ABSTRACT
Background
One of the etiologies of stroke is intracerebral hemorrhage and the demand for potential therapeutic agents to alleviate symptoms and improve quality of life in patients with ICH is growing. Several phytocompounds capable of such potency with minimal adverse effects can replace drugs in market. This requires high throughput screening and in vivo experimental demonstration of the potency in ICH models that simulate mammalian pathophysiology. We aimed to study the neuroprotective activity of Asiaticoside using an adult zebrafish ICH model.
Materials and Methods
Atorvastatin-induced ICH models were treated with non-toxic concentrations of Asiaticoside. Behavioural, biochemical, and cellular assays were performed to validate the developed model. Antioxidant potency was identified by testing the levels of lipid peroxidation, superoxide dismutase and xanthine oxidase in the brain homogenate. Iron chelating potency was assessed in Perl’s-stained brain tissue sections. The anti-inflammatory potency was observed by measuring fold change in the expression of inflammatory gene IL-1β in brain homogenates by RT-PCR method and by immunohistochemistry techniques. The neuroprotective role of the compound was studied by fluorescent staining of the brain whole cell suspension to check apoptotic and necrotic population post-treatment.
Results and Discussion
The iron accumulation in tissue sections and brain homogenates reduced 3-fold post treatment. With 600 μg/mL concentration of Asiaticoside, the malondialdehyde and xanthine levels reduced 3-fold, and scavenging activity was found to increase after 24 hr, indicating antioxidant potency. This concentration also resulted in one third reduction in the expression of pro-inflammatory cytokine IL-1β and reduced inflammatory cell infiltration showing anti-inflammatory properties. The compound was found to be neuroprotective by reducing the apoptotic cell population from 84% to 20% in comparison to untreated control. In addition, post-treatment the nitric oxide levels were restored better than the untreated control indicating modulation in cellular signalling mechanisms.
Conclusion
The adult Zebrafish ICH model was developed to present the multifaceted mammalian pathophysiology of ICH in one system. The phytocompound Asiaticoside is shown to modulate neuroinflammation through a cycle of biological properties thus being neuroprotective, opening avenues for further pre-clinical testing.
INTRODUCTION
Intracerebral Hemorrhage (ICH) is a pathological condition of leakage of blood from the vessels resulting in accumulation and hematoma formation that may further progress into stroke1,2 The two etiologies of ICH are trauma and hypertension based on which they are classified as traumatic ICH and spontaneous ICH respectively. This form of stroke caused by spontaneous Intracerebral Hemorrhage (ICH) is more fatal than the other resulting in over 50% mortality in Western population and 20% mortality in the Asian population.3 The other causes of spontaneous ICH may include Cerebral Amyloid Angiopathy (CAA), vasculitis and arterio-venous malformation. Even with all the data that we have today, there is still a lag in knowledge on diagnostic and prognostic markers in case of ICH.4–6 Majority of the patients post-ICH experience a high functional dependence and many secondary inflammatory consequences like brain oedema, psychosis and seizures7,8 injury and triggers inflammation in perihematomal regions, leading to secondary inflammatory responses.9
ICH-induced Secondary Inflammation
Initially, there is leakage of blood from the vessels due to chronic hypertension, followed by the formation of hematoma around the site of haemorrhage. Secondly, an increase in the size of the hematoma leads to increased intracranial pressure and mechanical obstruction of neurotransmitter release. This aggravates neuronal Secondary inflammation results in the activation of the coagulation cascade and further stimulates the release of thrombin in the perihematomal region; this thrombin exhibits its action through PAR 1 receptor binding which activates microglial cells and complementary system. Additionally, hemolysis at the perihematomal region leads to the breakdown of haemoglobin into heme and globin. This heme is converted into ferric iron and stimulates cell death by ferroptosis.10–12
Microglia cells which are activated by the thrombin exhibit M1 and M2 phenotypes. The former serves as a pro-inflammatory cell and activates neuroinflammation via various mechanisms. This phenotype is the one that is responsible for the secondary inflammation in ICH. The latter is anti-inflammatory and phagocytic in nature, resolving the inflammation that was initially caused by the pro-inflammatory microglial cells.13,14 The infiltration of the immune cells and the released cytokines are eliminated by these cells.15–17
Natural Compounds to Manage ICH and the Need for a Model
Currently used drugs in therapy for ICH like levetiracetam, mannitol, frusemide citicoline and calcium channel blockers are associated with some serious side effects like anaphylactic reactions, rebound brain edema and electrolyte imbalance which may be fatal to the patients.5,18–20 For the aforementioned reasons, the use of phytocompounds which are naturally extracted from the plants seems to be fruitful. Asiaticoside, is a triterpenoid extracted from the plant Centella asiatica. The compound has been reported with anti-inflammatory and anti-oxidant properties.21–23 An effective model is the prerequisite to identifying the biological properties of such phytocompounds. It is evident that Zebrafish brain development occurs in sequential developmental stages. While the larval model has the limitation of an underdeveloped cranium and blood-brain barrier, the adult supports the establishment of ICH and portrays the secondary inflammation complications similar to humans.24–26Atorvastatin, a hypolipidemic drug acts by inhibiting the enzyme HMG CoA reductase. This drug at certain concentrations is understood to disrupt the tight junctions between the endothelial cells and produce significant haemorrhage in Zebrafish embryos and larvae.27 The study has established atorvastatin-induced ICH in adult Zebrafish.
MATERIALS AND METHODS
Maintenance of Adult Zebrafish
Adult Zebrafish (Danio rerio) was maintained at 28-30°C under standard conditions. All the procedures were performed according to the OECD guidelines with approval from the Institutional Animal Ethics Committee (IAEC/66/SRIHER/766/2022).28 Wildtype adult Zebrafish of age 3-6 months were used for the study. The fish were fed with multinutrient micro pellets and freeze-dried worms. The tanks were maintained in 10 hr light-dark cycle with sufficient aeration using motor-powered pumps.
Toxicity Assessments
The Zebrafish were treated with 600, 800, 1000 μg/mL concentrations of Asiaticoside (Merck 16830-15-2) through oral pool administration. After 24 hr, the fish were observed for behavioral and phenotypic changes. Distribution parameters like schooling/shoaling behaviour and surface/bottom distribution was observed and noted. Spiral swimming, hyperactivity, hypoactivity, aggression, convulsions and avoidance behaviour were observed as a part of behavioural analysis.28 Changes in appearance like irritated skin, Edema, skin colour (darkening/ lightening), hemorrhage (petechias/haematomas) and exophthalmia were also noted for assessing the toxicity of the compound. Genotoxicity was assessed by Comet assay.
Comet Assay
The assay was performed according to the protocol by Jarvis et al. (2003).29 Briefly, caudal vein blood of 50 μL was added with low melting agarose. Then the content was layered on a pre-coated slide and soaked in a lysis buffer. Electrophoresis was done and comets were observed, if any.
