ABSTRACT
Background
Xanthine oxidase and tyrosinase are enzymes involved in various functions in the body. Tyrosinase and xanthine oxidase have high activity levels in various diseases like gout hyperpigmentation and neurodegradative disease. In our study, we checked for the ability of locally available black and brown rice varieties to inhibit the two enzymes.
Materials and Methods
We carried out in vitro assay of the activity of the two enzymes in the presence of rice extracts. To identify the phytochemicals potentially responsible for inhibitory activity we carried out in silico docking investigation using Auto dock followed by molecular dynamics simulation analysis.
Results and Discussion
The in vitro assays showed reduction in the enzyme activity of xanthine oxidase and tyrosinase in the presence of the rice extracts. The molecular docking analysis showed that the major polyphenols of the rice extracts showed good binding with the two enzymes. Molecular dynamics simulation showed that catechin hydrate from black rice and β-sitosterol from brown rice showed steady binding with xanthine oxidase and tyrosinase respectively.
Conclusion
From our studies we can conclude that both rice extracts had inhibitory activity towards the enzymes and catechin hydrate and β-sitosterol could potentially be the compounds responsible for inhibition of xanthine oxidase and tyrosinase respectively.
INTRODUCTION
Xanthine oxidase (EC 1.17.3.2) is an enzyme that catalyses the conversion hypoxanthine to xanthine and then finally to uric acid. In this process, xanthine oxidase produces reactive oxygen species. High activity of xanthine oxidase leads to deposition of uric acid leading to hyperuricemia.1 High levels of xanthine oxidase has also been reported in parkinson’s disease.2 Xanthine oxidase is one of the major enzymes that promote ROS production, leading to death of dopamine producing cells which is a prime cause of parkinson’s disease.3 A high level of xanthine oxidase can lead to several other diseases. As such, the inhibition of xanthine oxidase becomes necessary in different disease situations and the current challenge is to obtain a xanthine oxidase inhibitor that is safe for consumption.4
Tyrosinase (EC 1.14.18.1.) is an enzyme involved majorly in the synthesis of melanin. It catalyses the conversion of L-tyrosine to 3,4-Dihydroxyphenylalanine (DOPA) followed by its conversion to o-dopaquinone which ultimately leads to melanin production.5 Based on the tissue of occurrence, the higher activity of tyrosinase can lead to different issues. In skin tissues, the higher activity of tyrosinase can lead to diseases like hyperpigmentation, melanoma etc.6 Tyrosinase overexpression in the brain can lead to increased production of neuromelanin. Accumulation of neuromelanin has been regarded as a major cause of the onset of Parkinson’s disease.7 There are reports of the correlations between the occurrence of melanoma and parkinson’s disease and tyrosinase overexpression has been the connecting link between the two pathologies.8 The current crop of tyrosinase inhibitors suffer issues of severe toxicity along with low efficacy.7 Thus it necessitates the search for new inhibitors from plant sources.
Black rice and brown rice are popular rice varieties in the South East Asia along with the North-Eastern states of India. The bran of both the rice varieties is rich in various polyphenols like anthocyanins, tocopherols, etc., and have various health benefits.9 In our current study, we analyse the ability of the methanolic extracts of the brown and black rice varieties to inhibit the enzymes tyrosinase and xanthine oxidase.
MATERIALS AND METHODS
Rice Extract preparation
For preparation of the rice extracts we followed the protocol of Rao et al., 2020 with modifications.10 We obtained the unpolished black and brown rice varieties from local suppliers in Guwahati, India. The rice was milled in a local rice grinding mill to obtain the rice bran. The rice bran was then microwaved, dried and grinded. The powder was washed with 80% methanol/water and kept on a shaker. The extracts were filtered using Whatman Number 1 filter paper. The extracts were concentrated using a rotary evaporator. The extracts were dissolved in DMSO followed by dilution in 50 mM phosphate buffer to a concentration of 0.05 g/mL.
Tyrosinase activity assay
To analyse the effect of rice samples on tyrosinase enzyme activity, we prepared 0.05M phosphate buffer and mixed tyroinase at a concentration of 2500 U/mL. In the reaction mixture, 15 uL of tyrosinase solution, 200 uL of the rice sample or buffer and 100 uL of the substrate L-DOPA at a concentration of 5 Mm were mixed and incubated at 37°C for 3 min. Then absorbance readings were taken at 492 nm. Kojic acid was used a positive control and the results were expressed in fold activity.11
Xanthine oxidase activity assay
To analyse the effect of the rice samples on xanthine oxidase activity we, dissolved xanthine oxidase in 100 mM phosphate buffer of pH 7.5 at a concentration of 0.5U/mL. 875 uL phosphate buffer, 50 uL of xanthine oxidase solution and 10 uL of extract/ control sample were mixed and incubated at 37 min C for 3 min. 65 μL of 0.82 mM xanthine solution was then added and incubated at 37 min C for 3 min. The readings were taken at 295 nm. Allopurinol was used a positive control and the results were expressed in percent enzyme activity.12
Statistical analysis
The results were expressed in Mean±standard deviation. The significance of the values between the control and the treated groups was calculated using One-way ANOVA. p<0.05 was considered significant.
Literature search for compounds of black and brown rice and ligand preparation
A literature search was carried to find out the reported constituents of the methanolic extract of the black and brown rice varieties. The structures of the reported compounds were obtained from the Pubchem website in their 3D.sdf format. These files were converted to. pdbqt files using the Open Babel software.13,14
Docking of compounds against tyrosinase and xanthine oxidase
The PDB formats of the tyrosinase (PDB ID-3NM8) and xanthine oxidase (PDB ID-3B9J) enzymes were obtained from the Protein Data Bank database. The pdb files were converted to pdbqt format using Autodock 4.0. This was followed by the docking of the compounds with selected amino acids of tyrosinase and xanthine oxidase as per protocol mentioned by Forli et al. The 2D images were generated using Discovery studio software.15
Homology modeling
The experimental structure of Chain J of Xanthine Oxidase in Protein Data Bank (PDB) (PDB ID: 3B9J) consisted of missing residues. Comparative homology modelling in the Robetta Server has been used in the current study to model the missing structures with the crystal structure of Xanthine Oxidase from Bovine Milk as the template protein with PDB ID 1FIQ.16
Molecular dynamics simulation studies
MD simulation studies are performed using the Groningen Machine for Chemicals Simulations (GROMACS) 2022.3 package. Ligand topology parameters for catechin hydrate are generated by the CHARMM General Force Field (CGenFF) program. Hydrogens in the entire molecular system are added by the inbuilt GROMACS program pdb2gmx. CHARMM36 function was used to generate the topology of the system. The system was solvated in a cubic box and neutral physiological conditions were maintained. Energy of the system was minimized followed by equilibration of the system in NVT and NPT ensembles. The equilibrated system was prepared for MD production run for 150ns. The dynamics in the various simulation trajectories are examined by calculating various parameters, viz., RMSD, Rg, RMSF and SASA of protein via inbuilt GROMACS utilities. Important residues essential to the interaction between protein and ligand are assessed using the mindist and rmsdist programs in GROMACS. All the conformations and trajectories were visualized using Visual Molecular Dynamics (VMD) software.17
Principal Component Analysis
A statistical method called Principal Component Analysis (PCA) is used to reduce the dimensionality of complicated data sets while retaining the majority of its intrinsic volatility. From the aligned structural data, a covariance matrix is produced in order to analyse the principal components. The pairwise relationships between several dimensions are quantified by the covariance matrix. The covariance matrix’s eigenvectors, or principal components and matching eigenvalues are obtained by diagonalizing the matrix. The dominating collective motions are frequently captured by the first few primary components. In this investigation, PCA computations were performed using the built-in GROMACS programmes, gmxcovar and gmxanaeig, respectively.18
RESULTS
The methanolic and aqueous extracts of the black and brown rice varieties were prepared and the extracts were checked for the ability to inhibit the activity of the xanthine oxidase and tyrosinase enzymes. The methanolic and aqueous extracts of black rice showed the best inhibition of xanthine oxidase with the percent enzyme activity dropping to 30.65±2.39 and 31.56±1.48 at a dose of 1.5 mg/mL with respect to the untreated control (p<0.01 in both the cases). In both the extracts, a dose dependent decrease in enzyme activity was observed (Figure 1, Table 1).
Table 1:
Table showing the percent enzyme activity of xanthine oxidase under different treatments.
Rice variety | Extract | Control | Allopurinol (Inhibitor)* | Concentration of sample (mg/mL) | ||
---|---|---|---|---|---|---|
Black rice | Methanolic | 100 | 47.83±1.17 | 0.5 | 1 | 1.5 |
52.21±2.11 | 41.77±1.94 | 30.45±2.39 | ||||
Aqueous | 100 | 52.67±2.66 | 0.5 | 1 | 1.5 | |
50.52±0.92 | 43.37±1.22 | 31.56±1.48 | ||||
Brown rice | Methanolic | 100 | 46.05±2.80 | 0.5 | 1 | 1.5 |
74.80±3.81 | 72.98±2.05 | 64.44±4.71’ | ||||
Aqueous | 100 | 51.83±3.56 | 0.5 | 1 | 1.5 | |
77.79±2.57 | 74.19±4.31 | 63.17±3.67 |
In case of tyrosinase, the methanolic and aqueous extracts of black rice showed the best inhibition with the percent enzyme activity dropping to 43.42±1.80 and 53.77±1.97 respectively at a dose of 1.5 mg/mL with respect to the untreated control (p<0.01) (Figure 2, Table 2).
Table 2:
Table showing the percent enzyme activity of tyrosinase under different treatments.
Rice variety | Extract | Control | Kojic acid (Inhibitor)* | Concentration of sample (mg/mL) | ||
---|---|---|---|---|---|---|
Black rice | Methanolic | 100 | 52.96±1.06 | 0.5 | 1 | 1.5 |
83.12±1.99 | 75.42±1.74 | 77.22±1.12 | ||||
Aqueous | 100 | 48.34±1.80 | 0.5 | 1 | 1.5 | |
76.86±4.20 | 69.15±1.73 | 56.61±2.91 | ||||
Brown rice | Methanolic | 100 | 47.03±1.30 | 0.5 | 1 | 1.5 |
54.56±2.80 | 48.42±2.55 | 43.42±1.80 | ||||
Aqueous | 100 | 53.32±2.88 | 0.5 | 1 | 1.5 | |
76.56±2.07 | 66.76±2.12 | 53.77±1.97 |
To identify the phytochemicals responsible for the lowering of the enzymatic acivities, we carried out a literature survey to obtain the major phytochemicals present in the rice varieties.19,20 For us in silico analysis, we docked the reported compounds with the pdb structures of the enzymes tyrosinase and xanthine oxidase. With respect to xanthine oxidase, the percentage identity of the template and target protein is 100% as per BLAST analysis. From our dockings, we observed that cathechin hydrate from black rice showed the best docking with xanthine oxidase and β-sitosterol from brown rice showed the best docking with tyrosinase (Tables 3 and 4).
Table 3:
Binding energy of the black rice phytochemicals with xanthine oxidase and tyrosinase.
Protein docked | Phytochemical | Binding energy (kcal/ mol) | Inhibition constant |
---|---|---|---|
Xanthine oxidase (PDB ID-39BJ) | Cyanidin-3-glucoside | -6.47 | 18.04 μM |
Cyanidin-3-galactoside | -3.56 | 2.46 mM | |
4-Hydroxybenzoic acid | -6.6 | 14.64 μM | |
±Catechin hydrate | -8.59 | 502.47 nM | |
Vanillic acid | -6.3 | 21.15 μM | |
Syringic acid | -5.03 | 204.92 μM | |
p-Tyrosol | -5.8 | 54.45 μM | |
±Alpha Tocopherol | -4.73 | 343.43 μM | |
Delphinidin-3-arabinoside | -2.3 | 17.75 mM | |
Delphinidin-3-galactoside | -3.23 | 4.79 mM | |
Xanthine oxidase Model structure | Cyanidin-3-glucoside | -6.55 | 8.41 μM |
Cyanidin-3-galactoside | -5.57 | 82.63 μM | |
4-Hydroxybenzoic acid | -5.6 | 79.17 μM | |
±Catechin hydrate | -6.96 | 7.91 μM | |
Vanillic acid | -5.24 | 144.45 μM | |
Syringic acid | -4.71 | 361.33 μM | |
p-Tyrosol | -5.17 | 162.18 μM | |
±Alpha Tocopherol | -6.38 | 21.07 μM | |
Delphinidin-3-arabinoside | -6.18 | 9.42 μM | |
Delphinidin-3-galactoside | -5.7 | 65.88 μM | |
Tyrosinase (4UOA) | Cyanidin-3- glucoside | -5.00 | 217.21 μM |
Cyanidin-3-galactoside | -5.94 | 44.22 μM | |
4-Hydroxybenzoic acid | -4.09 | 73.01 mM | |
±Catechin hydrate | -5.19 | 157.24 μM | |
Vanillic acid | -4.75 | 327.33 μM | |
Syringic acid | -5.07 | 192.05 μM | |
p-Tyrosol | -5.92 | 45.54 μM | |
±Alpha Tocopherol | -5.0 | 217.21 μM | |
Delphinidin-3-arabinoside | -4.93 | 243.91 μM | |
Delphinidin-3-galactoside | -4.85 | 276.47 μM |
Table 4:
Binding energy of the brown rice phytochemicals with xanthine oxidase and tyrosinase.
