ABSTRACT
INTRODUCTION
The benefit of systemic Donepezil absorption by transdermal patches is that they avoid hepatic first-pass metabolism. They make it possible for drugs to be gradually and carefully released into the bloodstream through unbroken skin.
Aim
The purpose of this study was to use a central composite design to manufacture and describe transdermal patches containing Donepezil (DPZ).
Materials and Methods
Using a solvent-casting technique, PVP K30, HPMC K15, and modified chitosan were used to make the patches. The formulas were created using Design Expert software, which also produced a central composite design, which was used to examine how independent variables affected the result. The FTIR technique was applied to investigate DPZ-excipient interactions.
Results
The patches demonstrated good appeal and uniformity in thickness and content. The folding ability of the patches was more than 150, and the DPZ permeated from the patches was extended beyond 24 h in a controlled manner.
Conclusion
The study concludes that using HPMC K15 (125 mg) and PVP K30 (40 mg) with modified chitosan (65.9 mg) effectively facilitates the permeation for the extended period and flexibility of DPZ transdermal patches.
INTRODUCTION
Unlike oral tablets, injections, and topical creams, transdermal patches provide a novel and efficient way to administer Donepezil (DPZ). Bypassing the gastrointestinal system and hepatic first-pass metabolism, they allow DPZs to enter the bloodstream through the skin and be absorbed directly.1 This enhances DPZ bioavailability and reduces side effects. Transdermal patches provide controlled and sustained DPZ discharge, maintaining steady plasma levels, which is particularly beneficial for DPZs requiring consistent plasma concentrations, unlike oral tablets which can cause fluctuating DPZ levels due to variable absorption rates.2 Additionally non-invasive and with little gastrointestinal side effects, this delivery route improves compliance by making it easier for individuals who avoid injections or have trouble swallowing pills. However, transdermal patches might irritate some patients’ skin, and only DPZs with particular physicochemical characteristics like a low molecular weight and enough lipophilicity that allow them to efficiently enter the skin can be considered useful. Challenges include adhesion problems as well as the complexity and expense of producing transdermal patches. Due to their simplicity of production and convenience, oral tablets are the most widely used dosage form; nonetheless, they suffer from substantial first-pass metabolism and gastrointestinal degradation. Despite offering quick and thorough DPZ absorption, injectables are intrusive, unpleasant, and need to be administered by a physician.
Transdermal patches offer a non-invasive alternative with sustained discharge, although they are not suitable for DPZs needing immediate effects. Topical creams are used for local DPZ delivery and are generally not intended for systemic effects, making them less effective at maintaining consistent plasma DPZ levels compared to transdermal patches. In summary, transdermal patches are beneficial for delivering DPZs that require steady plasma levels and those with significant first-pass metabolism, but they are limited to DPZs that can effectively penetrate the skin and may pose challenges related to skin irritation and adhesion.
Donepezil (DPZ) is a centrally-acting acetylcholinesterase inhibitor primarily used to treat Alzheimer’s disease by elevating cortical acetylcholine levels. Alzheimer’s Disease (AD) is the most prevalent type of senile dementia, impacting 6-8% of individuals over the age of 65 and nearly 30% of those over 85. By inhibiting the enzyme acetylcholinesterase, DPZ enhances cholinergic function, typically deficient in Alzheimer’s patients. This action can potentially improve cognitive function and slow the progression of symptoms associated with the disease, offering a therapeutic benefit to individuals suffering from AD.
Transdermal patches underwent in vitro testing to evaluate their controlled discharge capabilities.3 Multiple experimental observations suggest that DPZ is an excellent candidate for transdermal patches designed to deliver controlled-discharge formulations. A matrix polymer combination of PVP K30, HPMC K15, and modified chitosan was utilized to effectively regulate the discharge of DPZ, demonstrating the potential of this delivery system to enhance the therapeutic management of Alzheimer’s disease by providing a consistent and controlled discharge of the medication over time.
Because variables can be controlled individually and particular impacts may be observed, traditional research methods tend to concentrate on examining the impact of a single variable at a time. Because it makes statistical analysis easier to understand and guarantees that the effects seen are related to the variable under study, this method is chosen. However, as real-world systems frequently contain interactions between numerous variables, this method may have limitations. Independent variable analysis may miss these interactions, which could result in inaccurate or insufficient findings. Therefore, because it enables the simultaneous examination of numerous variables and their interactions, a Design of Experiments (DOE) approach is crucial for multivariate research.
This comprehensive method enhances the accuracy and reliability of the results, providing a more holistic understanding of the system under study.
The purpose of this work was to use a central composite design to construct and characterize DPZ transdermal patches. To improve the patches’ qualities and efficacy, PVP K30, HPMC K15, and modified chitosan were added to the formulation. The utilization of Design Expert software to develop the primary composite design enabled a methodical examination of the impact of various polymers on the patches’ mechanical characteristics and discharge profile. The goal was to develop a controlled-release formulation that would enable the efficient delivery of DPZ through the skin, possibly mitigating the adverse effects of oral treatment and enhancing patient compliance. Tensile strength, discharge kinetics, physical characteristics, and homogeneity of DPZ content were evaluated to characterize the patches and make sure they complied with the specifications needed for transdermal delivery systems.
MATERIALS AND METHODS
Materials
Intas Pharmaceuticals, Ltd., located in Secunderabad, provided DPZ for this study. The necessary polymers, HPMC K15, modified chitosan, and PVP K30, were produced by SD Fine Chem, based in Mumbai, India. Additionally, the cellulose acetate membrane required for the transdermal patches was supplied by Chemtech International, also situated in India. All other chemicals and materials were pharmaceutical grade.
Preparation of the patch
The solvent casting approach was utilized to manufacture transdermal patches that contained DPZ. In a 2:1 ratio, different amounts of HPMC K15, PVP K30, and modified chitosan were dissolved in ethanol and Dichloromethane (DCM). Propylene glycol was added to the mixture and mixed for 30 min using a magnetic stirrer. After that, DPZ was added to the mixture while it was constantly stirred. After that, a petri dish was filled with the polymeric solution containing DPZ, and it was let too dry for 6 hr at room temperature. After drying, the patches were cut into 1×1 cm squares, wrapped in aluminum foil, and stored in desiccators for subsequent analysis.4,5 To assess fifteen distinct blends, a central composite design was utilized. The Design Expert Software (Version 11) was utilized to analyze the DPZ permeation at different times (DP@8 hr, DP@16 hr, and DP@24 hr). The regression analysis yielded significant components that were eliminated sequentially both forward and backward. The final model did not include any factors that had non-significant impacts (p>0.05) on the dependent variables (Table 1).