ICH Induction
The fish were exposed to atorvastatin for 8 hr in tank water and observed for indications of haemorrhage. The brain dissected from the fish was observed as whole or slices under a stereo zoom microscope for haemorrhage.26
Assessing the Therapeutic Effects of Asiaticoside on Intracerebral Hemorrhage
The fish were grouped into three groups one healthy control group without ICH and one untreated control group with ICH (negative control) and the 3rd experimental group with ICH and Asiaticoside treatment each with thirty fish. The experimental groups were treated with 600, 800, and 1000 μg/mL concentrations of Asiaticoside after the induction period. The iron chelation, anti-oxidant and anti-inflammatory potency of the compound were measured in the experimental and control groups.
Iron accumulation and Iron Chelation
Iron accumulation in the brain tissue was observed in all the groups by Prussian blue staining performed following the standard protocol.30 The brain tissue sections fixed on a glass slide were stained with potassium ferrocyanide solution and counter-stained with nuclear fast red. The resultant pictures were quantified for the intensity of blue color using NIH software ImageJ. The iron chelating activity of Asiaticoside was also investigated by the ferrozine assay using brain homogenates.31
Histopathology and Immunohistochemistry
The tissue sectioning was performed and the slides were stained with hematoxylin and eosin stains following standard protocol.32 To specifically identify microglial cell infiltration immunohistochemistry of the tissue sections was done using microglia-specific marker CD68.33 The brown regions in the sections were determined to be an indicator of microglial cell accumulation.
Determination of Antioxidant Potency
Lipid Peroxidation Assay
Lipid peroxidation was measured following Ohkawa et al. (1979).34 Briefly, 20 μL of brain homogenate and 40 μL of TBA/ TCA/HCl reaction mixture were added. After centrifugation, the supernatant was read at 540 nm in a spectrophotometer.
Superoxide Dismutase Assay
Superoxide dismutase levels were measured following Xu et al. (2013).35 Briefly, 50 μL of the homogenate was added to the wells. The same volume of the pyrogallol; Tris-HCl; EDTA mixture was added. The absorbance of the solutions was measured in a spectrophotometer at 420 nm.
Xanthine Oxidase Assay
Xanthine oxidase was measured following Litwack et al. (1953).36 Briefly, the homogenate and the xanthine mixture were incubated at 37°C for 10 min. After the incubation sodium potassium buffer and sodium tungstate along with 2N Sulphuric acid were added sequentially to the homogenate. This mixture was spun at 5000 rpm for 10 min. Folin’s reagent was added to the supernatant and the absorbance was measured at 660 nm.
Apoptosis Assay
Suspension of intact brain cells with 90% viability was prepared and stained with acridine orange and ethidium bromide following the standard protocol.37 The stained cells were observed for fluorescence under the inverted microscope (Nikon Eclipse Ti-U).
Gene Expression Analysis
The level of pro-inflammatory cytokine IL-1β was measured using RT-PCR of the brain tissues from the experimental and control groups.25
Estimation of Nitrite Levels
Nitrite estimation was done according to the standard protocol.38 Briefly, 20 μL of homogenate was added with 40 μL of Griess reagent and read at 540 nm spectrophotometrically.
RESULTS
The fish showed no signs of toxicity up to exposure concentrations of 1000 μg/mL of Asiaticoside. The physical, behavioral and genotoxic observations showed no toxicity (Figure 1A and Table 1).
Toxicity Assessments | 500 μg/mL | 750 μg/mL | 1000 μg/mL |
---|---|---|---|
Normal behavior (Schooling and Shoaling) | Normal behavior was maintained. | Normal behavior was maintained. | Normal behavior was maintained. |
Hyperactivity | None observed. | Observed in negligible level. | Observed in negligible level. |
Appearance | No visible changes. | No visible changes. | No visible changes. |
Genotoxic changes | No comets observed. | No comets observed. | No comets observed. |
Toxicity assessment of Asiaticoside: Physical, behavioral and genotoxic observations.
ICH in Adult Zebrafish
ICH was successfully induced in adult Zebrafish using the drug Atorvastatin at the dosage of 1000 μM. After 6 hr of exposure, atorvastatin at 1000 μM induced multiple hemorrhage in the brain as shown in Figure 1B and 1D.
Validation of ICH Induction
Zebrafish brain was visualized under stereomicroscope (Nikon SMZ-745) and regions of haemorrhage were observed in whole brain and brain slices (Figure 1B).
Accumulation of iron was visualized under microscope using Perl’s staining. The extracellular ferrous content can indicate the presence of hemorrhage and following hemolysis.
When cell viability staining was performed by using AO/EB we observed that a significant number of cells were red or orange 6 hr post induction indicating the presence of ICH.
Asiaticoside Chelates Iron Accumulation Following Haemorrhage
Iron chelating activity of Asiaticoside was measured by estimating the amount of iron present in the brain homogenate. With time of exposure to Asiaticoside, the iron content was found to reduce 3-fold after 30 hr of exposure at 600 μg/mL (Figure 1F).
Prussian blue staining done in the tissue sections showed reduction in the iron accumulation 1.1 folds when compared with the untreated groups (Figure 1C and 1E).
Asiaticoside treatment curtails secondary inflammation following haemorrhage
The number of elongated active microglial cells was recorded. The infiltration of inflammatory cells in the brain tissue sections was observed to reduce in the fish treated with 600 μg/mL of Asiaticoside (Figure 2A). The inflammatory cell count was reduced 1.2 fold after treatment with 600 μg/mL of Asiaticoside (Figure 2C).
Immunohistochemistry of the sectioned brain tissues also showed the presence of microglial cells. The microglial population reduced to half of its initial count after treatment with Asiaticoside (Figure 2D).
Asiaticoside Exhibits Antioxidant Activity Following Haemorrhage
The results indicated a considerable increase in SOD activity 8 hr post induction in both 600 μg/mL and 1000 μg/mL concentrations of Asiaticoside treatment. 24 hr post induction there is a significant increase in SOD activity in all the three concentrations (Figure 3D).
Malondialdehyde (MDA) was found to reduce by 6-folds in 600 μg/mL treatment while with 800 μg/mL and 1000 μg/mL the decrease was 2 and 3-fold respectively. This is indicative of significant reduction in the lipid peroxidation at 600 μg/mL of Asiaticoside when compared to the other two concentrations (Figure 3B).
Xanthine oxidase enzyme activity was measured by estimating the amount of xanthine present in the brain homogenate. Asiaticoside concentration of 600 μg/mL showed significant decrease of 3-fold in the xanthine concentration between 24-30 hr compared to the other two concentrations (Figure 3C).
Gene Expression Analysis
There was 1.7-fold decrease in the levels of IL-1β after treatment with 600 μM concentration of Asiaticoside. In comparison to the negative control, the Asiaticoside treatment was found to regulate the expression of the inflammatory cytokine. The levels of the cytokine in the treated sample were found to be the same as the healthy control 6 hr post treatment and remained the same 30 hr post treatment (Figure 2B). This indicated the potency of Asiaticoside to resolve secondary inflammation following haemorrhage and bring back healthy physiological conditions in the brain tissue.
