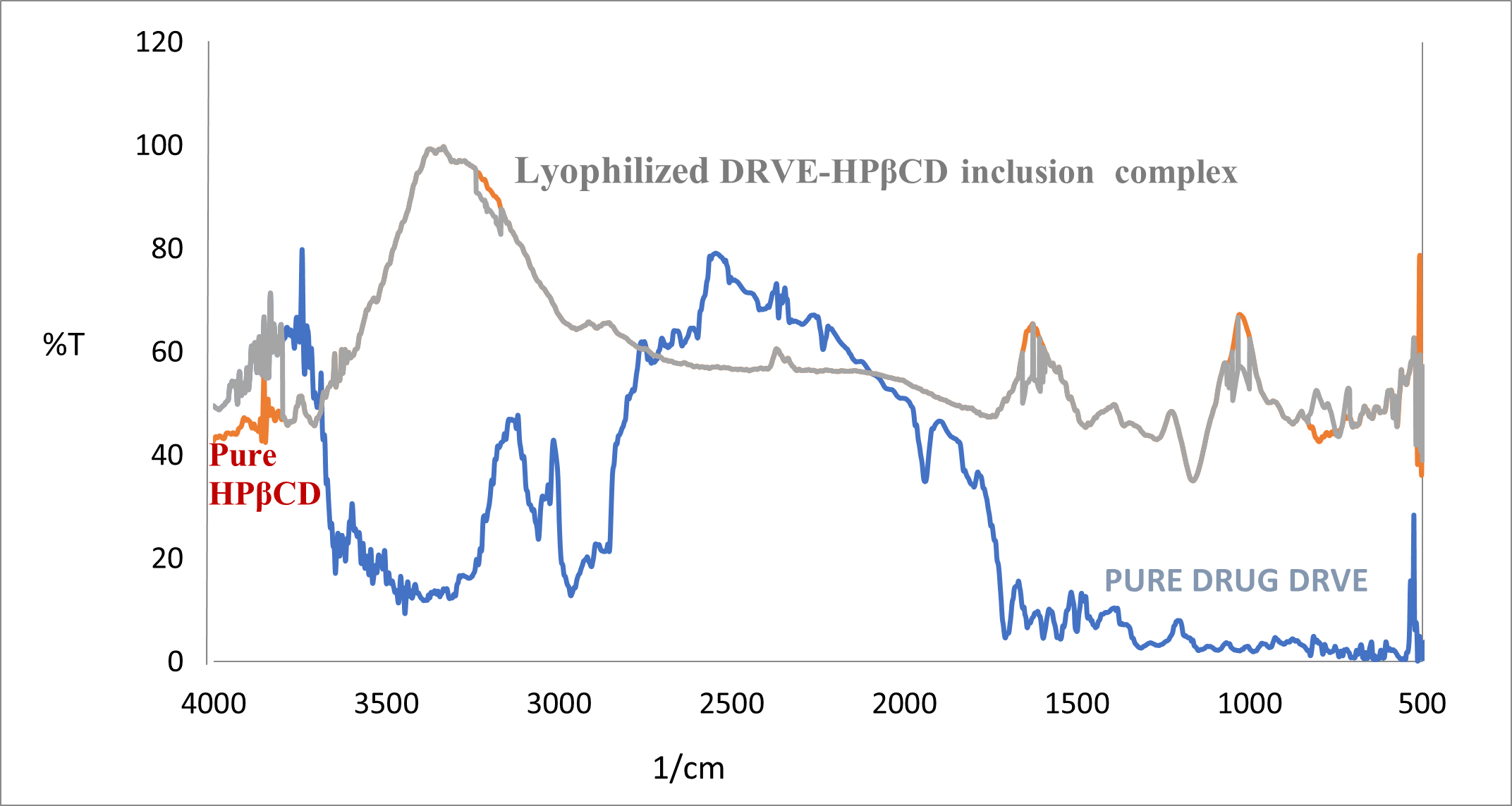
Figure 3:
Overlay FTIR spectrum of pure HPβCD, DRVE and DRVE-HPβCD inclusion complex.
