ABSTRACT
Background
Studies have indicated that atazanavir is prone to degradation in environments with high levels of acidity and oxidative stress. However, the drug demonstrated stability when exposed to basic, neutral, photolytic and thermal stresses. There were two Degradation Products (DPs) from the stress studies.
Materials and Methods
An assessment of Atazanavir sulphate (ATV) stability under various stress conditions were carried out using the International Conference on Harmonisation (ICH) Q1A (R2) recommendations as a foundation for work. Pre-treated silica gel 60F254 plates were combined with ethyl acetate: methanol (9:1 (v/v)) mobile phase to create a chromatographic profile.
Results
An approach that combines high-resolution Mass Spectrometry (MS/TOF) with Infrared (IR) spectroscopy was used on a variety of stress-exposed substances to clarify functional moieties and extract mass spectral data. First, a thorough fragmentation pathway for the parent medication was mapped using the high-resolution mass spectral data, which was then applied to its degradation products. Each fragment was given a structural suggestion based on the most likely molecular formulas.
Conclusion
Based on this investigation, two new degradation products were found: methyl N-[(1S) -1-{[(2S,3S) -3-hydroxy -4-[(2S)-2-[(methoxycarbonyl)amino] -3,3-dimethylbutanehydrazido] -1 -phenylbutan-2-yl] carbamoyl} -2,2-dimethylpropyl] carbamate and (2S,3S)-3-amino -4-phenyl-(1-(4-(pyridine-2-yl) benzyl)hydrazinyl)butan-2-ol.
INTRODUCTION
When it comes to being the cutting-edge treatment for HIV-1 infection, atazanavir sulphate (Figure 1) has a prominent place.1 This molecule is classified as a novel protease inhibitor (PI) with a structurally complex chemical profile; it is technically classified as (3S,8S,9S,12S)-3,12-Bis(1,1-dimethylethyl)-8 hydroxy-4,11-dioxo-9-(phenylmethyl)-6-[4-(2-pyridinyl) phenyl] methyl]-2,5,6,10,13pentaazatetradecanedioic acid dimethyl ester, sulphate (1:1). Its molecular weight, expressed as C38H52N6O7oH2SO4, is equivalent to 802.9 (sulfuric acid salt).2
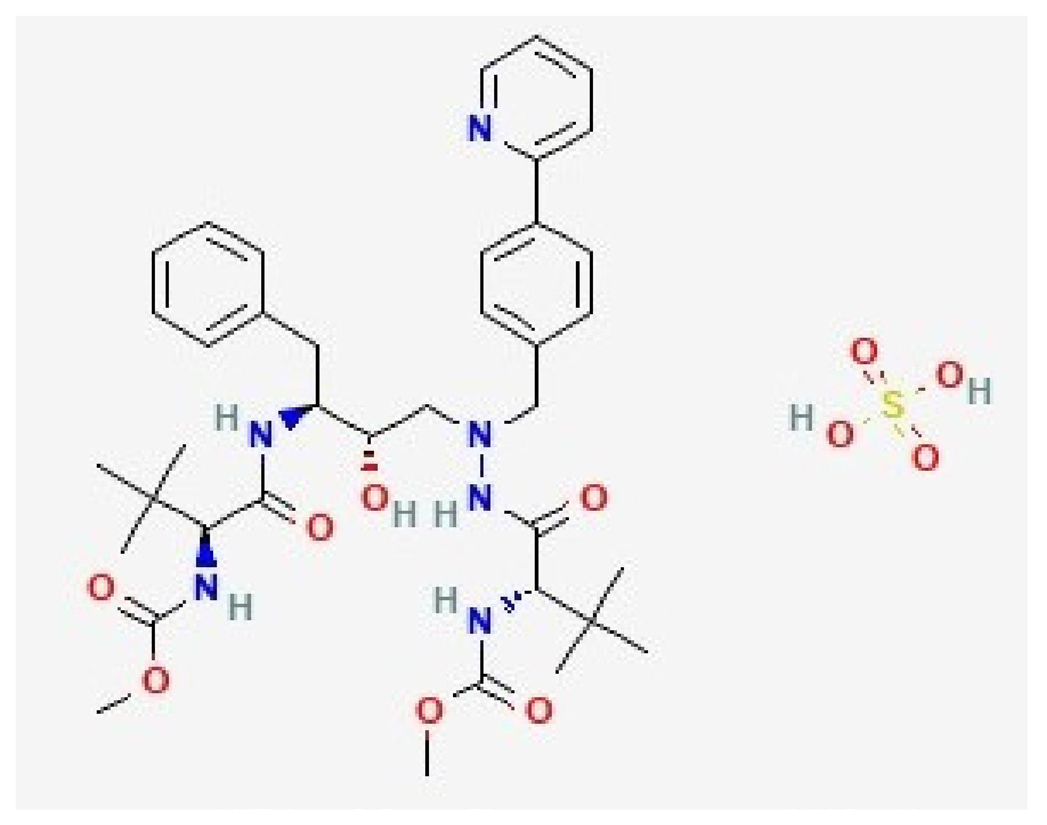
Figure 1:
Chemical Structure of Atazanavir Sulphate.
The scientific literature contains well-established methods like UV spectrometric3–5 and chromatographic methods6–11 for evaluating atazanavir sulphate in different formulations as well as when it’s isolated. For these types of examinations, a new and maybe better approach is provided by the recently established High-Performance Thin-Layer Chromatography (HPTLC) method. The limitations of previous approaches are surpassed by this methodology, which shows a substantially greater degree of efficacy in the analysis of atazanavir in both bulk and combination dosage forms.2 Furthermore, the effectiveness of well-known methods like UV-detected High-Performance Liquid Chromatography (HPLC) in the atazanavir sulphate analysis of human plasma samples has been recognised.12-15 A trustworthy SPE-LC-ESI-MS/MS method has also been described for use in human plasma analysis, providing even more sensitive detection capabilities.16
As per the ICH Q1A (R2)17 recommendations, there is a significant deficiency in the present scientific comprehension of a methodical approach to characterise the degradation products resulting from atazanavir sulphate under-regulated stress environments. The difference in comprehension provided a compelling opportunity for more scientific research. The current study was thoughtfully planned to develop an effective HPTLC method that can effectively separate atazanavir sulphate from its degradant products in bulk and pharmaceutical formulations.2
Our goal in conducting this study was to provide some illumination on important details about the stability profile of atazanavir sulphate and the related degradation mechanism. These results have the potential to make a substantial contribution to the overall collection of research on this area of science.
MATERIALS AND METHODS
Chemicals and Reagents
Pharmaceutical-grade Atazanavir sulphate was obtained as a gift sample by Cipla Ltd., Vikroli (Mumbai, India). Analytical-grade ethyl acetate and methanol were procured from S. D. Fine Chemicals (Mumbai). The commercially available Atazor capsules were bought from a nearby pharmacy; one capsule is equal to 300 mg of atazanavir sulphate.
Instrumentation
Aluminium plates pre-coated with Merck’s 60F254 (10 cmx10 cm, 6 mm thick) silica gel was used as the study’s stationary phase (Darmstadt, Germany). These plates were activated for 5 min at 110°C and pre-washed with methanol before being subjected to chromatography. Using a Camag 100-microliter sample syringe (Hamilton, Bonaduz, Switzerland), samples were administered in bands of 6 mL wide with a continuous flow of nitrogen. This procedure has been simplified by the CamagLinomat V (Switzerland) sample applicator (Camag, Muttenz, Switzerland). Within a twin-trough glass chamber (10×10 cm, Camag, Muttenz, Switzerland), linear ascending development was carried out. Utilising a Camag TLC scanner III in reflectance-absorbance mode and win-CATS software (V 3.15, Camag), densitometric scanning was carried out. An FT/IR-4100 type AS N: B131361016 instrument was used to get Fourier-Transform Infrared (FT/ IR) spectra. Utilising a MicroTOF-Q mass spectrometer and a Detector DLA-TGS detector, mass spectrum analysis was carried out.
Sample Preparation for HPTLC Analysis
Methanol was added to a 100 mL volumetric flask along with 100 mg of precisely weighed atazanavir sulphate. The following procedure was to dilute this solution with methanol to the requisite concentration for making a standard stock solution. It requires a further dilution to achieve 100 μg/mL. The process required filling a 50 mL volumetric flask containing 5 mL of the stock solution. Following that, methanol was employed to dilute the solution until the desired concentration level was obtained. A 0.2 μm membrane filter was subsequently employed to filter the resulting solution.
Sample solution for the assay of atazanavir sulphate capsules
Sample preparation for HPTLC analysis of atazanavir sulphate capsules must be done carefully. Twenty capsules are weighed first and then they are ground into a fine powder. A 100 mL flask is filled with a carefully calculated quantity of this powder or around 100 mg of atazanavir sulphate. To extract the drug, 30 mL of methanol were added and the liquid was sonicated for 15 min. After introducing methanol to adjust the solution to volume, any capsule fillers are filtered out. To prepare for HPTLC analysis, a 1 mL aliquot of the filtrate is eventually diluted with methanol in a 10 mL flask to get a final concentration of 100 mL.
Development of the Stability-Indicating Assay (SIAM) Method and Optimisation
Following an overview of the literature, methods for calculating the dose forms’ Atazanavir sulphate content were determined to be established and verified. In these procedures, a C18 column Phenomenix (250 mmx4.6 mm, 5 μm) was used and the mobile phase consisted of 900 mL of methanol and 100 mL of HPLC- grade water.11 This method of analysis led to the development of a liquid chromatography process that is quicker, less expensive and more efficient. This technique of chromatographic separation employed pre-coated silica gel 60F254 HPTLC plates with ethyl acetate: methanol (9:1 v/v) mobile phase. To achieve good separation of the medication and its Degradation Products (DPs), several tests were conducted at a detection wavelength of 282 nm (corresponding to the maximum absorbance of atazanavir sulphate) using pre-coated silica gel 60F254 HPTLC plates. Before doing tests on combinations of stressed samples, HPTLC analysis was first carried out on individual samples under each stress condition.