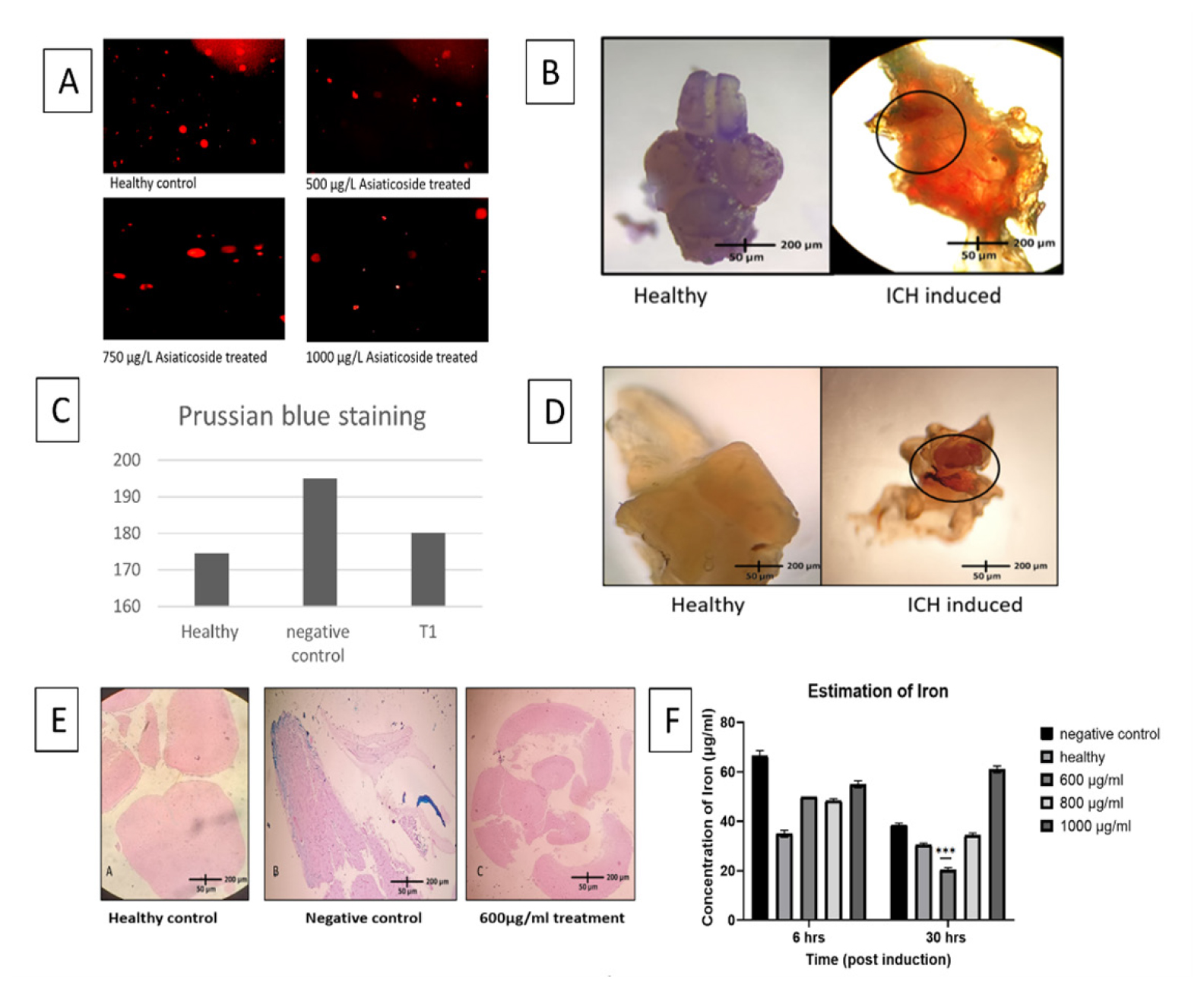
Figure 1:
A) Different concentrations of Asiaticoside showing no genotoxic changes. B) Induction of Intracerebral hemorrhage validated by stereomicroscope showing hemorrhagic regions (rounded regions) in both brain slices (D) and whole brain (B). E) Iron accumulation seen in Prussian blue staining over different groups. C) Graph showing the intensity of iron accumulation in the slides across the groups (analysed through ImageJ software). F) Mean±SD graph showing iron content in the brain homogenate observed after 6 hr and 30 hr of exposure to Asiaticoside.
Asiaticoside Exhibits Neuroprotective Activity by Preventing Apoptosis
Nitric oxide levels in the homogenate were measured to investigate the normal homeostatic signalling. The results showed increased nitrite levels in almost all the concentrations of Asiaticoside (Figure 3A).
Acridine orange/ethidium bromide staining was performed by preparing a cell suspension from the zebrafish brain tissue. The apoptosis is measured in terms of a number of dead cells per unit area which is 80-100 in the first 8 hr and >130 in the next 16 hr in the untreated samples. The results displayed a 2-fold reduction in the number of dead cells in treated samples when compared to the untreated samples (Figure 4).