Preparation of freeze-dried/unloaded HPβCD nanogel
Nanogel synthesis reaction
Various methods have been reported in the literature regarding the synthesis of nanogel. Nanogel is formed by a physically or chemically cross-linked polymer network composed of either homopolymers or copolymers of nanoscale size. The chosen polymer in this study is HPβCD and HPMC, while Ethylene Glycol Diglycidyl Ether (EGDE) is used as a crosslinker. The -OH groups present in the HPβCD and HPMC(non-toxic cellulose ether) react with the epoxide groups of EGDE (non-toxic cross-linker) in the presence of mild alkaline condition (0.2M NaoH) and high temperature of 60ºC to form a highly cross-linked complex. The -OH groups of HPMC at C2 and C6 and in the case of HPβCD,-OH groups at C2of backbone linkage and -OH groups at C2 and C6 of branch react with epoxide rings of EGDE lead to the formation of stable HPβCD and HPMC nanogel (Moya-Ortega et al., 2017). Nanogel was synthesized as per the scheme provided in Table 1 by varying the concentration of HPMC and Span80. A schematic diagram as shown in Figure 4.
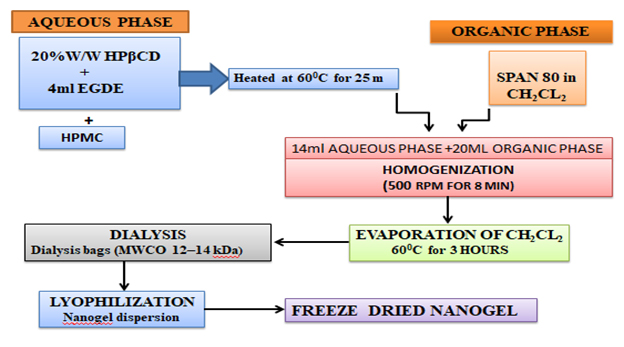
Figure 4:
A schematic diagram of HPβCD nanogel synthesis.
Formula for Nanogel Preparation | |||
---|---|---|---|
20(%W/W)HPβCD | HPMC(%w/w) | Span (80%w/v) | Batch |
1 | 0 | F1 | |
0.5 | F2 | ||
1 | F3 | ||
2 | F4 | ||
2 | 0 | F5 | |
0.5 | F6 | ||
1 | F7 | ||
2 | F8 |
Nanogel formulation batches.
Percentage yield, Particle size, Polydispersity Index (PDI) analysis of unloaded nanogel
The percentage yield of synthesized nanogel, its particle size and PDI were determined which are indicated in Table 2. Batch F8 shows the highest percentage yield and batch F1 with the least yield. The data shows an increase in the percentage yield of the nanogel as the concentration of HPMC and Span 80 increases. Span 80 acts as a stabilizer during the formation of nanogel leading to more crosslinking of HPMC with HPβCD by Ethylene Glycol Diglycidyl Ether (EGDE). Also, the concentration of Span 80 had an effect on the particle size of the nanogel. Batch F1 to F4 and F5 to F8 with a fixed level of HPMC (1% and 2% respectively) showed decreases in particle size as the concentration of Span 80 increased. Batch F4 with HPMC (1%) and Span 80 (2%) were found to have the smallest particle size 263.6 nm, with a PDI of 0.525 and batch F5 with HPMC (2%) and Span 80 (0%) were found to have the largest size of 328.6 nm and PDI of 0.382. Span80 acts as an emulsifier/stabilizer an increase in the amount of emulsifier reduces the droplet size, which in turn reduces the final particle size. Thus, it can be concluded that the concentration of both HPMC and Span 80 plays a vital role in determining the particle size of the nanogel.