Method Validation
After the HPTLC method was developed, a thorough validation procedure was carried out in full compliance with the International Council for Harmonisation’s Q2 (R1)18 requirements (ICH). This validation evaluated several significant performance standards.
Linearity
A prepared stock solution of Atazanavir sulphate (ATV) in methanol (10 mg/100 mL) was used to evaluate the test’s linearity. Five different concentration levels of test solutions were prepared using this stock solution. For each concentration, three separate experiments were carried out using 10 μL aliquots for HPTLC analysis. The peak area data for each concentration were evaluated using least-squares linear regression analysis to generate a calibration curve. The correlation coefficient of the calibration curve was determined to evaluate the strength of the linear relationship between concentration and peak area.
Accuracy
The accuracy of the procedure was assessed using the usual addition methodology. A triplicate of known doses of ATV (100 μg) was applied in triplicate to pre-analysed stressed samples at three different concentration levels (80, 100 and 120 μg/mL). The percentage recovery of the extra ATV was then used to calculate the technique’s accuracy over a range of concentrations.
Precision
When analysing the procedure’s precision, both intra-day and inter-day variances were taken into account. To evaluate intra-day precision, three triplicate samples (n=3) of a 10 μg/ mL ATV solution were analysed on the same day. The next day, the same concentration solution investigation was conducted to determine the inter-day precision. For both intra-day and inter-day experiments, the Relative Standard Deviation (RSD) was calculated to assess the accuracy of the approach. The slope of the calibration curve and the y-intercept standard deviation were used to calculate the LOD and LOQ. The lowest concentration levels at which ATV may be accurately detected and measured are determined by these values, respectively.
Robustness
Variations in the mobile phase composition’s volume (±0.1 mL), amount (±1 mL), duration of chamber saturation (±20%), time from spotting to development (5, 10 and 15 min) and time from development to scanning (5, 20 and 40 min) were used to test the robustness of the examined method. The impacts on the drug’s peak area and Rf values were explored.
Stress Studies
A thorough analysis of the Atazanavir sulphate (ATV) stability profile was conducted. In order to replicate a range of real-world scenarios that can potentially cause the drug to deteriorate, we used a number of exacting stress degradation experiments. The testing process complied with the predetermined standards established by ICH Q1A (R2). The drugs were subjected to a variety of stress situations, such as hydrolysis, oxidation, dry heat and photolysis.
Selection and Optimisation of the Mobile Phase
The mobile phase’s makeup plays a critical role in HPTLC analysis by separating ATV from its breakdown products. A mobile phase of ethyl acetate, toluene and methanol (7.5:2:0.5 v/v) was used, with UV detection at 234 nm. The approach was inspired by previously described HPTLC methods for Ritonavir (RTV) and ATV.19 The maximum absorbance of ATV, or 282 nm, was chosen as the detecting wavelength when this mobile phase evolved for the current investigation. Utilising Mass Spectrometry (MS/ TOF), the degradation products produced under the different stress levels were characterised.
Hydrolytic Degradation
To determine the drug’s sensitivity to hydrolysis, it was subjected to both acidic and alkaline environments. 1 mL aliquots of 100 micrograms per mL ATV solution, 1 mL aliquots of 0.1 N Sodium Hydroxide (NaOH) and 0.1 N Hydrochloric acid (HCl) were heated up for 4 hr at 80°C. The samples were neutralized using solutions of similar strength (base for samples treated with acid and vice versa) after this exposure.
Oxidative Degradation
To test the possibility of oxidative ATV degradation, a 1 mL aliquot of a 100 μg/mL ATV solution was treated for 4 hr at room temperature with 1 mL of 3% hydrogen peroxide (H2O2).
Thermal Degradation
Enclosed in a glass ampoule, the drug was heated for 12 hr at 80°C in an oven to examine the solid-state thermal degradation of ATV. A control sample was maintained at room temperature for the purpose of comparison.
Photodegradation
In order to assess the light sensitivity of ATV, a solid drug and a 100 μg/mL solution of the drug in acetonitrile were subjected to fluorescent light and UV light (200 Whm2) for a duration of 12 hr within a photostability chamber. The control samples were shielded from light exposure by aluminium foil. All of the samples were diluted using the methanol diluent used in the HPTLC technique for further analysis after being exposed to these different stress conditions. Table 1 presents the optimum conditions for each stress treatment.
Stress Condition (50°C) for 2 hr | Percent assay of Active substance (ATV) | Rf Value of degraded product |
---|---|---|
Acidic HCl (0.1 M). | 23.10 | 0.31 |
Alkali (0.1M NaOH). | 2.00 | 0.42 |
Oxide (3% H2O2). | 19.28 | 0.22,0.48 |
Heat In oven. | — | — |
UV (240 nm) 12 hr. | — | — |
Acid (1 N HCl) 12 hr. | 77.05 | 0.49 |
Oxidation (6% H2O2) 12 hr. | 78.33 | 0.29 |
Optimized stress condition for ATV and Extensive Degradation Study (acidic and H2O2)
Preparation of Extensive Acidic and Oxidation-Induced Degradation Product
Stress degradation testing was used to evaluate the stability of Atazanavir sulphate (ATV). Accurately weighed 100 mg samples were dissolved in methanol and exposed to acidic conditions (25 mL 1 N HCL) or oxidative conditions (6% H2O2) in different flasks. After that, both flasks were heated for 12 hr at 80°C. The solutions were moved to volumetric flasks, neutralised with the appropriate agents (1 N NaOH for acid and 1 N sodium bisulfite for oxidation) and brought to volume with methanol following almost full concentration under vacuum and chilling. Under both circumstances, the suggested HPTLC approach verified total degradation (no intact ATV peaks). Table 5 and Figures 11 and 12 show the degradation route and regression equations were used to quantify the remaining ATV concentration in the undegraded samples.
Temperature (°C) | Regression equation parameters (n=6) a | ||
---|---|---|---|
Slope ±SD | Intercept±SD | Correlation r2 | |
40 | 1.643±0.022 | 1.926±0.524 | 0.9980 |
60 | 1.639± 0.015 | 1.922±0.478 | 0.9653 |
80 | 1.64l±0.0l8 | 1.924±0.475 | 0.9980 |
100 | 1.645±0.027 | 1.929±0.525 | 0.9905 |
H2O2 concentration 3%. | 1.638± 0.017 | 1.92l±0.469 | 0.9878 |
H2O2 concentration 6%. | 1.643±0.022 | 1.929±0.525 | 0.9980 |
HCl concentration 0.5 M. | 1.644±0.025 | 1.925±0.470 | 0.9985 |
HCl concentration 1 M. | 1.646±0.029 | 1.929±0.485 | 0.9988 |
Results for the effect of temperature and HCl concentration.
MS/TOF and IR Studies on Drug and Degradants
MS/TOF analysis was used to better understand the Atazanavir sulphate (ATV) fragmentation pattern. Using positive Electrospray Ionisation (ESI) mode, directly inject a 10 μg/mL solution of ATV in methanol within the 50-800 m/z range. Acquiring the molecular ion peak was ensured by the optimisation of mass parameters. The drug’s fragmentation pattern was obtained by additional optimisation, as indicated in Table 6. Four decimal places were used to record the mass of each fragment. Following separation using the proposed approach, the stressed sample was further analysed by MS/TOF with the same optimised settings (Table 6). The fragmentation patterns for each degradant were generated based on the obtained mass spectra and precise m/z values and they are displayed in Figure 14. Moreover, an IR spectra (KBr pellet) of ATV in a 100:1 ratio was generated, as shown in Figure 13 and its absorption frequencies were found to range between 400 and 4000 cm1.
m/z measured EI-MS in Acidic | Corresponding fragments |
---|---|
370.20 ÷341.02 | M+[ M-C6H 4–N- NH2] + |
370.2 ÷ 354.20 | M+[ M-C6H 5– C6H 4-N]+ |
302.7,341.02, 354.20 | Unassigned peak. |
m/z measured EI-MS in oxidation (H2O2) | |
378.2÷ 341.88 | M+[ N-C6H 5–N- C6H 4] + |
378.2÷284.42 | M+[ N-C6H 4– C6H 4] + |
378.2÷301.20 | M+[ M-O] + |
341.00, 348.2, 148.3 | Unassigned peak. |
Identification of the fragments obtained by acidic oxidation (H2O2) of ATV.
RESULTS AND DISCUSSION
Method Development and Validation
The material breaks down as a result of stress testing, and the byproducts that emerge have a distinct order (I to II) that corresponds to the peaks that are seen travelling from left to right on the HPTLC analysis (Figure 2). Two degradation products resulting from certain stressors were found by HPTLC analysis and listed beneath the chromatogram. These results demonstrated that ATV is prone to hydrolytic stress, that is, conditions that are both acidic and oxidative and disintegrate. But when it was only subjected to light or heat (photolytic stress), it stayed stable. The optimum separation of the drug and its breakdown products was checked on pre-coated silica gel 60F254 HPTLC plates using a 9:1 (v/v) mixture of ethyl acetate and methanol. This newly developed method was thoroughly validated, showing its accuracy, precision, linearity, selectivity and specificity.