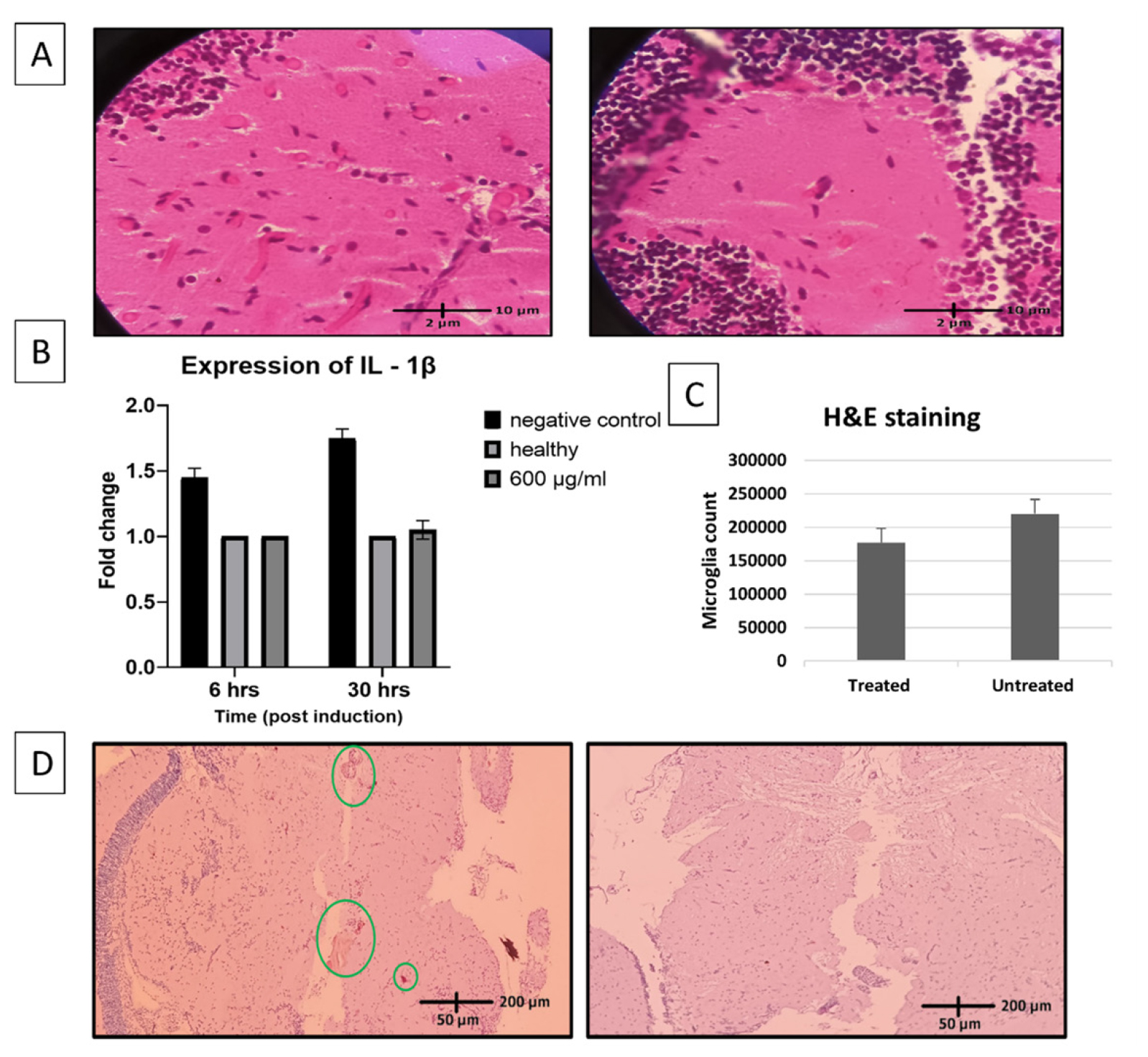
Figure 2:
Anti-inflammatory potency of Asiaticoside validated through histopathological sectioning and gene expression analysis. A-microglial cell infiltration (long elongated cells) seen through histopathological sectioning (left-negative control group; right-600 μg/mL treated). B-Mean±SD graph showing expression of IL-1β levels in the brain homogenate observed after 6 hr and 30 hr of exposure to Asiaticoside. C- Mean±SD Graph showing microglial cell count (cells/unit area) between treated and untreated tissue sections. D-Immunohistochemistry done with CD68 marker showing accumulation of microglial cells (left-negative control; right-600μg/mL).
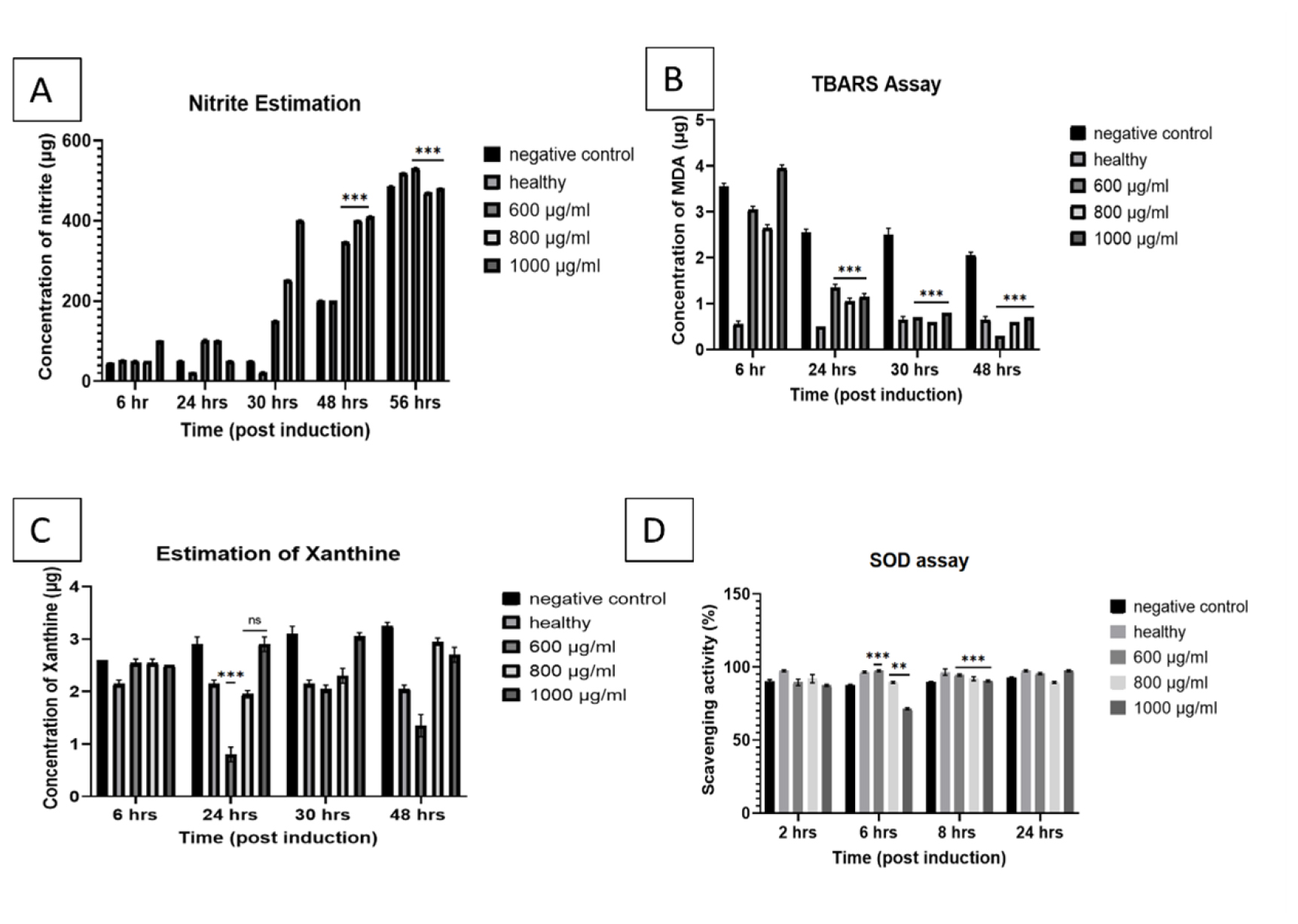
Figure 3:
Antioxidant potency validated through estimation of different oxidative markers. A) Mean±SD graph showing Nitrite concentration in the brain homogenate from 6 hr post induction to 56 hr of exposure of Asiaticoside at different dosages. B) Mean± SD graph showing MDA concentration in the brain homogenate from 6 hr post induction to 48 hr of exposure of Asiaticoside at different dosages. C) Mean±SD graph showing Xanthine concentration in the brain homogenate from 6 hr post induction to 48 hr of exposure of Asiaticoside at different dosages. D) Mean±SD graph showing scavenging activity of SOD from 6 hr post induction to 24 hr of exposure of Asiaticoside at different dosages.