Protein docked | Phytochemical | Binding energy (kcal/ mol) | Inhibition constant |
---|---|---|---|
Xanthine oxidase (PDB | Peonidin-3-O-glucoside | -3.98 | 1.2 mM |
ID-39BJ) | Cyanidin-3-O-rutinoside | +2.8 | |
Epicatechin | -7.29 | 4.57 μM | |
Gallic acid | -6.45 | 18.82 μM | |
Luteolin | -8.41 | 682.65 nM | |
Tricin | -9.2 | 180.02 nM | |
Myricetin | -8.28 | 850.4 nM | |
Vanillic acid | -6.15 | 31.28 μM | |
Cinnamic acid | -5.9 | 47.08 μM | |
Ellagic acid | -7.12 | 6.03 μM | |
β-sitosterol | -8.89 | 306.6 nM | |
Xanthine oxidase Model structure | Peonidin-3-O-glucoside | -7.84 | 1.8 μM |
Cyanidin-3-O-rutinoside | -6.77 | 10.87 μM | |
Epicatechin | -7.11 | 6.09 μM | |
Gallic acid | -5.86 | 50.32 μM | |
Luteolin | -7.18 | 5.46 μM | |
Tricin | -6.54 | 16.12 μM | |
Myricetin | -6.55 | 15.92 μM | |
Vanillic acid | -5.22 | 149.05 μM | |
Cinnamic acid | -6.1 | 33.63 μM | |
Ellagic acid | -5.39 | 112.91 μM | |
β-sitosterol | -7.18 | 5.5 μM | |
Tyrosinase (PDB ID- 4UOA) | Peonidin-3-O-glucoside | -5.03 | 205.47 μM |
Cyanidin-3-O-rutinoside | -5.05 | 199.16 μM | |
Epicatechin | -6.38 | 21.15 μM | |
Gallic acid | -2.87 | 7.94 mM | |
Luteolin | -6.14 | 31.42 μM | |
Tricin | -5.34 | 121.87 μM | |
Myricetin | -5.19 | 156.25 μM | |
Vanillic acid | -3.35 | 3.25 mM | |
Cinnamic acid | -3.93 | 1.32 mM | |
Ellagic acid | -5.13 | 174.18 μM | |
β-sitosterol | -7.55 | 2.93 μM |
Catechin hydrate formed hydrogen bonds with conserved residues in the xanthine oxidase active site. β-sitosterol docked with several conserved residues of the tyrosinase active site (Figure 3). We carried out molecular simulation dynamics studies to look into the stabilities of catechin hydrate-xanthine oxidase and β-sitosterol-tyrosinase complexes.
In the present work, it is seen that the target protein Xanthine Oxidase shows higher variations in Root Mean Square Deviation (RMSD) in the presence of ligand catechin hydrate. Similarly, the complex of tyrosinase and β-sitosterol also show higher RMSD value as compared to the tyrosinase protein alone. The shift in RMSD value is more prominent in case of the complex of xanthine oxidase-catechin hydrate with a 0.2 nm deviation (Figure 4a, 4b).
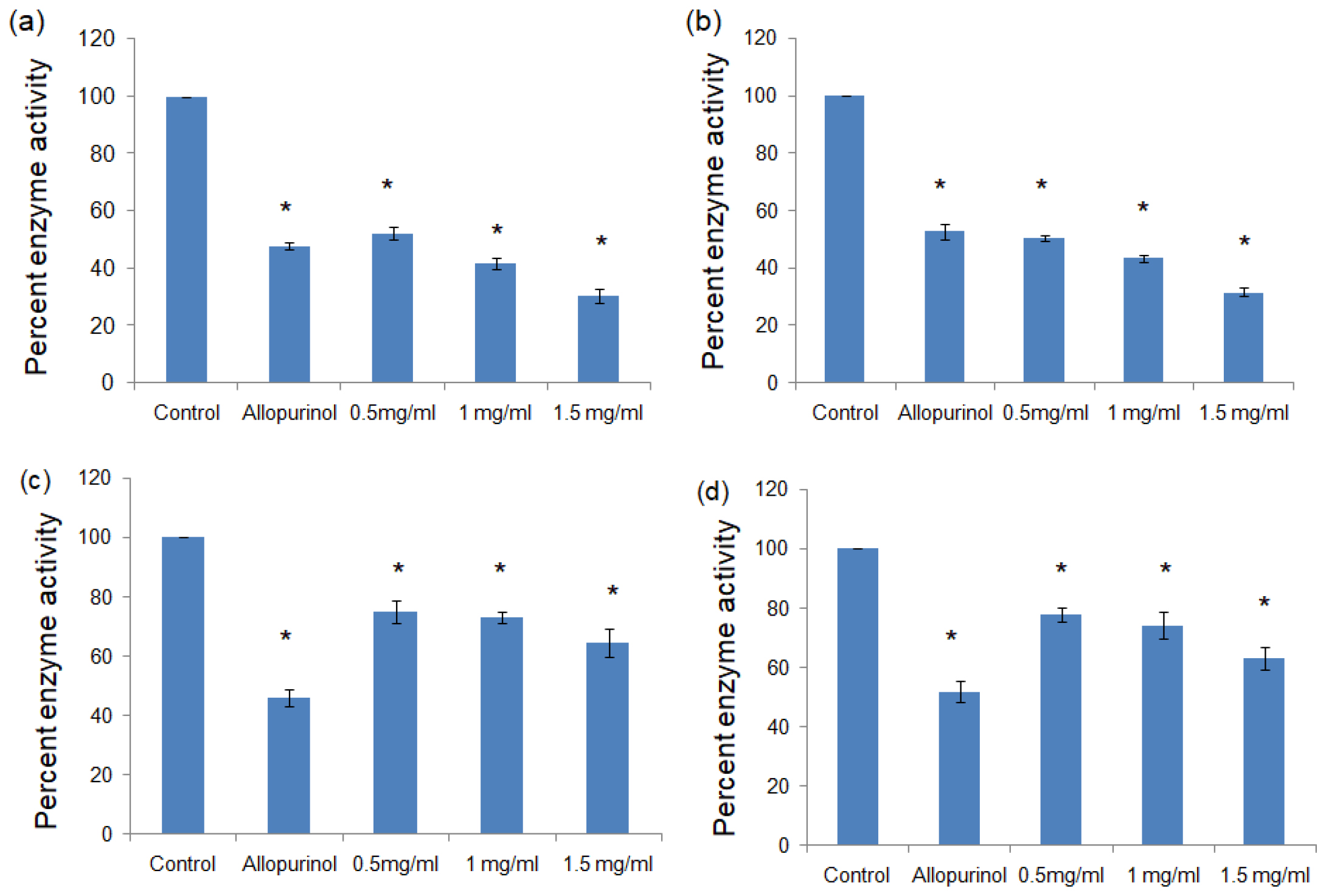
Figure 1:
Percent enzyme activity of xanthine oxidase in the presence of (a) methanolic extract of black rice, (b) aqueous extract of black rice, (c) methanolic extract of brown rice, (d) aqueous extract of brown rice. All values expressed as mean± standard deviation. *p>0.01 vs Control.
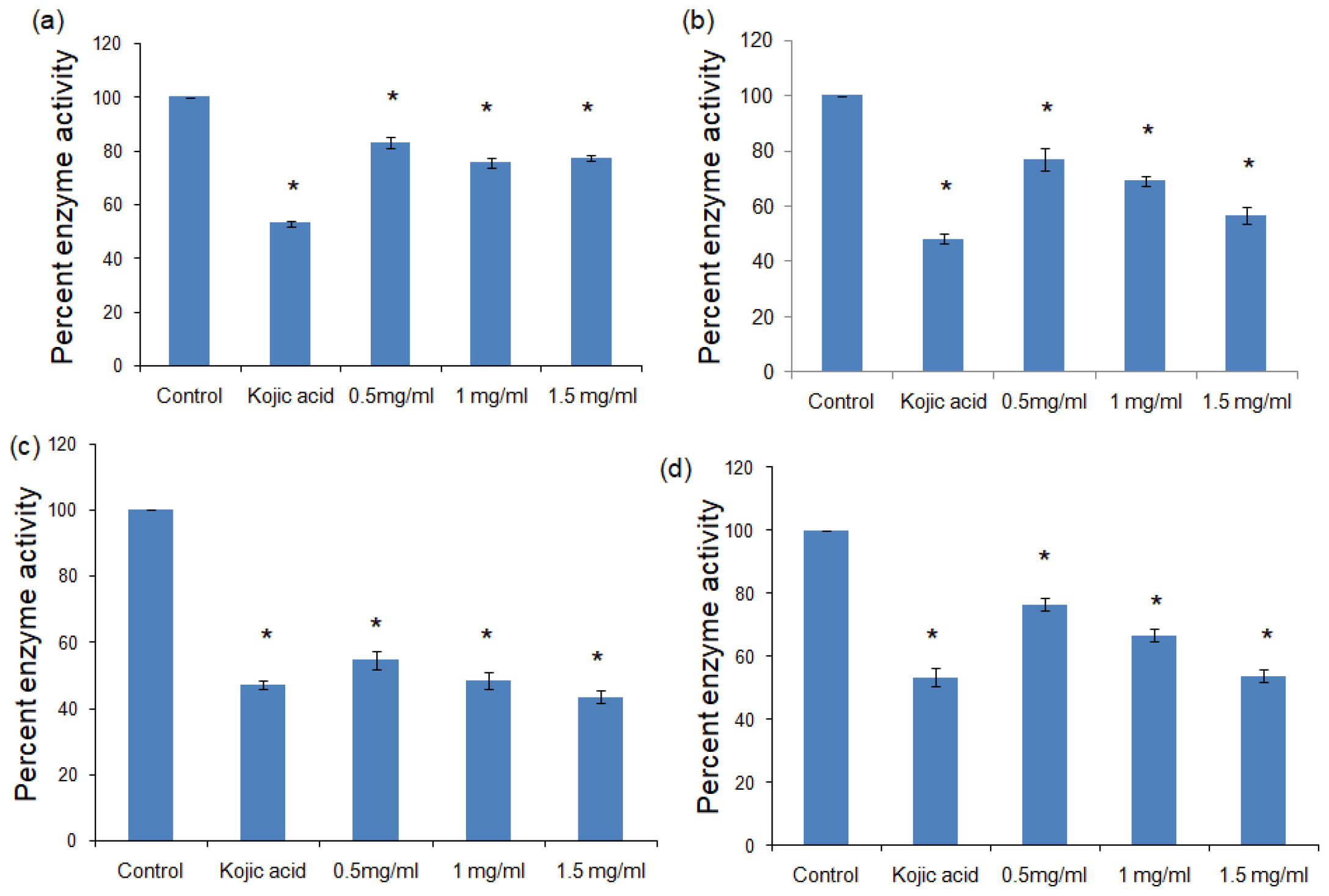
Figure 2:
Percent enzyme activity of tyrosinase in the presence of (a) methanolic extract of black rice, (b) aqueous extract of black rice, (c) methanolic extract of brown rice, (d) aqueous extract of brown rice. All values expressed as mean± standard deviation. *p>0.01 vs Control.
The Root Mean Square Fluctuation (RMSF) values of the amino acids in the presence and absence of catechin hydrate show a difference in the amino acids in the range of residue number 400 to 500 of the xanthine oxidase. In case of tyrosinase, there is a deviation in the in the RMSF values in the amino acid residues in the range of 50 to 90 in the presence of β-sitosterol (Figure 4c, 4d).
Radius of gyration (Rg) is a measure of the compactness or spatial extent of a molecular structure. Figure 5a and 5b shows the fluctuations in Rg for both the bound and unbound forms of Xanthine Oxidase with the selected ligand. It is clearly evident that the protein in bound form shows increased Rg with respect of the simulation time. For tyrosinase, there is an increase in the Rg values at 120 ns. We observed an increase in the Solvent Accessible Surface Area (SASA) values of the proteins when bound to their respective ligands (Figure 5c, 5d).