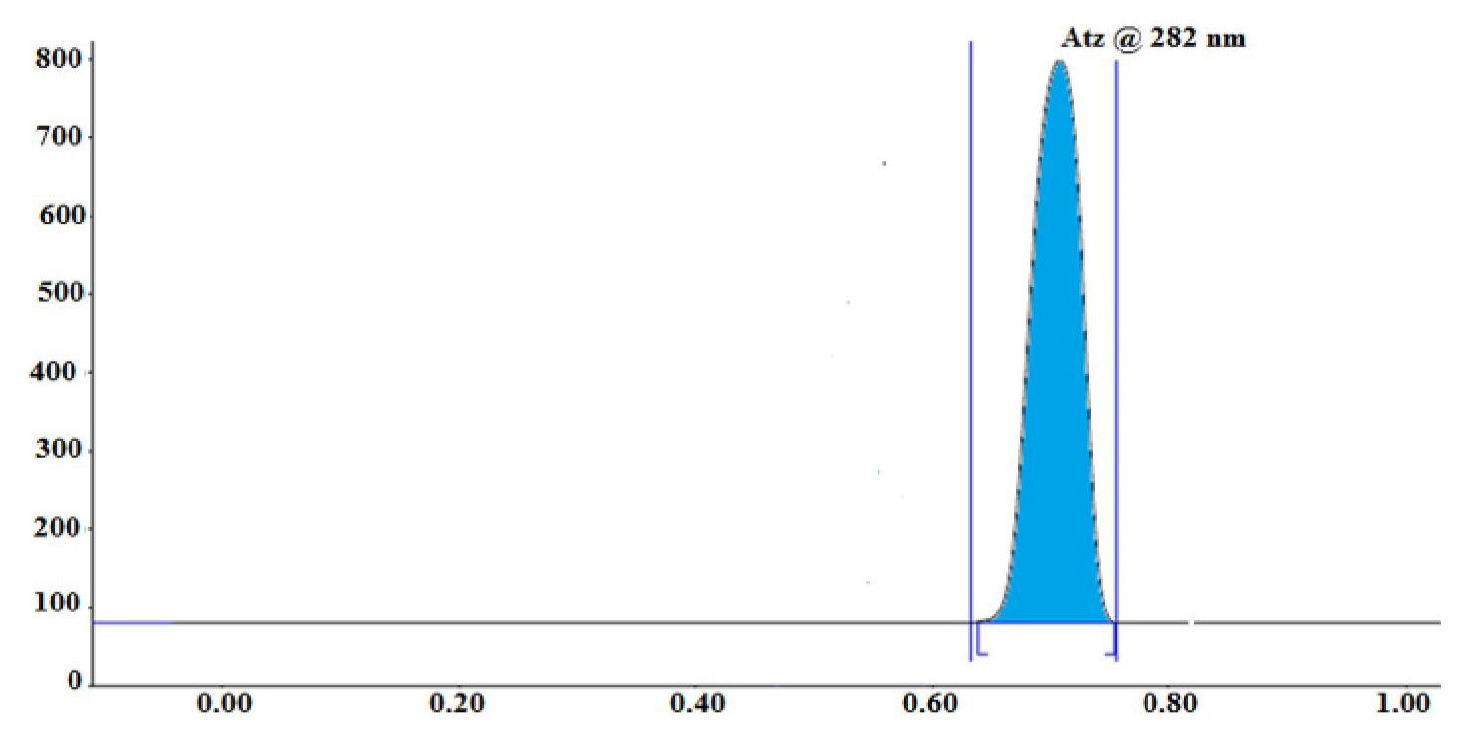
Figure 2:
HPTLC Chromatogram of Atazanavir sulphate standard 100 μg/mL at 282 nm.
The combination of degradation products using this validated method was successfully validated. Notably, the method demonstrated good selectivity, which ensured a clear separation of all peaks, including those from the original drug and its breakdown products and great peak specificity, which meant each degradation product, had a separate position on the HPTLC plate. A resolution was found to be larger than two between the peaks, suggesting strong separation. A Camag TLC scanner III operating in reflectance-absorbance mode (Table 2) verified that the medication (ATV) and its breakdown products had high peak purity (0.9994). (Figure 3) shows that the drug’s reaction was strictly linear in the concentration range of 2-12 μg/mL. (Figure 4) displays a two-dimensional linearity plot. The method’s linearity was further supported by the determination of the slope and correlation coefficient (r2), which were found to be 128724 and 0.9994, respectively (Table 2).
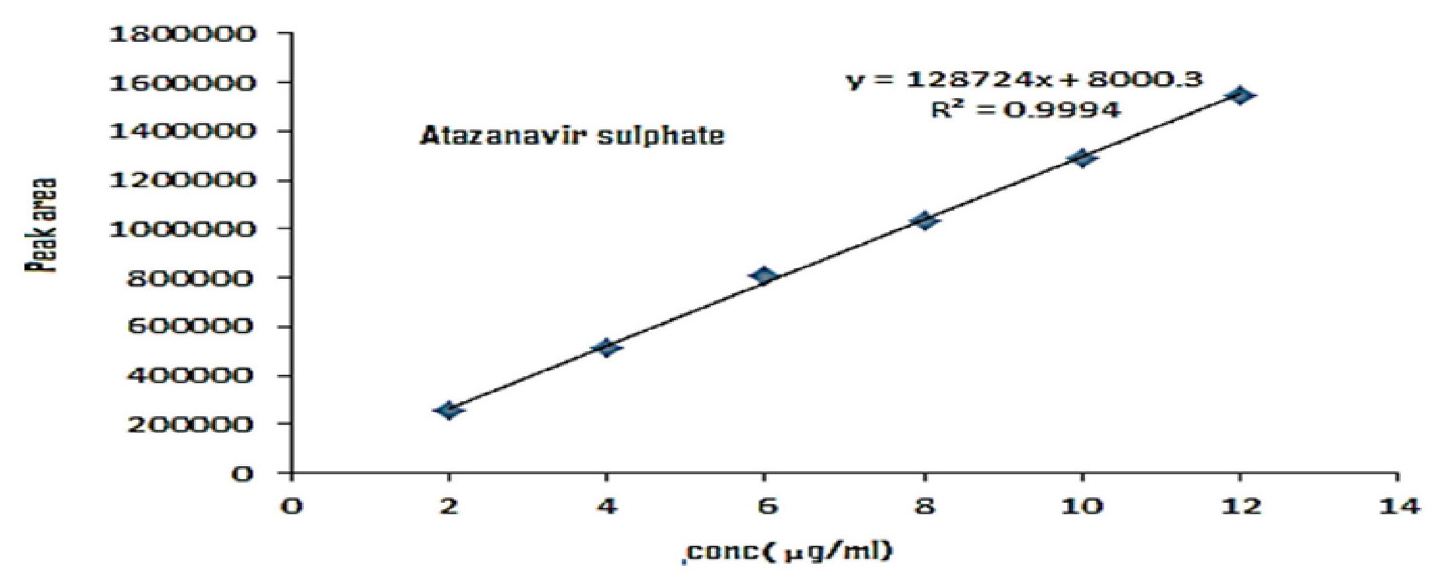
Figure 3:
Linearity plot of Atazanavir Sulphate.
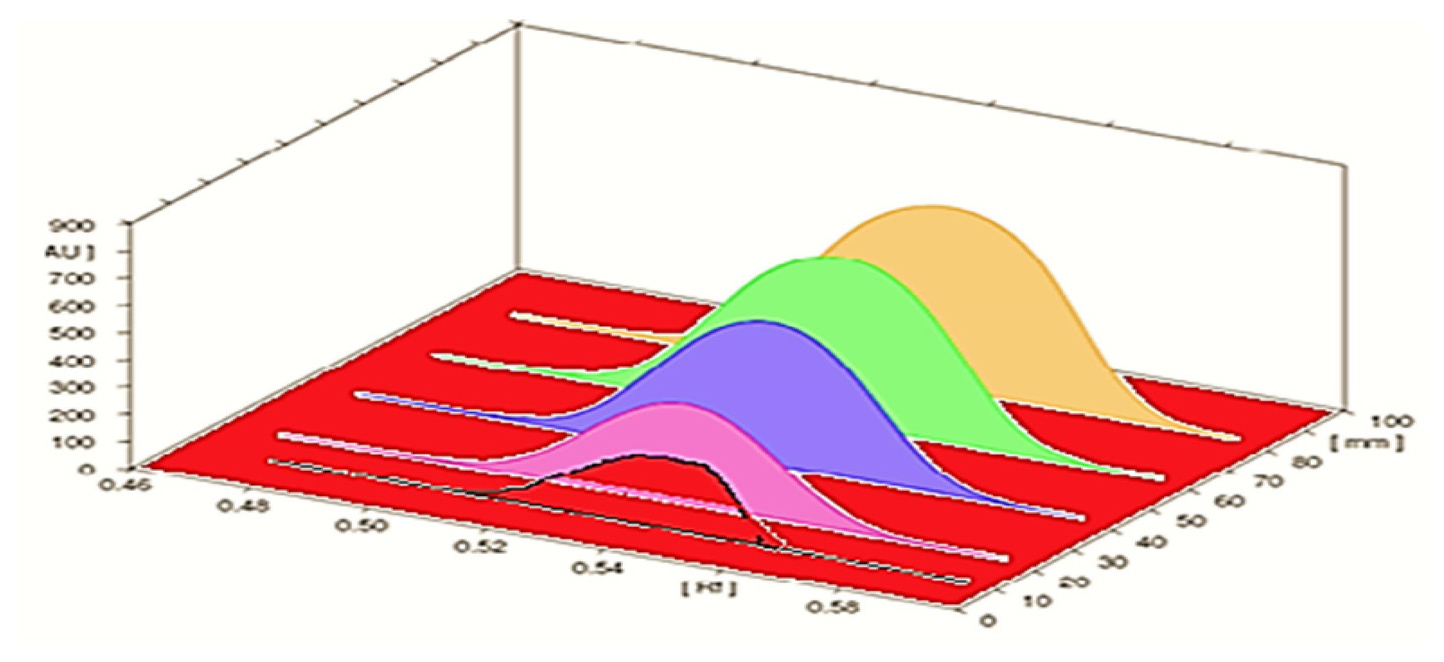
Figure 4:
2D calibration linearity graph (2 – 12 μg/band)
Drug ATV | Linearity | Concentration (μg/mL) | Mean Peak Area | |
---|---|---|---|---|
2 | 348305 | |||
4 | 586610 | |||
6 | 814915 | |||
10 | 1349523 | |||
12 | 1585326 | |||
Intra-day | % Meana | S. D. | C. V. | |
99.81 | ±0.2712 | 0.3874 | ||
Inter-day | 99.98 | ±0.6723 | 0.3567 | |
Limit of Detection | 58.08 (ng/band). | |||
Limit of Quantification | 70.10 (ng/band). |
Linearity Intra-day and Inter-day Precision Data and LOD and LOQ.