Batch | Percentage yield | Particle size (nm) | PDI |
---|---|---|---|
F1 | 30.43(±0.0267) | 321.6(±0.017778) | 0.413(±0.04667) |
F2 | 38.73(±0.0069) | 302.3(±4.694444 | 0.456(±0.0333) |
F3 | 42.31(±0.0007) | 283.6(±0.694444) | 0.425(±0.02) |
F4 | 65.87(±0.0427) | 263.6(±0.751111) | 0.525(±0.0167) |
F5 | 44.31(±0.0002) | 328.6(±0.004444) | 0.382(±0.0667) |
F6 | 49.87(±0.0152) | 313.4(±0.09) | 0.397(±0.397) |
F7 | 54.32(±0.0324) | 294.5(±0.071111) | 0.467(±0.0333) |
F8 | 67.12(±0.1419) | 265.9(±0.587778) | 0.497(±0.0497) |
Percentage yield, particle size, PDI of unloaded nanogel.
Determination of swelling ratio
The swelling ratio of different trial batches is presented in Table 3. Batch F8 with 2% HPMC and 2% Span 80 was found to have the least swelling ratio and batch F1 with 1% HPMC and 0% span80 was found to have the highest swelling ratio. The swelling ratio was found to have a direct relationship with the amount of HPMC, as the amount of HPMC increases the swelling ratio decreases as it acts as a barrier for water to penetrate the nanogel, while as the concentration of Span80 increases leading to more stability of nanoparticles which in turn needs more energy and time to break the bonds leading to swelling. Swelling of the nanogel on contact with the medium in this case the SVF determines its capability of controlled release of the drug. Thus more the swelling more the drug is retained in the nanogel and the slower/delayed/controlled drug release. As for our research is a concern we like the drug to get released faster from the nano gel and reach the target area in its optimum concentration to render the person protected from HIV infection.
Batch | 1 hr | 3 hr | 6 hr | 12 hr |
---|---|---|---|---|
F1 | 310(±1) | 500(±1.78) | 650(±0.11) | 650(±0.11) |
F2 | 300(±1.78) | 450(±1) | 550(±1) | 550(±1) |
F3 | 270(±2.78) | 380(±0.11) | 530(±0.44) | 530(±1) |
F4 | 240(±5.44) | 350(±1.78) | 500(±2.78) | 500(±0.11) |
F5 | 220(±1) | 330(±1) | 485(±4.00) | 485(±1) |
F6 | 175(±0.11) | 315(±2.78) | 460(±0.44) | 460(±0.13) |
F7 | 155(±0.44) | 295(±1) | 420(±0.44) | 420(±1.86) |
F8 | 125(±4) | 225(±0.69) | 300(±0.44) | 300(±1) |
Swelling ratio of all unloaded nanogel batches.
DSC studies nanogel
DSC overlay of pure Darunavir ethanolate, HPMC, HPβCD, unloaded nanogel and Darunavir ethanolate-loaded HPβCD nanogel is shown in Figure 5. The characteristic endothermic peak of pure DRVE, HPMC and HPβCD is observed at 80ºC, 92.48ºC and 284.06ºC respectively. The sharp endothermic peak of Darunavir Ethanolate at 80ºC is missing in unloaded (as it does not contain the drug) and loaded nanogel indicating the inclusion of DRVE in the HPβCD cavity.
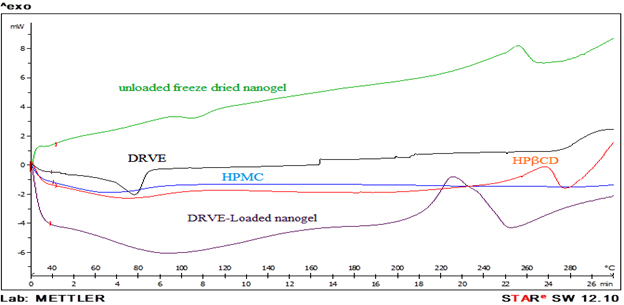
Figure 5:
DSC overlay of pure Darunavir ethanolate, HPMC, HPβCD, unloaded nano gel and Darunavir ethanolate-loaded HPβCD nano gel.