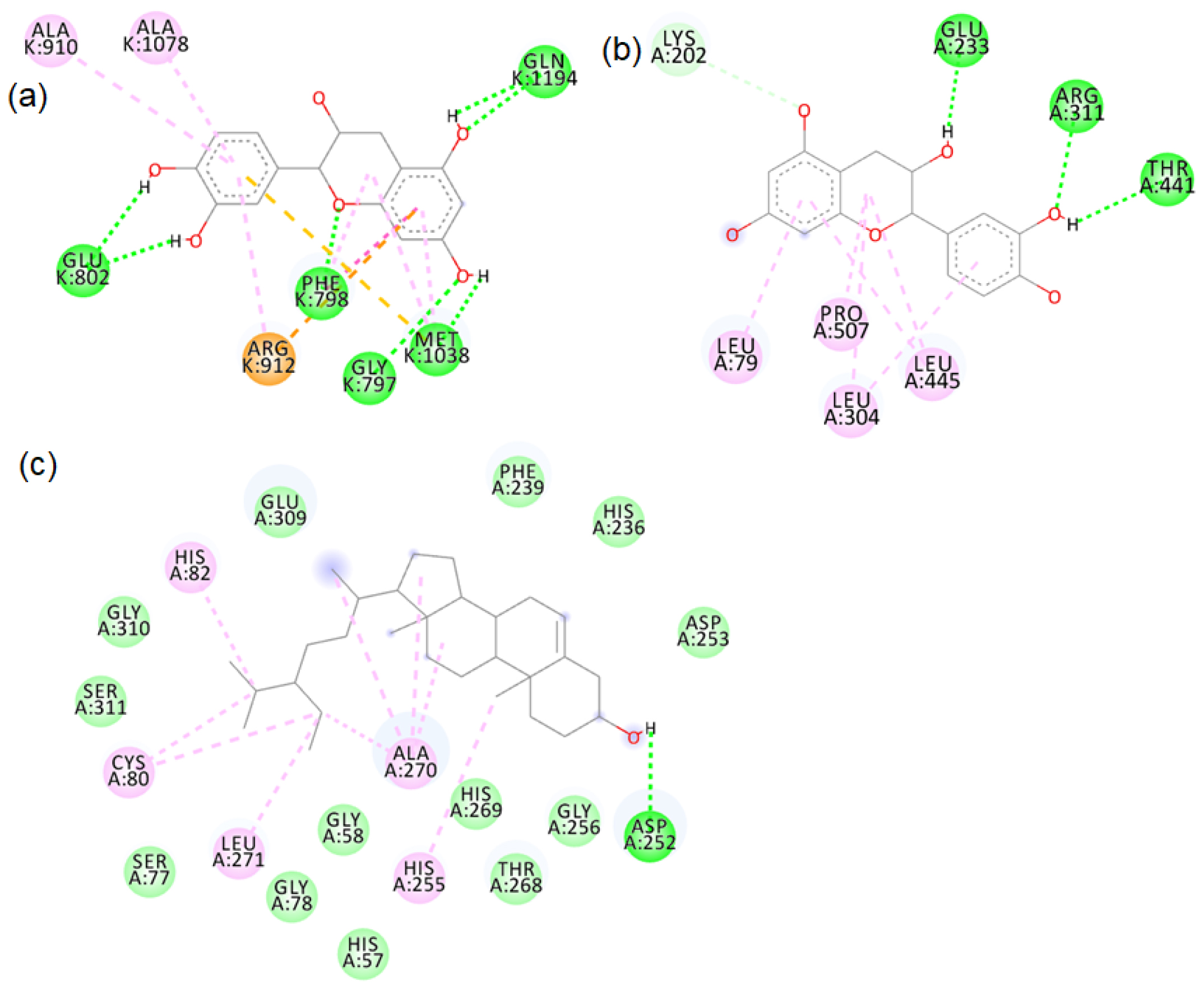
Figure 3:
2D images of the binding of catechin hydrate with (a) xanthine oxidase (PDB- 3B9J), (b) xanthine oxidase (model structure) and (c) β-sitosterol with tyrosinase (PDB- 4OUA).
In the current analysis, Principal Component Analysis (PCA) is calculated on the backbone Cα atoms in the proteins both in presence and absence of their respective ligands; catechin hydrate and β-sitosterol. Projection of two-dimensional principal components PC1 and PC2 for the two largest Eigen values is shown in Figure 6a and 6b, which represents the relevant conformational space. It is evident that the free proteins in absence of ligand show a reduced space as compared to the more dispersed region in the bound state with ligand.
DISCUSSION
The significant inhibition of xanthine oxidase by the methanolic and aqueous extracts of black rice point the potential of the black rice bran constituents like anthocyanins to act as potential inhibitors of xanthine oxidase. Although brown rice extracts also inhibited the xanthine oxidase activity, but the inhibition of black rice extract was more prominent. As such black rice could be further studied as a potential therapy against gout.21 Both black rice and brown rice extracts were able to lower the activity of tyrosinase enzyme significantly as compared to the untreated control. However, brown rice extract was more prominent in its inhibiting effect. Thus, both of the rice extracts can be further researched for their potential to act as therapeutic against diseases like melanoma, hyperpigmentation and neurodegradative issues like parkinson’s disease.22
The major phytochemicals in black and brown rice which are reported in the methanolic and aqueous extracts showed favourable binding with xanthine oxidase and tyrosinase proteins. There was bond formation between the xanthine oxidase amino acid residues and black rice polyphenols like cyaniding-3 glucoside, cyaniding-3 galactoside, catechin hydrate (Figure S1 to S4) and brown rice polyphenols like peonidin-3-O-glucoside, epicatechin, gallic acid and luteolin (Figure S5 to S8). These polyphenols formed hydrogen bonds with the conserved active site amino acids like ASP233, ARG311. Binding of small compounds to these amino acids has led to the inhibition of the xanthine oxidase.23 The black rice phytotchemicals like hydroxybenzoic acid, vallinic acid, syringic acid bound with critical amino acids like HIS82, CYS80 and PHE454 of tyrosinase (Figure S9 to S10). The brown rice polyphenols like peonidin-3-O-glucoside, β-sitosterol, vanillic acid, cinnamic acid and ellagic acid formed bonds with conserved HIS residues present in positions 82, 251 and 255 of tyrosinase (Figure S11 to S12). Compounds that bind to these amino acids have caused inhibition of tyrosinase activities.24 We further proceeded with the molecular dynamic’s simulation of the catechin hydrate-xanthine oxidase complex and β-sitosterol-tyrosinase complex.
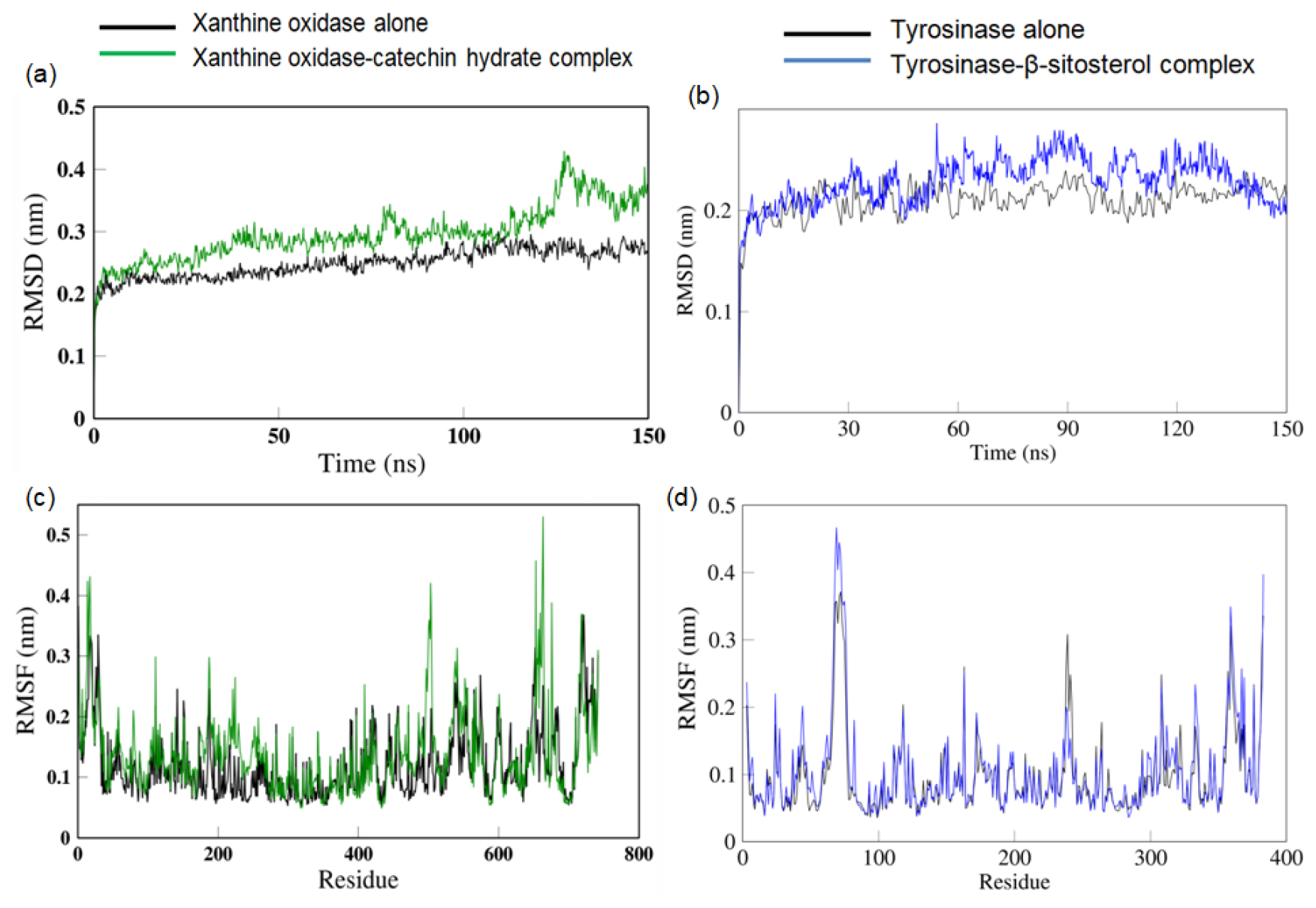
Figure 4:
RMSD values of (a) xanthine oxidase alone and in complex with catethin hydrate, (b) tyrosinase alone and in complex with β-sitosterol. RMSF values of (c) xanthine oxidase alone and in complex with catethin hydrate, (d) tyrosinase alone and in complex with β-sitosterol.
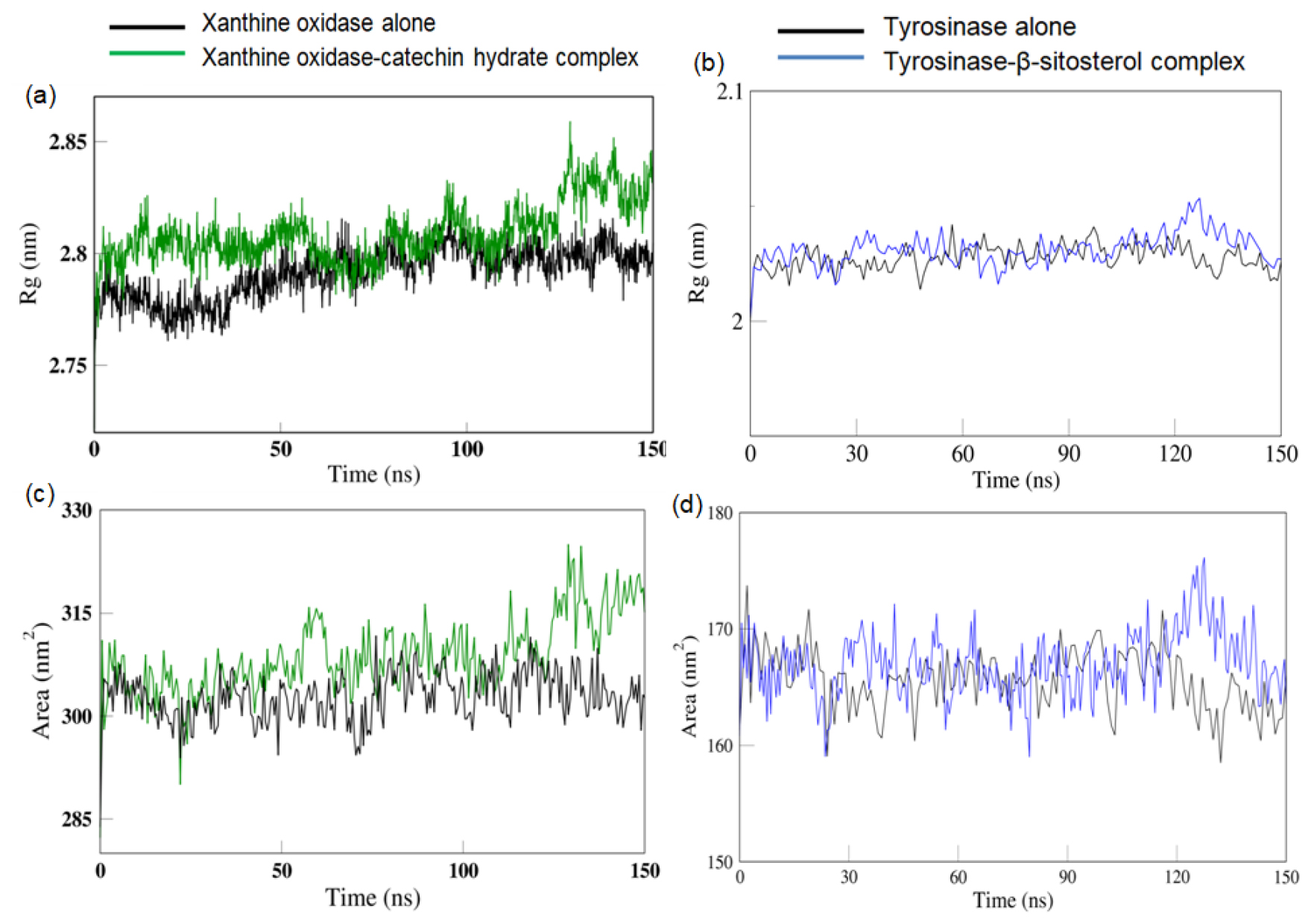
Figure 5:
Rg values of (a) xanthine oxidase alone and in complex with catethin hydrate, (b) tyrosinase alone and in complex with β-sitosterol. SASA values of (c) xanthine oxidase alone and in complex with catethin hydrate, (d) tyrosinase alone and in complex with β-sitosterol.
