ABSTRACT
Background
The study focuses on the synthesis of novel 4-Hydrazinyl-7H-Pyrrolo[2,3-d] Pyrimidine (HPP) derivatives using microwave-assisted synthesis starting from substituted benzaldehydes (a-j) and HPP in the presence of a strong acid.
Materials and Methods
The synthesized compounds were validated through mass spectrometry, 1H NMR and infrared spectroscopy. The antibacterial activity was tested using the disc diffusion method against two gram-positive bacteria (Staphylococcus aureus MCC 2010 and Bacillus subtilis MCC 2010) and two gram-negative bacteria (Pseudomonas aeruginosa MCC 2080 and Escherichia coli MCC 2412). Dimethylformamide (DMF) was used as a control, with standard antibiotics serving as the positive control.
Results
The antimicrobial screening revealed that all synthesized compounds exhibited significant antibacterial activity. Notably, several compounds displayed greater activity compared to the reference drugs used in the study.
Conclusion
The microwave-assisted synthesis of HPP derivatives from substituted benzaldehydes yielded compounds with promising antibacterial properties, surpassing the effectiveness of some standard antibiotics.
INTRODUCTION
Nitrogen heterocycles are a significant class of natural and manufactured compounds, many of which have advantageous biological features. Antibacterial (Tyagiet al., 2023) anti-inflammatory (Košaket al., 2020), and anti-tumor (Sabtet al., 2020) are only a few of the several biological activities exhibited by pyrazoles and their derivatives. The antibacterial properties of several nitrogen heterocycles have been documented in the literature. These include quinolines, imidazolidine acridines, pyrimidines, phenothiazines, purines, pyrazolo [3,4b] pyridine, bis-benzamidines and benzothiazoles (Agarwalet al., 2009).
The field of inorganic synthetic chemistry heavily uses pyrimidine and its byproducts. Pyrimidine does not naturally occur in its pure form but is extensively dispersed in several derivative forms. Pyrimidine derivatives have garnered significant attention in the field of pharmacology due to their diverse range of pharmacological properties. These properties include antitumor, antiviral, antifungal, anticancer, antibacterial, anti-inflammatory, analgesic, antagonist, antifolate, antimicrobial, anti-HIV, antiproliferative, antiplatelet and antithrombotic activities, as well as ant filarial effects (Oukoloffet al., 2018; Behalo, 2018; Bernatet al., 2020; Pasupathi and Santhi, 2019; Sarode and Bhole, 2019; Huoet al., 2021; Gomhaet al., 2018; Patilet al., 2018; Karouiet al., 2014). Furthermore, aromatic aldehydes (Maharramovet al., 2017; Sondhiet al., 1999; Okudaet al., 2015) are considered important pharmacodynamics and have demonstrated a broad range of actions when integrated into various heterocyclic frameworks.
Considering these facts, the main objective of this paper is to synthesize new HPP derivatives with substituted benzaldehydes that contain an active azomethine group. The azomethine group’s nitrogen can be replaced with thiazole, pyrimidine, or quinoxaline groups, which have been shown to have antimicrobial properties (Wilcoxenet al., 2002; Mosleyet al., 2010; Liet al., 2018; Masaret and Farghaly, 2018).
MATERIALS AND METHODS
Experimental Design
Chemistry
The S-Lab Junction is off. The melting points were determined using the Boiling Point Apparatus and the Melting Point Calculator. Microanalytical laboratories at Pune University conducted elemental analysis (C, H and N) using the Carlo Erba 1108 Elemental Analyzer. Each compound was within 0.4% of what was anticipated. A BRUKER IR 100 spectrophotometer collected the infrared measurements (KBr). DMSO-d6 was used as the solvent for the 1H NMR spectra, while TMS served as the internal standard. On a JASCO V650 spectrophotometer, UV spectra were recorded using methanol solvent at room temperature. All reactions were monitored using Thin-Layer Chromatography (TLC), Merck 60 F254 silica gel, a UV lamp and pre-coated aluminum sheets.
Substituted benzaldehyde derivatives of HPP (2a-j)
HPP (1) (2 mmol), substituted benzaldehydes a-j (2 mmol), CuI (0.2 mmol) and DMSO (4.0 mL) are added to a 20 mL process vial that is equipped with a stirring bar. After that, the mixture had been stirred for 1 min. A Teflon septum contained within an aluminum crimp top was used to cap the vial, thereby sealing it. After being subjected to microwave radiation for 2 hr at 100ºC, the mixture was allowed to cool down to room temperature. After pouring the contents of the vial into 20 mL of water, they are extracted with 3×25 mL of EtOAc. The mixed organic layers are then washed with 20 mL of NaCl-saturated solution and 20 mL of water. Finally, they are dried over Na2SO4 and evaporated under reduced pressure. The intended HPP derivatives were generated by flash chromatography of the residue, which consisted of silica, 3:1 ether and light petroleum.
(E)-4-(2-(4-methoxy-3-hydroxybenzylidene) hydrazinyl)-7H-pyrrolo[2,3-d]pyrimidine (2a)
% Yield: 72.44. MP (ºC):186. IR (KBr, cm-1): 3424 (-OH), 3268 (-CH3),3179 (Ar. NH), 3115 (Ali NH), 1590/1442 (C=C), 1526 (C=N), 1037 (N-N), 741 (Benzene ring). 1H NMR (DMSO, 400 MHz) : 13.19 (1H, s, -OH), 12.96 (1H, s,Ali. NH), 9.66 (1H, s,Ar-NH), 8.60 (1H, s, -CH=), 8.49 (1H, d, Ar-H, J=8.60Hz),7.77 (1H, d, Ar-H, J=8.17Hz), 7.59 (1H, d, Ar-H, J=8.33Hz), 7.11-7.13 (1H, d, Ar-H, J=3.73Hz), 6.92-6.96 (2H, dd, Ar-H, J=8.55, 1.44Hz), 3.88 (3H, s, -OCH3). Mass (m/z): 284.62.