DISCUSSION
Commonly used drugs like mannitol, levetiracetam, benzodiazepine, coagulation factors and diuretics may precipitate many adverse effects like rebound edema, hypersensitivity, electrolyte imbalance and CNS depression.18,20,39–44 Some commonly used drugs and their adverse effects are mentioned in Table 2. It is clear that a multidrug regimen is needed to alleviate the symptoms of ICH.
Class of drug-uses | Uses | Side effects | Examples |
---|---|---|---|
Osmotic diuretics | Edema | Rebound edema. | Mannitol. |
Anti-convulsant | Convulsions | CNS depression, psychosis. | Levetiracetam, diazepam. |
Diuretics | Edema | Electrolyte imbalance. | Torsemide Frusemide. |
Calcium channel blockers | Vasodilation | Palpitation, dizziness. | Nimodipine, Amlodipine. |
β-blockers | Reduce hypertension. | Bradycardia, hypotensionn. | Atenolol, Metoprolol. |
Drugs used in ICH therapy and their possible adverse effects.
The ICH pathology was successfully developed in adult Zebrafish models using Atorvastatin concentration of 1000 μM. Atorvastatin is a hypolipidemic drug, which inhibits the enzyme HMG-CoA reductase, thereby reducing the synthesis of cholesterol. Crilly (2019) repurposed the drug to create a model for ICH in Zebrafish larvae. The same procedure was adopted in adult fish in the current study for the first time. This established model of ICH was further used to study the neuroprotective effects of Asiaticoside.
Previously, mouse models of ICH were established using collagenase injection directly in the brain which degraded the extracellular matrix proteins and disrupted the vessel wall integrity, finally leading to the ICH.45 This induction is described to be traumatic as it causes injury at the region of injection.
Ischemic stroke models are developed by occluding the cerebral artery of the mouse leading to cessation of blood to certain parts of the brain. Direct stabbing of the brain of adult Zebrafish also provides a model of a traumatic brain haemorrhage. In another study, the hemorrhage pathology was considered as a “blood in brain” consequence hence autologous blood was injected inside the brain to investigate the pathological consequences.46 Amidst the existing ethical constraints in using higher animal models, Zebrafish provides a valuable and cheap tool to study neuroinflammation.47 The limitation mentioned before can be overcome in our current study by chemically inducing the ICH which leaves the brain pathology intact.
Larval models of ICH possess limitations like underdeveloped neural systems, BBB and cranium which makes the investigation of cognitive functions and infiltration of inflammatory cells challenging.48 Larval studies required whole organism staining to see the apoptotic regions. Current study addresses all these limitations by using an adult Zebrafish model in which behavioral studies and tissue sections showing infiltration of microglial cells are seen. Multisystem involvement and a well-developed nervous system in the current model are translatable to humans.
Natural phytocompounds can provide a multifaceted therapy with very minimal or no adverse effects. Asiaticoside from Centella asiatica showed a neuroprotective role in adult Zebrafish intracerebral haemorrhage model.49 Reduction in inflammation, chelation of the accumulated iron, reduction in brain cell death rate, hampered ROS production, modulation of proinflammatory cytokine levels were observed in the treated groups.
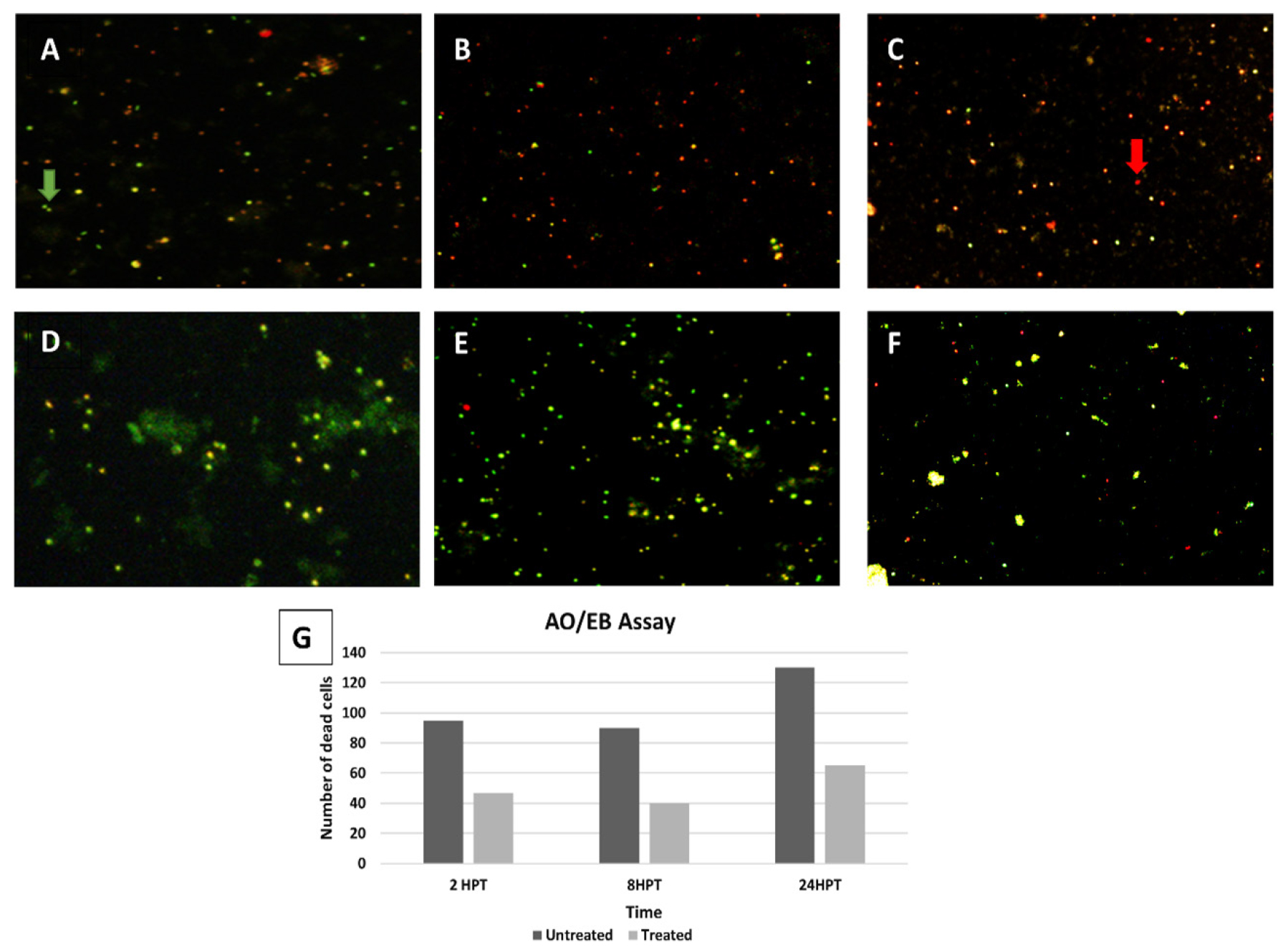
Figure 4:
Neuroprotective activity of the Asiaticoside assessed through AO/EB cell viability assay. Dead cells (red) and viable cells (green) are shown in treated (D,E,F) and untreated (A,B,C) samples in the time frame of 2,8 and 24 hr post induction. (G) Graph showing number of dead cells (cells/unit area) between treated and untreated samples in the time frame of 2, 8 and 24 hr post induction (analysed through ImageJ software).