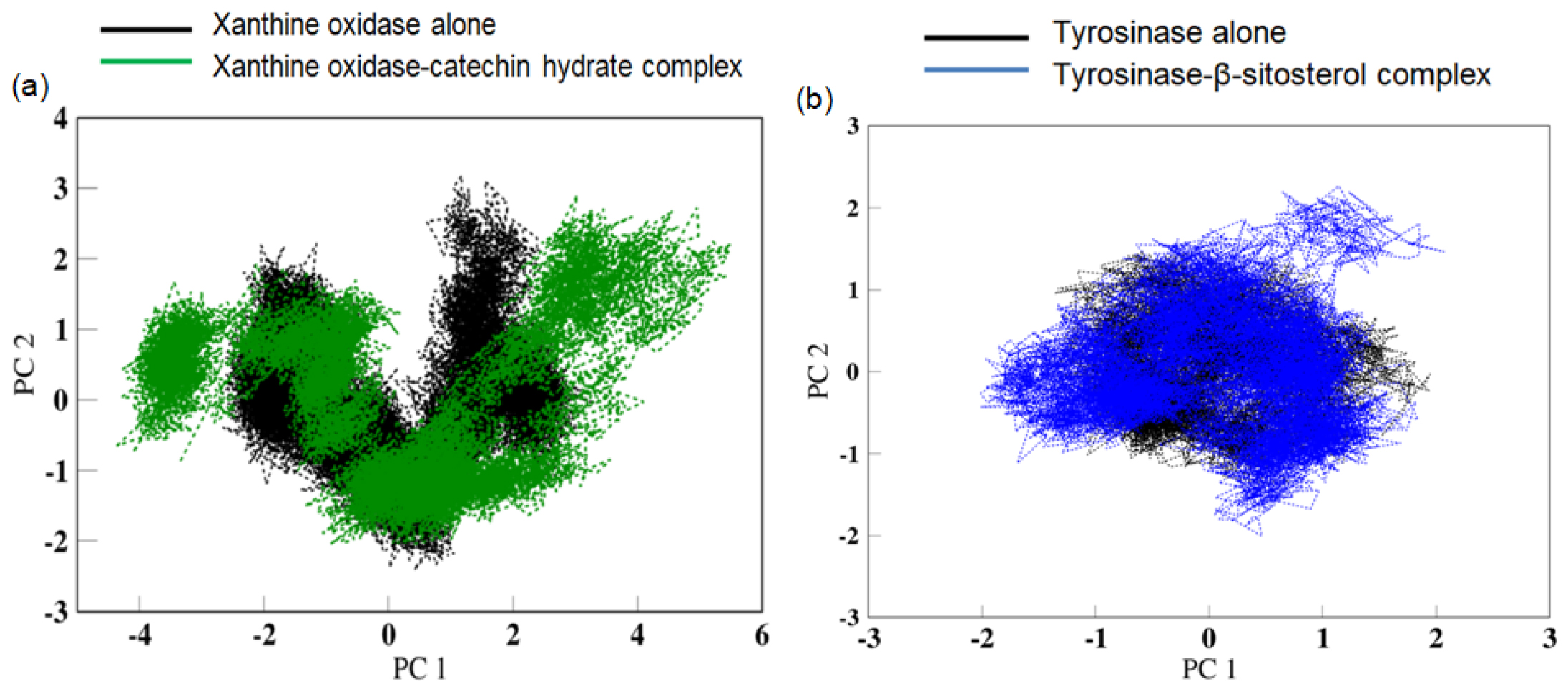
Figure 6:
Range of Conformational space related to (a) xanthine oxidase alone and xanthine oxidase-catechin hydrate complex and, (b) tyrosinase alone and tyrosinase-β-sitosterol complex as calculated from the first two principal components PC1 and PC2.
The increase in RMSD values of xanthine oxidase and tyrosinase observed in the presence of their respective ligands indicates the change in the structure of the proteins caused by the binding of the ligand.25 The increased RMSF values of amino acids residues the increased flexibility of the amino acids in that region that allows them to interact with the ligand.26 The increase in the Rg and SASA values in the presence of ligand correlates with the increase in RMSD values all of which indicates a distinct change in the shape of the protein in the presence of the ligand to accommodate better interactions with the ligand. The PCA analysis reveals that the protein in absent of ligand was limited to a lower number of conformations, depicting stability in structural dynamics. In contrast to this, a larger space in the protein conformations in presence of ligand reflects the dynamic nature of the protein visiting more conformations in the course of simulation, speculative of higher interactions of the protein and ligand. Thus, the presence of catechin hydrate has a distinct consequence on the protein motions with greater movement which will thus change the functional attributes of the target protein.27,28
CONCLUSION
From our study, we have observed that both the black rice extracts and the brown rice extracts have shown inhibitory activity towards xanthine oxidase and tyrosinase enzymes. Molecular docking and molecular simulation dynamics studies reveal that catechin hydrate from black rice and β-sitosterol from brown rice are the potential phytochemicals which could bind to xanthine oxidase and tyrosinase enzymes respectively to give the observed inhibitory effects.
ACKNOWLEDGEMENT
Authors would like to thank financial support in the form of ‘Student Research Project’ provided by the Assam Royal Global University for carrying out the work. Authors would also like to thank Guwahati Biotechnology Park for providing the facility to carry out the molecular dynamic’s simulation studies.
CONFLICT OF INTEREST
The authors declare that there is no conflict of interest.
ABBREVIATIONS
ROS | Reactive Oxygen Species |
---|---|
DOPA | 3,4-Dihydroxy-L-phenylalanine |
DMSO | Dimethyl Sulphoxide |
PDB | Protein Data Bank |
PDBQT | Protein Data Bank, Partial Charge (Q), and Atom Type (T) |
RMSD | Root means square deviation |
RMSF | Root mean square fluctuation |
SASA | Solvent accessible surface area |
Rg | Radius of Gyration |
Supplementary information
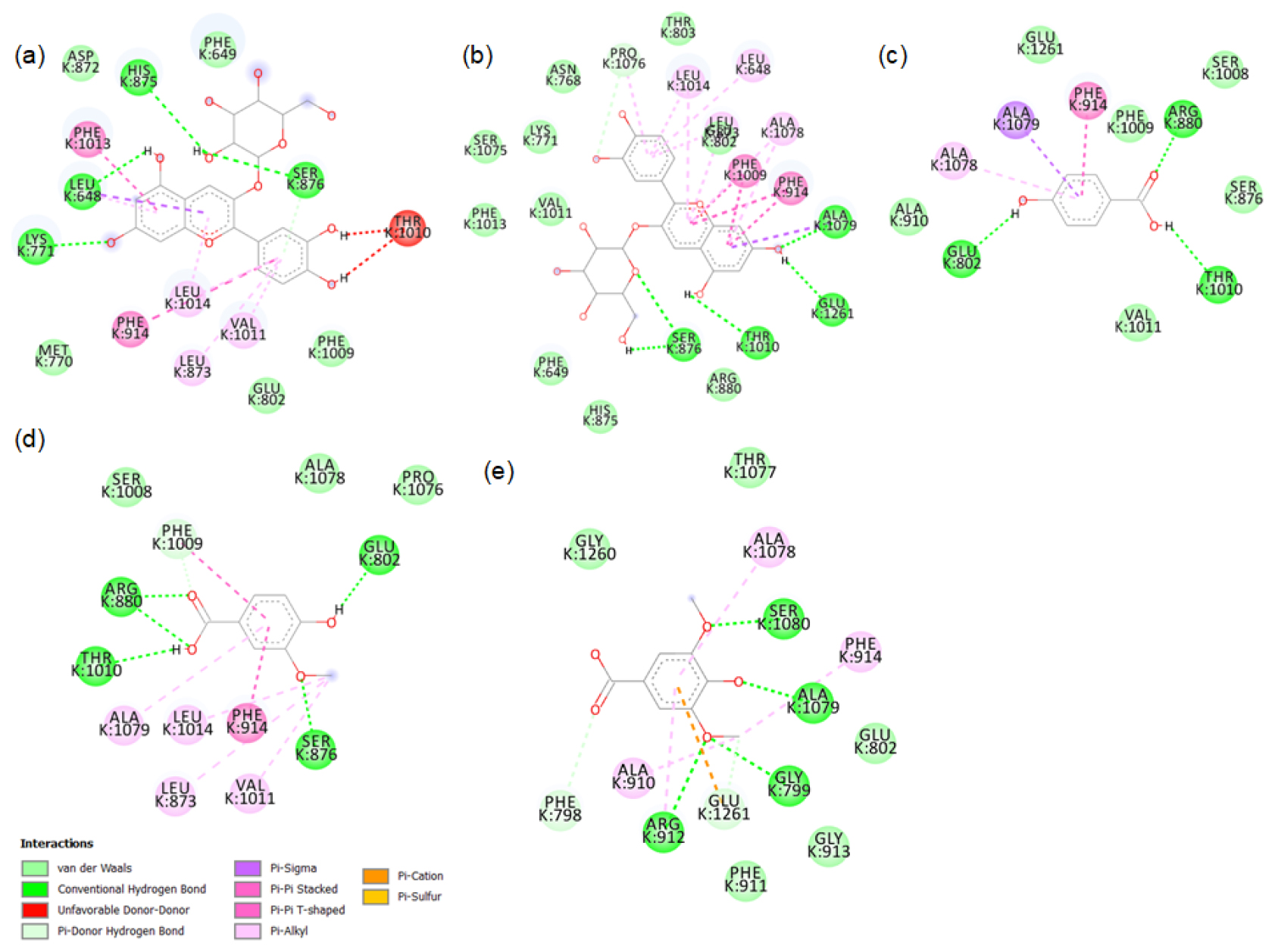
Figure S1:
2D images of the binding of xanthine oxidase (PDB-3B9J) with black rice- a. cyanidin3 glucoside, b. cyanidin3 galactoside, c. hydroxybenzoic acid, d. vallinic acid, e. syringic acid.