(E)-4-(2-(3-methoxy-4-hydroxybenzylidene) hydrazinyl)-7H-pyrrolo[2,3-d]pyrimidine (2b)
% Yield: 69.26. MP (ºC):190. IR (KBr, cm-1): 3256 (-OH), 3136 (Ar. NH), 3036 (Ali NH), 1588/1443 (C=C), 1662 (C=N), 1005 (N-N), 759 (Benzene ring). 1H NMR (DMSO, 400 MHz) : 13.56 (1H, s, -OH), 13.15 (1H, s,Ali. NH), 12.96 (1H, s,Ar-NH), 9.84 (1H, s, -CH=), 8.50 (1H, d, Ar-H, J=8.60Hz), 8.38 (1H, d, Ar-H, J=1.36Hz), 7.68 (1H, d, Ar-H, J=1.95Hz), 7.59 (1H, d, Ar-H, J=3.26Hz), 7.37-7.40 (1H, dd, Ar-H, J=3.85Hz),6.92-6.97 (2H, dd, Ar-H, J=8.39, 0.44Hz), 3.76 (3H, s, -OCH3). Mass (m/z): 283.91.
(E)-4-(2-(2-methoxy-4-hydroxybenzylidene) hydrazinyl)-7H-pyrrolo[2,3-d]pyrimidine (2c)
% Yield: 77.03. MP (ºC):187. IR (KBr, cm-1): 3193 (-OH), 3087 (Ar. NH), 2980 (Ali NH), 2955 (-CH=), 1589/1441 (C=C), 1680 (C=N), 1005 (N-N), 776 (Benzene ring). 1H NMR (DMSO, 400 MHz) : 11.92 (1H, s, -OH), 11.29 (2H, s,NH), 8.60 (1H, s, -CH=), 8.29-8.31 (2H, dd, Ar-H, J=8.44, 1.94Hz), 7.77-7.83 (2H, dd, Ar-H, J=1.92, 0.43Hz), 7.40 (1H, d, Ar-H, J=3.73Hz), 6.83 (1H, dd, Ar-H, J=8.39, 0.43Hz), 3.37 (3H, s, -OCH3). Mass (m/z): 282.55.
(E)-4-(2-(3-chlorobenzylidene)hydrazinyl)-7H-pyrrol o[2,3-d]pyrimidine (2d)
% Yield: 79.04. MP (ºC):183. IR (KBr, cm-1): 3290 (Ar. NH), 3126 (Ali NH), 1584/1439 (C=C), 1628 (C=N), 1010 (N-N), 734 (Benzene ring). 1H NMR (DMSO, 400 MHz) : 13.01 (1H, s, Ali. NH), 8.69 (1H, s, Ar-NH), 8.59(1H, s, -CH=), 7.53-8.49 (7H, m, Ar-H). Mass (m/z): 270.33.
(E)-4-(2-(2,3-diclorobenzylidene) hydrazinyl)-7H-pyrrolo[2,3-d]pyrimidine (2e)
% Yield: 78.10. MP (ºC):187. IR (KBr, cm-1): 3189 (Ar. NH), 3072 (Ali NH), 2978 (-CH=), 1588/1444 (C=C), 1570 (C=N), 1040 (N-N), 879 (C-Cl), 715 (Benzene ring). 1H NMR (DMSO, 400 MHz) : 11.85 (2H, s, -NH), 8.62 (1H, s, -CH=), 8.29 (1H, dd, Ar-H, J=7.69, 1.55Hz), 8.05-8.07 (1H, d, Ar-H, J=3.71Hz), 7.69-7.71 (1H, dd, Ar-H, J=7.49, 7.69Hz), 7.51-7.55 (1H, dd, Ar-H, J=7.73, 7.16Hz), 7.34 (1H, dd, Ar-H, J=7.61, 1.66Hz), 6.93 (1H, d, Ar-H, J=3.56). Mass (m/z): 305.87.
(E)-4-(2-(2,4-diclorobenzylidene) hydrazinyl)-7H-pyrrolo[2,3-d]pyrimidine (2f)
% Yield: 77.12. MP (ºC):188. IR (KBr, cm-1): 3186 (Ar. NH), 3070 (Ali NH), 2973 (-CH=), 1589/1445 (C=C), 1573 (C=N), 1022 (N-N), 878 (C-Cl), 710 (Benzene ring). 1H NMR (DMSO, 400 MHz) : 11.85 (2H, s, -NH), 8.62 (1H, s, -CH=), 8.29 (1H, dd, Ar-H, J=7.69, 1.55Hz), 8.05-8.07 (1H, d, Ar-H, J=3.71Hz), 7.69-7.71 (1H, dd, Ar-H, J=7.49, 7.69Hz), 7.51-7.55 (1H, dd, Ar-H, J=7.73, 7.16Hz), 7.34 (1H, dd, Ar-H, J=7.61, 1.66Hz), 6.93 (1H, d, Ar-H, J=3.56). Mass (m/z): 305.84.