Evaluation DRVE-Loaded HPβCD Nanogel
Entrapment efficiency, Particle size, Polydispersity index and Zeta potential of DRVE-loaded HPβCD Nanogel
DSC overlay of pure Darunavir ethanolate, HPMC, HPβCD, unloaded nanogel and Darunavir ethanolate-loaded HPβCD nanogel is shown in Figure 5. The characteristic endothermic peak of pure DRVE, HPMC and HPβCD observe Entrapment efficiency, particle size, polydispersity index and zeta potential of DRVE-loaded HPβCD Nanogel is reported in Table 4. It can be observed that as the concentration of Span 80 increases, entrapment efficiency increases while with an increase in HPMC concentration, entrapment efficiency decreased. Batch F4 with 1% HPMC and 2% of Span80 was found to have the highest (%) entrapment efficiency at 94.19, while batch F8 with 2% HPMC and 2% of Span80 showed (%) entrapment efficiency at 91.71. HPMC plays a major role as compared to Span80 in increasing or decreasing the entrapment efficiency. Low drug entrapment efficiency at higher HPMC concentration may be due to the hindrance of HPMC by blocking the HPβCD cavity for loading while high entrapment efficiency at higher Span80 concentration may be due to the stabilizing effect of Span80. Batch F8 with 2% HPMC and 2% span 80 was found to be highly stable with a particle size of 338.8, PDI of 0.344 and zeta potential of -40 mV and batch F1 with a particle size of 436.2 nm with PDI of 0.301 and zeta potential of 14.3 mV were found to be in a relatively stable range. Based on particle size, PDI, zeta potential and (%) Entrapment Efficiency of DRVE-loaded nanogel batch F8 was selected as an optimized batch, which was further analyzed for mucoadhesion and drug released at 80ºC, 92.48ºC and 284.06ºC respectively. The sharp endothermic peak of Darunavir Ethanolate at 80ºC is missing in unloaded (as it does not contain the drug) and loaded nanogel indicating the inclusion of DRVE in the HPβCD cavity.
Batch | (%) Entrapment efficiency | Particle size(nm) | PDI(±SD) | Zeta potential (mV) |
---|---|---|---|---|
F1 | 89.79(±0.14) | 436.2(±0.03) | 0.301(±0.08) | 14.3(±0.75) |
F2 | 91.40(±0.02) | 383.2(±0.49) | 0.411(±0.03) | 16.1(±1.87) |
F3 | 92.71(±0.25) | 339.4(±1) | 0.428(±0.06) | -34.7(2.45) |
F4 | 94.19(±0.08) | 326.9(±0.00) | 0.313(±0.02) | -31.1(±1.96) |
F5 | 84.51(±0.00) | 455.3(±2.56) | 0.429(±0.03) | 16.3(±1.28) |
F6 | 89.23(±0.00) | 434.6(±4.13) | 0.369(±0.04) | 20.3(±0.87) |
F7 | 91.71(±0.00) | 340.3(±0.03) | 0.396(±0.02) | -39.6(±1.14) |
F8 | 93.52(±0.01) | 338.8(±1.87) | 0.344(±0.01) | -40.3(±0.02) |
% Entrapment efficiency, particle size, PDI and zeta potential of drug loaded nanogel.
Mucoadhesion anddrug release study of selected nanogel batch
The mucoadhesion of an optimized F8 batch was found in the range of 14.8 g to 16.7 g indicating firm adherence gel in the vaginal lumen and least chances of spillage. % cumulative release vs time profile is indicated in Figure 6. It can be seen that the drug release showed an initial lag time of 0.45 to 1 hr. 83% to 89% drug release was observed at the end of 22 hr. Mathematical modeling was done to analyze the mechanism of drug release according to selection criteria (R2, AIC, RMSE) as indicated in Table 5. Drug release followed a mixed pattern and The Korsmeyer-Peppas model was observed as the best fit indicating drug release from polymeric system and also Higuchi model was observed as the second-best fit indicating drug release from the matrix system and zero-order model as third best fit indicating drug release is independent of its concentration. Drug flux was found to be 0.4421 µg/cm2/hr as calculated from the slope of the linear part of the plot.