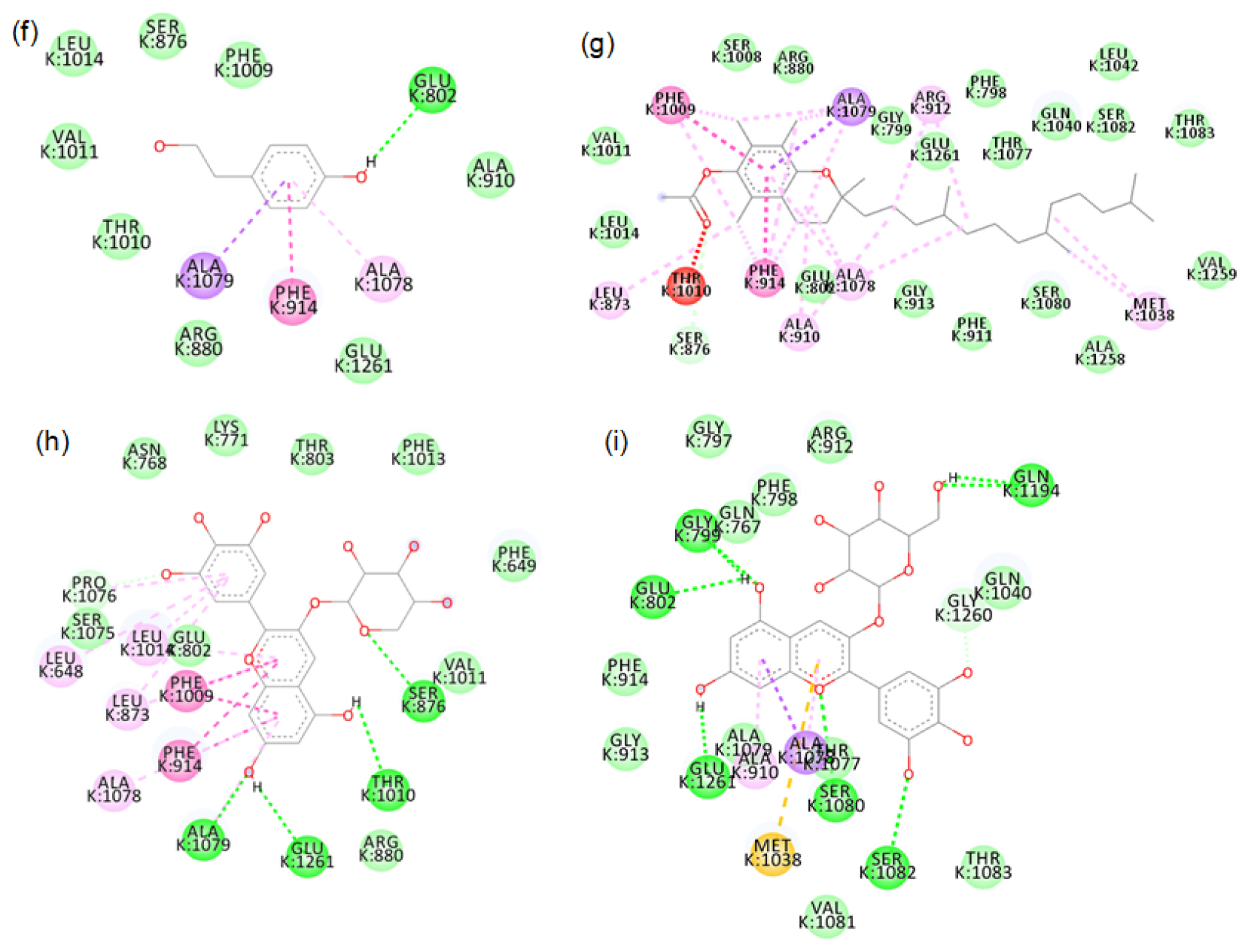
Figure S2:
2D images of the binding of xanthine oxidase (PDB-3B9J) with black rice- f. p-tyrosol, g. alpha tocopherol, h. Delphinidin-3-arabinoside, i. Delphinidin-3-galactoside.
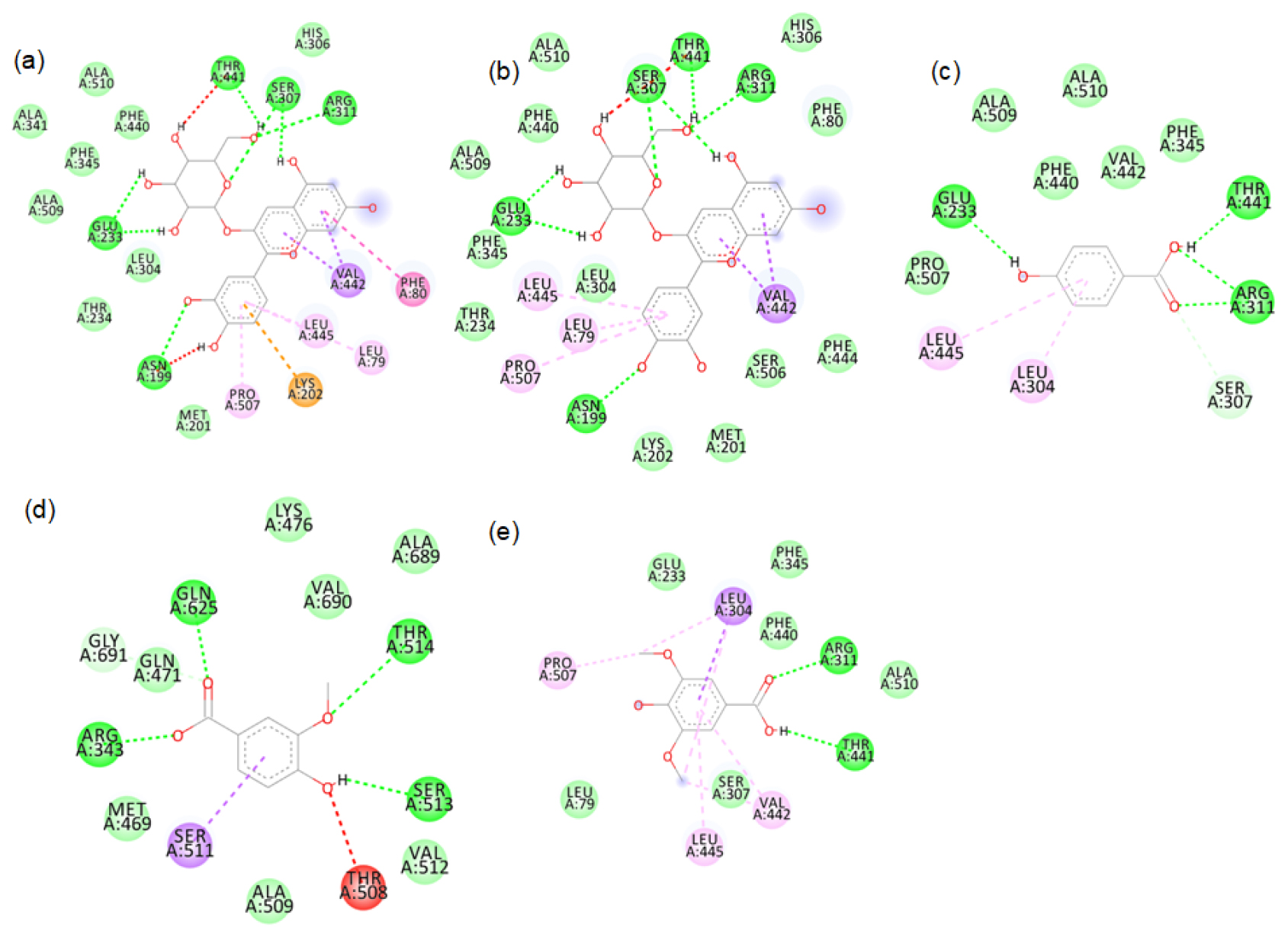
Figure S3:
2D images of the binding of xanthine oxidase (Model structure) with black rice- a. cyanidin3 glucoside, b. cyanidin3 galactoside, c. hydroxybenzoic acid, d. vallinic acid, e. syringic acid.
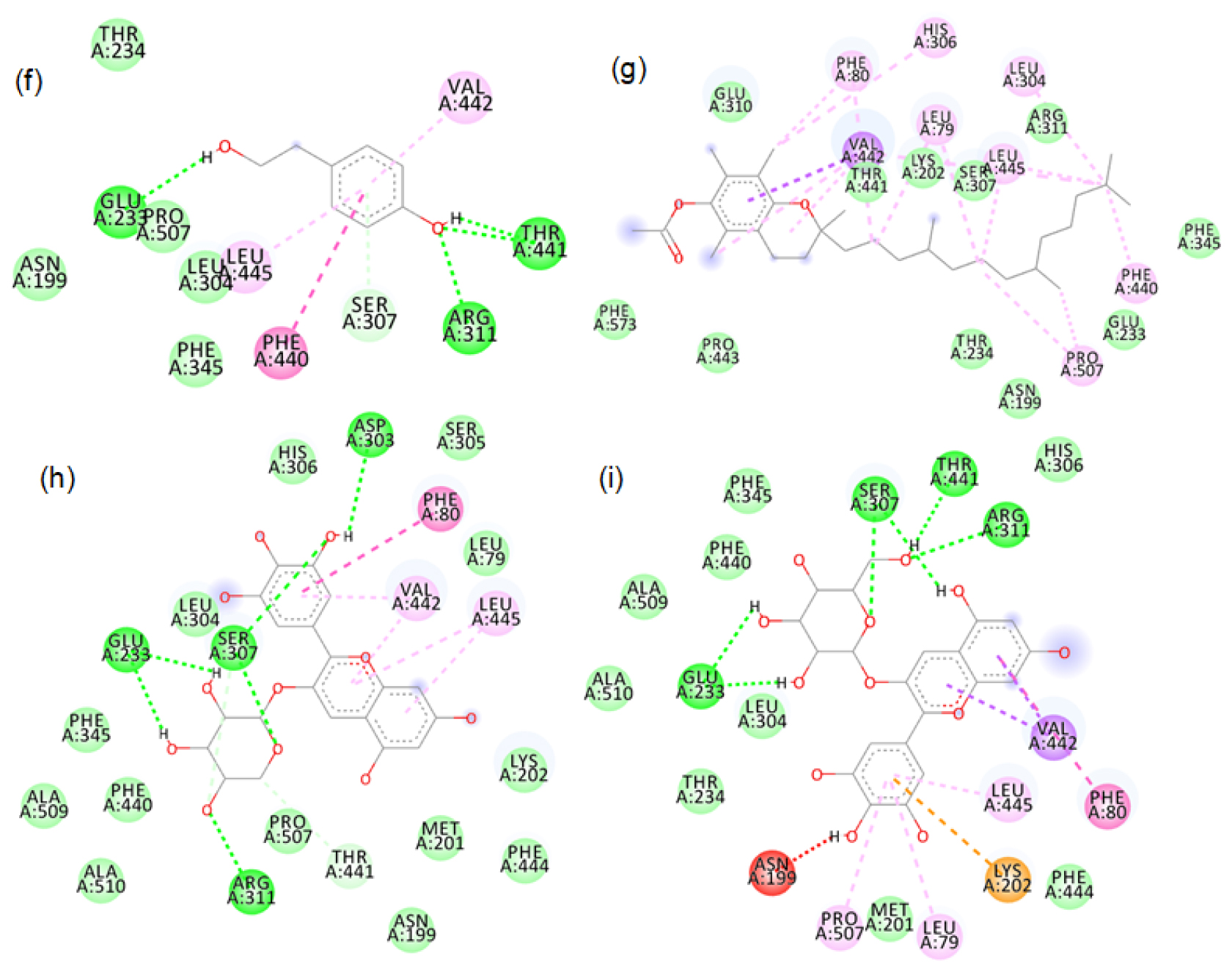
Figure S4:
2D images of the binding of xanthine oxidase (Model) with black rice- f. p-tyrosol, g. alpha tocopherol, h. Delphinidin-3-arabinoside, i. Delphinidin-3-galactoside.