(E)-4-(2-(2,5-diclorobenzylidene)hydrazinyl )-7H-pyrrolo[2,3-d]pyrimidine (2g)
% Yield: 72.55. MP (ºC):193. IR (KBr, cm-1): 3190 (Ar. NH), 3084 (Ali NH), 2975 (-CH=), 1597/1442 (C=C), 1570 (C=N), 1042 (N-N), 856 (C-Cl), 756 (Benzene ring). 1H NMR (DMSO, 400 MHz) : 11.93 (2H, s, -NH), 8.55 (1H, s, Ar-H), 8.31 (1H, s, -CH=), 8.00 (1H, dd, Ar-H, J=1.61, 0.50Hz), 7.61-7.63 (1H, dd, Ar-H, J=8.06, 0.51Hz), 7.52 (1H, d, Ar-H, J=3.73Hz), 7.41 (1H, dd, Ar-H, J=8.10, 1.59Hz), 6.86(1H, d, Ar-H, J=3.69Hz). Mass (m/z): 306.07.
(E)-4-(2-(2,6-diclorobenzylidene) hydrazinyl)-7H-pyrrolo[2,3-d]pyrimidine (2h)
% Yield: 82.68. MP (ºC):186. IR (KBr, cm-1): 3355 (Ar. NH), 3188 (Ali NH), 2982 (-CH=), 1581/1481 (C=C), 1650 (C=N), 1025 (N-N), 859 (C-Cl), 730 (Benzene ring). 1H NMR (DMSO, 400 MHz) : 14.53 (1H, s, -NH Ali), 13.07 (1H, s, -NH Ar), 8.69 (1H, s, Ar-H), 8.51(1H, s, -CH=), 8.12 (2H, dd, Ar-H, J=8.22, 1.19Hz), 7.68 (1H, d, Ar-H, J=3.65Hz), 7.58 (1H, t, Ar-H, J=8.11Hz), 7.27 (1H, d, Ar-H, J=3.90Hz). Mass (m/z): 307.98.
(E)-4-(2-(3,4-diclorobenzylidene) hydrazinyl)-7H-pyrrolo[2,3-d]pyrimidine (2i)
% Yield: 74.51. MP (ºC): 193. IR (KBr, cm-1): 3355 (Ar. NH), 3188 (Ali NH), 2982 (-CH=), 1581/1481 (C=C), 1650 (C=N), 1025 (N-N), 859 (C-Cl), 730 (Benzene ring). 1H NMR (DMSO, 400 MHz) : 14.53 (1H, s, -NH Ali), 13.07 (1H, s, -NH Ar), 8.69 (1H, s, Ar-H), 8.51(1H, s, -CH=), 8.12 (2H, dd, Ar-H, J=8.22, 1.19Hz), 7.68 (1H, d, Ar-H, J=3.65Hz), 7.58 (1H, t, Ar-H, J=8.11Hz), 7.27 (1H, d, Ar-H, J=3.90Hz). Mass (m/z): 307.98.
(E)-4-(2-(3,5-diclorobenzylidene) hydrazinyl)-7H-pyrrolo[2,3-d]pyrimidine (2j)
% Yield: 74.50. MP (ºC): 196. IR (KBr, cm-1): 3191 (Ar. NH), 3086 (Ali NH), 2974 (-CH=), 1591/1437 (C=C), 1708 (C=N), 1085 (N-N), 859 (C-Cl), 715 (Benzene ring). 1H NMR (DMSO, 400 MHz) : 11.78-11.84 (2H, s, -NH), 8.46(1H, s, Ar-H), 8.31(1H, s, -CH=), 7.75 (2H, dd, Ar-H, J=1.87, 1.61Hz), 7.44-746 (1H, d, Ar-H, J=3.88Hz), 7.29 (1H, t, Ar-H, J=1.63Hz), 6.94 (1H, d, Ar-H, J=3.76Hz). Mass (m/z): 306.23.
Antimicrobial evaluation
Many different fungal, bacterial and synthetic compounds were used, all of which were purchased as pure, dry powder specimens (clinical isolates). Among the fungus strains were Candida albicans MCC1439 and Saccharomyces cerevisiae MCC1033. Before being subcultured on antimicrobial-free potato dextrose agar, the mold isolates were maintained alive and undamaged in sterile water. Bacillus subtilis and Staphylococcus aureus were among the Gram-positive bacteria, whereas Escherichia coli and Pseudomonas aeruginosa were among the Gram-negative bacteria. We acquired the organisms from the National Centre for Molecular Research in Pune, India.
Dimethyl Formamide (DMF), in which the compounds were dissolved for storage at 4ºC, had no antibacterial effects at the concentrations used in the experiments. Bacteria are cultured in nutritional broth (NB; Difco) and Nutrient Agar (NA), whereas fungi are grown on potato dextrose agar and sabouraud liquid media.
Antibacterial screening
The gram-positive (Staphylococcus aureus MCC 2010) and gram-negative (Bacillus subtilis MCC 2010) bacteria were used to evaluate the compounds’ antibacterial activity. (Escherichia coli MCC 2412 and Pseudomonas aeruginosa MCC 2080). For antimicrobial testing, we autoclaved Muller Hilton agar medium at 15 lbs/in 2 for 15 min to eliminate any bacteria. Newly developed medicines’ antibacterial efficacy was measured by disc diffusion (Costaet al., 2022). To test for antibacterial activity, the inoculum was diluted by floating the culture in sterile distilled water until there were less than 108 cfu/mL. Cultures of the desired microorganisms were swabbed and placed on Petri dishes with 20 mL of Muller Hilton agar media. After pre-inoculation, plates were cleaned and perforated to a diameter of 6 mm. Then, 100 L of a DMSO-reconstituted solution containing a 4.0 mg/mL solution of each chemical was added. The plates were kept at 37ºC for 24 hr for incubation. The plates were kept at 37ºC for 24 hr for incubation. To evaluate the antibacterial efficacy of each synthetic medication, the inhibition zone surrounding the wells was utilised. A negative control was DMF, while a positive control was streptomycin (Maddila and Jonnalagadda, 2012).