The unruly infiltration of inflammatory cells and activation of microglial cells cause hemolysis, apoptosis and increased ROS generation in the brain.16,50–52 The microglial cells in the H&E-stained sections (Figure 2C) and CD68 immunohistochemistry observation were low in the treated group. Asiaticoside treatment reduced the proinflammatory cytokine release, proving its anti-inflammatory nature.
Microglial activation triggers the complement system and induces apoptosis in cells; they can induce erythrocyte destruction or hemolysis in the extravasated blood and precipitate the complication of iron accumulation, which may induce iron-mediated apoptosis.12 Perl’s staining of the brain sections showed a reduction in iron accumulation of 1.1-fold following treatment (Figure 1C).
Asiaticoside proved to be anti-apoptotic, preventing expansion of neurodegeneration during secondary inflammation after ICH.53 Brain cell suspension is reported for the first time in adult Zebrafish which provided >95% viable cells. The AO/EB double staining of the untreated group cell suspension showed a significant increase in the number of dead cells indicating the induction of ICH and apoptosis. After treatment with 600 μg/ mL of Asiaticoside, the number of dead cells decreased by 2-fold (Figure 4G). The number of dead cells increases with time in untreated samples due to increased accumulation of iron and initiation of ferroptosis. Previously viability testing has been performed in higher animal models and neural cell line in which there is a 2-fold decrease of apoptosis in the treated group when compared to an untreated group.54,55 The current study results are comparable with these data.
Oxidative stress contributes to myelin degradation and neuronal injury in ICH.56,57 There was a maximum of up to 12-fold increase in scavenging ratio in treatment groups with the least being 9-fold. Xanthine is a compound which when broken down by xanthine oxidase produces uric acid and ROS which in ICH can lead to disruption of vessels and cause further hemorrhage increasing hematoma volume.58 Estimation of xanthine in the samples can predict the level of antioxidant potency of Asiaticoside. The results indicate a 3-fold decrease of xanthine in the 600 μg/mL treated group within 24 hr. which is higher compared to 800 μg/ mL and 1000 μg/mL which results in a 1.6- and 1.8-fold decrease respectively (Figure 3C). Lipid peroxidation is another major indicator of oxidative damage to the cells.11 Malondialdehyde (MDA) was found to reduce by 6-fold in 600 μg/mL treatment while with 800 μg/mL and 1000 μg/mL, the decrease was 2- and 3-fold respectively (Figure 3B). The reactive oxygen species release and rate of apoptosis in ICH fall into a vicious cycle, contributing to each other. In previous studies, Asiaticoside was proven to exhibit antioxidant properties both in vitro and in vivo using brine shrimp model and neural cell line.55,59
Nitric oxide is another important molecule for cell signaling and reduction of oxidative stress thereby being neuroprotective.60 Exponential increases in nitric oxide levels serve to mediate the inflammatory process. The nitrite level in the treated groups was found to increase 3-, 1.4- and 2.2-fold in 600, 800 and 1000 μg/ mL respectively (Figure 3A).
The aforementioned observations were between 6-30 hr after induction of ICH; the inflammation and cell death seem to be resolving after that, owing to the complexity level and regenerative capacity of the animal. But this short-time pathology of ICH in Zebrafish can be compared to the long-term illness in higher animal models and humans. Though the proposed model proves promising in ICH, there are some limitations like having fragile and non-calcified bones which cannot withstand the increase of intracranial pressure, the water environment being different from that of humans, the immediate mortality that is more common in humans with ICH not seen in the fish. To overcome these limitations more relevant higher animal models could be used for pre-clinical testing.
CONCLUSION
The adult Zebrafish intracerebral haemorrhage model was developed and validated to present the multifaceted mammalian pathophysiology of the condition. Asiaticoside is shown to modulate neuroinflammation through a spectrum of biological properties thus being neuroprotective, opening avenues for further pre-clinical testing.
Cite this article:
Srinivasan MK, Vaidyanathan L, Malinika J, Chellapandi S. Asiaticoside Alleviates Neuroinflammatory Complications of Intracerebral Hemorrhage in Adult Zebrafish. Int. J. Pharm. Investigation. 2024;14(4):1138-46.
ACKNOWLEDGEMENT
The authors acknowledge Sri Ramachandra Institute of Higher Education and Research for the infrastructure support. The Department of Pathology is acknowledged for its support in histochemical analysis.
ABBREVIATIONS
ICH | Intracerebral Hemorrhage |
---|---|
IL-1β | Interleukin 1β |
RT-PCR | Reverse transcriptase-polymerase chain reaction |
CAA | Cerebral amyloid angiopathy |
HMG CoA | Hydroxy methyl glutaryl Coenzyme A |
ROCK | Rho-associated coiled-coil containing protein kinase |
AO/EB | Acridine orange/Ethidium bromide |
TBA | Thiobarbituric acid |
TCA | Trichloroacetic acid |
CD68 | Cluster of differentiation |
References
- Flaherty ML. Long-term mortality after intracerebral hemorrhage From the Departments of Neurology. Neurology. 2006;66(8):1182-6. [Google Scholar]
- Sutherland GR, Auer RN. Primary. J Clin Neurosci. 2006;13(5):511-7. [PubMed] | [CrossRef] | [Google Scholar]
- Wu G, Xi2 G, Huang1 F. Spontaneous intracerebral hemorrhage in humans: hematoma enlargement, clot lysis, and brain edema. 2006 [PubMed] | [CrossRef] | [Google Scholar]
- An SJ, Kim TJ, Yoon BW. Epidemiology, risk factors, and clinical features of intracerebral hemorrhage: an update. J Stroke. 2017;19(1):3-10. [PubMed] | [CrossRef] | [Google Scholar]