Formulation | Donepezil (mg) | HPMC K15 (mg) | Modified chitosan (mg) | PVP K30 (mg) | Dichloromethane (mL) | Propylene glycol (mL) |
---|---|---|---|---|---|---|
DTDP-1 | 20 | 100 | 100 | 30 | 25 | 2.5 |
DTDP-2 | 20 | 150 | 100 | 30 | 25 | 2.5 |
DTDP-3 | 20 | 100 | 200 | 30 | 25 | 2.5 |
DTDP-4 | 20 | 150 | 200 | 30 | 25 | 2.5 |
DTDP-5 | 20 | 100 | 100 | 50 | 25 | 2.5 |
DTDP-6 | 20 | 150 | 100 | 50 | 25 | 2.5 |
DTDP-7 | 20 | 100 | 200 | 50 | 25 | 2.5 |
DTDP-8 | 20 | 150 | 200 | 50 | 25 | 2.5 |
DTDP-9 | 20 | 82.95 | 150 | 40 | 25 | 2.5 |
DTDP-10 | 20 | 167.04 | 150 | 40 | 25 | 2.5 |
DTDP-11 | 20 | 125 | 65.91 | 40 | 25 | 2.5 |
DTDP-12 | 20 | 125 | 234.09 | 40 | 25 | 2.5 |
DTDP-13 | 20 | 125 | 150 | 23.18 | 25 | 2.5 |
DTDP-14 | 20 | 125 | 150 | 56.82 | 25 | 2.5 |
DTDP-15 | 20 | 125 | 150 | 40 | 25 | 2.5 |
Different formulae made in the study.
Compatibility studies
Each sample is mixed with dry Potassium Bromide (KBr) powder and compressed into thin, transparent pellets. These pellets are then scanned using an FTIR spectrophotometer in the range of 4000-400 cm-1, with appropriate resolution settings, typically at 4 cm-1. The recorded spectra are analyzed by comparing the characteristic peaks of the pure DPZ and excipients with those of the physical mixtures. Significant shifts, the disappearance of peaks, or the appearance of new peaks in the mixtures’ spectra can indicate potential interactions. For instance, DPZ, when analyzed with HPMC K15, PVP K30, and modified chitosan, should maintain its characteristic peaks without significant changes if no interactions occur. This method provides a non-destructive way to evaluate compatibility, aiding in the formulation of stable and effective dosage forms.
Physical appearance
The color, clarity, flexibility, and smoothness of all Donepezil Transdermal Patch (DTDP) samples were assessed visually. Additionally, the thickness of the DTDP was measured using a micrometer from H. L. Scientific Industries, Ambala Cantt, India.
The measurements were performed three times, and the average value was calculated to obtain a more accurate representation of the thickness. Visual inspection allows for a qualitative assessment of the DTDP samples, with color referring to the perceived hue, clarity to its transparency or lack of opaqueness, flexibility to the material’s ability to bend or deform without breaking, and smoothness to the absence of rough or uneven surfaces. In contrast, the micrometer, a precise measuring instrument, quantitatively determines the thickness of the DTDP samples by measuring the distance between two parallel surfaces with high accuracy. By performing three measurements and calculating the mean value, any measurement errors or variations are minimized, providing a more reliable measurement of the thickness.6,7
Uniformity of weight
Each DTDP sample underwent individual weighing, and the average weight of the five DTDP samples from each batch was determined by adding the weights of the individual samples and dividing by five. This approach offers a standardized assessment of the DTDP sample weights, acknowledging potential differences among individual samples within a batch. It’s crucial to highlight that the random selection of samples and the computation of mean values serve to address potential variations or anomalies within a batch, thereby enhancing the precision and trustworthiness of the measurements.8,9
Folding Endurance
The DTDP film underwent repeated folding at the designated cutting location to evaluate its resilience to multiple pleats without any interruptions or failures. The process entailed folding the film at a precise spot and then unfolding it to its original state.
This folding and unfolding sequence was reiterated numerous times, consistently positioning the film at the same location. The objective was to determine the film’s maximum capacity to endure pleating at that specific spot without experiencing tearing or breakage.10,11
Throughout the experiment, the film likely underwent visual inspection after each pleating cycle to detect any indications of damage or failure. Criteria for identifying interruptions or failures could include visible tears, cracks, or structural deformations that signal the film’s inability to withstand further pleats. By conducting this investigation, researchers aimed to acquire insights into the mechanical characteristics and endurance of the DTDP film.
Such findings hold significance across various domains, including packaging, textiles, and material science, where the ability to withstand repeated folding is paramount. Understanding the film’s resistance to folding provides valuable knowledge for enhancing product durability and performance in practical applications.
Tensile strength
To provide support for the DTDP when placed inside the patch holder, adhesive tape was affixed to one end of the DTDP. At the opposite end, a small pin was inserted into the adhesive tape to maintain its straight alignment as it expanded. Additionally, the adhesive tape was punctured in the region where the hook was inserted.12,13
To measure the tensile strength of the DTDP, a pulley system was employed. One end of the thread was attached to a small pin, which was then passed over a pulley. Weights were attached to the other end of the thread to increase the pulling force on the DTDP. As the weights were gradually added, a small pointer moved along the thread, tracing its path on the baseplate of the graph paper. The breaking force of the DTDP was determined by the amount of weight required to cause it to break.