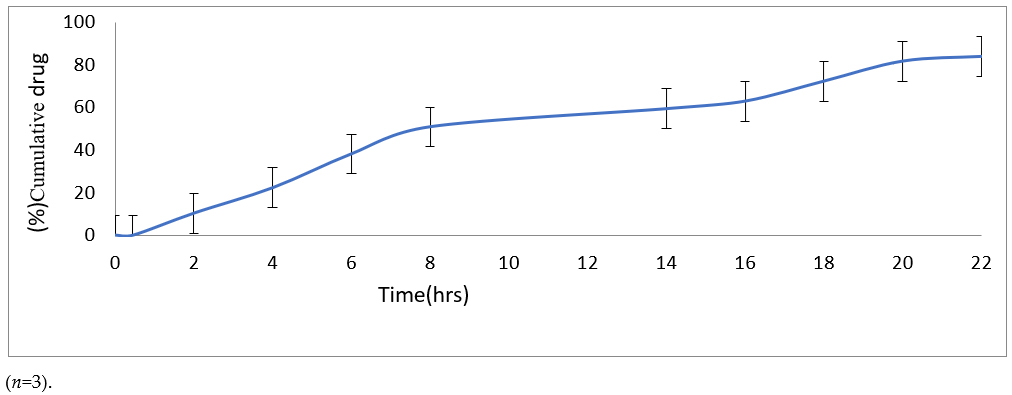
Figure 6:
Cumulative drug release vs. time profile of Darunavir Ethanolate nanogel.
Model | R2 | RMSE | AIC |
---|---|---|---|
Zero order kinetics | 0.933 | 6.414 | 64.196 |
First order kinetics | 0.764 | 11.2477 | 75.196 |
Second order kinetics | 0.5208 | 166.7411 | 129.354 |
Third order kinetics | 0.3564 | 49.4528 | 105.046 |
Korsmeyer-Peppas model | 0.9536 | 5.3646 | 60.623 |
Hickson-Crowell | 0.8369 | 8.8510 | 70.636 |
Higuchi | 0.9544 | 5.5244 | 61.209 |
Mathematical modeling for selected batch F8.
CONCLUSION
The present work demonstrated the capability of Hydroxypropyl-β-cyclodextrin nanogel to increase the solubility and dissolution profile of a poor solubility drug, Darunavir ethanolate. Here we can conclude that after application of the nanogel 2 hr before the actual intercourse in the vaginal lumen with approximately 20% of solubilized drug present can render the person safe from transfer of HIV infection: the dose of gel being 300 mg. Our research opens the door to several future research directions in the area of HIV infection and exploring other potential anti-retroviral drugs to be developed as microbicides. In summary, our findings support the potential of cyclodextrin nanogels as versatile carriers for the anti-retroviral drug Darunavir as a microbicide agent. However, further research and development are needed to unlock their full potential and address any remaining challenges such as an in vivo study to assess the in vivo performance of the formulated nanogel.
Cite this article:
Bandivadekar M, Madgulkar A, Rashid SM, Padakanti R, Godbole V. Formulation and Evaluation of Darunavir Ethanolate Nanogel as Microbicide in Pre-Exposure Prophylaxis for HIV Infection. Int. J. Pharm. Investigation. 2025;15(2):10-8.