- Rincon F, Mayer SA. Novel therapies for intracerebral hemorrhage. Curr Opin Crit Care. 2004;10(2):94-100. [PubMed] | [CrossRef] | [Google Scholar]
- Fu X, Zhou G, Zhuang J, Xu C, Zhou H, Peng Y, et al. White matter injury after intracerebral hemorrhage. Front Neurol. 2021;12:562090 [PubMed] | [CrossRef] | [Google Scholar]
- Mehta A, Zusman BE, Choxi R, Shutter LA, Yassin A, Antony A, et al. Seizures after intracerebral hemorrhage: incidence, risk factors, and impact on mortality and morbidity. World Neurosurg. 2018;112 [PubMed] | [CrossRef] | [Google Scholar]
- Zheng H, Chen C, Zhang J, Hu Z. Mechanism and therapy of brain edema after intracerebral hemorrhage. Cerebrovasc Dis. 2016;42(3-4):155-69. [PubMed] | [CrossRef] | [Google Scholar]
- John S, Garg R. Intracerebral hemorrhage. Handbook of stroke and neurocritical care. Published online. 2013:209-22. [PubMed] | [CrossRef] | [Google Scholar]
- Kashgsbngjcahtebkvccdjw Andrei. Prevalence, characteristics and outcomes of undetermined intracerebral hemorrhage: A systematic review and meta-analysis. 2009;10(1) [PubMed] | [CrossRef] | [Google Scholar]
- Wan J, Ren H, Wang J. Iron toxicity, lipid peroxidation and ferroptosis after intracerebral haemorrhage. Stroke Vasc Neurol. 2019;4(2):93-5. [PubMed] | [CrossRef] | [Google Scholar]
- Derry PJ, Tran A, Vo T. The chemical basis of intracerebral hemorrhage and cell toxicity with contributions from eryptosis and ferroptosis. 2020;14:1-18. [CrossRef] | [Google Scholar]
- Liu J, Liu L, Wang X, Jiang R, Bai Q. Microglia: A double-edged sword in intracerebral hemorrhage from basic mechanisms to clinical research. 2021;12:1-11. [CrossRef] | [Google Scholar]
- Moritz C, Berardi F, Abate C, Peri F. Live imaging reveals a new role for the sigma-1 (σ1) receptor in allowing microglia to leave brain injuries. Neurosci Lett. 2015;591:13-8. [PubMed] | [CrossRef] | [Google Scholar]
- Sabogal-Guáqueta AM, Marmolejo-Garza A, de Pádua VP, Eggen B, Boddeke E, Dolga AM, et al. Microglia alterations in neurodegenerative diseases and their modeling with human induced pluripotent stem cell and other platforms. Prog Neurobiol. 2020;190:101805 [PubMed] | [CrossRef] | [Google Scholar]
- Li LZ, Huang YY, Yang ZH, Zhang SJ, Han ZP, Luo YM, et al. Potential microglia-based interventions for stroke. CNS Neurosci Ther. 2020;26(3):288-96. [PubMed] | [CrossRef] | [Google Scholar]
- Flores JJ, Klebe D, Tang J, Zhang JH. A comprehensive review of therapeutic targets that induce microglia/macrophage-mediated hematoma resolution after germinal matrix hemorrhage. J Neurosci Res. 2020;98(1):121-8. [PubMed] | [CrossRef] | [Google Scholar]
- Misra UK, Kalita J, Ranjan P, Mandal SK. Mannitol in intracerebral hemorrhage: A randomized controlled study. J Neurol Sci. 2005;234(1-2):41-5. [PubMed] | [CrossRef] | [Google Scholar]
- Naidech AM, Batjer HH, Bernstein RA. Prior antiplatelet therapy and outcome following intracerebral hemorrhage: A systematic review. Neurology. 2011;76(18):1607 [PubMed] | [CrossRef] | [Google Scholar]
- Mackey J, Ashley D, Blatsioris EM. Prophylactic anticonvulsants in intracerebral hemorrhage Jason. Lancet Gastroenterol Hepatol. 2020;5(6):537-47. [CrossRef] | [Google Scholar]
- Sun T, Liu B, Li P. Nerve protective effect of asiaticoside against ischemia-hypoxia in cultured rat cortex neurons. Med Sci Monit. 2015;21:3036-41. [PubMed] | [CrossRef] | [Google Scholar]
- Tabassum R, Vaibhav K, Shrivastava P, Khan A, Ejaz Ahmed M, Javed H, et al. Array. Neurol Sci. 2013;34(6):925-33. [PubMed] | [CrossRef] | [Google Scholar]
- Zhang C, Chen S, Zhang Z, Xu H, Zhang W, Xu D, et al. Asiaticoside Alleviates Cerebral ischemia-reperfusion Injury via NOD2/mitogen-activated protein kinase (MAPK)/nuclear factor kappa B (NF-kB) Signaling Pathway. Med Sci Monit. 2020;26 [PubMed] | [CrossRef] | [Google Scholar]
- Crilly SC. Using zebrafish for preclinical brain haemorrhage research. Published online. 2019 Available fromhttps://www.research.manchester.ac.uk/portal/files/184636141/FULL_TEXT.PDF
[PubMed] | [CrossRef] | [Google Scholar] - Crilly S, Cooper J, Bradford L, Prise IE, Krishnan S, Kasher PR, et al. RNA-seq dataset from isolated leukocytes following spontaneous intracerebral hemorrhage in zebrafish larvae. Front Cell Neurosci. 2021;15:1-7. [CrossRef] | [Google Scholar]
- Crilly S, Njegic A, Parry-Jones AR, Allan SM, Kasher PR. Using zebrafish larvae to study the pathological consequences of hemorrhagic stroke. J Vis Exp. 2019(148):1-9. [PubMed] | [CrossRef] | [Google Scholar]
- yan ZZ, Huang B, Li S. Sodium tanshinone IIA sulfonate promotes endothelial integrity via regulating VE-cadherin dynamics and RhoA/ROCK-mediated cellular contractility and prevents atorvastatin-induced I ..sodium tanshinone IIA sulfonate promotes endothelial integrity via r. Toxicol Appl Pharmacol. 2020;350:32-42. [CrossRef] | [Google Scholar]
- NIH. Guidelines for use of zebrafish in the NIH Intramural Research Program scientific. Published online. 2020:1-4. [CrossRef] | [Google Scholar]
- Jarvis RB, Knowles JF. DNA damage in zebrafish larvae induced by exposure to low-dose rate γ-radiation: detection by the alkaline comet assay. Mutat Res. 2003;541(1-2):63-9. [PubMed] | [CrossRef] | [Google Scholar]
- Perl DP, Good PF. Comparative techniques for determining cellular iron distribution in brain tissues. Ann Neurol. 1992;32(Suppl(1 S)) [PubMed] | [CrossRef] | [Google Scholar]
- Nasrallah GK, Younes NN, Baji MH, Shraim AM, Mustafa I. Zebrafish larvae as a model to demonstrate secondary iron overload. Eur J Haematol. 2018;100(6):536-43. [PubMed] | [CrossRef] | [Google Scholar]
- Cardiff RD, Miller CH, Munn RJ. Manual hematoxylin and eosin staining of mouse tissue sections. Cold Spring Harb Protoc. 2014;2014(6):655-8. [PubMed] | [CrossRef] | [Google Scholar]
- Micklem K, Rigney E, Cordell J, Simmons D, Stross P, Turley H, et al. A human macrophage-associated antigen (CD68) detected by six different monoclonal antibodies. Br J Haematol. 1989;73(1):6-11. [PubMed] | [CrossRef] | [Google Scholar]
