ABSTRACT
Background
Alzheimer’s disease has become a serious health problem in recent years. Certain acetylcholinesterase inhibitors have been shown to have anti-Alzheimer’s properties. In the current work, a number of oxazepine compounds were developed and docked against acetylcholinesterase (PDB ID: 7e3h) in order to investigate such activity. The ligands and conventional acetylcholinesterase inhibitors were contrasted.
Materials and Methods
The ligands were sketched in .mol format using ChemSketch and then converted to .pdb format using Avogadro. The iGEMDOCK software was used for molecular docking investigations and Discovery Studio Visualizer was used to display the results.
Results and Discussion
Most of the compounds showed improved binding affinities for acetylcholinesterase enzyme. Compared to common acetylcholinesterase inhibitors like galantamine (-102.1 kcal/mol) and rivastigmine (-91.8 kcal/mol), all of the ligands demonstrated lower binding energies. For visualization, the top two compounds, 8d (-109.2 kcal/mol) and 9c (-102.6 kcal/mol), were selected.
Conclusion
Oxazepine derivatives show promise as an anti-Alzheimer’s disease treatment. Because compared to conventional antagonists, they have a higher binding affinity to the acetylcholine esterase enzyme.
INTRODUCTION
A progressive loss of cognitive function and episodic memory is the hallmark of Alzheimer’s Disease (AD), the most prevalent kind of dementia. In addition to verbal and visuospatial deficits, this results in behavioral issues like melancholy, aggressiveness and indifference. Extracellular plaques of insoluble β-amyloid peptide (Aβ) and Neurofibrillary Tangles (NFTs) containing hyperphosphorylated tau Protein (P-tau) in the cytoplasm of neurons are two important pathological characteristics seen in the brains of AD patients. Genetics is responsible for around 70% of the chance of acquiring AD. Moreover, acquired variables that raise the risk of AD include diabetes, obesity, dyslipidemia, hypertension and cerebrovascular illnesses (Silvaet al., 2019).
Different pharmacological strategies are used to treat Alzheimer’s disease, a progressive neurodegenerative ailment marked by cognitive decline and memory loss. Among the medication groups being investigated for their possible advantages in treating this illness are acetylcholinesterase inhibitors and benzodiazepines. Oxazepines are a class of drugs that act on the brain’s neurotransmitter systems to modulate neuroprotective and cognitive-enhancing effects. Through its effects on the GABA and glutamate pathways, they may aid in mood stabilization and cognitive enhancement. On the other hand, acetylcholinesterase inhibitors work by inhibiting the enzyme that breaks down acetylcholine, an essential neurotransmitter for memory and learning. These medications improve cholinergic transmission and cognitive performance by blocking this enzyme, which raises acetylcholine levels in the brain. Among the common acetyl cholinesterase inhibitors are galantamine, rivastigmine and donepezil. All of these therapies work to reduce symptoms, delay the onset of the illness and enhance the quality of life for those who have Alzheimer’s. Nevertheless, further study is necessary to completely comprehend their effectiveness and maximize their application in therapeutic contexts (Nordberg and Svensson, 1998; Abduljelilet al., 2022). Oxazepine derivatives were first introduced in 1965 and it is used in the relief of the psychoneuroses which is characterized by anxiety and tension. Oxazepines are seven membered heterocyclic compounds with oxygen and nitrogen (Wilson and Gisvold, 1966). Oxazepines can be prepared by multistep reactions. In the first step schiff base derivative is prepared from amino group of primary amine compounds reacts with an active carbonyl group of aldehydes or ketones. And within the second step condensation reaction takes place between imine or schiff base (-N=CH-) compounds and various anhydrides such as pthalic, maleic and succinic anhydride to form oxazepine compounds according to pericyclic reaction (Ruaa et al., 2012; Abdullatieef, 2010; Abdullatieef, 2010; Ayadet al., 2015; Nagham, 2013; Vermet al., 2015). Oxazepine and their derivatives have some important biological and pharmacological activities such as enzyme inhibitors (Moawad, 1989), analgesic (Muhammadet al, 2024), antidepressant, psychoactive drugs (Mesripouret al., 2023), anti-bacterial (Yaoet al., 2021), anti-fungal (Serrano-Wuet al., 2002), hypnotic muscle relaxant (Aljohaniet al., 2022), antagonistic (Qiet al., 2022), anti-epileptic (Siddiquiet al., 2020) and anti-inflammatory activity (Hussainet al., 2020; Vasudhaet al., 2024).