ABBREVIATIONS
HIV | Human immunodeficiency virus |
---|---|
WHO | World Health Organization |
DRVE | Darunavir Ethanolate |
SVF | Simulated vaginal fluid |
HPβCD | Hydroxypropyl-β-cyclodextrin |
FTIR | Fourier transforms infrared |
NaOH | Sodium Hydroxide |
EGDE | Ethylene glycol diglycidyl ether |
HPMC | Hydroxy propyl methyl cellulose |
PDI | Polydispersity index |
DSC | Differential scanning calorimetry |
References
- Adeoye O., Cabral-Marques H.. (2017) Cyclodextrin nanosystems in oral drug delivery: A mini review. International Journal of Pharmaceutics 531: 521-531 Google Scholar
- Baeten J. M., Palanee-Phillips T., Brown E. R., Schwartz K., Soto-Torres L. E., Govender V., Mgodi N. M., Matovu Kiweewa F., Nair G., Mhlanga F., Siva S., Bekker L.-G., Jeenarain N., Gaffoor Z., Martinson F., Makanani B., Pather A., Naidoo L., Husnik M., et al. (2016) Use of a vaginal ring containing dapivirine for HIV-1 prevention in women. The New England Journal of Medicine 375: 2121-2132 Google Scholar
- Balasch J., Fábregues F., Ordi J., Creus M., Peñarrubia J., Casamitjana R., Manau D., Vanrell J. A., et al. (1996) Further data favoring the hypothesis of the uterine first-pass effect of vaginally administered micronized progesterone. Gynecological Endocrinology 10: 421-426 Google Scholar
- Berginc K., Skalko-Basnet N., Basnet P., Kristl A.. (2012) Development and evaluation of an in vitro vaginal model for assessment of drug’s biopharmaceutical properties: Curcumin. AAPS PharmSciTech 13: 1045-1053 Google Scholar
- Bhalekar M., Upadhaya P., Madgulkar A.. (2017) Formulation and characterization of solid lipid nanoparticles for an anti-retroviral drug Darunavir. Applied Nanoscience 7: 47-57 Google Scholar
- Bhalekar M. R., Pokale R., Bandivadekar M., Madgulkar A., Micro N. P. S., Madgulkar A., et al. (2016) Self micro-emulsifying drug delivery system for lymphatic uptake of Darunavir. J Drug Discov develop and Deliv 3: 1024 Google Scholar
- Coelho L. E., Torres T. S., Veloso V. G., Landovitz R. J., Grinsztejn B.. (2019) Pre-exposure prophylaxis 2.0: New drugs and technologies in the pipeline. The Lancet. HIV 6: e788-e799 Google Scholar
- Cutler B., Justman J.. (2008) Vaginal microbicides and the prevention of HIV transmission. The Lancet. Infectious Diseases 8: 685-697 Google Scholar
- Garg S., Kandarapu R., Vermani K., Tambwekar K. R., Garg A., Waller D. P., Zaneveld L. J. D., et al. (2003) Development pharmaceutics of microbicide formulations. Part I: preformulation considerations and challenges. AIDS Patient Care and STDs 17: 17-32 Google Scholar
- Grammen C., Augustijns P., Brouwers J.. (2012) In vitro profiling of the vaginal permeation potential of anti-HIV microbicides and the influence of formulation excipients. Antiviral Research 96: 226-233 Google Scholar
- He Z.-X., Wang Z.-H., Zhang H.-H., Pan X., Su W.-R., Liang D., Wu C., et al. (2011) Doxycycline and hydroxypropyl-β-cyclodextrin complex in poloxamer thermal sensitive hydrogel for ophthalmic delivery. Acta Pharmaceutica Sinica B 1: 254-260 Google Scholar
- Higuchi T. K.. (1965) A phase solubility technique. Adv. Anal. Chem. Instrum. 4: 117-211 Google Scholar
- Imperiale J. C., Bevilacqua G., de Rosa P., Sosnik A.. (2014) Production of pure indinavir free base nanoparticles by a supercritical anti-solvent (SAS) method. Drug Development and Industrial Pharmacy 40: 1607-1615 Google Scholar