Antifungal Activity
Disc diffusion testing was used to determine how well each compound worked against two different types of fungus (Okudaet al., 2015; Wilcoxenet al., 2002). (Yeast strains S. cerevisiae MCC1033 and Candida albicans MCC1439). The test solution was injected into the 5 mm diameter and 1 mm thick discs using a micropipette. We continued to leave the dishes at 37 ºC for an additional 24 hr after that (Brulloet al., 2020). Throughout this period, the test solution disseminated and impeded the injected fungus’s growth. The diameter of the inhibition was measured after 36 hr of incubation at 37ºC. Research on compounds with potential antifungal effects employed minimum inhibitory doses (Kumaret al., 2016). The MIC of a fungal agent was determined by determining the concentration of the medicine needed to stop the microbe from growing further after overnight incubation. Clinical laboratories used the MIC to confirm that microbes were resistant to antimicrobial medications and to assess how well new antimicrobial medications worked (Sharmaet al., 2022).
RESULTS
As shown in Scheme 1, the target compounds were synthesized using the following procedures. Within the scope of this research, the interaction between substituted methoxy-and chloro-benzaldehydes and HPP was examined. To completely define each organic component, mass spectrum analyses were also used. To completely define each organic component, mass spectrum analyses were also utilized. The molecular weights of the structures that predicted using the (M)+ ion were compatible with the molecular ion peaks of every organic molecule.
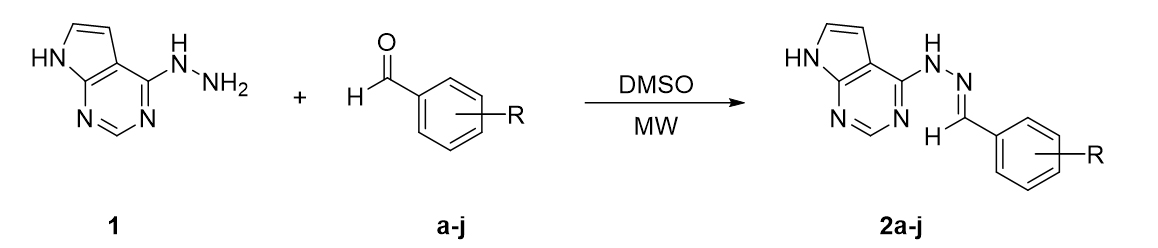
Scheme 1:
Preparation of compounds 2a-j.
FTIR spectra
A band with a wavelength range of 3189-3424 cm-1 that, when observed, denotes the presence of an aromatic amine group (-NH-) (Table 1). The IR analysis results reveal that certain functional groups are present in every molecule that was produced. The presence of -OH and C-Cl substitution groups is indicated by absorption at 3189-3424 cm-1 and 856-879 cm-1, respectively, in the produced compounds 2a-c and 2d-2j.
Comp | NH- aromatic) | NH- aliphatic) | -CH= | (>C=C<) | >C=NN- | -N-N- | Di sub Benz ring | C-Cl | -OH |
---|---|---|---|---|---|---|---|---|---|
2a | 3268 | 3179 | 3115 | 1590/1462 | 1526 | 1037 | 741 | – | 3424 |
2b | 3136 | 3036 | 3035 | 1586/1443 | 1662 | 1005 | 741 | – | 3256 |
2c | 3087 | 3087 | 2980 | 1589/1441 | 1680 | 1005 | 776 | – | 3193 |
2d | 3290 | 3126 | 2999 | 1585/1439 | 1628 | 1010 | 734 | 879 | – |
2e | 3072 | 2978 | 2970 | 1588/144 | 1570 | 1040 | 715 | 878 | – |
2f | 3186 | 3070 | 2973 | 1589/1445 | 1573 | 1022 | 710 | 856 | – |
2g | 3190 | 3084 | 2975 | 1597/1442 | 1570 | 1042 | 756 | 859 | – |
2h | 3355 | 3188 | 2982 | 1581/1481 | 1650 | 1025 | 730 | 859 | – |
2i | 3355 | 3188 | 2982 | 1581/1481 | 1650 | 1025 | 730 | 859 | – |
2j | 3191 | 3086 | 2974 | 1591/1437 | 1708 | 1085 | 715 | 859 | – |
FTIR spectral data of compounds 2a-2j.
1H NMR spectra
The synthesis of a pyrimidine ring at the synthesized compound can be inferred from the presence of 7.68-7.89 ppm of the 1H, s, pyrimidine isotope (Table 2). At the chemical that was produced, the presence of 8.50-9.66 ppm and 12.96-13.15 (1H, s, -NH-) indicated the existence of aliphatic and aromatic amine groups, respectively. The findings of 3.37-388 ppm point to the existence of a methoxy group in the molecule 2a-c that was produced. A second singlet peak in the 886-9.84 ppm range is ascribed to the aldehydic -CH= group in all of the produced compounds, while compounds 2a-c exhibit the -OH singlet peak in the 11.92-13.56 ppm range. All of the produced derivatives’ 1H NMR spectra lack the broad singlet signal at 9.84 ppm (2H), which corresponds to the -NH2 of HPP; this suggests that the amino group was successfully replaced by the Schiff base (Saifuddinet al., 2020) (Table 3).