MATERIALS AND METHODS
General scheme for synthesis of oxazepine derivative
STEP 1: PREPARATION OF SCHIFF BASES
Equimolar amounts of aldehyde derivatives (0.01 moles) and anthranilic acid (0.01 moles) were accurately weighed and dissolved in 25 mL of ethyl alcohol. A small amount of glacial acetic acid and sodium lauryl sulfate was added to the solution. The resulting mixture was refluxed for 2-4 hr. The solid product formed was vacuum-filtered, purified by recrystallization from ethanol and then dried.
STEP 2: PREPARATION OF OXAZEPINE DERIVATIVE
Equimolar amounts of Schiff base (0.01 moles) from step-1 and various anhydrides (0.01 moles) of phthalic anhydride (4), maleic (5) and succcinic anhydrides (6) were taken and dissolved in 20 mL of Dimethyl Formamide (DMF) and add 2-3 drops of glacial acetic acid and reflex it for 8-10 hr. The reaction time was determined by thin layer chromatography with mobile phase n-hexane: ethyl acetate (7:3). The resultant synthesized oxazepines were collected after filtration of the reaction mixture and purified by recrystallization using absolute alcohol and then washed, dried.
Synthesis of oxazepine derivatives
The synthetic procedures for oxazepine derivatives were taken from the existing literature and final products were designed in accordance with the scheme. Vast library of compounds was acquired and they underwent an in silico toxicity screening using the software TopKat (Prival, 2001) and then, utilizing SwissADME software, their ADME characteristics were anticipated (Dainaet al., 2017; Dainaet al., 2019). For molecular docking investigations, designed compounds with good ADME properties and predicted non-toxic and non-carcinogenic compounds were selected.
Molecular Docking
The Swiss Target Prediction software was used to choose the protein target from a library of compounds that were prepared in accordance with the previously outlined scheme. Swiss Target Prediction software predicted every possible combination for the target protein, including safe, non-toxic and non-carcinogenic ligands. Acetyl cholinesterase enzyme has been identified as a potential target to the majority of ligands.
ChemSketch
After drawing the ligands’ 2D structures with ChemSketch, they were saved in .mol format. The ligand structures were translated from the .mol format to the .pdb format using the Avogadro tool (Hanwellet al., 2012). For the produced compounds that are safe, non-carcinogenic and have strong ADME properties, docking studies were carried out to assess binding poses and interactions.
The iGEMDOCK version 2.1
For docking, software was utilized. The acronym for Genetic Evolutionary Method for Molecular Docking is GEMDOCK. iGEMDOCK is the name of a graphical-automated drug design system for docking, screening and analysis. With reference to the protein’s active site, this software determines the ligands’ orientation and structure. To evaluate how the selected safe chemicals interact molecularly with the enzyme acetyl cholinesterase (PDB ID: 7e3h) (Figure 1) (Hsu, 2011).
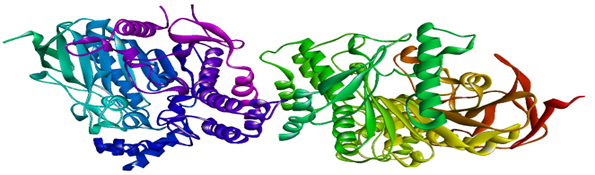
Figure 1:
Cleaned Acetylcholinesterase Enzyme PDB ID: 7e3h.
Discovery Studio Visualizer (Biovia)
The ligand interactions were observed and examined using an accurate docking approach following the conventional docking protocol. The scoring function was utilized to evaluate the best docking solutions by integrating electrostatic, hydrogen bonding and van der Waals energies. Post-docking interaction profile analysis of the best poses for the top compounds revealed the interactions between the ligand and the target protein. These interactions were visualized and analyzed using Discovery Studio Visualizer (Biovia).
In silico toxicity prediction was utilized to identify safe and non-carcinogenic chemicals. These compounds were chosen for molecular docking in addition to common Acetyl choline esterase inhibitors such as Galantamine and Rivastigmine. Docking simulations were used to evaluate molecular interactions and binding affinities. Out of the top 10 compounds with the best binding energies, the top two compounds with the best binding energies and molecular interaction profile were selected for post-docking interaction study (Vijaya et al., 2023; Peitzikaet al., 2023; Vijaya et al., 2023; Gudiseet al., 2024).