To assess the method’s reproducibility, three different concentrations (100 μg/mL) within the linear range for both intra-day (within a day) and inter-day (across different days) were calculated to evaluate the precision of the procedure (Table 2). Both intra-day and inter-day measurements had extremely low Relative Standard Deviation (%RSD) values (less than 2). When the % RSD is low, the precision of the approach is good, indicating that the results are consistent no matter when the analysis is conducted.
The method’s accuracy was verified by adding known amounts of pure ATV to stressed samples (containing degradation products) at three different concentrations (80, 100 and 120 μg/mL). Excellent recoveries were achieved, with an average of 99.93% of the added drug being detected (Table 3). This indicates the method accurately measures the amount of ATV present. Additionally, the detection limit (58.08 ng/band) and quantification limit (70.10 ng/band) were determined (Table 2), defining the lowest amount of ATV that can be reliably identified and precisely measured, respectively.
% Recovery | Level of recovery | Amount of Table No. t powder equivalent to ATV taken (mg) | Amount of drug added (mg) | Amount of drug recovered (mg) |
---|---|---|---|---|
ATV | ATV | ATV | ||
99.60 | 80 % | 100.00 | 80 | 79.98 |
99.58 | 100.00 | 80 | 79.97 | |
100.17 | 100.00 | 80 | 80.01 | |
99.18 | 100 % | 100.00 | 100 | 99.81 |
99.67 | 100.00 | 100 | 99.85 | |
100.23 | 100.00 | 100 | 100.32 | |
99.14 | 120 % | 100.00 | 120 | 118.25 |
100.14 | 100.00 | 120 | 120.01 | |
100.18 | 100.00 | 120 | 118.21 | |
Mean a 99.69 S.D 0.525 %R.S.D 0.52 S.E 0.30 |
Results of Accuracy Studies and Statistical Values.
The robustness of the method, which refers to its ability to handle slight variations in test conditions, was also investigated (Table 4). Finally, the method was successfully applied to analyse capsules containing ATV and the results closely matched the labelled amount of the drug.
Factor | Chromatographic Changes | ||
---|---|---|---|
Mobile phase composition (±0.1 mL) | Level | Peak area | Rf Value |
4.9:2.9:0.5:0.5 | – 0.1 | 1353052 | 0.71 |
5.0+3.0+1.0+1.0 | 0 | 1343011 | 0.72 |
5.1:3.1:1.5:1.5 | +0.1 | 1352811 | 0.75 |
RSD | 0.20 | ||
Amount of mobile phase (v/v) (±1 mL) | |||
9 | – 0.1 | 1362621 | 0.70 |
10 | 0 | 1353012 | 0.72 |
11 | +0.1 | 1363032 | 0.77 |
RSD | 0.42 | ||
Duration of Chamber Saturation (±20 %) | |||
8 min | -20% | 1342623 | 0.77 |
10 min | 0 | 1353042 | 0.72 |
12 min | +20% | 1353051 | 0.78 |
RSD | 0.08 | ||
Time from Spotting to development | |||
5 min | – | 1362876 | 0.75 |
20 min | – | 1363052 | 0.72 |
40 min | – | 1367568 | 0.81 |
RSD | 0.90 | ||
Time from development to scanning | |||
5 min | – | 1362119 | 0.71 |
20 min | – | 1353050 | 0.72 |
40 min | – | 1344561 | 0.73 |
RSD | 0.102 |
Experimental domain for Robustness testing and obtained concentration.
Laboratory prepared mixtures
A series of samples with different ratios were prepared to evaluate the method’s ability to handle mixtures of the drug (ATV) and its breakdown products. Ten flasks were filled with precisely defined portions (1 to 9 mL) of a 100 μg/mL ATV solution. Next, they created combinations containing 10% to 90% breakdown products compared to ATV by adding varying volumes of acidic and oxidative degradation product solution (also 100 μg/mL) to each flask. They eventually added enough methanol to the flasks to make a final capacity of 10 mL. The developed HPTLC method was then used to examine these generated samples. Using matching regression models, the concentration of the intact medication (ATV) in each sample was determined; results are probably provided (Table 5).
Pathway of ATV
The degradants are depicted in the pathway of ATV in Figure 5.
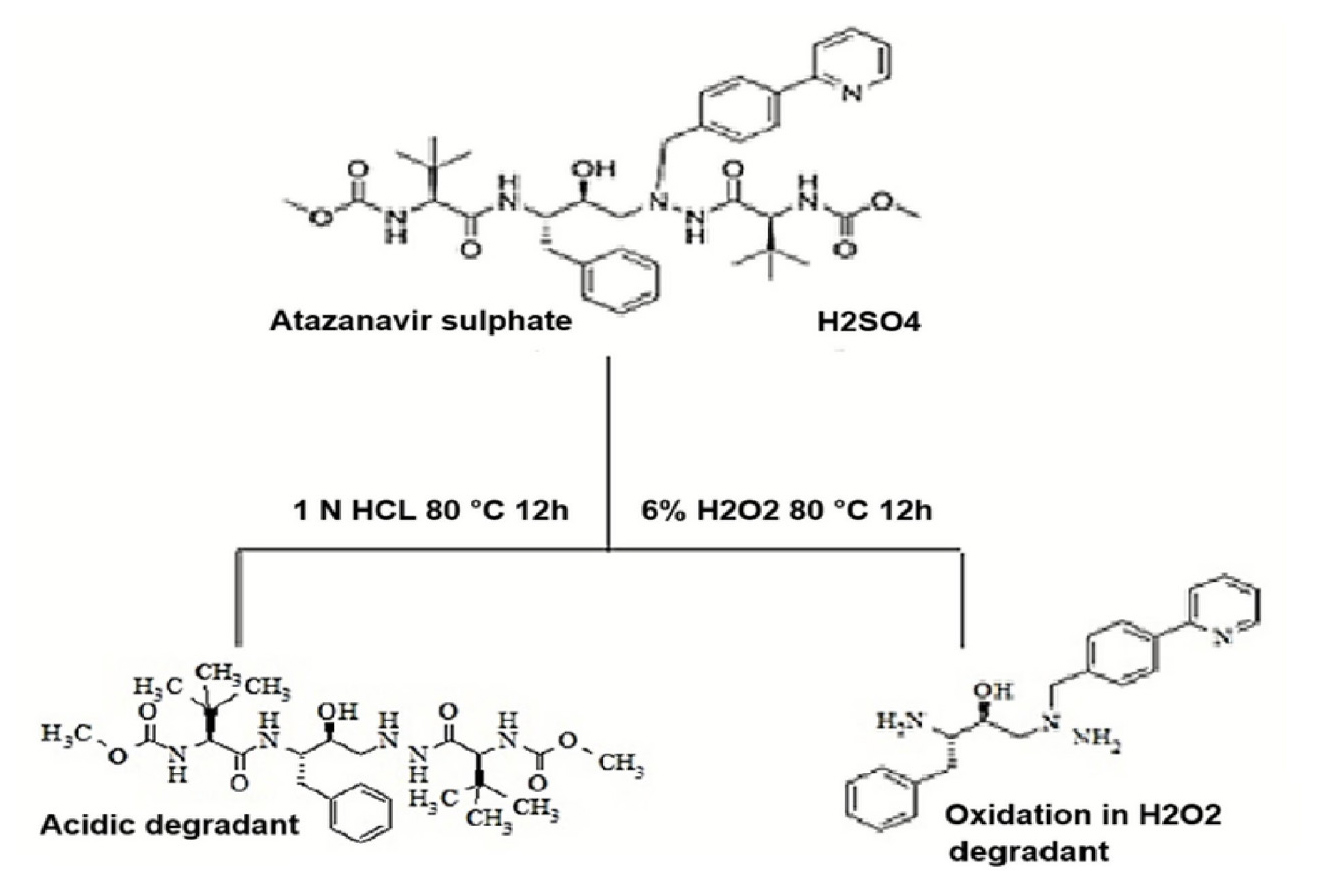
Figure 5:
Pathway of acidic Degradation and 6 % H2O2 degradation of Atazanavir sulphate are depicted below.
Stress studies
Investigations of how ATV breaks in different situations (stress testing) were conducted. It was found that ATV was stable in basic, light (photolytic) and heat (thermal) stress conditions but only broke down in acidic and oxidative settings (Table 4). By examining these diverse situations using a method known as HPTLC (High-Performance Thin-Layer Chromatography), this stability was verified. The HPTLC analysis (densitograms) is probably displayed in Figures 6–10, which are not displayed here. Crucially, the method’s capacity to effectively isolate the original drug from any breakdown products suggests that it is a useful tool for evaluating ATV stability.