Moisture content
The DTDP samples are stored at room temperature within desiccators containing CaCl2 to uphold a low-humidity environment. These desiccators aid in moisture absorption from the surroundings, establishing a dry atmosphere around the DTDP samples. The procedure entails regular weighing of the DTDP samples at predetermined intervals. Initially, the samples are weighed while wet, representing their total weight inclusive of any moisture content. Subsequently, the samples undergo drying to eliminate moisture. Once the weight stabilizes across consecutive weighing, indicating no further moisture loss, the samples are deemed to be in their dry state.14 The difference between the wet and dry weights of the DTDP samples signifies the weight of moisture initially present. This variance is then divided by the dry weight and multiplied by 100 to express the moisture content as a percentage.15 Through periodic monitoring of the DTDP sample moisture content, researchers can evaluate the desiccators’ effectiveness and appraise sample stability under specific storage conditions. Such insights are vital for maintaining the quality and integrity of DTDP samples across diverse applications (E.q.1).
Assay
The DTDP was segmented into 1×1 cm2, which were then immersed in a tube containing 100 mL of Phosphate-Buffered Saline (PBS). To facilitate thorough mixing and dissolution, a magnetic bead was employed to stir the tube’s contents. Following the interaction between the DTDP squares and the PBS, the tube’s contents underwent filtration using Whatman filter paper. This filtration step served to segregate any solid particles or insoluble components from the liquid solution. The resulting filtrate, devoid of solid residues, was collected for subsequent analysis.16 A spectrophotometric assessment was performed on the collected solution at a wavelength of 271 nm. This specific wavelength was likely selected due to its correlation with an absorbance peak or characteristic absorption band pertinent to the DTDP or its constituents. The spectrophotometric analysis at 271 nm furnished quantitative insights into the concentration of specific substances or compounds present in the solution. In instances where the obtained result was deemed unreliable, the process was likely iterated until a consistent and dependable outcome was achieved. This iterative approach serves to ensure precision and reproducibility in the spectrophotometric analysis, thereby minimizing potential errors or discrepancies in the measurements.17
In vitro Diffusion Study
In the in vitro diffusion studies described, a Franz diffusion cell system was employed to examine the diffusion of DTDP through a cellophane membrane. The receptor section of the Franz with a pH of 7.4, serving as the receiving medium. The DTDP was firmly applied onto the center of a cellophane membrane, acting as a barrier between the donor and receptor compartments. Positioned within the donor compartment, the DTDP-loaded cellophane membrane was aligned so that it lightly contacted the surface of the receptor compartment. This arrangement facilitated the diffusion of the DPZ through the membrane into the receptor section. To maintain consistent experimental conditions, the entire Franz diffusion cell assembly was immersed in a water bath set at a constant temperature of 32°C. Regular sampling from the receptor compartment was conducted every 16 hr to monitor DTDP diffusion.18 These samples underwent analysis to detect the presence and concentration of the DPZ. Moreover, equal volumes of buffer solution were added to each receptor cell at every interval to uphold a constant volume and create sink conditions conducive to DPZ diffusion. By systematically collecting and analyzing samples at specified intervals, researchers could observe and quantify the diffusion of DTDP across the cellophane membrane and into the receptor compartment.19 This experimental setup facilitated the exploration of DPZ discharge and permeation characteristics, offering insights into factors such as DPZ solubility, concentration gradients, and diffusion kinetics.
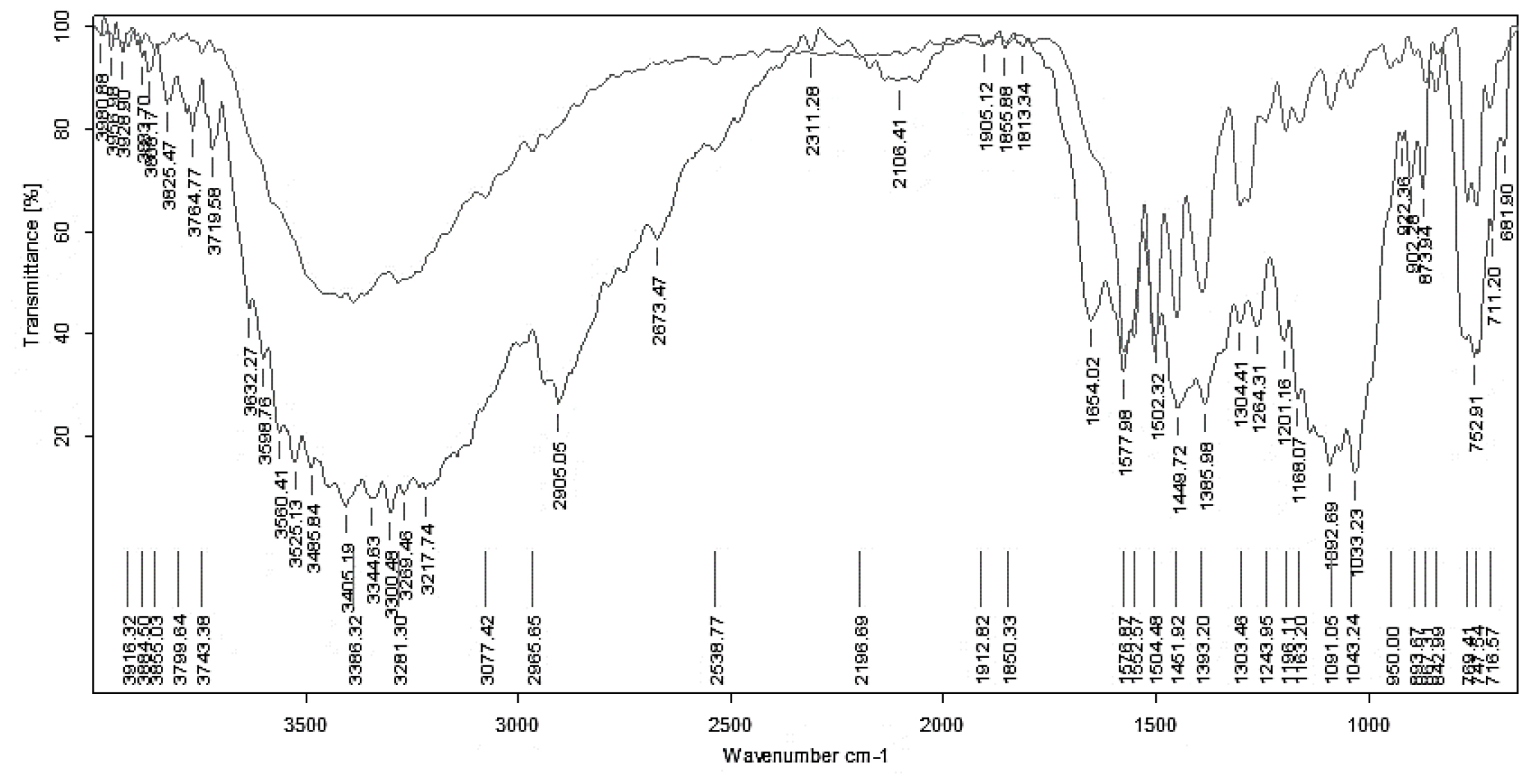
Figure 1:
FTIR spectra of DPZ and its excipients.