Comp | -NH- aliphatic (s, 1H) | NH aromatic (s, 1H) | -CH= (s, 1H) | pyrimidine-H (s, 1H) | aromatic-H (m, 6H) | -OH |
---|---|---|---|---|---|---|
2a | 12.96 | 9.66 | 8.60 | 8.49 | 7.77-6.92 | 13.19 |
2b | 13.15 | 12.96 | 9.84 | 8.50 | 8.38-6.92 | 13.56 |
2c | 11.29 | 8.66 | 8.60 | 8.29 | 7.83-6.83 | 11.92 |
2d | 13.01 | 13.00 | 8.59 | 7.53 | 8.49-7.55 | – |
2e | 11.85 | 11.84 | 8.62 | 8.29 | 8.07-6.93 | – |
2f | 11.85 | 11.84 | 8.62 | 8.29 | 8.07-6.93 | – |
2g | 11.93 | 11.92 | 8.55 | 8.31 | 8.00-6.86 | – |
2h | 14.53 | 13.07 | 8.69 | 8.12 | 7.68-7.27 | – |
2i | 14.53 | 13.07 | 8.69 | 8.51 | 8.12-7.27 | – |
2j | 11.84 | 11.78 | 8.46 | 8.31 | 7.75-6.94 | – |
NMR spectral data of compounds 2a-2j.
Comp Code | MW | Formula | MP (oC) | Structure |
---|---|---|---|---|
2a | 283.27 | C14H13N5O3 | 186 | |
2b | 283.27 | C14H13N5O3 | 182 | |
2c | 283.27 | C14H13N5O3 | 187 | |
2d | 271.00 | C13H10ClN5 | 183 | |
2e | 306.15 | C13H9Cl2N5 | 187 | |
2f | 306.15 | C13H9Cl2N5 | 188 | |
2g | 306.15 | C13H9Cl2N5 | 193 | |
2h | 306.15 | C13H9Cl2N5 | 186 | |
2i | 306.15 | C13H9Cl2N5 | 193 | |
2j | 306.15 | C13H9Cl2N5 | 196 |
Structures, melting points and molecular weights of compounds 2a-j.
Antimicrobial evaluation
Candida albicans (MCC1439), Saccharomyces cerevisiae (MCC1033), Staphylococcus aureus (MCC 2010), Bacillus subtilis (MCC 2010), Escherichia coli (MCC 2412) and Pseudomonas aeruginosa (MCC 2080) were some of the organisms tested for antibacterial and antifungal activity in vitro.
The bacterial isolates were grown in nutritional broth at 37ºC for a duration of 24 hr. Fungal spore suspensions were prepared by incubating sabouraud dextrose agar at 25ºC for 24 hr and then storing it in malt broth. The fungi were allowed to multiply for 7 days before being harvested using tween 80. For fungus, the ideal inoculum OD was 0.5 while for bacteria, it was 0.2 to 0.3. At the concentrations examined, the bacterium is unaffected by the Dimethyl Sulfoxide (DMSO) utilized to prepare the stock solutions. At a concentration of 1000 µg/mL, bacterial and fungal populations each doubled. Powdered forms of antifungal and antibacterial drugs like streptomycin and fluconazole were widely used. After 24 hr at 37ºC, the antibacterial activity was assessed and the antifungal test was carried out after 48 hr at 25ºC.
Antibacterial activity
Streptomycin, a broad-spectrum antibiotic with a MIC of 50 µg/mL against the bacterial species, was used in the experiment. Suppression zones ranged in size from 6-10 mm for Escherichia coli (MCC 2412), 6-11 mm for Bacillus subtilis (MCC 2010), 0-13 mm for Pseudomonas aeruginosa (MCC 2080) and 8-14 mm for Staphylococcus aureus (MCC 2408). The zone of inhibition for the tested chemicals in Table 4 ranged from 50 to 250 µg/mL, indicating that all the microorganisms used in the experiment were susceptible.
Compound | Antibacterial Activity (zone of inhibition) | |||
---|---|---|---|---|
S. aureus | B. subtilis | E. coli | P. aeruginosa | |
2a | 11 | 6 | 9 | 7 |
2b | 10 | 7 | 10 | 0 |
2c | 8 | 7 | 11 | 0 |
2d | 8 | 8 | 10 | 9 |
2e | 6 | 13 | 9 | 10 |
2f | 8 | 7 | 14 | 9 |
2g | 6 | 6 | 11 | 8 |
2h | 7 | 11 | 11 | 10 |
2i | 7 | 9 | 8 | 7 |
2j | 7 | 10 | 8 | 8 |
Streptomycin | 8 | 8 | 8 | 7 |
Antibacterial studies of compounds 2a-2j.
Results showed that 2e was more successful than the reference medicine against P. aeruginosa, 2 g was less effective against S. aureus and 2f (14 mm) was more active against P. aeruginosa overall (Figure 1). Effective against E. coli were the 1d, 1e and 1g, while effective against B. subtilis were the 2e and 2h. Since bacteria and other pathogens have less robust lipophilic cell walls, they can penetrate cells with relative ease, resulting in the antibacterial effect. The reason is, gram-negative bacteria’s lipid cell membrane is permeable to the molecule because of its lipophilic alkyl chain. According to the data, antibacterial activity declines as the carbon chain length grows. One possible explanation is that the large molecular weight of the carbon chain makes it unable to cross the bacterial cell membrane (Phillips, 1949).