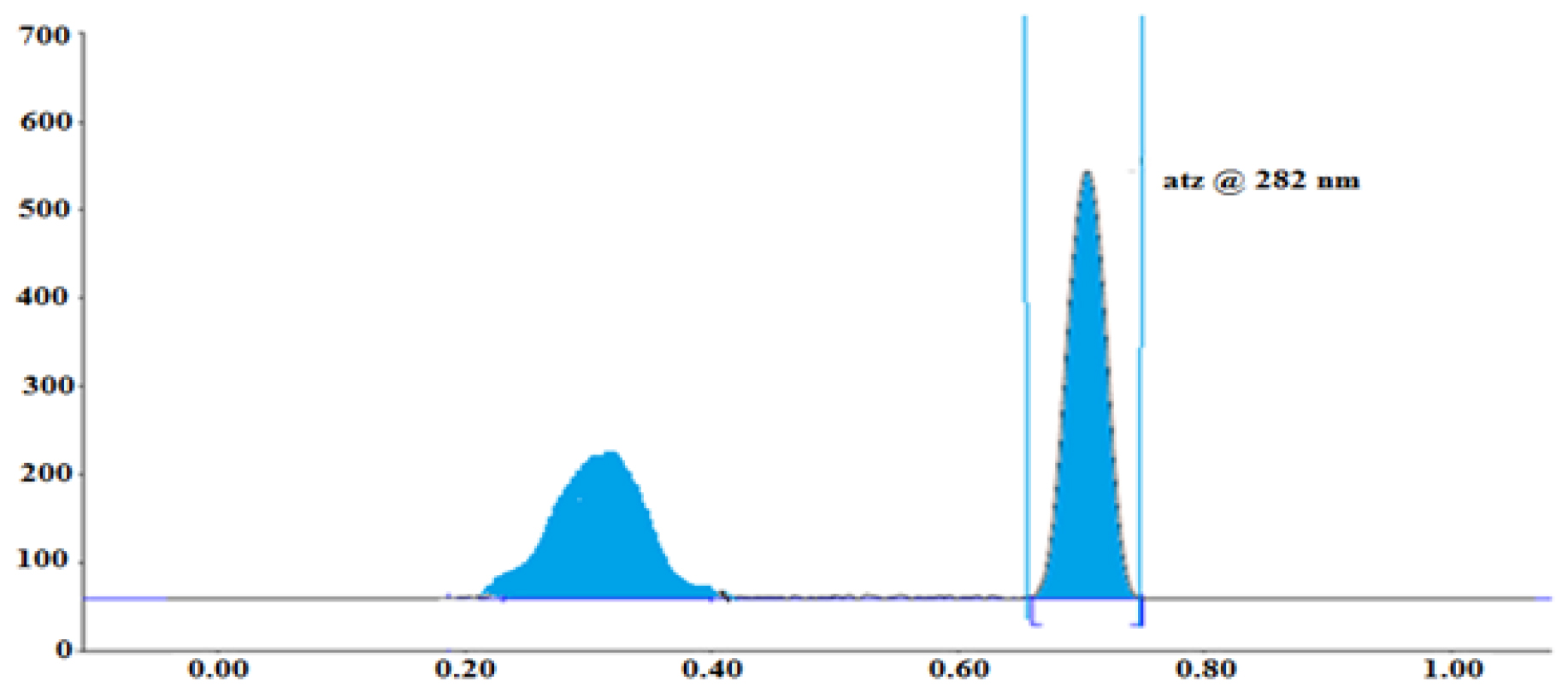
Figure 6:
Chromatogram showing Acidic degradation of Atazanavir sulphate capsule.
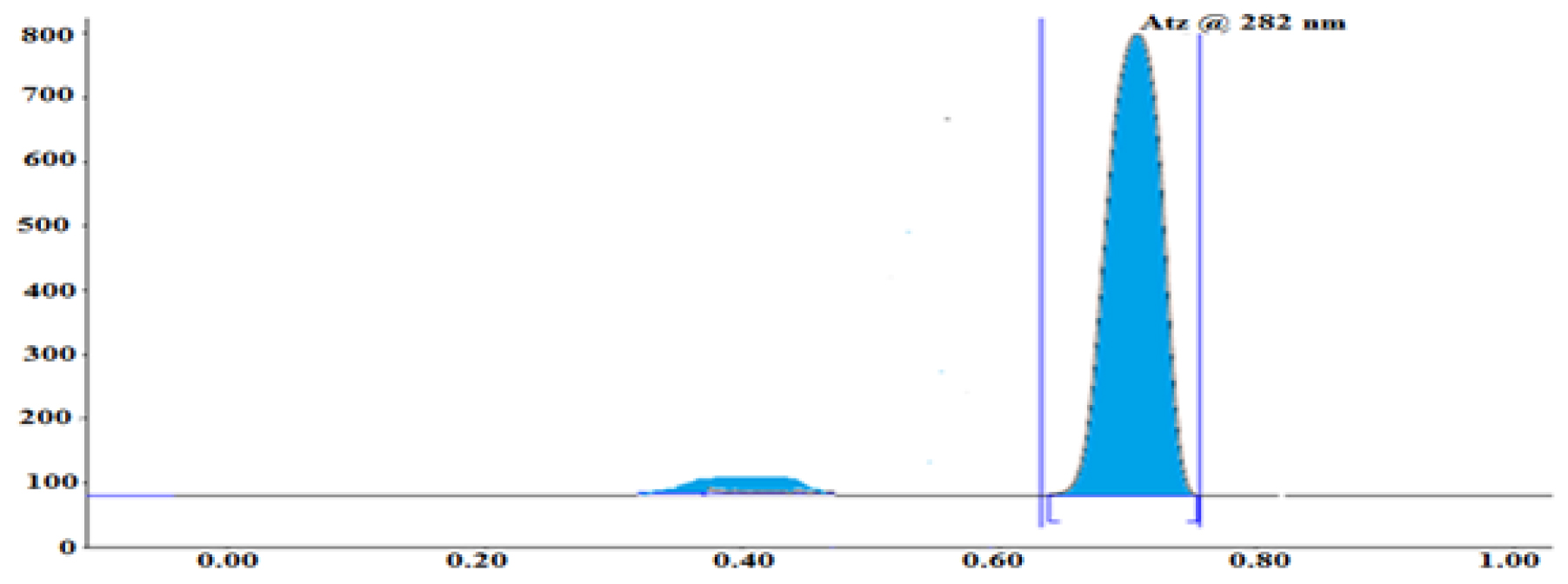
Figure 7:
Chromatogram showing alkali degradation of Navigator sulphate capsule.
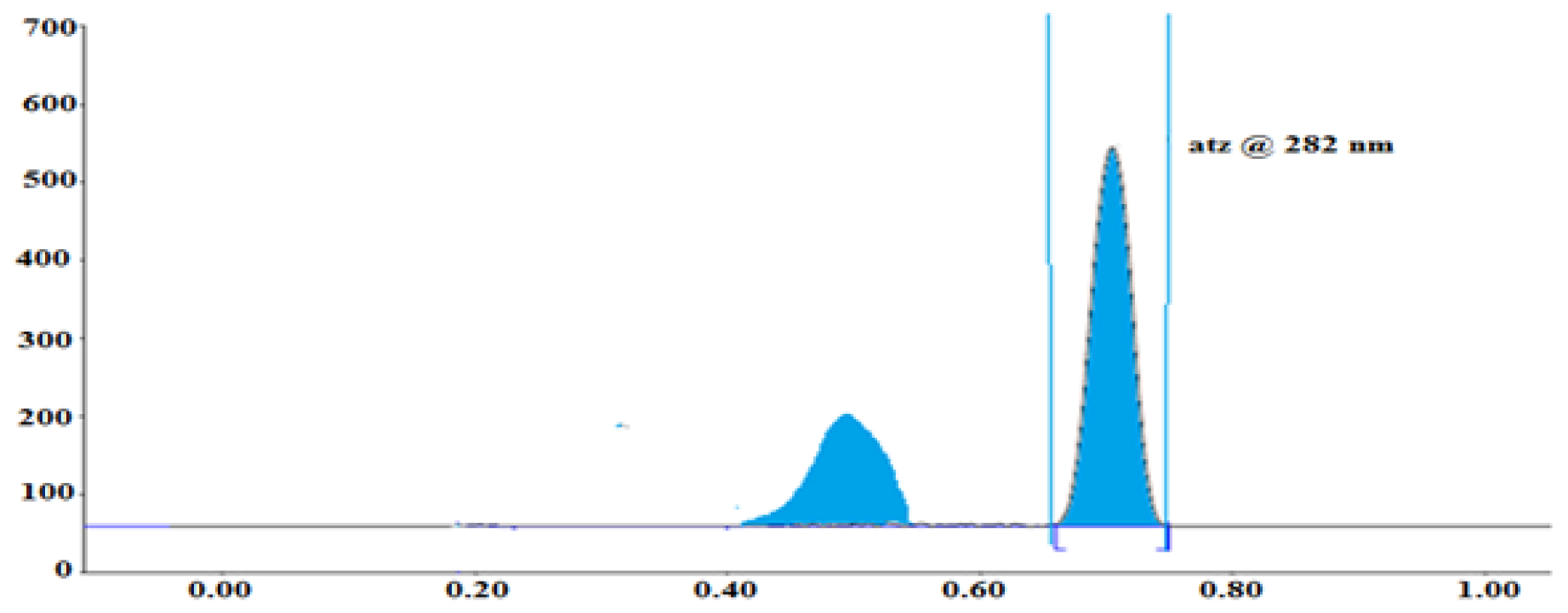
Figure 8:
Chromatogram showing Oxidative degradation of Atazanavir sulphate.