RESULTS
In this study, FTIR spectra (Figure 1) were used to assess the compatibility of DPZ with the polymers employed. The characteristic peaks of DPZ remained unchanged when combined with various excipients, indicating no significant interactions or chemical reactions. This preservation of DPZ’s structural integrity confirms its compatibility with the selected excipients, ensuring that the DPZ’s efficacy and stability are maintained. The results demonstrate that the FTIR spectra provide clear evidence of DPZ’s compatibility with the excipients, essential for developing a stable and effective pharmaceutical formulation (Figure 1).
DTDP exhibited satisfactory physical and chemical properties, including appearance, thickness, weight uniformity, Folding Endurance (FE), percentage elongation at break, DPZ content percentage, moisture content, and tensile strength. Specifically, DTDP-11 showed the highest DPZ discharge at 8 hr, 16 hr, and 24 hr. In addition to it, they demonstrated optimal folding characteristics as they were flexible and broke after 161 folding. The tensile strength across all batches ranged from 0.422±0.01 to 0.433±0.02 mg/cm2/hr, and all batches had acceptable moisture content (Table 2).
Formulation | Physical appearance | Thickness (mm) | Uniformity of weight (mg) | Folding endurance | Moisture content (%) | Tensile strength (mg/cm2/hr) | Assay (%) |
---|---|---|---|---|---|---|---|
DTDP-1 | Good | 39.29±0.22 | 704.60±2.53 | 135±2 | 3.3±0.03 | 0.428±0.02 | 93.39±2.36 |
DTDP-2 | Good | 38.72±0.35 | 706.51±5.15 | 160±5 | 3.2±0.06 | 0.426±0.01 | 94.53±5.67 |
DTDP-3 | Good | 39.17±2.02 | 718.44±4.95 | 137±3 | 3.3±0.06 | 0.427±0.01 | 94.32±2.52 |
DTDP-4 | Good | 38.89±1.25 | 711.73±2.57 | 151±2 | 3.5±0.01 | 0.429±0.01 | 96.62±3.48 |
DTDP-5 | Good | 39.54±1.11 | 709.28±1.97 | 134±5 | 3.6±0.04 | 0.431±0.01 | 97.51±2.58 |
DTDP-6 | Good | 36.22±0.89 | 702.71±3.53 | 155±4 | 3.4±0.05 | 0.429±0.02 | 96.84±2.08 |
DTDP-7 | Good | 37.14±0.62 | 702.28±3.67 | 155±2 | 3.1±0.06 | 0.427±0.02 | 96.62±3.59 |
DTDP-8 | Good | 36.07±0.13 | 718.46±6.32 | 154±2 | 3.2±0.03 | 0.428±0.02 | 94.58±4.27 |
DTDP-9 | Excellent | 39.42±0.80 | 709.21±4.80 | 153±4 | 3.3±0.02 | 0.422±0.01 | 97.21±3.01 |
DTDP-10 | Excellent | 37.42±0.67 | 705.77±2.34 | 152±4 | 3.3±0.06 | 0.425±0.01 | 95.85±4.88 |
DTDP-11 | Excellent | 38.52±0.50 | 707.59±1.25 | 161±2 | 3.2±0.04 | 0.433±0.02 | 98.23±5.02 |
DTDP-12 | Excellent | 37.71±0.75 | 707.28±3.40 | 157±2 | 3.1±0.01 | 0.429±0.01 | 96.29±4.67 |
DTDP-13 | Good | 36.66±0.46 | 704.54±2.75 | 158±3 | 3.2±0.04 | 0.427±0.02 | 94.33±3.95 |
DTDP-14 | Good | 38.84±0.95 | 708.29±1.62 | 154±3 | 3.1±0.06 | 0.426±0.03 | 92.52±2.84 |
DTDP-15 | Excellent | 38.51±0.82 | 706.48±3.95 | 152±5 | 3.2±0.05 | 0.423±0.02 | 97.74±4.58 |
Physicochemical of DTDP.
In vitro DPZ permeation
The study conducted in vitro DPZ permeation experiments to assess the controlled discharge capabilities of various patches. Results revealed consistent DPZ permeation across all patches. Notably, formulation DTDP-11, comprising HPMC (125 mg), PVP K30 (40 mg), and modified chitosan (16.91 mg), exhibited superior DPZ discharge compared to other formulations. This suggests that the specific combination of ingredients in DTDP-11 contributed to its enhanced performance in facilitating DPZ discharge (Figure 2).
Model Fit summary
Analysis of the responses (DP@8 hr, DP@16 hr, and DP@24 hr) using Design Expert software yielded the fit summary (Table 3) and ANOVA details (Table 4). Reducing the number of terms in the model can be advantageous if it contains many insignificant terms, provided the hierarchy is maintained. The final equation for the responses is as follows.
Response 1: DP@8 hr | |||
---|---|---|---|
Source | Sequential p -value | Adjusted R2 | Predicted R2 |
Linear | <0.0001 | 0.9716 | 0.9578 |
2FI | 0.9084 | 0.9633 | 0.9474 |
Quadratic | 0.0291 | 0.9890 | 0.9633 |
Cubic | 0.2000 | 0.9990 | 0.9781 |
Response 2: DP@I6 hr | |||
Linear | <0.0001 | 0.9802 | 0.9721 |
2FI | 0.0771 | 0.9879 | 0.9840 |
Quadratic | 0.0215 | 0.9968 | 0.9896 |
Cubic | 0.1754 | 0.9998 | 0.9951 |
Response 3: DP@24 hr | |||
Linear | <0.0001 | 0.9697 | 0.9661 |
2FI | 0.7845 | 0.9633 | 0.9492 |
Quadratic | 0.0074 | 0.9937 | 0.9774 |
Cubic | 0.1870 | 0.9995 | 0.9891 |
Fit Summary of the responses.