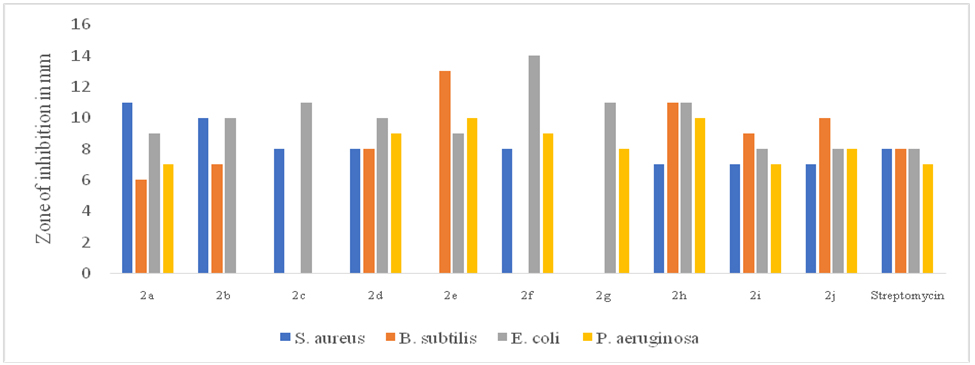
Figure 1:
Antibacterial activity of compounds 2a to 2j.
Antifungal activity
The fungi that were tested in this study had Minimum Inhibitory Concentrations (MICs) of 50 g/mL for fluconazole and the inhibition zones for Candida albicans (MCC1439) and Saccharomyces cerevisiae (MCC1033) were 10-19 mm and 11-19 mm, respectively (Figure 2). In comparison to the reference drug, all of the compounds in Table 5 showed promising fungicidal activity, with Minimum Inhibitory Concentrations (MICs) of 54 g/mL against Candida albicans (MCC1439) and Saccharomyces cerevisiae (MCC1033).
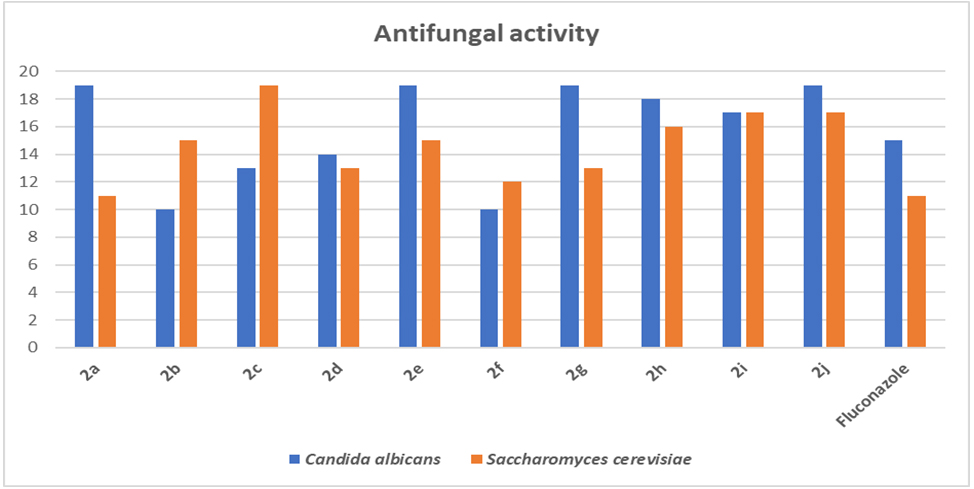
Figure 2:
Antifungal activity of compounds 2a to 2j.
Compound | Candida albicans | Saccharomyces cerevisiae |
---|---|---|
2a | 19.00 | 11.00 |
2b | 10.00 | 15.00 |
2c | 13.00 | 19.00 |
2d | 14.00 | 13.00 |
2e | 19.00 | 15.00 |
2f | 10.00 | 12.00 |
2g | 19.00 | 13.00 |
2h | 18.00 | 16.00 |
2i | 17.00 | 17.00 |
2j | 19.00 | 17.00 |
Fluconazole | 15.00 | 11.00 |
Antifungal studies of compounds 2a-2j.
DISCUSSION
The research successfully synthesized a series of novel 4-Hydrazinyl-7H-Pyrrolo[2,3-d] Pyrimidine (HPP) derivatives, as depicted in Scheme 1. The focus was on the interaction between substituted methoxy- and chloro-benzaldehydes with HPP under microwave-assisted conditions. This approach proved to be efficient, providing a straightforward method for synthesizing these derivatives.
Mass spectrometry, 1H NMR and FTIR spectral analyses were crucial in confirming the successful synthesis and structural integrity of the compounds. The mass spectral data, particularly the molecular ion peaks, were consistent with the predicted molecular weights of the synthesized derivatives. The FTIR spectra further supported the presence of key functional groups, such as the aromatic amine group (-NH-), observed at 3189-3424 cm-1 and the -OH and C-Cl substitutions, which were confirmed by absorption peaks at 3189-3424 cm-1 and 856-879 cm-1, respectively. The 1H NMR spectra provided additional evidence for the presence of the pyrimidine ring and other relevant functional groups, confirming the successful formation of the target compounds.
The synthesized compounds were evaluated for their antimicrobial properties against a range of bacterial and fungal strains. The antibacterial activity was particularly noteworthy, with compounds such as 2e, 2f and 2g showing significant activity against gram-negative bacteria like Pseudomonas aeruginosa and gram-positive bacteria like ss. The antimicrobial effectiveness of these compounds can be attributed to their ability to penetrate bacterial cell membranes, which is facilitated by the lipophilic nature of their alkyl chains. The observation that antibacterial activity decreases with increasing carbon chain length suggests that steric factors may hinder the ability of larger molecules to cross bacterial cell membranes.
The antifungal activity of the synthesized compounds was also promising, with several compounds displaying inhibition zones comparable to or better than the reference drug fluconazole. Compounds like 2e demonstrated superior activity against Candida albicans and Saccharomyces cerevisiae, indicating their potential as effective antifungal agents.