- Inugala S., Eedara B. B., Sunkavalli S., Dhurke R., Kandadi P., Jukanti R., Bandari S., et al. (2015) Solid self-nanoemulsifying drug delivery system (S-SNEDDS) of Darunavir for improved dissolution and oral bioavailability: In vitro and in vivo evaluation. European Journal of Pharmaceutical Sciences 74: 1-10 Google Scholar
- Jicsinszky L., Martina K., Cravotto G.. (2021) Cyclodextrins in the antiviral therapy. Journal of Drug Delivery Science and Technology 64 Google Scholar
- Jóhannesson G., Stefánsson E., Loftsson T.. (2016) Microspheres and nanotechnology for drug delivery. Developments in Ophthalmology 55: 93-103 Google Scholar
- Klein R. R., Tao J. Q., Burchett K.. (2016) Development of an in vitro release test (IVRT) for a Vaginal Microbicide Gel. Journal of Pharmacy and Pharmacology 68: 762-771 Google Scholar
- Kogawa A. C., Antonio S. G., Salgado H. R.. (2015) Characterization of Darunavir: β-cyclodextrin complex and comparison with the forms of Darunavir ethanolate and hydrate. Journal of Pharmaceutical Sciences and Emerging Drugs 3 Google Scholar
- Kogawa A. C., Corrêa J. C., Salgado H. R.. (2014) Influence of Darunavir: β-cyclodextrin complex on the solubility of Darunavir. Research and reviews. Journal of Pharmacology and Toxicology : 50-55 Google Scholar
- Kogawa A. C., Regina H., Salgado N.. (2016) Drug Optimization: Fighting research to achieve the greatest use of Darunavir. 1: 21-22 Google Scholar
- Kogawa A. C., Zoppi A., Quevedo M. A.. (2014) Complexation between Darunavir ethanolate and β-Cyclodextrin experimental and Theoretical studies. World Journal of Pharmacy and Pharmaceutical Sciences 3: 298-309 Google Scholar
- Malik N. S., Ahmad M., Minhas M. U.. (2017) Cross-linked β-cyclodextrin and carboxymethyl cellulose hydrogels for controlled drug delivery of acyclovir. PLOS One 12: Article e0172727 Google Scholar
- Manchun S., Dass C. R., Sriamornsak P.. (2016) Stability of freeze-dried pH-responsive dextrin nanogels containing doxorubicin. Asian Journal of Pharmaceutical Sciences 11: 648-654 Google Scholar
- Manna S., Lakshmi U., Racharla M., Sinha P., Kanthal L., Kumar S., et al. (2016) Bioadhesive HPMC gel containing gelatin nanoparticles for intravaginal delivery of tenofovir. Journal of Applied Pharmaceutical Science 6: 22-29 Google Scholar
- Marques M. R. C., Loebenberg R., Almukainzi M.. (2011) Simulated biological fluids with possible application in dissolution testing. Dissolution Technologies 18: 15-28 Google Scholar
- Mennini N., Casella G., Cirri M., Maestrelli F., Mura P.. (2016) Development of cyclodextrin hydrogels for vaginal delivery of dehydroepiandrosterone. The Journal of Pharmacy and Pharmacology 68: 762-771 Google Scholar
- Mohanraj V. J., Chen Y.. (2007) Nanoparticles-a review. Tropical Journal of Pharmaceutical Research 5: 561-573 Google Scholar
- Moya-Ortega M. D., Alvarez-Lorenzo C., Sigurdsson H. H., Concheiro A., Loftsson T.. (2012) Cross-linked hydroxypropyl-β-cyclodextrin and γ-cyclodextrin nanogels for drug delivery: Physicochemical and loading/release properties. Carbohydrate Polymers 87: 2344-2351 Google Scholar
- Moya-Ortega M. D., Alves T. F. G., Alvarez-Lorenzo C., Concheiro A., Stefánsson E., Thorsteinsdóttir M., Loftsson T., et al. (2013) Dexamethasone eye drops containing γ-cyclodextrin-based nanogels. International Journal of Pharmaceutics 441: 507-515 Google Scholar