Response | F-value | p-value |
---|---|---|
DP@8 hr | 140.46 | <0.0001 |
DP@16 hr | 24.28 | 0.0124 |
DP@24 hr | 246.6 | <0.0001 |
ANOVA for a Quadratic Model.
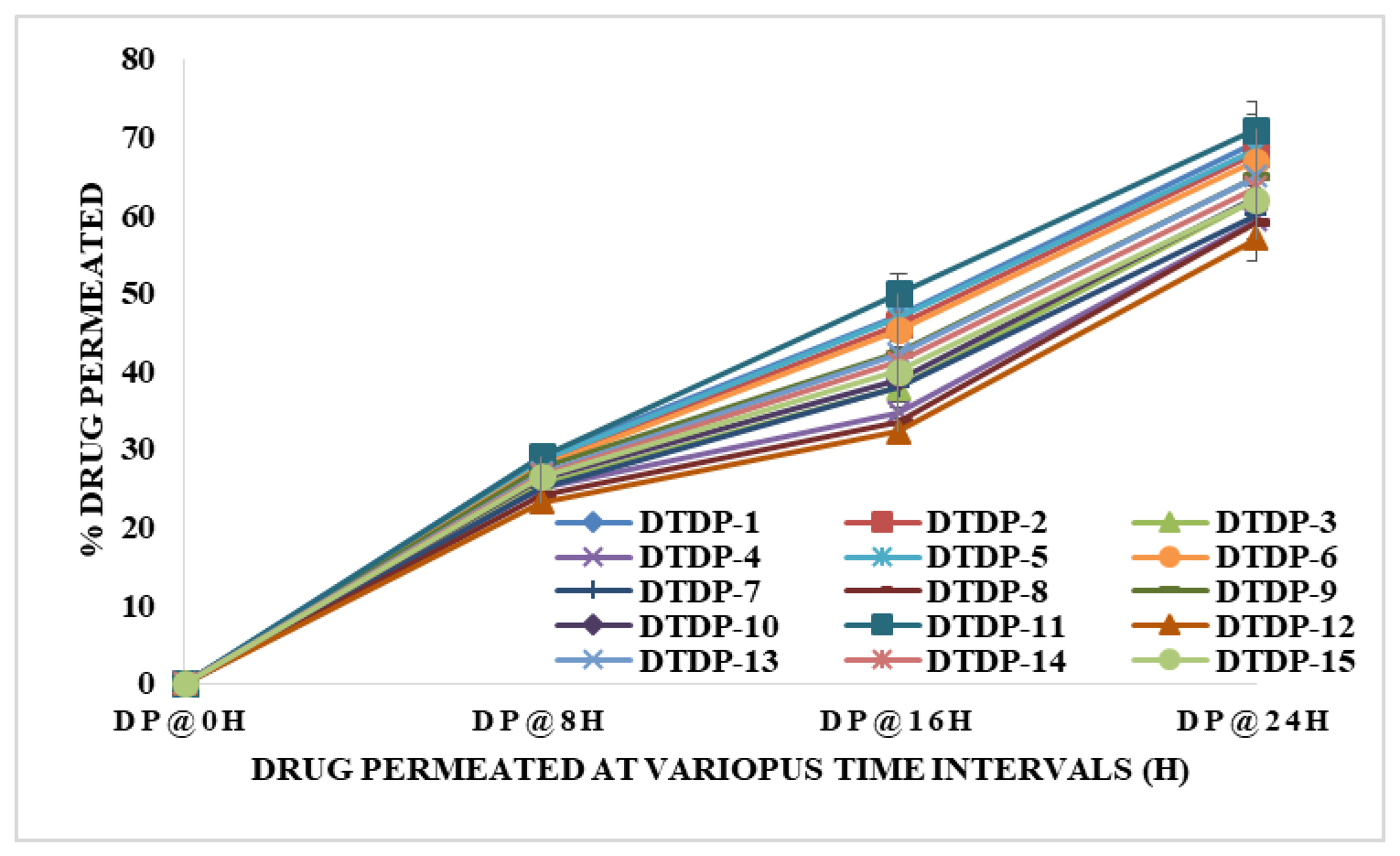
Figure 2:
In vitro drug DPZ permeation from the patches.
The responses (DP@8 hr, DP@16 hr, and DP@24 hr) in points matched the graphs in Figures 3 and 4. There was a close correlation between the residuals and the foreseen appraisals. The residual and predicted values
The Model F-values of 140.46, 483.15, and 246.60 indicate significant models, with only a 0.01% chance of these values occurring due to noise. p-values below 0.0500 mark significant model terms: A, B, and C in the first model; A, B, C, AB, B2, and C2 in the second; and A, B, C, A2, B2, and C2 in the third. Values above 0.1000 denote non-significant terms, suggesting that model reduction may enhance the models if many insignificant terms are present (Table 4).
DISCUSSION
An FTIR study was conducted to assess the chemical and physical interactions between the excipients and DPZ. The IR spectra revealed no changes in peaks and stretches when DPZ was mixed with HPMC K15, PVP K30, and modified chitosan, indicating no physical interaction between DPZ and these polymers.
HPMC is a versatile cellulose derivative commonly used in pharmaceutical formulations. As a matrix former, HPMC plays a crucial role in controlling DPZ discharge rates. This property is particularly beneficial in the design of sustained or controlled-discharge DPZ delivery systems, where maintaining a consistent discharge profile over an extended period is essential for therapeutic efficacy. HPMC achieves this by forming a gel-like matrix upon contact with bodily fluids, which modulates the diffusion of the DPZ, thereby slowing its discharge into the system.