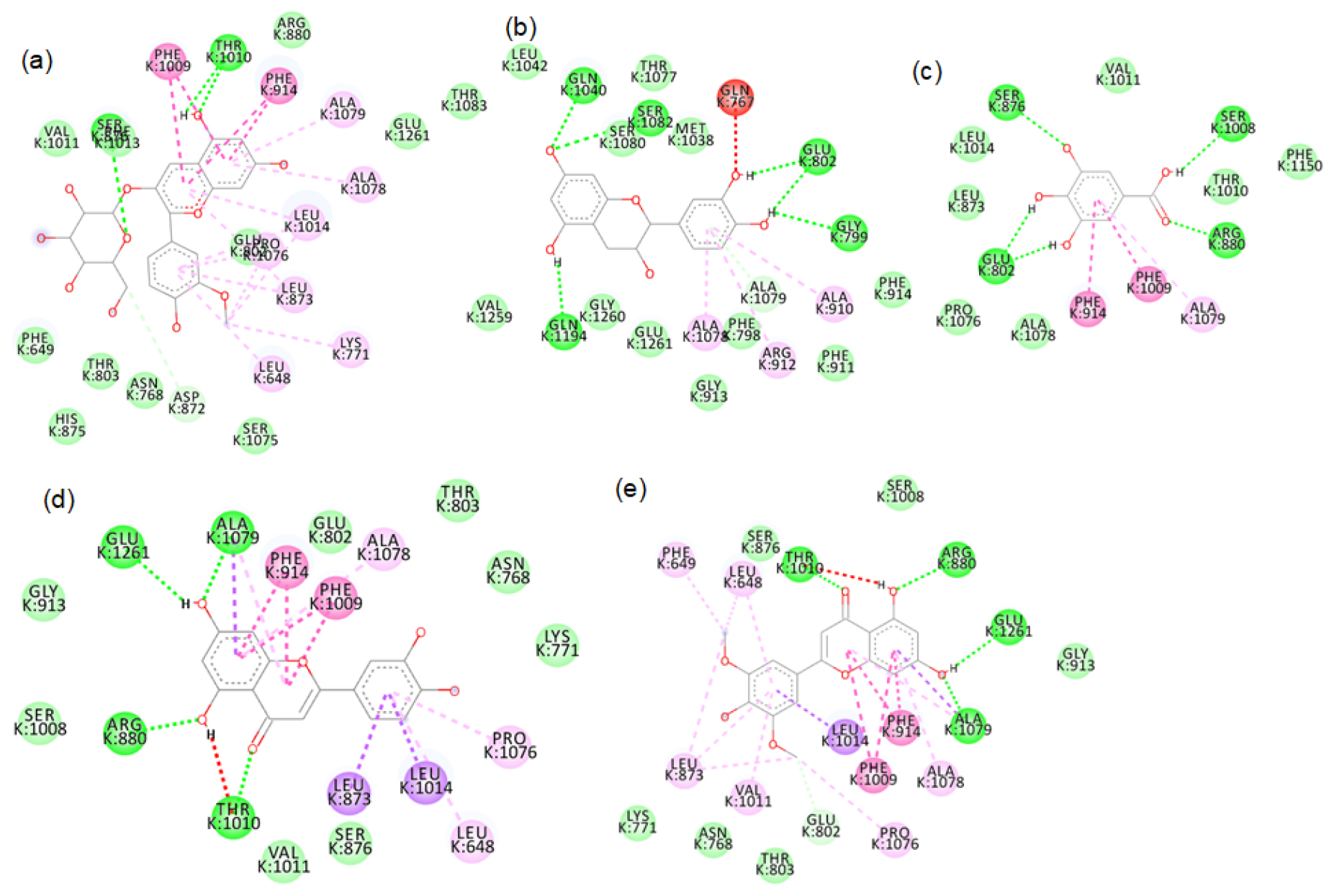
Figure S5:
2D images of the binding of xanthine oxidase (PDB-3B9J) with brown rice- a.Peonidin-3-O-glucoside, b. Epicatechin, c. Gallic acid, d. Luteolin, e. Tricin.
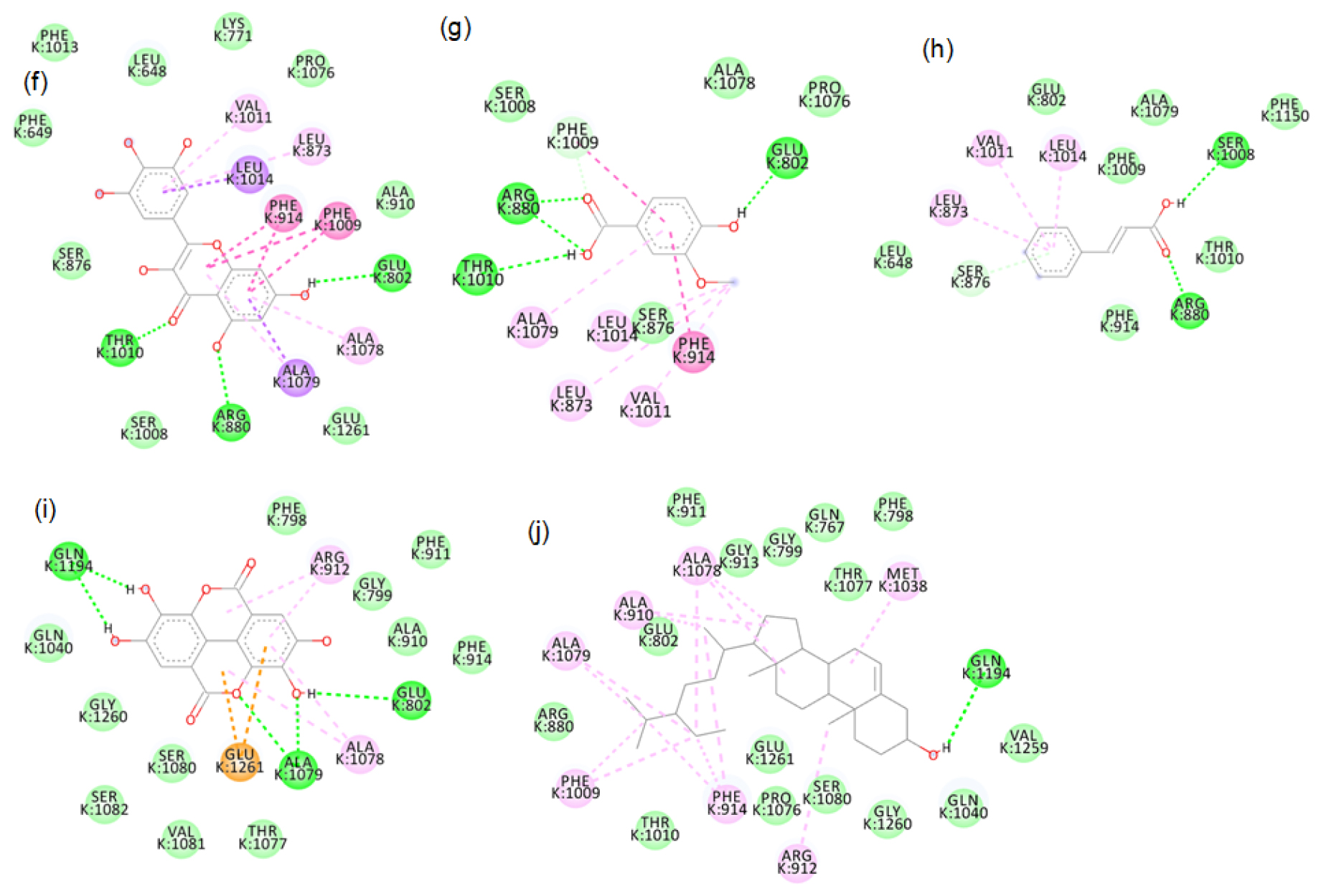
Figure S6:
2D images of the binding of xanthine oxidase (PDB-3B9J) with brown rice- f. Myricetin, g. Vanillic acid, h. Cinnamic acid, I. Ellagic acid, j. β-sitosterol.
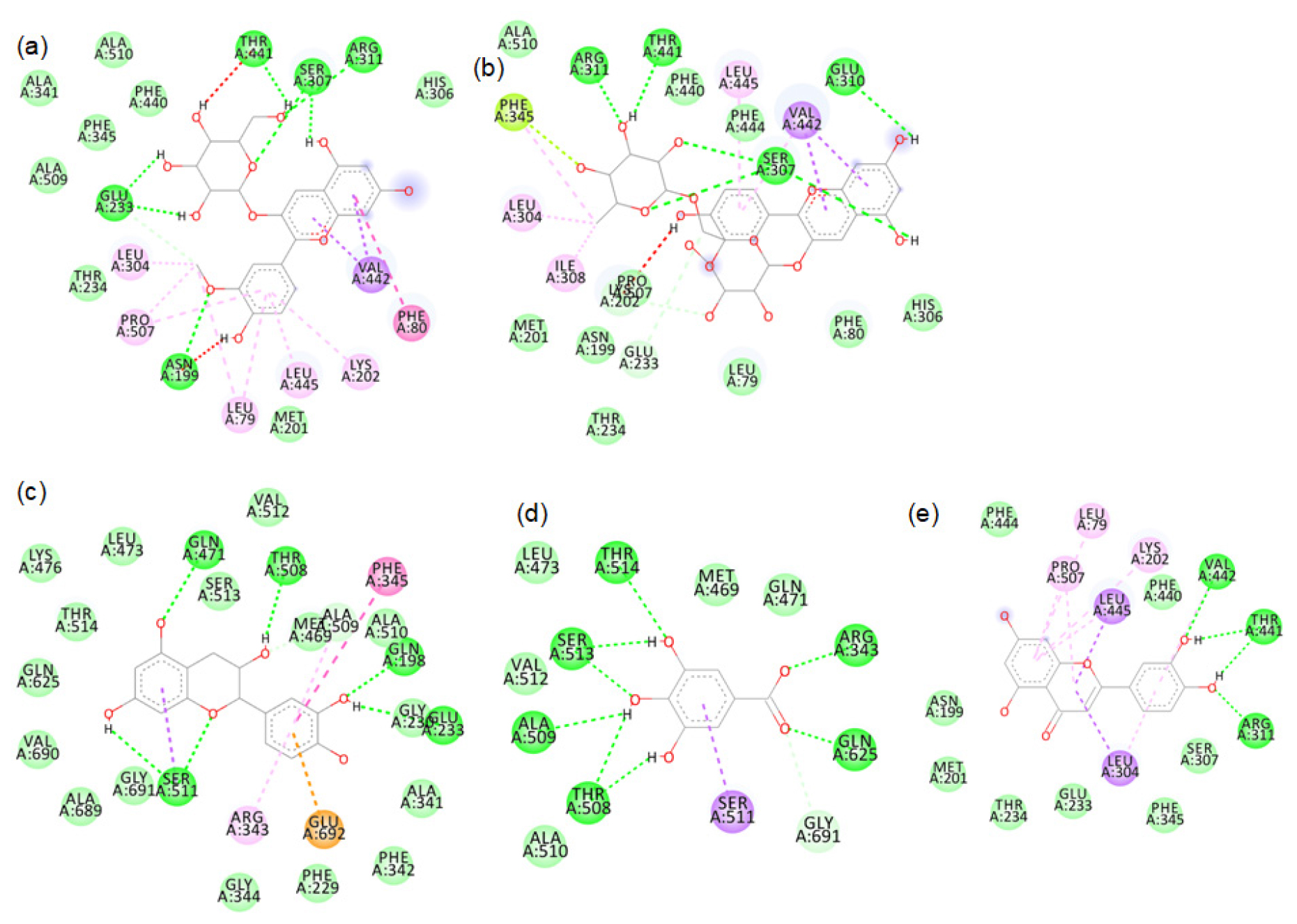
Figure S7:
2D images of the binding of xanthine oxidase (Model) with brown rice- a.Peonidin-3-O-glucoside, b.Cyanidin-3-O-rutinoside, c. Epicatechin, d. Gallic acid, e. Luteolin.
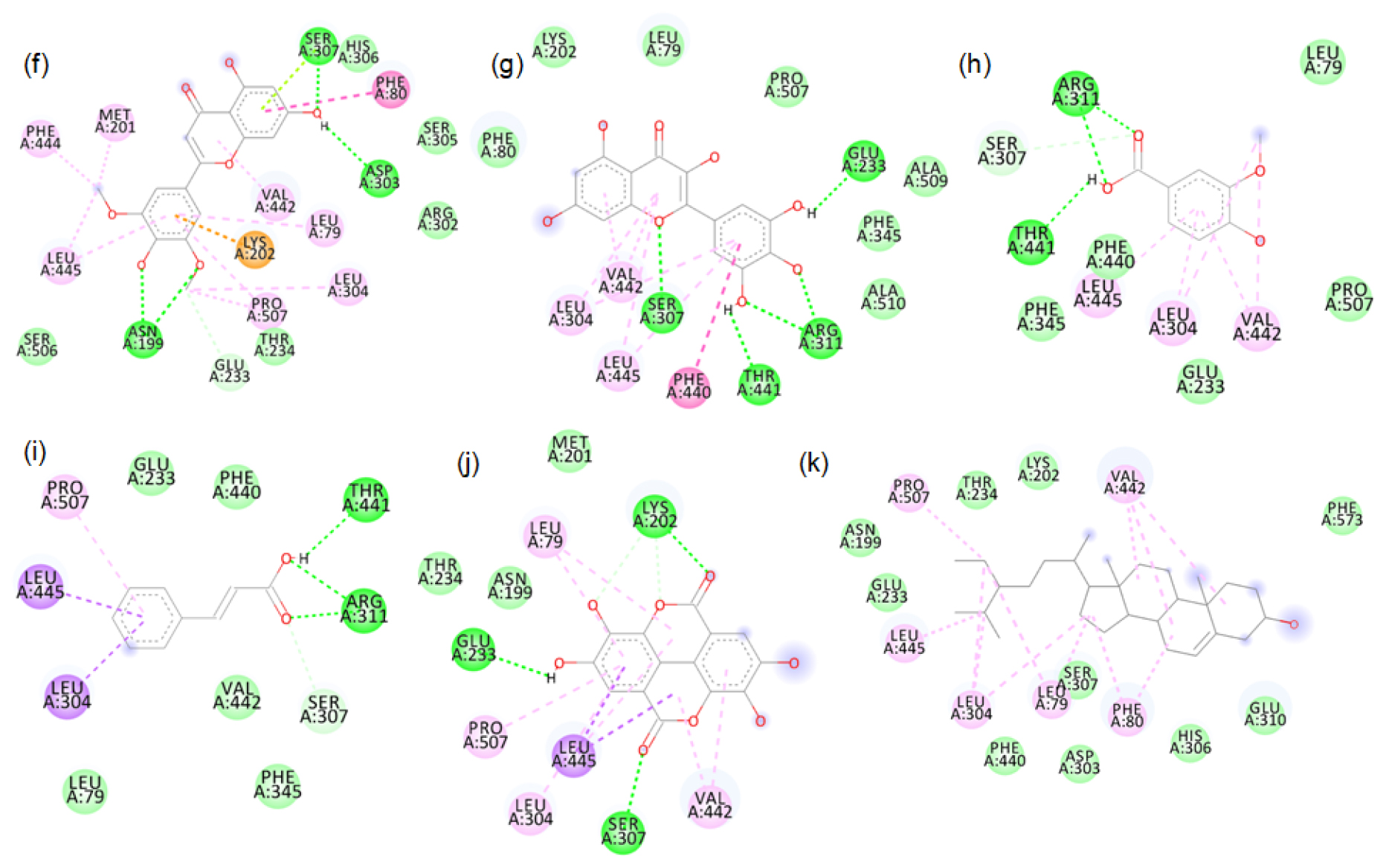
Figure S8:
2D images of the binding of xanthine oxidase (Model) with brown rice- f. Tricin, g. Myricetin, h. Vanillic acid, i. Cinnamic acid, j. Ellagic acid, k. β-sitosterol.