RESULTS
DISCUSSION
When compared to standard inhibitors like galantamine, which has a binding energy of -96.45 k.cal/mol and rivastigmine, which has a binding energy of -89.81 k.cal/mol, compound 8d and compound 9c have binding energies of -115.75 k.cal/mol and -113.08 k.cal/mol, respectively as depicted in Table 1, Figure 2. The binding energies of nearly all of the top 10 compounds have greater binding energies than standard Acetylcholineesterase inhibitors. Therefore, the binding energies of these two compounds are greater and hence selected for visualization.
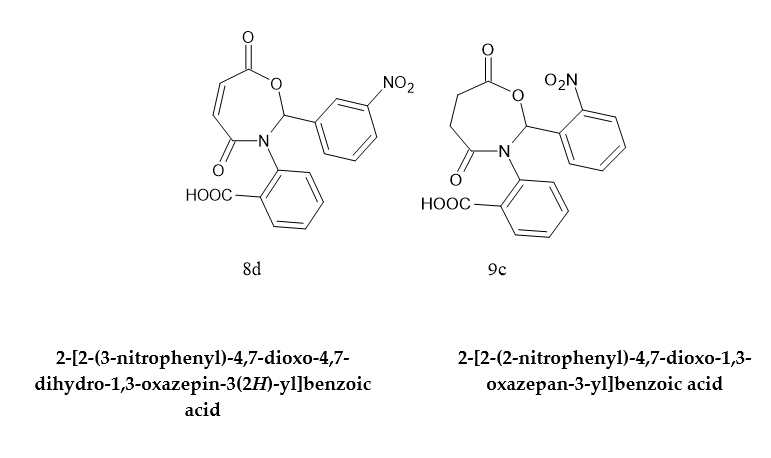
Figure 2:
Top 2 ligands having better binding affinity.
Ligands | Energies | Amino acid residues interaction |
---|---|---|
8d | -115.75 | GLY:121, TRP:86, TYR:124, ASPP:74, GLY:448, HIS:447, GLU:202, ALA:204, GLY:120, SER:203, GLY:122, PHE:338, TYR:341, VAL:73, TYR:72, ASN:87, TYR:337, SER:125. |
9c | -113.08 | SER: 125, TYR: 124, TYR: 337, PHE: 338, TRP: 86. |
8e | -109.66 | SER:203, GLY:121, TRP:86, TYR:72, ASP:74, SER:125, TYR:124, GLU:202, GLY:122, HIS:447, GLY:448, GLU:120, ALA:204, PHE:297, PHE:338, TYR:341, VAL:73, ASN:87, GLY:126. |
7d | -109.53 | TYR: 341, TRP: 86, ASP: 74, GLU: 202, TYR: 124. |
9f | -107.56 | SER: 125, TYR: 124, TYR: 337, TRP: 86. |
9i | -103.45 | SER:125, TYR:337, TRP:86, TYR:124, HIS:447, ASN:87, ASP:74, THR:83, TYR:341, GLY:448, PHE:338, PHE:297, GLY:122, GLY:121, TYR:133, GLY:120, GLY:126. |
9e | -103.28 | SER:203, GLY:122, PHE:297, TRP:86, SER:125, THR:83, ASP:74, TYR:341, TYR:337, TYR:124, ALA:204, PHE:295, PHE:338, GLY:121, GLY:120, HIS:447, GLY:126. |
8j | -101.95 | TYR: 124, TYR: 341, TYR: 337, TRP: 86, GLU: 202, ASP: 74. |
7j | -100.92 | TYR:124, PHE:297, TRP:86, LEU:130, TYR:341, ASP:74, PHE:338, TYR:337, GLY:122, SER:203, HIS:447, GLY:448, GLU:202, GLY:121, GLY:120, TYR:119, SER:125, GLY:126, ALA:127, ASN:87, TYR:72. |
9d | -100.25 | SER:125, TYR:337, TYR:341, SER:203, GLY:122, TRP:86, HIS:447. |
CO-CRYSTALIZED LIGAND | -96.99 | SER:203, TYR:337, PHE:338, SER:125, GLU:202, VAL:73, TRP:86, PHE:295, PHE:297, HIS:447. |
RIVASTIGMINE | -89.91 | THR:75, LEU:76, TRP:286, TYR:341, SER:293. |
GALANTAMINE | -96.45 | PHE:338, TRP:86, HIS:447, TYR:133, GLU:202, GLY:121. |
Interactions and binding energies of the top 10 Ligands with the enzyme Acetylcholinesterase.