- Ohkawa H, Ohishi N, Yagi K. Assay for lipid peroxides in animal tissues by thiobarbituric acid reaction. Anal Biochem. 1979;95(2):351-8. [PubMed] | [CrossRef] | [Google Scholar]
- Xu C, Liu S, Liu Z, Song F, Liu S. Superoxide generated by pyrogallol reduces highly water-soluble tetrazolium salt to produce a soluble formazan: A simple assay for measuring superoxide anion radical scavenging activities of biological and abiological samples. Anal Chim Acta. 2013;793:53-60. [PubMed] | [CrossRef] | [Google Scholar]
- LITWACK G, BOTHWELL JW, WILLIAMS JN, ELVEHJEM CA. A colorimetric assay for xanthine oxidase in rat liver homogenates. J Biol Chem. 1953;200(1):303-10. [PubMed] | [CrossRef] | [Google Scholar]
- Kasibhatla S, Amarante-Mendes GP, Finucane D, Brunner T, Bossy-Wetzel E, Green DR, et al. Acridine orange/ethidium bromide (AO/EB) staining to detect apoptosis. CSH Protoc. 2006;2006(3):pdb [PubMed] | [CrossRef] | [Google Scholar]
- Visavadiya NP, Patel SP, VanRooyen JL, Sullivan PG, Rabchevsky AG. Cellular and subcellular oxidative stress parameters following severe spinal cord injury. Redox Biol. 2016;8:59-67. [PubMed] | [CrossRef] | [Google Scholar]
- Horn J, De Haan RJ, Vermeulen M, Limburg M. Very Early Nimodipine Use in Stroke (VENUS): A randomized, double-blind, placebo-controlled trial. Stroke. 2001;32(2):461-5. [PubMed] | [CrossRef] | [Google Scholar]
- Peacock IV WF, Hilleman DE, Levy PD, Rhoney DH, Varon J. A systematic review of nicardipine vs labetalol for the management of hypertensive crises. Am J Emerg Med. 2012;30(6):981-93. [PubMed] | [CrossRef] | [Google Scholar]
- Adeoye O, Broderick JP. Advances in the management of intracerebral hemorrhage. Nat Rev Neurol. 2010;6(11):593-601. [PubMed] | [CrossRef] | [Google Scholar]
- Secades JJ, Álvarez-Sabín J, Rubio F, Lozano R, Dávalos A, Castillo J, et al. Citicoline in intracerebral haemorrhage: A double-blind, randomized, placebo-controlled, multi-centre pilot study. Cerebrovasc Dis. 2006;21(5-6):380-5. [PubMed] | [CrossRef] | [Google Scholar]
- Ortega-Gutierrez S, Thomas J, Reccius A, Agarwal S, Lantigua H, Li M, et al. Effectiveness and safety of nicardipine and labetalol infusion for blood pressure management in patients with intracerebral and subarachnoid hemorrhage. Neurocrit Care. 2013;18(1):13-9. [PubMed] | [CrossRef] | [Google Scholar]
- Brouwers HB, Goldstein JN. Therapeutic strategies in acute intracerebral hemorrhage. Neurotherapeutics. 2012;9(1):87-98. [PubMed] | [CrossRef] | [Google Scholar]
- Zhu W, Gao Y, Chang CF, Wan JR, Zhu SS, Wang J, et al. Mouse models of intracerebral hemorrhage in ventricle, cortex, and hippocampus by injections of autologous blood or collagenase. PLOS ONE. 2014;9(5) [PubMed] | [CrossRef] | [Google Scholar]
- Cheng J, Tang JC, Pan MX, Chen SF, Zhao D, Zhang Y, et al. L-lysine confers neuroprotection by suppressing inflammatory response via microRNA-575/PTEN signaling after mouse intracerebral hemorrhage injury. Exp Neurol. 2020;327:113214 [PubMed] | [CrossRef] | [Google Scholar]
- Srinivasan MK, Vaidyanathan L. Exploring biomarkers associated with secondary inflammation following intracerebral hemorrhage using adult Zebrafish Model. 2022:2022 Available fromhttp://thesciencein.org/cbl
- Schier AF. Genetics of neural development in zebrafish. Curr Opin Neurobiol. 1997;7(1):119-26. [PubMed] | [CrossRef] | [Google Scholar]
- Bonte F, Dumas M, Chaudagne C, Meybeck A. Influence of Asiatic acid, madecassic acid, and asiaticoside on human collagen I synthesis. Planta Med. 1994;60(2):133-5. [PubMed] | [CrossRef] | [Google Scholar]
- Tanaka M, Sackett S, Zhang Y. Endocannabinoid modulation of microglial phenotypes in neuropathology. Front Neurol. 2020;11:87 [PubMed] | [CrossRef] | [Google Scholar]
- Li Y, Tan MS, Jiang T, Tan L. Microglia in Alzheimer’s disease. BioMed Res Int. 2014;2014:437483 [PubMed] | [CrossRef] | [Google Scholar]
- Perry VH, Teeling J. Microglia and macrophages of the central nervous system: the contribution of microglia priming and systemic inflammation to chronic neurodegeneration. Semin Immunopathol. 2013;35(5):601-12. [PubMed] | [CrossRef] | [Google Scholar]
- Mazzolini J, Chia K, Sieger D. Isolation and RNA extraction of neurons, macrophages and microglia from Larval zebrafish brains. J Vis Exp. 2018(134):1-8. [PubMed] | [CrossRef] | [Google Scholar]
- He ZH, Hu Y, Zhang Y, Xie J, Niu Z, Yang G, et al. Asiaticoside exerts neuroprotection through targeting NLRP3 inflammasome activation. Phytomedicine. 2024;127:155494 [PubMed] | [CrossRef] | [Google Scholar]
- Qi FY, Yang L, Tian Z, Zhao MG, Liu SB, An JZ, et al. Neuroprotective effects of asiaticoside. Neural Regen Res. 2014;9(13):1275-82. [PubMed] | [CrossRef] | [Google Scholar]
- Duan X, Wen Z, HS GC. Intracerebral hemorrhage, oxidative stress, and antioxidant therapy. Oxid Med Cell Longev. 2016;17:1-17. [PubMed] | [CrossRef] | [Google Scholar]
- Yao Z, Bai Q, Wang G. Mechanisms of Oxidative Stress and Therapeutic Targets following Intracerebral Hemorrhage. Oxid Med Cell Longev. 2021;2021:8815441 [PubMed] | [CrossRef] | [Google Scholar]
- Higgins P, Dawson J, Walters M. The potential for xanthine oxidase inhibition in the prevention and treatment of cardiovascular and cerebrovascular disease. Cardiovasc Psychiatry Neurol. 2009;2009:282059 [PubMed] | [CrossRef] | [Google Scholar]
- Rashid MH, Akter MM, Uddin J, Islam S, Rahman M, Jahan K, et al. Antioxidant, cytotoxic, antibacterial and thrombolytic activities of L.: possible role of phenolics and flavonoids. Clin Phytosci. 2023;9(1) [CrossRef] | [Google Scholar]
- Prüss H, Prass K, Ghaeni L, Milosevic M, Muselmann C, Freyer D, et al. Inducible nitric oxide synthase does not mediate brain damage after transient focal cerebral ischemia in mice. J Cereb Blood Flow Metab. 2008;28(3):526-39. [PubMed] | [CrossRef] | [Google Scholar]