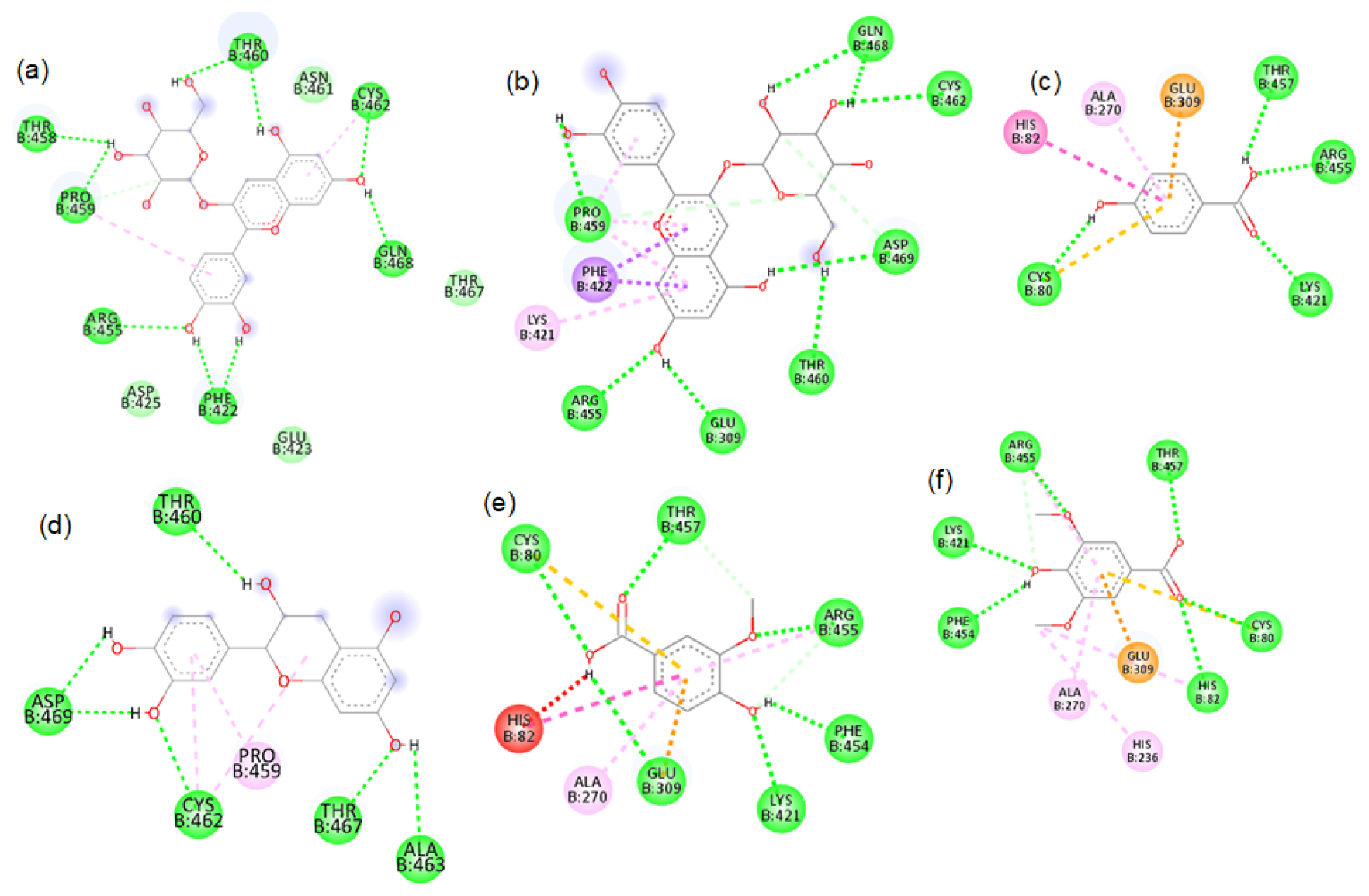
Figure S9:
2D images of the binding of tyrosinase (PDB-4OUA) with black rice- a. cyanidin3 glucoside, b. cyanidin3 galactoside, c. hydroxybenzoic acid, d. cathechin hydrate, e. vallinic acid, f. syringic acid.
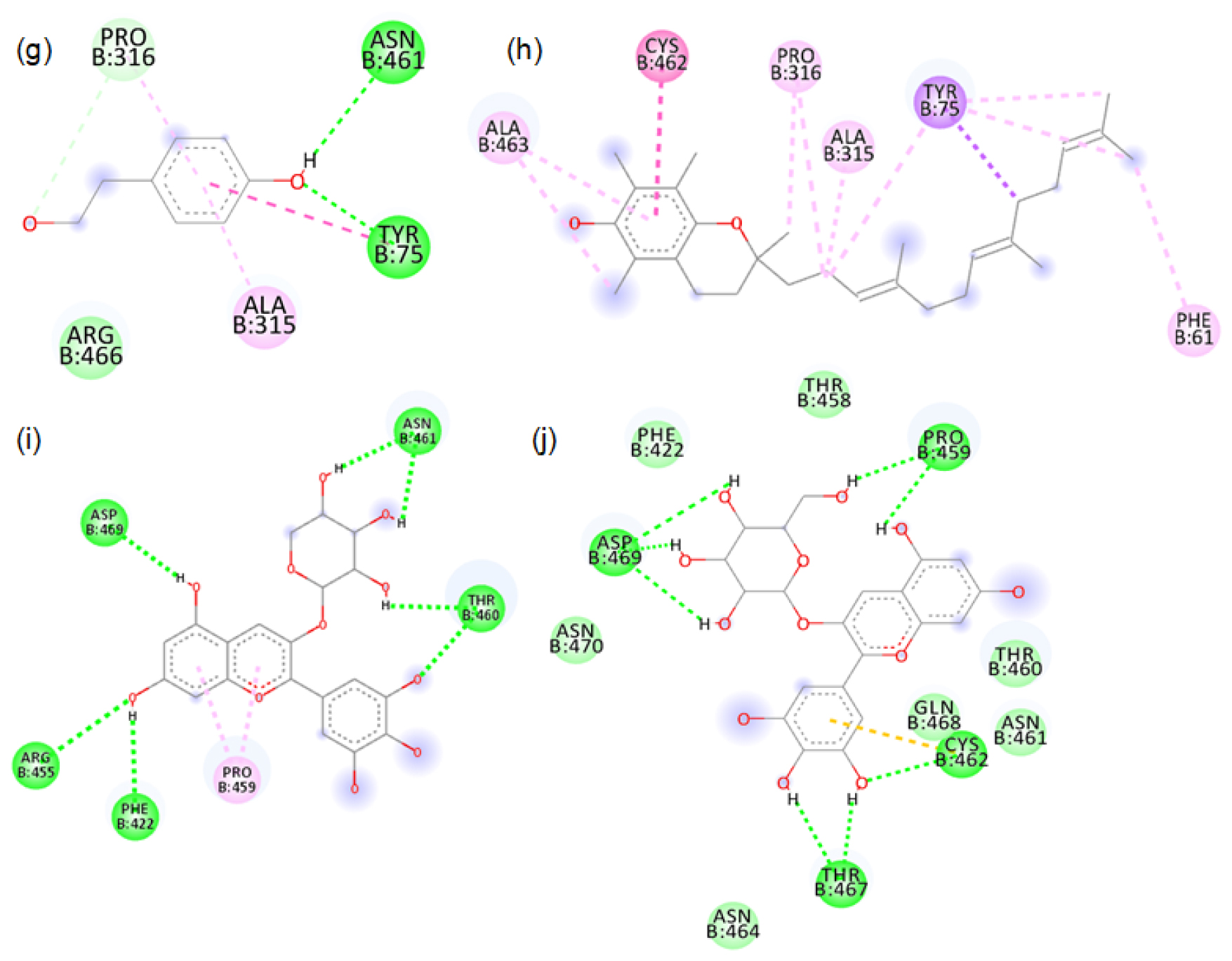
Figure S10:
2D images of the binding of tyrosinase (PDB-4OUA) with black rice- g. p-tyrosol, h. alpha tocopherol, i. Delphinidin-3-arabinoside, j. Delphinidin-3-galactoside.
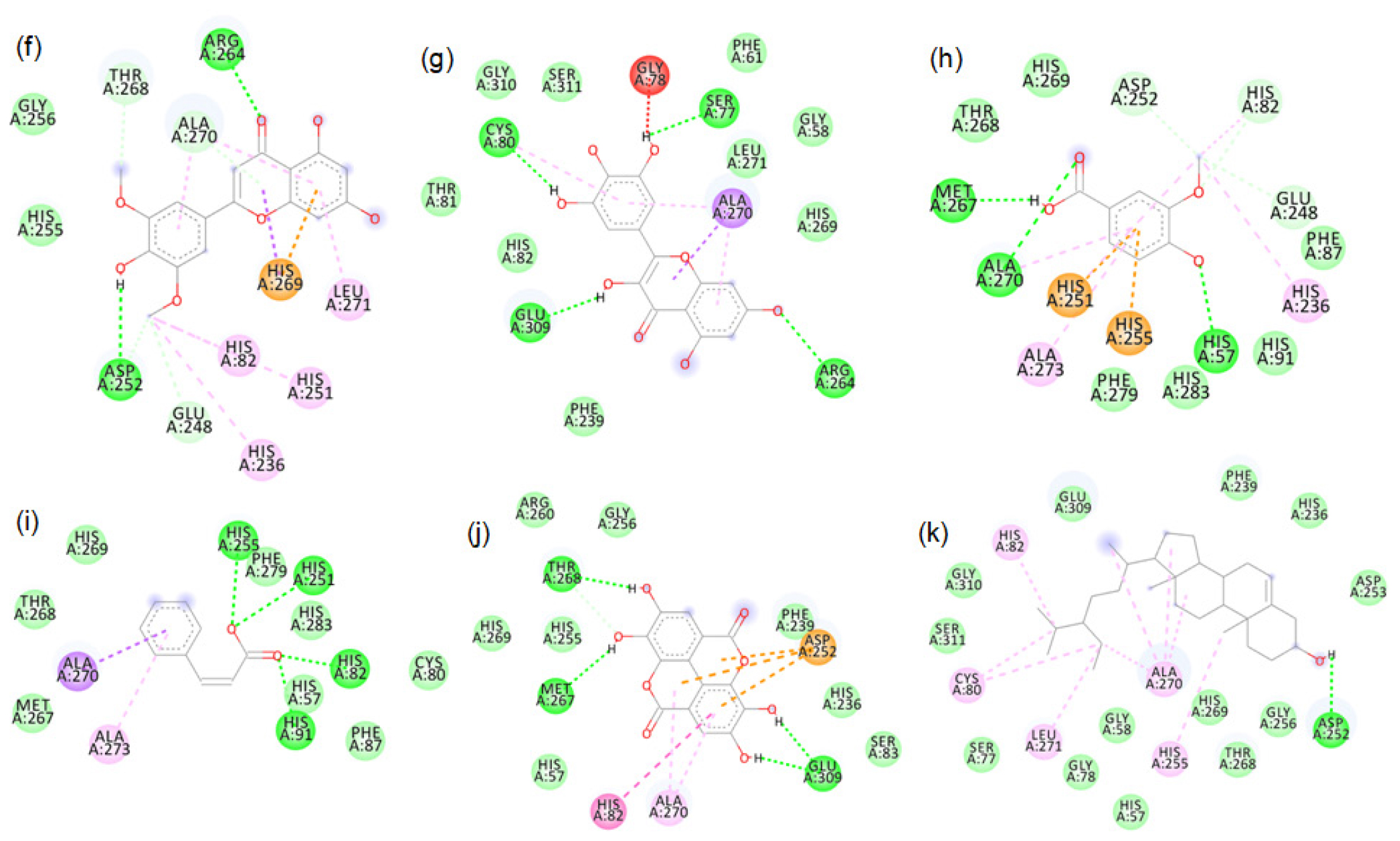
Figure S11:
2D images of the binding of tyrosinase (PDB-4OUA) with brown rice- a.Peonidin-3-O-glucoside, b.Cyanidin-3-O-rutinoside, c. Epicatechin, d. Gallic acid, e. Luteolin.