The co-crystallized ligand and the top two ligands were compared for structural similarity during the visualization process. The number of conventional hydrogen bonds was displayed in a three-dimensional interaction model. The 2D interaction diagram clearly illustrated which amino acid residues were interacting with the ligands and their respective distances from the active site pocket which can be observed in Table 2. In this study focusing on acetylcholinesterase enzyme inhibitors, several ligands were investigated for their interactions with the enzyme through hydrogen bonding. The findings reveal distinct patterns among the inhibitors studied.
8d LIGAND 3D INTERACTIONS | 8d LIGANDS 2D INTERACTIONS |
9C LIGAND 3D INTERACTIONS | 9C LIGAND 2D INTERACTIONS |
CO-CRYSTALIZED LIGAND 3D INTERACTION | CO-CRYSTALIZED LIGAND 2D INTERACTION |
GALANTAMINE 3D INTERACTION | GALANTAMINE 2D INTERACTION |
RIVASTIGMINE 3D INTERACTIONS | RIVASTIGMINE 2D INTERACTIONS |
3D AND 2D interactions of top ligands and standard ligands.
Firstly, Ligand 8d was observed to form a single conventional hydrogen bond with the amino acid Glycine at position 121 (GLY: 121) of the enzyme’s active site. This interaction suggests a specific binding mode that potentially influences the inhibitor’s efficacy. Conversely, Ligand 9C exhibited a more complex interaction profile, establishing three conventional hydrogen bonds with key residues on the enzyme: Serine 125 (SER:125), Tyrosine 124 (TYR:124) and Tyrosine 337 (TYR:337). Such multiple interactions likely contribute to a robust binding affinity, possibly enhancing the inhibitor’s potency. The cocrystalized Ligand 2, on the other hand, formed two hydrogen bonds with Serine 203 (SER:203) and Tyrosine 337 (TYR:337). This binding pattern, shared with another ligand, suggests a common mode of interaction that may be critical for stabilizing the inhibitor within the enzyme’s active site. Among the standard acetyl cholinesterase enzyme inhibitors studied, Rivastigmine demonstrated interactions with Threonine 75 (THR:75) and Leucine 76 (LEU:76) through two hydrogen bonds. These specific interactions highlight key residues involved in Rivastigmine’s binding, potentially contributing to its effectiveness as an inhibitor. Interestingly, Galantamine did not form any hydrogen bonds with the enzyme under these experimental conditions, suggesting an alternative mode of interaction or binding mechanism that does not rely on direct hydrogen bonding interactions. Overall, these findings provide valuable insights into the molecular interactions between acetyl cholinesterase inhibitors and their target enzyme, shedding light on the structural basis for their inhibitory activity. Such detailed understanding is crucial for the rational design and optimization of future enzyme inhibitors aimed at treating conditions such as Alzheimer’s disease.
CONCLUSION
As the top ligands 8d and 9c were non-toxic, having better ADME, better binding affinities and common amino acid residue interactions with that of the standard Acetylcholine esterase inhibitors, they can be further synthesized and used for in vivo studies.
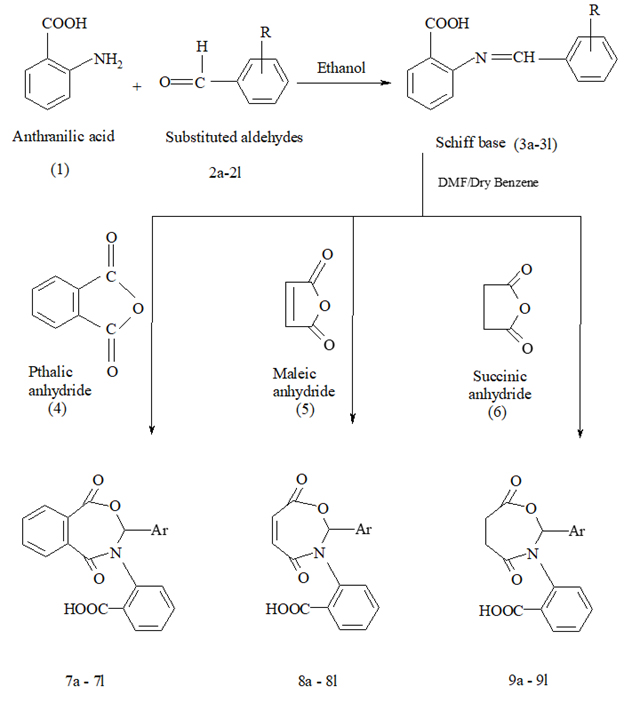
Scheme:
Synthesis of oxazepine derivatives.