PVP K30 is a versatile polymer known for its excellent swelling properties and ability to form hydrogels, making it particularly effective in transdermal DPZ delivery systems. When PVP K30 hydrates, it swells and retains a significant amount of water, creating a moist environment on the skin surface conducive to DPZ absorption. This water-retentive nature keeps the skin hydrated and soft, increasing its permeability and facilitating easier DPZ penetration through the skin barrier. By maintaining a hydrated environment and softening the skin, PVP K30 enhances DPZ permeation, which is crucial for the therapeutic efficacy of transdermal delivery systems. These characteristics make PVP K30 a valuable excipient for improving DPZ permeation and ensuring better therapeutic outcomes.
Due to its unique properties, modified chitosan is increasingly used in transdermal DPZ delivery systems. It enhances skin permeability, making DPZ penetration more efficient by temporarily disrupting the stratum corneum. Biocompatible and biodegradable, it ensures safety and breaks down harmlessly after use. Its mucoadhesive nature prolongs DPZ retention at the application site, aiding absorption. Modified chitosan can be tailored for controlled DPZ discharge, maintaining therapeutic levels in the bloodstream. Its antimicrobial activity protects against infections, crucial for prolonged skin contact. Chemically modifiable, it offers versatility to enhance solubility, permeability, and other functional aspects, making it an ideal polymer for effective, safe, and patient-friendly transdermal DPZ delivery.
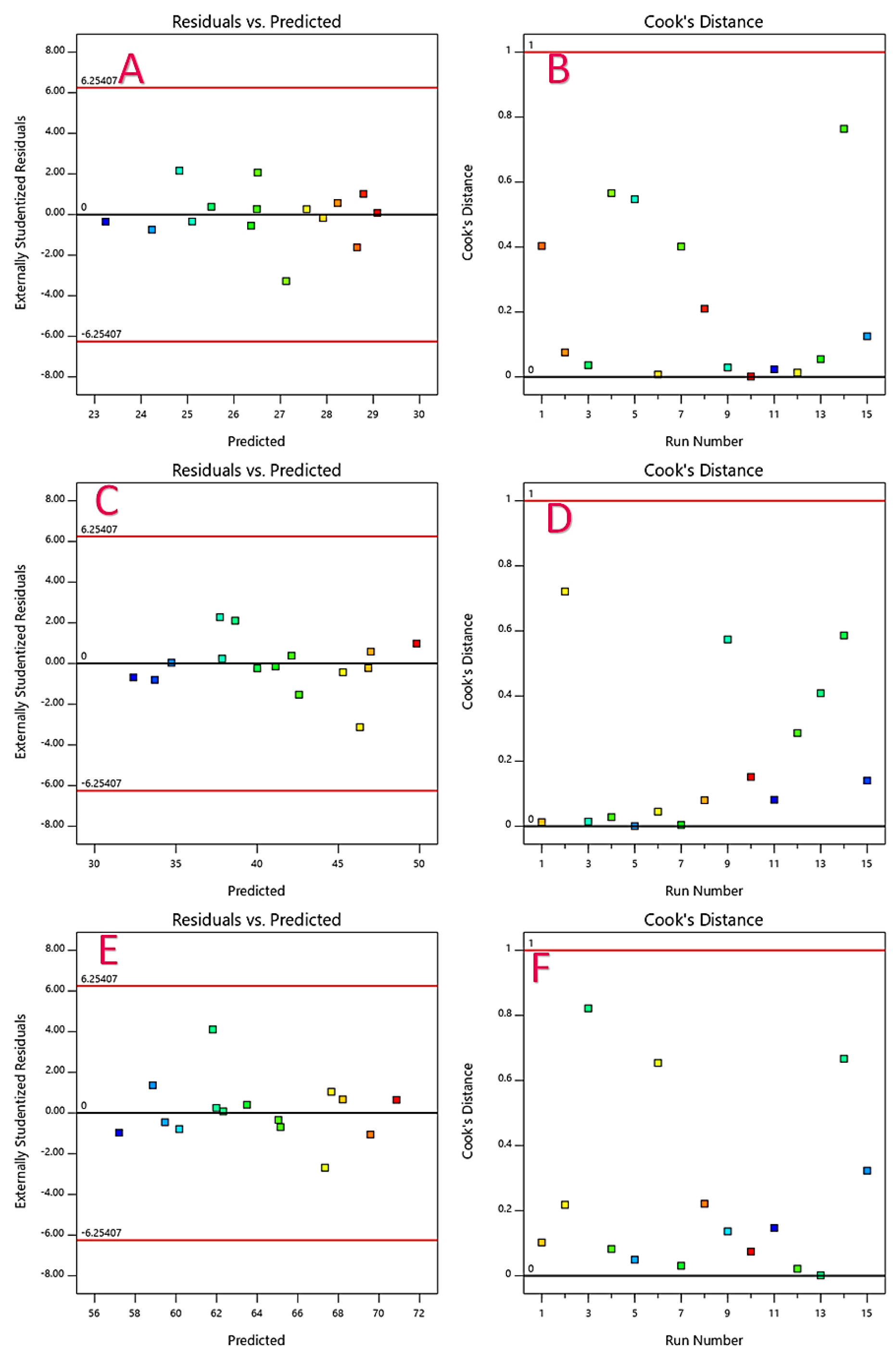
Figure 3:
Residual vs. predicted (A, C and E), Cooks distance (B, D and F) showing the effect of inputs on the response.
Together, HPMC K15, PVP K30, and modified chitosan play crucial roles in the formulation and performance of transdermal patches. HPMC K15 facilitates controlled and sustained DPZ discharge, ensuring consistent therapeutic levels over time. Meanwhile, PVP K30 and modified chitosan enhance DPZ solubility and bioavailability, contributing to improved DPZ absorption and efficacy. These polymers collectively serve as valuable components in the development of efficient and effective transdermal DPZ delivery systems, addressing key challenges in DPZ delivery and enhancing patient outcomes.
The DTDP exhibited desirable characteristics, such as a transparent and uniform appearance without cracks or voids, consistent thickness, uniform weight distribution (ensuring even distribution of DPZ and polymers), good Flexibility (FE), and high % elongation at break (indicating the film’s strength and ability to withstand handling stress). Additionally, the maximal DP@8 hr, DP@16 hr, and DP@24 hr values demonstrated the patch’s effectiveness in ensuring systemic reachability and availability of DPZ over time.