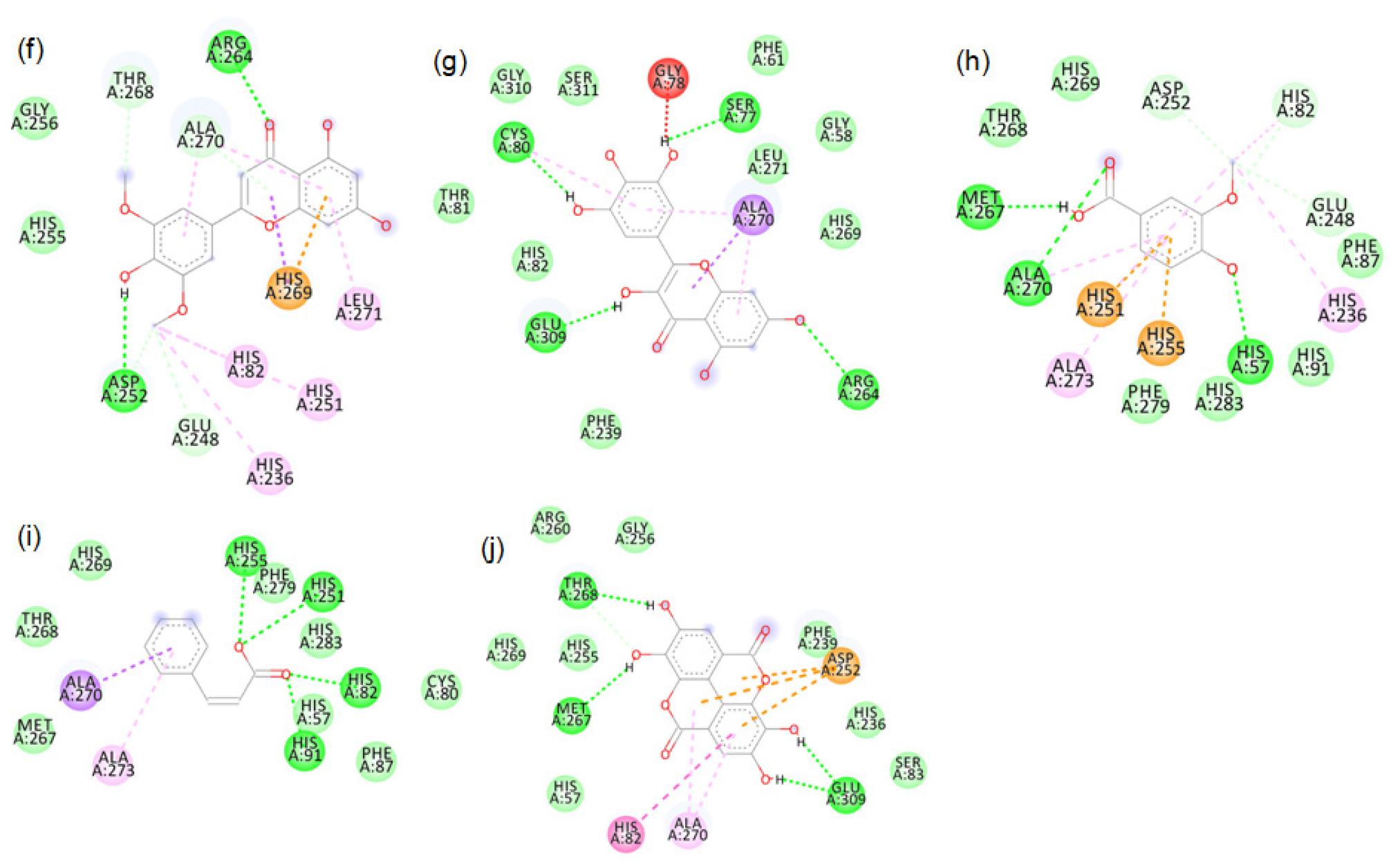
Figure S12:
2D images of the binding of tyrosinase (PDB-4OUA) with brown rice- f. Tricin, g. Myricetin, h. Vanillic acid, i. Cinnamic acid, j. Ellagic acid.
REFERENCES
- Rismana E, Ningsih S, Fachrudin F. In vitro study of xanthine oxidase inhibitory of gambir (Uncaria gambir) hunter roxb extracts. Pharmacogn J. 2017;9(6):862-5. [CrossRef].
- Chang KH, Chen CM. The role of oxidative stress in Parkinson’s disease. Antioxidants (Basel). 2020;9(7):597. [CrossRef], PMID 32650609.
- Kim TY, Leem E, Lee JM, Kim SR. Control of reactive oxygen species for the prevention of Parkinson’s disease: the possible application of flavonoids. Antioxidants (Basel). 2020;9(7):583. [CrossRef], PMID 32635299.
- Aladdin NA, Husain K, Jalil J, Sabandar CW, Jamal JA. Xanthine oxidase inhibitory activity of a new isocoumarin obtained from Marantodes pumilum var. pumila leaves. BMC Complement Med Ther. 2020;20(1):324. [CrossRef], PMID 33109178.
- Di Petrillo A, González-Paramás AM, Era B, Medda R, Pintus F, Santos-Buelga C, et al. Tyrosinase inhibition and antioxidant properties of Asphodelus microcarpus extracts. BMC Complement Altern Med. 2016;16(1):453. [CrossRef], PMID 27829416.
- Dej-Adisai S, Parndaeng K, Wattanapiromsakul C, Nuankaew W, Kang TH. Effects of selected Moraceae plants on tyrosinase enzyme and melanin content. Pharmacogn Mag. 2019;15(65). [CrossRef].
- Carballo-Carbajal I, Laguna A, Romero-Giménez J, Cuadros T, Bové J, Martinez-Vicente M, et al. Brain tyrosinase overexpression implicates age-dependent neuromelanin production in Parkinson’s disease pathogenesis. Nat Commun. 2019;10(1):973. [CrossRef], PMID 30846695.
- Jin W, Stehbens SJ, Barnard RT, Blaskovich MA, Ziora ZM. Dysregulation of tyrosinase activity: a potential link between skin disorders and neurodegeneration. J Pharm Pharmacol. 2024;76(1):13-22. [CrossRef], PMID 38007394.
- Das M, Dash U, Mahanand SS, Nayak PK, Kesavan RK. Black rice: A comprehensive review on its bioactive compounds, potential health benefits and food applications. Food Chem Adv. 2023;3:100462. [CrossRef].
- Rao AS, Reddy SG, Babu PP, Reddy AR. The antioxidant and antiproliferative activities of methanolic extracts from Njavara rice bran. BMC Complement Altern Med. 2010;10:4. [CrossRef], PMID 20109194.
- Di Petrillo A, González-Paramás AM, Era B, Medda R, Pintus F, Santos-Buelga C, et al. Tyrosinase inhibition and antioxidant properties of Asphodelus microcarpus extracts. BMC Complement Altern Med. 2016;16(1):453. [CrossRef], PMID 27829416.
- Azmi SM, Jamal P, Amid A. Xanthine oxidase inhibitory activity from potential Malaysian medicinal plant as remedies for gout. Int Food Res J. 2012;19(1).
- O’Boyle NM, Banck M, James CA, Morley C, Vandermeersch T, Hutchison GR. Open Babel: an open chemical toolbox. J Cheminform. 2011;3(1):33. [CrossRef], PMID 21982300.
- Kim S, Thiessen PA, Bolton EE, Chen J, Fu G, Gindulyte A, et al. PubChem Substance and Compound databases. Nucleic Acids Res. 2016;44(D1):D1202-13. [CrossRef], PMID 26400175.
- Forli S, Huey R, Pique ME, Sanner MF, Goodsell DS, Olson AJ. Computational protein-ligand docking and virtual drug screening with the AutoDock suite. Nat Protoc. 2016;11(5):905-19. [CrossRef], PMID 27077332.
- Kim DE, Chivian D, Baker D. Protein structure prediction and analysis using the Robetta server. Nucleic Acids Res. 2004; 32(Web Server issue):W526-31. [CrossRef], PMID 15215442.
- Abraham MJ, Murtola T, Schulz R, Páll S, Smith JC, Hess B, et al. GROMACS: high performance molecular simulations through multi-level parallelism from laptops to supercomputers. SoftwareX. 2015; 1-2: 19-25. [CrossRef].
- Kitao A, Go N. Investigating protein dynamics in collective coordinate space. Curr Opin Struct Biol. 1999;9(2):164-9. [CrossRef], PMID 10322205.
- Das AB, Goud VV, Das C. Extraction and characterization of phenolic content from purple and black rice (Oryza sativa L) bran and its antioxidant activity. J Food Meas Char. 2018;12(1):332-45. [CrossRef].
- Ravichanthiran K, Ma ZF, Zhang H, Cao Y, Wang CW, Muhammad S, et al. Phytochemical profile of brown rice and its nutrigenomic implications. Antioxidants (Basel). 2018;7(6):71. [CrossRef], PMID 29789516.
- Cicero AF, Fogacci F, Cincione RI, Tocci G, Borghi C. Clinical effects of xanthine oxidase inhibitors in hyperuricemic patients. Med Princ Pract. 2021;30(2):122-30. [CrossRef], PMID 33040063.
- Onul N, Ertik O, Mermer N, Yanardag R. Research Article Synthesis and Biological Evaluation of S-Substituted Perhalo-2-nitrobuta-1, 3-dienes as Novel xanthine oxidase, tyrosinase, elastase and neuraminidase Inhibitors. J Chem. 2018; 2018:1-11. [CrossRef].
- Pauff JM, Zhang J, Bell CE, Hille R. Substrate orientation in xanthine oxidase: crystal structure of enzyme in reaction with 2-hydroxy-6-methylpurine. J Biol Chem. 2008 Feb 22;283(8):4818-24. [CrossRef], PMID 18063585.
- Mauracher SG, Molitor C, Al-Oweini R, Kortz U, Rompel A. Latent and active abPPO4 mushroom tyrosinase cocrystallized with hexatungstotellurate(VI) in a single crystal. Acta Crystallogr D Biol Crystallogr. 2014;70(9):2301-15. [CrossRef], PMID 25195745.
- Wu N, Zhang R, Peng X, Fang L, Chen K, Jestilä JS. Elucidation of protein-ligand interactions by multiple trajectory analysis methods. Phys Chem Chem Phys. 2024;26(8):6903-15. [CrossRef], PMID 38334015.
- Sharma J, Kumar Bhardwaj V, Singh R, Rajendran V, Purohit R, Kumar S. An in silico evaluation of different bioactive molecules of tea for their inhibition potency against non-structural protein-15 of SARS-CoV-2. Food Chem. 2021;346:128933. [CrossRef], PMID 33418408.
- Prieto-Martínez FD, Medina-Franco JL. Flavonoids as putative epi-modulators: insight into their binding mode with BRD4 bromodomains using molecular docking and dynamics. Biomolecules. 2018;8(3):61. [CrossRef], PMID 30041464.
- Sasidharan S, Radhakrishnan K, Lee JY, Saudagar P, Gosu V, Shin D. Molecular dynamics of the ERRγ ligand-binding domain bound with agonist and inverse agonist. PLOS ONE. 2023;18(4):e0283364. [CrossRef], PMID 37023008.