Cite this article:
Addanki A, Nadendla R, Kanakaraju VK. Computational Docking Study of Oxazepine Based Compounds as Inhibitors of Acetylcholinesterase Enzyme in Alzheimer’s Disease. Int. J. Pharm. Investigation. 2025;15(2):1-8.
ACKNOWLEDGEMENT
The authors of this article would like to extend their sincere gratitude to the management of Chalapathi Institute of Pharmaceutical Sciences for providing the essential laboratory facilities.
ABBREVIATIONS
AD | Alzheimer’s disease |
---|---|
DMF | Dimethyl formamide |
mL | Millilitre |
kcal/mol | Kilocalorie per mole |
GABA | Gamma-aminobutyric acid |
PDB | Protein Data Bank |
References
- Abduljelil A., Uzairu A., Shallangwa G., Abechi S.. (2022) In silico design, molecular docking and pharmacokinetics studies of some tacrine derivatives as anti-Alzheimer agents: Theoretical investigation. Adv. J. Chem. Sect. A 5: 59-69 Google Scholar
- Abdullatieef M.. (2010) [Unpublished PhD thesis]. College of Education for Pure Science Ibn-ALHaitham, Baghded University Google Scholar
- Aljohani A. S. M., Alhumaydhi F. A., Rauf A., Hamad E. M., Rashid U.. (2022) In vivo anti‐inflammatory, analgesic, sedative, muscle relaxant activities and molecular docking analysis of phytochemicals from Euphorbia pulcherrima. Evidence-Based Complementary and Alternative Medicine: eCAM 2022: Article 7495867 Google Scholar
- Al-Juburi R. M.. (2012) Synthesis and characterization of some heterocyclic compounds (oxazepine, tetrazole) derived from Schiff bases. Journal of Al-Nahrain University Science 15: 60-67 Google Scholar
- Ayad K., Israa B., Hyder J.. (2015) Synthesis, characterization of some new azo compounds containing 1,3-oxazepine, anthraquinone moieties and studying their activity against pathogenic bacteria. Journal of Natural Sciences Research 15: 69-80 Google Scholar
- Daina A., Michielin O., Zoete V.. (2017) SwissADME: A free web tool to evaluate pharmacokinetics, drug-likeness and medicinal chemistry friendliness of small molecules. Scientific Reports 7: Article 42717 Google Scholar
- Daina A., Michielin O., Zoete V.. (2019) SwissTarget Prediction: Updated data and new features for efficient prediction of protein targets of small molecules. Nucleic Acids Research 47: W357-W364 Google Scholar
- Gudise P., Thasleema S. C., Podila N., Yazdan S. K., Yanadaiah J. P., Krishnaveni K., et al. (2024) Molecular docking studies of Schiff based derivatives against adenosine A2a receptor as potential anti parkinsonian agents. African Journal of Biological Sciences 6: 166-180 Google Scholar
- Hanoon H. D.. (2011) Synthesis and characterization of new seven-membered heterocyclic compounds from reaction of new Schiff bases with maleic and phthalic anhydrides. National Journal of Chemistry 41: 44-89 Google Scholar
- Hanwell M. D., Curtis D. E., Lonie D. C., Vandermeersch T., Zurek E., Hutchison G. R., et al. (2012) Avogadro: An advanced semantic chemical editor, visualization and analysis platform. Journal of Cheminformatics 4: 17 Google Scholar
- Hsu K.. (2011) chen yf, lin sr, yang jm. igemdock: A graphical environment of enhancing gemdock using pharmacological interactions and post-screening analysis. BMC Bioinformatics 12: 1-1 Google Scholar
- Hussain M. S., Azam F., Eldarrat H. A., Alkskas I., Mayoof J. A., Dammona J. M., Ismail H., Ali M., Arif M., Haque A., et al. (2020) Anti-inflammatory, analgesic and molecular docking studies of Lanostanoic acid 3-O-α-D-glycopyranoside isolated from Helichrysum stoechas. Arabian Journal of Chemistry 13: 9196-9206 Google Scholar
- Kanakaraju V. K., Rahaman Sk. A.. (2023a) Ravi chandra sekhara reddy danduga. Molecular Docking Studies of, 12(regular issue 6):2641-53, 2-amino–4, 6- disubstituted pyridine-3-carbonitriles against monoamine oxidase -b as potential anti parkinsonian agents eur. chem. bull. Google Scholar