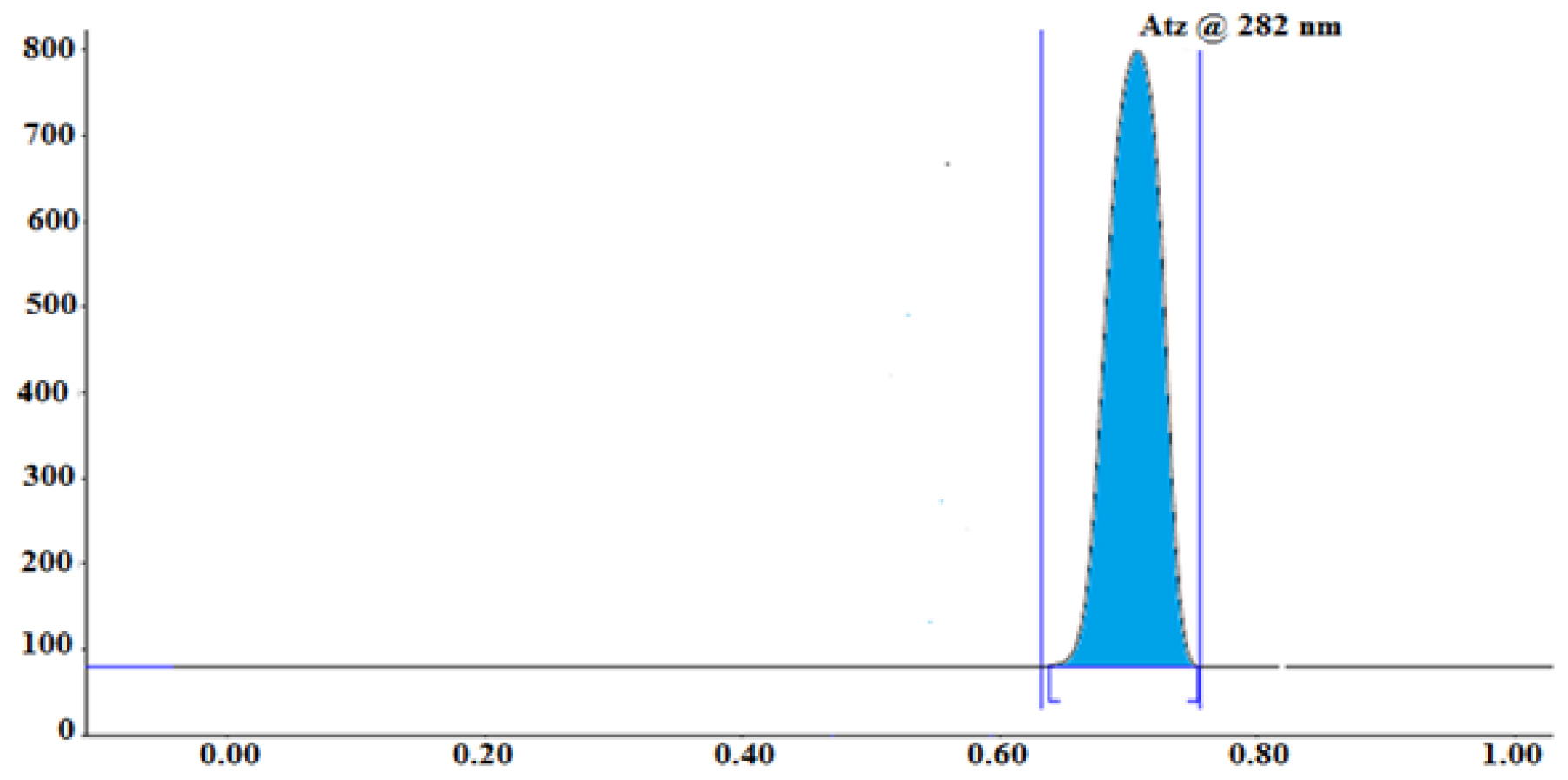
Figure 9:
Chromatogram showing Thermal degradation of Atazanavir sulphate capsule.
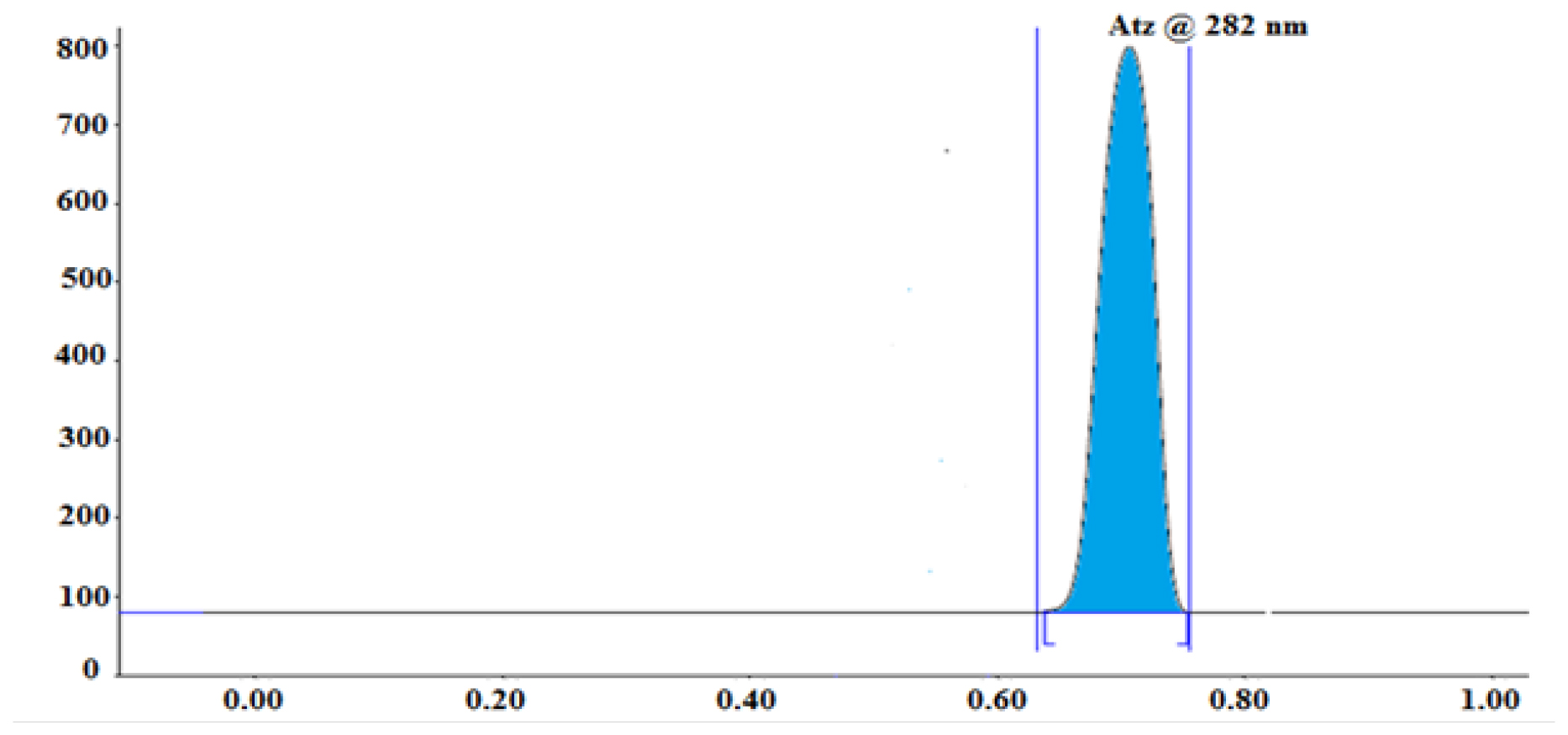
Figure 10:
Chromatogram showing UV degradation of Atazanavir sulphate Capsule.
Identification of acidic and oxide degradation of ATV
After heating for 12 hr at 80°C, ATV was hydrolyzed using 1N HCL and 6% H2O2, resulting in the breaking of the ester and alcohol groups, respectively. The mechanism proposed for the acidic and oxidative breakdown of atazanavir sulphate. The primary breakdown products observed in these studies (Figures 11 and 12) can be explained by this mechanism. Under oxidative and acidic conditions, their HPTLC method successfully verified total degradation (Table 5). This is probably because the regression equations employed for the analysis produced a high response at the temperatures and acid/alkali contents that were used. Significantly, no intact drug was found in the degraded samples (Table 5), adding more evidence to the method’s efficacy. Finally, mass spectrometry and Infrared (IR) spectroscopy were used to confirm the structures of the Degradation Products (DPs).
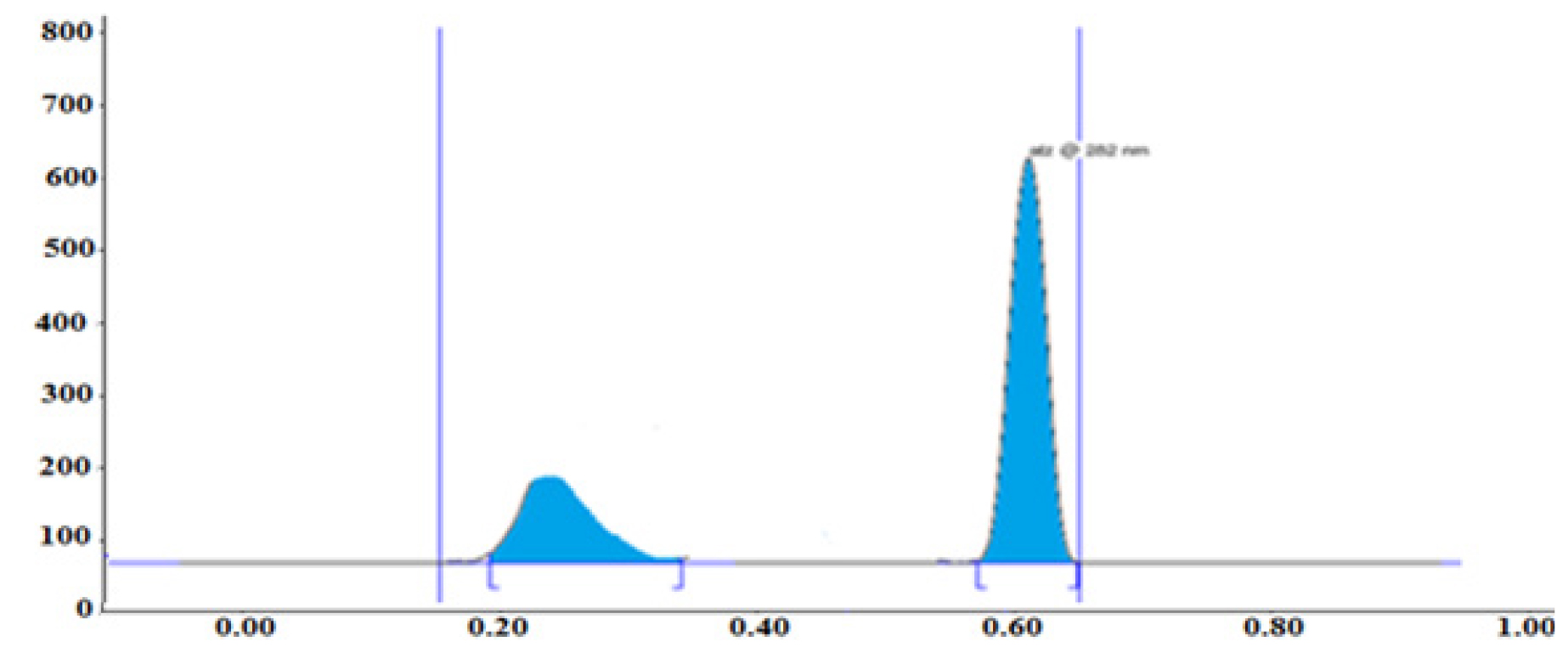
Figure 11:
Chromatogram of Acidic Degradation of ATV.