In statistical analysis, the F-value is widely used in ANOVA and regression models to evaluate the overall significance of the model. A low p-value (typically<0.05) indicates that the model or specific terms within it are statistically significant, implying a meaningful relationship between the variables. On the other hand, a high p-value (typically>0.10) suggests that the model or specific terms are not statistically significant, indicating a lack of meaningful relationships. However, without additional details about the specific analysis, variables, and context, it is challenging to provide a comprehensive interpretation of the F-values, p-values, and their implications for your model.
By comparing the coefficients of the coded factors in the equation, one can evaluate and control the virtual impact or influence of each factor on the response variable. Residuals, which are the differences between observed and predicted values, show a strong correlation with the expected appraisals. This indicates that the model effectively explains the variability in the response variable. The Cook’s distances for DP@8 hr, DP@16 hr, and DP@24 hr were below the critical threshold (red line) on the charts, suggesting that the experimental conditions or factor levels significantly influence these responses. Contour and 3D charts provide visual representations of the effects of drug permeation at 8 hr, 16 hr, and 24 hr, aiding in the understanding of the relationships and interactions between factors and response variables.
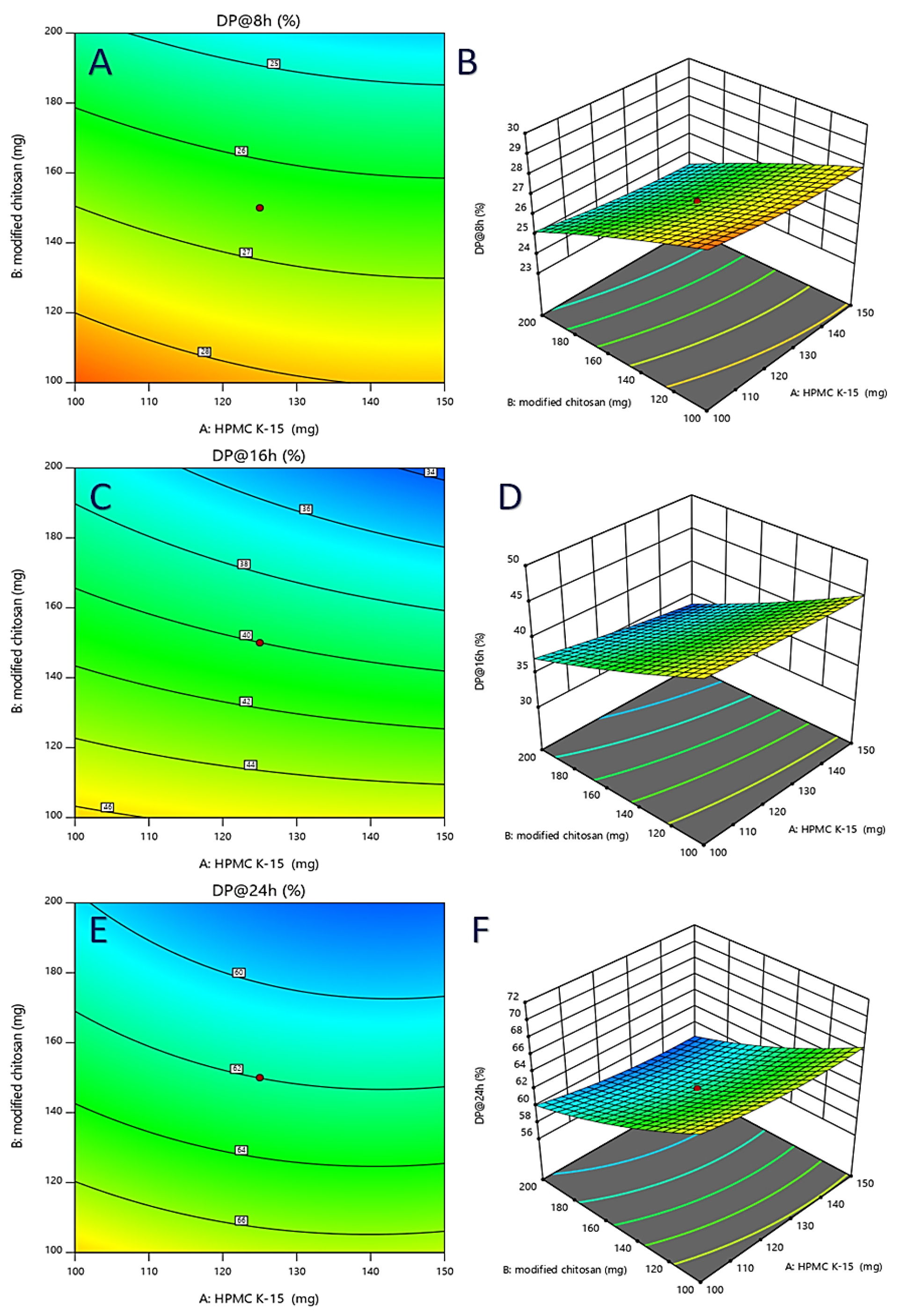
Figure 4:
Response surface plots 2D (A, C and E) and 3D (B, D and F) indicate the effect of inputs on the responses.
The findings suggest that HPMC K15, PVP K30, and modified chitosan have distinct impacts on the response variables under investigation. These results highlight the differential effects of these factors on DP@8 hr, DP@16 hr and DP@24 hr. Overall, the analysis and interpretation of experimental results focus on the effects of coded factors on response variables, with particular attention to how HPMC K15, PVP K30, and modified chitosan influence the outcomes at different time points.
CONCLUSION
The transdermal Donepezil (DPZ) delivery method in question combines modified chitosan, PVP K30, and HPMC K15. This combination has shown promise for the regulated release of DPZ from transdermal patches of the matrix kind. Excipients like PVP K30 and HPMC K15 are frequently utilized in pharmaceutical formulations. A cellulose derivative called HPMC K15 functions as a matrix former and can regulate DPZ discharge rates. The solubility and bioavailability of DPZ were improved by PVP K30 and modified chitosan. Through the use of matrix-type transdermal patches, the transdermal DPZ delivery system hopes to accomplish controlled discharge of DPZ by combining these polymers. Transdermal patches of the matrix type release the DPZ from a polymer matrix upon direct skin contact. The combination of HPMC K15, PVP K30, and modified chitosan in the transdermal DPZ delivery system offers the potential for an extended discharge of DPZ through the skin over a desired period.