Overall, the study highlights the successful synthesis of novel HPP derivatives with potent antimicrobial properties. The findings suggest that these compounds, particularly those with optimal chain lengths, could serve as promising candidates for the development of new antibacterial and antifungal therapies. Further investigations, including in vivo studies and Structure-Activity Relationship (SAR) analyses, would be beneficial to fully understand the therapeutic potential and to optimize the efficacy of these compounds.
CONCLUSION
In this study, we synthesized numerous novel substituted HPP-benzaldehyde derivatives. Analytical data, FT-IR, UV-vis, NMR spectrum examinations and electrochemical data all support the production of the proposed compounds. Analysed and recorded were the FT-IR, elemental analysis (C, H, N, O), UV-vis and 1H/13C NMR spectra of the benzaldehyde-based compounds. It is recommended by the researchers that modified benzaldehydes and HPP be mixed in a 1:1 ratio. All synthetic materials demonstrated exceptional antibacterial activity.
Cite this article:
Kadam PD, Nagargoje GR, Bondge AS, Momin KI, Panchgalle SP, More VS Microwave-Assisted Synthesis of Benzaldehyde Derivatives and their Biological Activity. Int. J. Pharm. Investigation. 2025;15(2):10-8.
ACKNOWLEDGEMENT
SPP thanks Department of Science and Technology for funding to K. M. C. College Khopoli under DST-FIST scheme.
ABBREVIATIONS
HPP | 4-hydrazinyl-7H-pyrrolo[2,3-d]pyrimidine |
---|---|
DMSO | Dimethyl sulfoxide |
References
- Agarwal K. C., Sharma V., Shakya N., Gupta S.. (2009) Design and synthesis of novel substituted quinazoline derivatives as antileishmanial agents. Bioorganic and Medicinal Chemistry Letters 19: 5474-5477 Google Scholar
- Behalo M. S.. (2018) Synthesis of Some Novel thiazolo [3, 2‐a] pyrimidine and pyrimido [2, 1‐b][1, 3] thiazine Derivatives and their antimicrobial Evaluation. Journal of Heterocyclic Chemistry 55: 1391-1397 Google Scholar
- Bernat Z., Szymanowska A., Kciuk M., Kotwica-Mojzych K., Mojzych M.. (2020) Review of the Synthesis and Anticancer Properties of pyrazolo [4, 3-e][1, 2, 4] triazine Derivatives. Molecules 25: 3948 Google Scholar
- Brullo C., Massa M., Rapetti F., Alfei S., Bertolotto M. B., Montecucco F., Signorello M. G., Bruno O., et al. (2020) New hybrid pyrazole and imidazopyrazole antiinflammatory agents can reduce ROS production in different biological targets. Molecules 25: 899 Google Scholar
- Costa Feito A., González Fernández A. M., Cervantes Blanco M.. (2022) EEG-based consumer neuroscience and neuromarketing dataset Google Scholar
- Gomha S. M., Mohamed A. M. G., Zaki Y. H., Ewies M. M., Elroby S. A.. (2018) Structural Elucidation and antimicrobial Evaluation of Novel [1,2,4] triazolo [4,3‐a] pyrimidines and pyrido [2,3‐d][1,2,4] triazolo [4,3‐a] pyrimidinones. Journal of Heterocyclic Chemistry 55: 1147-1156 Google Scholar
- Huo X.-S., Jian X.-E., Ou-Yang J., Chen L., Yang F., Lv D.-X., You W.-W., Rao J.-J., Zhao P.-L., et al. (2021) Discovery of highly potent tubulin polymerization inhibitors: Design, synthesis and structure–activity relationships of novel 2,7-diaryl-[1,2,4]triazolo[1,5-a] pyrimidines. European Journal of Medicinal Chemistry 220: Article 113449 Google Scholar
- Karoui A., Allouche F., Deghrigue M., Agrebi A., Bouraoui A., Chabchoub F., et al. (2014) Synthesis and pharmacological evaluation of pyrazolopyrimidopyrimidine derivatives: Anti-inflammatory agents with gastroprotective effect in rats. Medicinal Chemistry Research 23: 1591-1598 Google Scholar
- Košak U., Strašek N., Knez D., Jukič M., Žakelj S., Zahirović A., Pišlar A., Brazzolotto X., Nachon F., Kos J., Gobec S., et al. (2020) N-alkylpiperidine carbamates as potential anti-Alzheimer’s agents. European Journal of Medicinal Chemistry 197: Article 112282 Google Scholar
- Kumar A., Chowdhury S. R., Sarkar T., Chakrabarti T., Majumder H. K., Jha T., Mukhopadhyay S., et al. (2016) A new bisbenzylisoquinoline alkaloid isolated from Thalictrum foliolosum, as a potent inhibitor of DNA topoisomerase IB of Leishmania donovani. Fitoterapia 109: 25-30 Google Scholar
- Li H., Fang F., Liu Y., Xue L., Wang M., Guo Y., Wang X., Tian C., Liu J., Zhang Z., et al. (2018) Inhibitors of dihydrofolate reductase as antitumor agents: Design, synthesis and biological evaluation of a series of novel nonclassical 6-substituted pyrido [3, 2-d] pyrimidines with a three- to five-carbon bridge. Bioorganic and Medicinal Chemistry 26: 2674-2685 Google Scholar