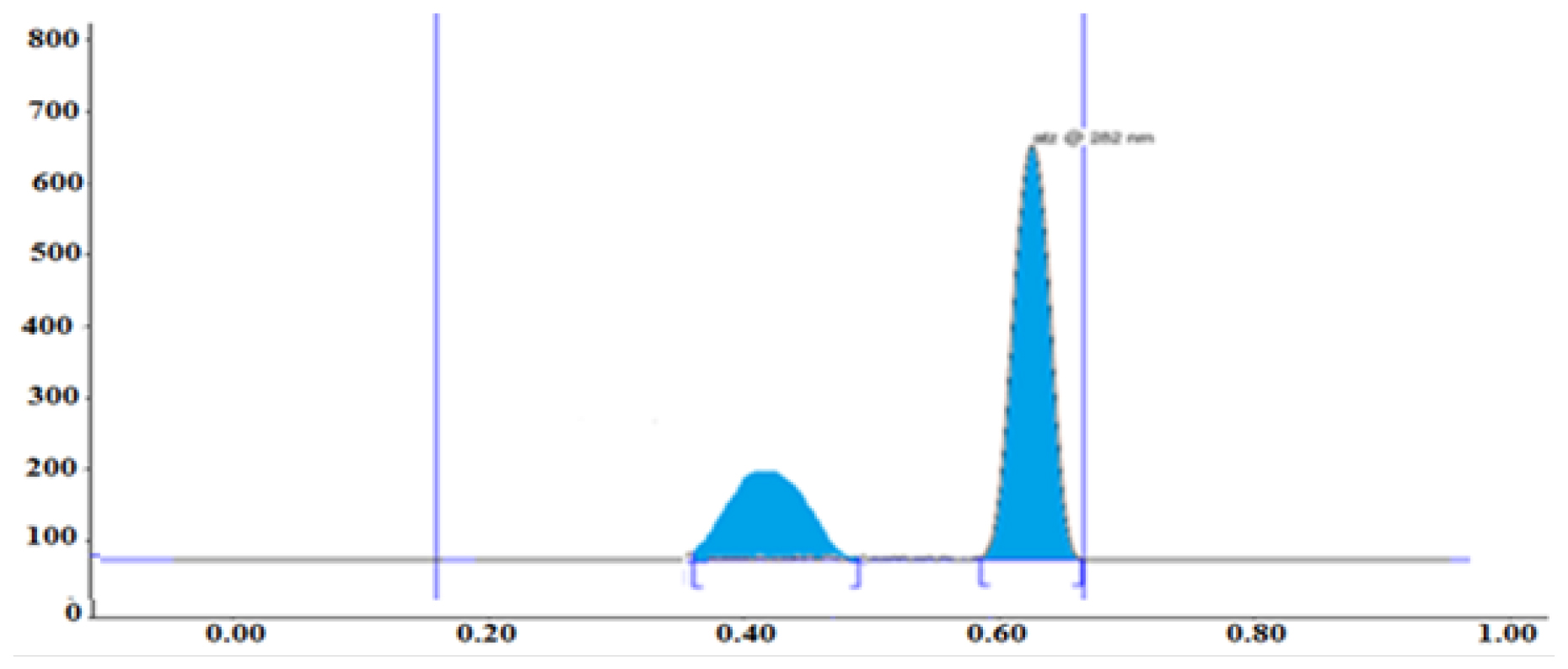
Figure 12:
Chromatogram of Oxidation Degradation Atazanavir sulphate.
H2O2 concentration on the rate of acidic and H2O2 degradation of 100 ug /mL of ATV.
Predicted structure of degradation products by IR and MS studies
The primary atazanavir sulphate degradation products were successfully recovered in the experiment, matching the predictions obtained from the suggested mechanism for acidic and oxidative breakdown. This supports the mechanism’s validity even further. In addition, these degradation products’ identities and structures were validated by mass spectrometry and Infrared (IR) analysis. The parent Molecule mass (M) and its isotope peak (M+2) in Table 6 were also observed to validate the molecular weights of the proposed breakdown products.
DP-I
Using Infrared (IR) spectroscopy for analysis, the acidic Degradation Product (DP) was shown to have unique characteristics. In particular, absorption peaks indicative of functional groups such as Amine (NH2) at 3150.3 cm1, Carboxylic acid (COOH) at 1777 cm1 and 1207.8 cm1 and Alkane (C-H) at 3402.5 cm1 were observed in the infrared spectrum (Figure 13. a). These features were compared with the undegraded ATV standard’s spectrum (Figure 13. c). Similarly, the molecular weight of the suggested degradation product was verified by mass spectrometry analysis. Molecular ion peaks at 370.02 m/z and 341.02 m/z were visible in the mass spectrum, matching the predicted weight. (Figure 14) probably shows the structures of both the initial ATV and the degradation result (14. a compared to 14. c). In general, the mass spectral and infrared data strongly indicate the identification and structural features of the acidic degradation product.
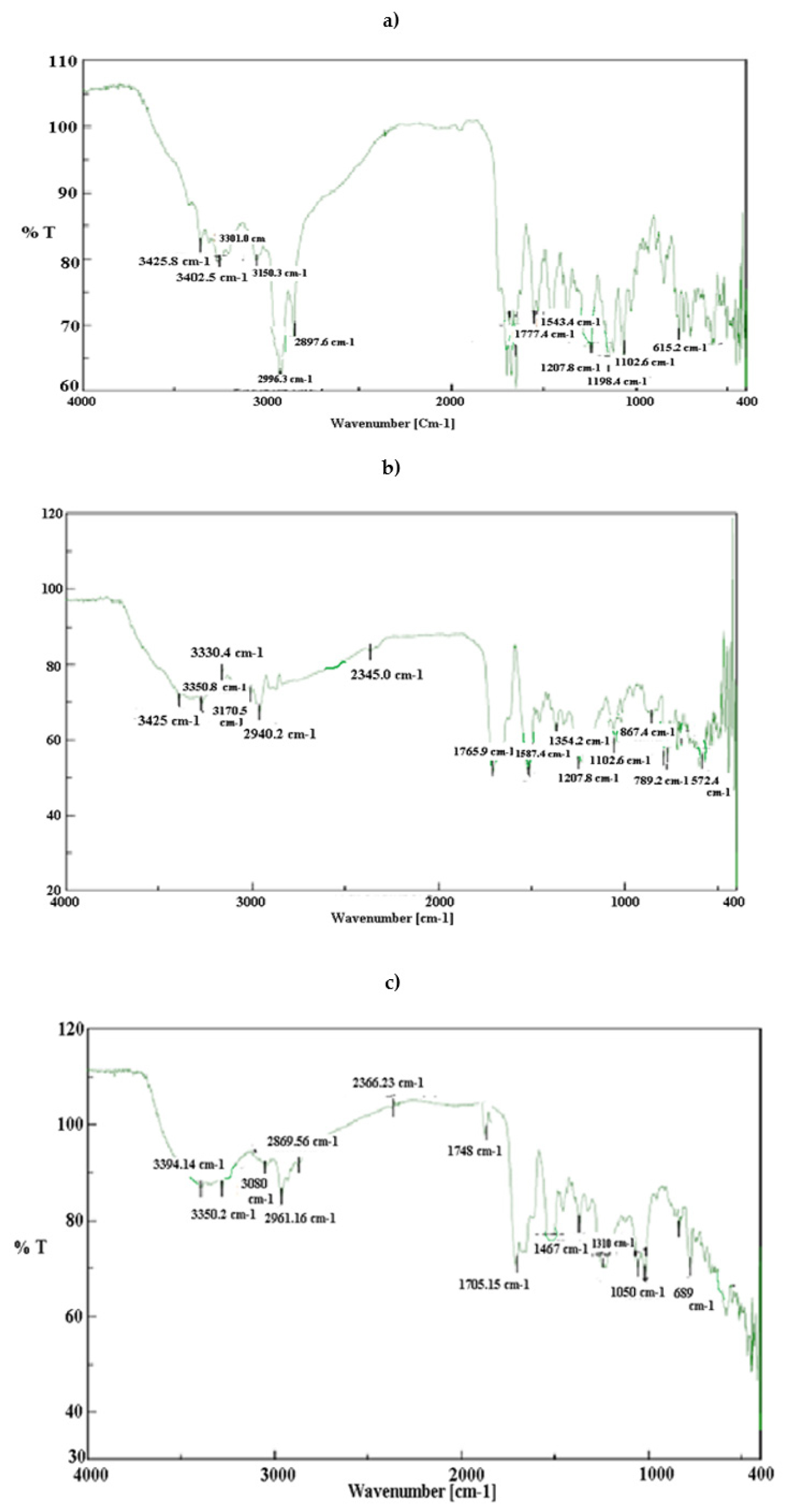
Figure 13:
Spectra obtained by FTIR (TGS) a) acidic degradation b) degradation after oxidation using H2O2 c) Standard Atazanavir sulphate.