- Murphy D. J., Desjardins D., Boyd P., Dereuddre-Bosquet N., Stimmer L., Caldwell A., Le Grand R., Kelly C., van Roey J., Malcolm R. K., et al. (2018) Impact of ring size and drug loading on the pharmacokinetics of a combination dapivirine-darunavir vaginal ring in cynomolgus macaques. International Journal of Pharmaceutics 550: 300-308 Google Scholar
- Nagendrakumar A., Rao S., Rao B.. (2014) Validated rp-hplc method for the determination of Darunavir in bulk and pharmaceutical formulation. Research Journal of Pharmaceutical, Biological and Chemical Sciences 5: 63-72 Google Scholar
- Nel A., Van Niekerk N., Kapiga S., Bekker L.-G., Gama C., Gill K., Kamali A., Kotze P., Louw C., Mabude Z., Miti N., Kusemererwa S., Tempelman H., Carstens H., Devlin B., Isaacs M., Malherbe M., Mans W., Nuttall J., et al. (2016) Safety and efficacy of a dapivirine vaginal ring for HIV prevention in women. The New England Journal of Medicine 375: 2133-2143 Google Scholar
- Padmasri B., Kalyani R., Deepthi K.. (2016) Formulation and evaluation of Darunavir chewable tablets. Int. J. Pharm. Anal Res 5: 400-409 Google Scholar
- Rençber S., Karavana S. Y., Şenyiğit Z. A., Eraç B., Limoncu M. H., Baloğlu E., et al. (2017) Mucoadhesive in situ gel formulation for vaginal delivery of clotrimazole: Formulation, preparation and in vitro/in vivo evaluation. Pharmaceutical Development and Technology 22: 551-561 Google Scholar
- Ruela Corrêa J. C., D’Arcy D. M., dos Reis Serra C. H., Nunes Salgado H. R.. (2012) Darunavir: A critical review of its properties, use and drug interactions. Pharmacology 90: 102-109 Google Scholar
- Saraogi G. K., Tholiya S., Mishra Y., Mishra V., Albutti A., Nayak P., Tambuwala M. M., et al. (2022) Formulation development and evaluation of pravastatin-loaded nanogel for hyperlipidemia management. Gels 8: 81 Google Scholar
- Shaikh H. K., Kshirsagar R. V., Patil S. G.. (2015) Mathematical models for drug release characterization: A review. World Journal of Pharmacy and Pharmaceutical Sciences 4: 324-338 Google Scholar
- Shen C. Y., Xu P. H., Shen B. D., Min H. Y., Li X. R., Han J., Yuan H. L., et al. (2016) Nanogel for dermal application of the triterpenoids isolated from Ganoderma lucidum (GLT) for frostbite treatment. Drug Delivery 23: 610-618 Google Scholar
- Smeets A., Koekoekx R., Clasen C., Van den Mooter G.. (2018) Amorphous solid dispersions of Darunavir: Comparison between spray drying and electrospraying. European Journal of Pharmaceutics and Biopharmaceutics 130: 96-107 Google Scholar
- . (2008) PREZISTATM (Tibotec, Inc.) (Darunavir) 6: 445 Google Scholar
- Wong R. S. H., Ashton M., Dodou K.. (2015) Effect of crosslinking agent concentration on the properties of unmedicated hydrogels. Pharmaceutics 7: 305-319 Google Scholar
- Yallapu M. M., Reddy M. K., Labhasetwar V.. (2007) Nanogels: Chemistry to drug delivery. Journal of Biomedical Nanotechnology 30: 131-171 Google Scholar
- Yao Y., Xia M., Wang H., Li G., Shen H., Ji G., Meng Q., Xie Y., et al. (2016) Preparation and evaluation of chitosan-based nanogels/gels for oral delivery of myricetin. European Journal of Pharmaceutical Sciences 91: 144-153 Google Scholar
- Zidane S., Maiza A., Bouleghlem H., Herizi W., Dahmani S.. (2016) Investigation of cyclodextrin inclusion compounds using FT-IR, SEM and X-ray diffraction. International Journal of Chemical Engineering and Applications 7: 182-185 Google Scholar