- Maddila S., Jonnalagadda S. B.. (2012) Synthesis and biological activity of ethyl 2‐(substituted benzylthio)‐4‐(3′‐(ethoxycarbonyl) biphenyl‐4‐yl)‐6‐methyl‐1, 4‐ dihydropyrimidine‐5‐carboxylate derivatives. Archiv der Pharmazie 345: 163-168 Google Scholar
- Maharramov A. M., Ramazanov M. A., Guliyeva G. A., Huseynzada A. E., Hasanova U. A., Shikhaliyev N. G., Eyvazova G. M., Hajiyeva S. F., Mamedov I. G., Aghayev M. M., et al. (2017) Synthesis, investigation of the new derivatives of dihydropyrimidines and determination of their biological activity. Journal of Molecular Structure 1141: 39-43 Google Scholar
- Masaret G. S., Farghaly T. A.. (2018) Synthesis of 8, 10-disubstituted- triazoloperimidines from (e)-3-(dimethylamino)-1-(8-phenyl-8h-[1, 2, 4] triazolo [4, 3-a] perimidin-10-yl) prop-2-en-1-one and their antimicrobial activity. Current Organic Synthesis 15: 126-136 Google Scholar
- Mosley C. A., Acker T. M., Hansen K. B., Mullasseril P., Andersen K. T., Le P., Vellano K. M., Bräuner-Osborne H., Liotta D. C., Traynelis S. F., et al. (2010) Quinazolin-4- one derivatives: A novel class of noncompetitive NR2C/D subunit-selective N-methyl-D-aspartate receptor antagonists. Journal of Medicinal Chemistry 53: 5476-5490 Google Scholar
- Okuda K., Takarada S., Hirota T., Sasaki K.. (2015) Polycyclic N‐Heterocyclic compounds. Part 86: Synthesis and Evaluation of antiplatelet Aggregation Activity of 2, 4‐ Disubstituted 9‐chloro‐5, 6‐dihydropyrimido [5, 4‐d] benzazepine and Related compounds. Journal of Heterocyclic Chemistry 52: 780-792 Google Scholar
- Oukoloff K., Kovalevich J., Cornec A.-S., Yao Y., Owyang Z. A., James M., Trojanowski J. Q., Lee V. M.-Y., Smith A. B., Brunden K. R., Ballatore C., et al. (2018) Design, synthesis and evaluation of photoactivatable derivatives of microtubule (MT)-active [1, 2, 4] triazolo [1, 5-a] pyrimidines. Bioorganic and Medicinal Chemistry Letters 28: 2180-2183 Google Scholar
- Pasupathi M., Santhi N.. (2019) Synthesis characterization and antimicrobial activity of ethyl 4-(2,4-dichlorophenyl)-1,2,3,4-tetrahydro-6-methyl-2-thiox opyrimidine-5-carboxylate. World Scientific News 117: 212-220 Google Scholar
- Patil P. T., Warekar P. P., Patil K. T., Undare S. S., Jamale D. K., Vibhute S. S., Valekar N. J., Kolekar G. B., Deshmukh M. B., Anbhule P. V., et al. (2018) A simple and efficient one-pot novel synthesis of pyrazolo [3,4-b][1, 8] naphthyridine and pyrazolo [3, 4-d] pyrimidine [1, 2-a] pyrimidine derivatives as anti-inflammatory agents. Research on Chemical Intermediates 44: 1119-1130 Google Scholar
- Phillips A. P.. (1949) Condensation of aromatic aldehydes with γ-picoline methiodide. The Journal of Organic Chemistry 14: 302-305 Google Scholar
- Sabt A., Eldehna W. M., Al-Warhi T., Alotaibi O. J., Elaasser M. M., Suliman H., Abdel-Aziz H. A., et al. (2020) Discovery of 3, 6-disubstituted pyridazines as a novel class of anticancer agents targeting cyclin-dependent kinase 2: Synthesis, biological evaluation and insights. Journal of Enzyme Inhibition and Medicinal Chemistry 35: 1616-1630 Google Scholar
- Saifuddin M., Guo C., Biewenga L., Saravanan T., Charnock S. J., Poelarends G. J., et al. (2020) Enantioselective aldol addition of acetaldehyde to aromatic aldehydes catalyzed by proline-based carboligases. ACS Catalysis 10: 2522-2527 Google Scholar
- Sarode V. I., Bhole R. P.. (2019) Synthesis, Characterization of 2-methylthio-1, 4-dihydropyrimidines for its antibacterial potential. Research Journal of Pharmacy and Technology 12: 1585-1589 Google Scholar
- Sharma D., Chatterjee R., Dhayalan V., Dhanusuraman R., Dandela R.. (2022) Recent advances in the practical synthesis of C1 deuterated aromatic aldehydes enabled by catalysis and beyond. Chemistry, an Asian Journal 17: Article e202200485 Google Scholar
- Sondhi S. M., Sharma V. K., Verma R. P., Singhal N., Shukla R., Raghubir R., Dubey M. P., et al. (1999) Synthesis, anti-inflammatory and analgesic activity evaluation of some mercapto pyrimidine and pyrimidobenzimidazole derivatives. Synthesis 1999: 878-884 Google Scholar
- Tyagi K., Sunkum A., Gupta P., Kilambi H. V., Sreelakshmi Y., Sharma R., et al. (2023) Reduced γ-glutamyl hydrolase activity likely contributes to high folate levels in Periyakulam-1 tomato. Horticulture Research 10: Article uhac235 Google Scholar
- Wilcoxen K. M., Zhu Y. F., Connors P. J., Saunders J., Gross T. D., Gao Y., Reinhart G. J., Struthers R. S., Chen C., et al. (2002) Synthesis and initial structure–activity relationships of a novel series of imidazole [1, 2-a] pyrimid-5-ones as potent GnRH receptor antagonists. Bioorganic and Medicinal Chemistry Letters 12: 2179-2183 Google Scholar