- Kanakaraju V. K., Rahaman Sk. A.. (2023b) Ravi chandra sekhara reddy danduga Molecular docking studies of 2-amino-4,6- disubstituted pyridine-3-carbonitriles against adenosine A2a receptor as potential anti parkinsonian agents eur. Chemistry Bulletin 12: 5767-5776 Google Scholar
- Mesripour A., Jafari E., Hajibeiki M. R., Hassanzadeh F.. (2023) Design, synthesis, docking and antidepressant activity evaluation of isatin derivatives bearing Schiff bases. Iranian Journal of Basic Medical Sciences 26: 438-444 Google Scholar
- Moawad E. B.. (1989) Synthesis of some new heterocyclic compounds with expected potential biological activity. Journal of Islamic Academy of Sciences 2: 237-240 Google Scholar
- Muhammad N., Khan R., Seraj F., Khan A., Ullah U., Wadood A., Ajmal A., Uzma , Ali B., Khan K. M., Ain Nawaz N. U., AlMasoud N., Alomar T. S., Rauf A., et al. (2024) In vivo analgesic, anti-inflammatory and molecular docking studies of S-naproxen derivatives. Heliyon 10: Article e24267 Google Scholar
- Nagham M.. (2013) Preparation and identification of macrocycles of oxazepine compounds. Journal of Scientific and Innovative Research 2: 53-60 Google Scholar
- Nordberg A., Svensson A. L.. (1998) Cholinesterase inhibitors in the treatment of Alzheimer’s disease: A comparison of tolerability and pharmacology. Drug Safety 19: 465-480 Google Scholar
- Peitzika S.-C., Pontiki E.. (2023) A review on recent approaches on molecular docking studies of novel compounds targeting acetylcholinesterase in Alzheimer disease. Molecules 28: 1084 Google Scholar
- Prival M. J.. (2001) Evaluation of the TOPKAT system for predicting the carcinogenicity of chemicals. Environmental and Molecular Mutagenesis 37: 55-69 Google Scholar
- Qi Y., Chen H., Chen S., Shen J., Li J.. (2022) Synthesis, bioactivity and molecular docking of novel arylpiperazine derivatives as potential AR antagonists. Frontiers in Chemistry 10: Article 947065 Google Scholar
- Serrano-Wu M. H., St Laurent D. R., Chen Y., Huang S., Lam K.-R., Matson J. A., Mazzucco C. E., Stickle T. M., Tully T. P., Wong H. S., Vyas D. M., Balasubramanian B. N., et al. (2002) Sordarin oxazepine derivatives as potent antifungal agents. Bioorganic and Medicinal Chemistry Letters 12: 2757-2760 Google Scholar
- Siddiqui A. A., Partap S., Khisal S., Yar M. S., Mishra R.. (2020) Synthesis, anti-convulsant activity and molecular docking study of novel thiazole pyridazinone hybrid analogues. Bioorganic Chemistry 99: Article 103584 Google Scholar
- Silva M. V. F., Loures C. M. G., Alves L. C. V., De Souza L. C., Borges K. B. G., Carvalho M. D. G., et al. (2019) Alzheimer’s disease: Risk factors and potentially protective measures. Journal of Biomedical Science 26: 33 Google Scholar
- Vasudha D., Jagadeesh A., Mali S. N., Bhandare R. R., Shaik A. B.. (2024) Synthesis, moleculardockingandpharmacologicalevaluationsofnovelnaphthalene-pyrazoline hybrids as new orally active anti-inflammatory agents. Chemical Physics Impact 8: Article 100500 Google Scholar
- Verma P., Gupta S., Yadav V. S.. (2015) Catalyst-free and facile green synthesis of some novel oxazepine derivatives. Der Chemica Sinica 6: 86-89 Google Scholar
- Wilson C. O., Gisvold O.. (1966) Ptiman Medical Publishing Co. London by (5 ed.) Google Scholar
- Yao X., Hu H., Wang S., Zhao W., Song M., Zhou Q., et al. (2021) Synthesis, antimicrobial activity and molecular docking studies of aminoguanidine derivatives containing an acylhydrazone moiety. Iranian Journal of Pharmaceutical Research 20: 536-545 Google Scholar