Cite this article:
Rajasekhar B, Chinthaginjala H. Improving Transdermal Donepezil Delivery: A Comprehensive Design Expert Screening Method for a Dependable Supply in Alzheimer’s Disease Management. Int. J. Pharm. Investigation. 2025;15(1):103-12.
ACKNOWLEDGEMENT
The authors are thankful to the college management for the support and encouragement.
ABBREVIATIONS
DPZ | Donepezil |
---|---|
PVP | Polyvinyl pyrrolidone |
HPMC | Hydroxy Propyl Methyl Cellulose |
FTIR | Fourier transform Infrared |
AD | Alzheimer’s disease |
DOE | Design of Experiments |
DCM | dichloromethane |
DTDP | Donepezil transdermal patch |
FE | Folding Endurance |
ANOVA | Analysis of Variance |
DP@8 hr | Drug permeated at 8th hr |
DP@16 hr | Drug permeated at 16th hr |
DP@24 hr | Drug permeated at 24th hr |
References
- Seltzer B. Donepezil: an update. Expert Opin Pharmacother. 2007;8(7):1011-23. [PubMed] | [CrossRef] | [Google Scholar]
- Ahad HA, Kumar CS, Ravindra B, Sasidhar C, Ramakrishna G, Venkatnath L, et al. Characterization and permeation studies of diltiazem hydrochloride- fruit mucilage transdermal patches. Int J Pharm Sci Rev Res. 2010;1(2):32-7. [PubMed] | [CrossRef] | [Google Scholar]
- Jyothika LS, Ahad HA, Haranath C, Kousar S, pal , Gowd HD, Sadiya SH, et al. Lakunde Sathish Kumar Jyothika. Array. 2022:157-62. [PubMed] | [CrossRef] | [Google Scholar]
- Kusuma K, Subhash P, Ahad HA, Poojari SJ, Abhishek J. Deciphering the puzzle: A thorough examination of microsphere optimization through factorial design methodology, unveiling novel strategies and promising directions. Nat Campano. 2024;28(1):3143-55. [PubMed] | [CrossRef] | [Google Scholar]
- Ahad HA, Ishaq BM, Shaik M, Bandagisa F. Designing and characterizing of tramadol hydrochloride transdermal patches prepared with fruit mucilage and povidone. Pak J Pharm Sci. 2016;29(3):945-51. [PubMed] | [Google Scholar]
- Sagare A, Yadav R, Gautam V, Yadav S, Malviya J, Sawale J, et al. Development, characterization, and evaluation of niosomal transdermal patch of lamotrigine drug. Macromol Symp. 2024;413(2) [CrossRef] | [Google Scholar]
- Jyothika LS, Ahad HA, Haranath C, Kousar S, Sadiya SH. Types of transdermal drug delivery systems: a literature report of the past decade. 2022 [CrossRef] | [Google Scholar]
- Alhaushey L, Darwish Ahmad RD. Formulation and evaluation of celecoxib transdermal patches. Res J Pharm Technol. 2023;16(4):1574-80. [CrossRef] | [Google Scholar]
- Ahad HA, Kumar CS, Anuradha C, Reddy KK. A Novel Mucilage from Fruits for transdermal Patches: taking indomethacin as a Model Drug: A novel mucilage from fruits. Iran J Pharm Sci. 2011;7(1):25-36. [CrossRef] | [Google Scholar]
- Jajoo VS, Shrirame DS, Sawale AV, Atram SC. Formulation and evaluation of transdermal Patch for the treatment of Migraine. J Drug Deliv Ther. 2023;13(5):47-52. [CrossRef] | [Google Scholar]
- Swetha T, Ahad HA, Reddy KK, Sekhar A, Sivaji S, Kumar B, et al. Formulation and permeation studies of ketoprofen- fruit mucilage transdermal patches. Pharm Lett. 2010;2(6):190-9. [CrossRef] | [Google Scholar]
- Gadag S, Narayan R, Nayak Y, Garg S, Nayak UY. Design, development and evaluation of resveratrol transdermal patches for breast cancer therapy. Int J Pharm. 2023;632:122558 [PubMed] | [CrossRef] | [Google Scholar]
- Shravani Y, Ahad HA, Haranath C, gari , Poojitha B, Rahamathulla S, Rupasree A, et al. Past decade work done on cubosomes using factorial design: A fast-track information for researchers. Int J Life Sci Pharm Res. 2021;11(1):124-35. [PubMed] | [CrossRef] | [Google Scholar]
- Pandey A, Gupta S. Evaluation of formulated transdermal patches. J Popul Ther Clin Pharmacol. 2023;30(16):793-8. [PubMed] | [CrossRef] | [Google Scholar]
- Dinh L, Lee S, Abuzar SM, Park H, Hwang SJ. Formulation, preparation, characterization, and evaluation of dicarboxylic ionic liquid donepezil transdermal patches. Pharmaceutics. 2022;14(1):205 [PubMed] | [CrossRef] | [Google Scholar]
- Haranath C, Poojitha N, Ahad HA, Yarra S, Eranti B. Recent advances in lipid based nanovesicles for transdermal drug delivery. J Med Pharm Allied Sci. 2022;11(6):5217-20. [PubMed] | [CrossRef] | [Google Scholar]
- Adelman M, Louis L. A novel formulation: donepezil patch. Sr Care Pharm. 2023;38(7):300-4. [PubMed] | [CrossRef] | [Google Scholar]
- Sozio P, Cerasa LS, Marinelli L, Di Stefano A. Transdermal donepezil on the treatment of Alzheimer’s disease. Neuropsychiatr Dis Treat. 2012;8:361-8. [PubMed] | [CrossRef] | [Google Scholar]
- Nakamura Y, Kim R, Nishiyama K, Kikuchi T, Ishikawa I, Aoki H, et al. Efficacy and safety of a transdermal donepezil patch in patients with mild-to-moderate Alzheimer’s disease: A 24-week, randomized, multicenter, double-blind, parallel group, non- inferiority study. Geriatr Gerontol Int. 2023;23(4):275-81. [PubMed] | [CrossRef] | [Google Scholar]