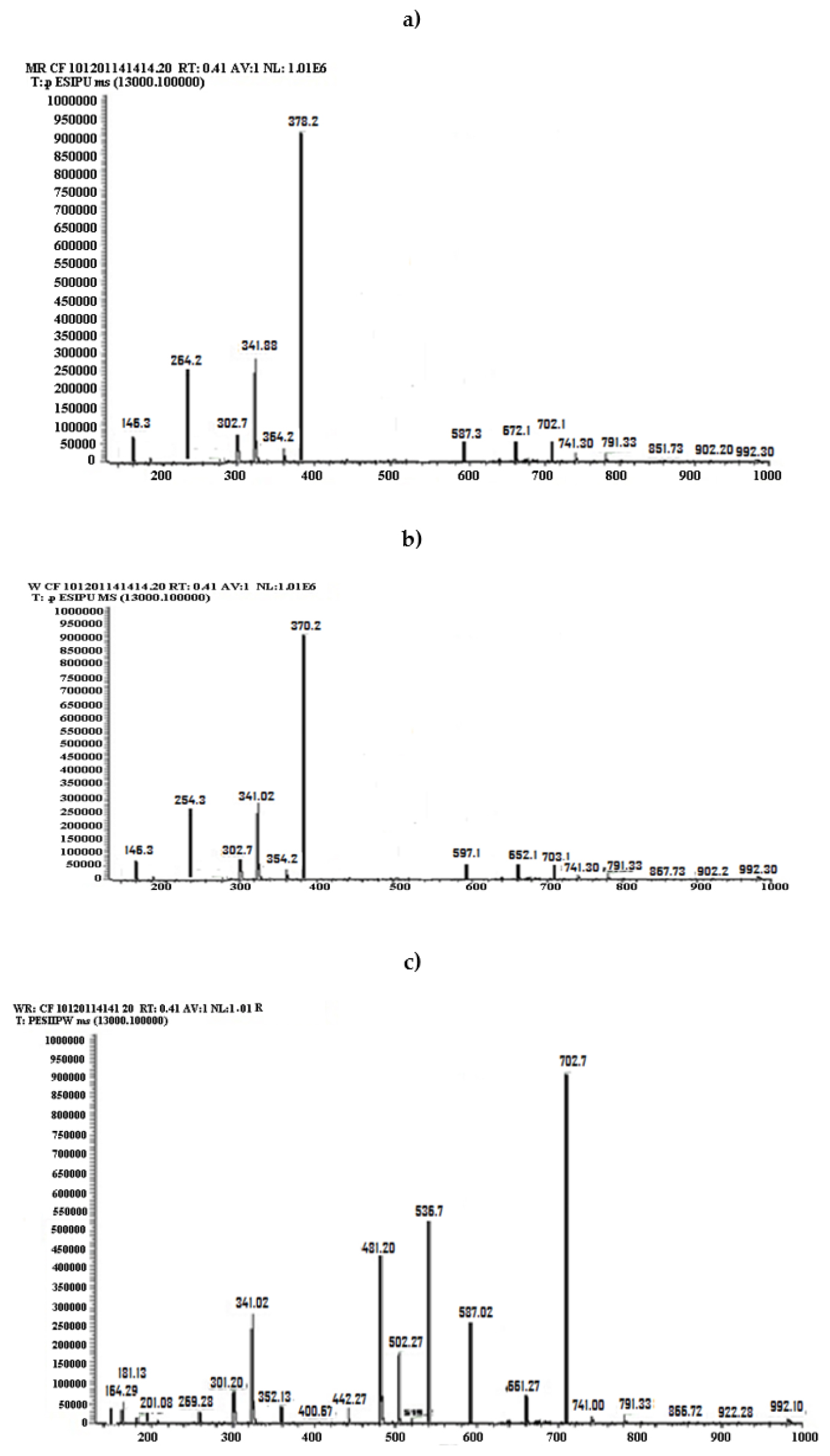
Figure 14:
Spectra obtained by TOF-Q Mass Spectrophotometer a) acidic degradation b) degradation after oxidation using H2O2 c) Standard Atazanavir sulphate.
DP-II
Using infrared spectroscopy, the oxidative degradation product was studied, just like the acidic degradation product. Absorption peaks indicative of functional groups such as amines (NH2 at 3425.4 cm-1), hydroxyl groups (OH at 3350.8 cm-1 and 3330.4 cm-1) and aromatic rings (1507.9 cm-1) have been observed in the IR spectrum (Figure 13B). The undegraded ATV standard was used to compare this spectrum (Figure 13C). Analysis using mass spectrometry confirmed the proposed oxidative DP structure. Molecular ion peaks at 378.2 m/z and 587.3 m/z were indicated by the mass spectrum, which matched the predicted molecular weight. (Figures 14A and 14B) most likely show the molecular structures of the oxidative DP and ATV, respectively. Overall, the identification and structural elucidation was supported by the findings from mass spectrometry and IR, which are compatible with the acidic DP (Figure 13A, B, C) and (Figure 14A ,B, C).
Mechanistic approach towards the degradation of ATV
The proposed pathways for atazanavir’s oxidative and acidic breakdown are shown in (Figures 13 and 14). It’s interesting to note that although aromatic amines are often resistant to hydrolysis, ATV is prone to breakdown in acidic environments due to the presence of an alkyne group conjugated to the aromatic ring.
DISCUSSION
ATV was found to be susceptible to acidic and oxidative stress conditions provided by ICH-recommended stress conditions. Three Degradation Products (DPs I-II) were separated using HPTLC. Degradants were elucidated through mass spectral data using IR and MS/TOF structures, and degradation pathways and degradation products were established. This study revealed detailed information on the degradation pathway of ATV for the first time, enhancing knowledge regarding the degradation pathway and degradation products and allowing strategies for its stabilisation in the future.
CONCLUSION
ATV degradation under stressful environments was investigated in a study that adopted the International Council for Harmonisation’s (ICH) recommendations. Except for acidic and oxidative conditions, ATV showed very little degradation. After stress testing, three novel degradation products (DPs I-II) were found using HPTLC. Notably, under acidic stress, DP-I developed and under oxidative stress, DP-II. This is supposedly the first report of this sort. The ATV’s whole degrading route was found via IR and MS/TOF analysis of the drug and DPs.
Cite this article:
Patil P, Bagde S, Shegokar N, Pawar V, Kalad K, Bharti S. Identification, Separation and Characterization of Acidic and Oxide Degradation of Atazanavir Sulphate Under ICH-Recommended Stress Conditions by HPTLC with MS/TOF. Int. J. Pharm. Investigation. 2024;14(3):966-79.
ACKNOWLEDGEMENT
The authors are grateful to Cipla Ltd., India, for proving the gift sample of atazanavir sulphate as a pure drug. The authors are also thankful to Anchrom Test Lab Pvt. Ltd., Mumbai, for conducting some of the analytical work using the HPTLC method and using the lab for the preparation of degradants.
ABBREVIATIONS
ATV | Atazanavir sulphate |
---|---|
DPs | Degradation Products |
MS/ TOF | Mass spectrometry/Time-of-flight |
PI | Protease Inhibitor |
HPTLC | High-Performance Thin-Layer Chromatography |
UV | Ultra violet |
SPE-LC-ESI-MS/MS | Solid Phase Extraction -Liquid Chromatography -Electrospray Ionization- Tandem Mass Spectrometry |
ICH | International Conference on Harmonisation |
FT/IR | Fourier-Transform Infrared Spectroscopy |
LOD | Limit of Detection |
LOQ | Limit of Quantification |
RTV | Ritonavir |
%RSD | % Relative Standard Deviation |
References
- Wempe MF, Anderson PL. Atazanavir metabolism according to CYP3A5 status: an assessment. Drug Metab Dispos. 2011;39(3):522-7. [PubMed] | [CrossRef] | [Google Scholar]
- Sivasankar K, Karunakaran K, Thambichetty A, Manasa D, Bhargavi K, Kagitala V, et al. Development and validation of HPTLC method for the estimation of atazanavir sulphate in bulk drugs and combined dosage form. International Journal of Pharmaceutical Sciences Review and Research. 2014;25:217-20. [PubMed] | [CrossRef] | [Google Scholar]
- Dey S, Reddy YV, Reddy T, Sahoo SK, Murthy PN, Mohapatra S, et al. Method development and validation for the estimation of atazanavir in bulk and pharmaceutical dosage forms and its stress degradation studies using UV-vis spectrophotometric method. Int J Pharm Biol Sci. 2010;1(3):1-2. [PubMed] | [CrossRef] | [Google Scholar]
- Khanage SG, Deshmukh VK, Mohite PB, Dhamak VM, Raju SA. Development of derivative spectrophotometric estimation of atazanavir sulfate in bulk drug and pharmaceutical dosage forms. Int J Pharm Health Sci. 2010;1(3):149-54. [PubMed] | [CrossRef] | [Google Scholar]
- Bari NA, Shailendra Kela P, Shailesh Sharma N, SarojShirse V, Vishnu Choudhari P. Spectrophotometric simultaneous determination of atazanavir and ritonavir in combined tablet dosage form by ratio derivative and area under curve method. Pharm Chem. 2012;4:208-13. [PubMed] | [CrossRef] | [Google Scholar]
- Konidala SK, Sujana K, Rani AP. New Validated RP-HPLC method for the Determination of atazanavir sulphate in Bulk and Dosage form. Pharm Chem. 2012;4(3):1305-10. [PubMed] | [CrossRef] | [Google Scholar]
- Srinivasu K, Rao JV, Raju NA, Mukkanti K. A validated RP-HPLC method for the determination of atazanavir in pharmaceutical dosage form. J Chem. 2011;8(1):453-6. [CrossRef] | [Google Scholar]
- Behera A, Sethy K, Sankar DG, Moitra SK, Si SC. Statistical correlation and simultaneous estimation of atazanavir sulfate and ritonavir in fixed dosage form by high performance liquid chromatography and high performance thin layer chromatography. J Liq Chromatogr Relat Technol. 2012;35(12):1731-49. [CrossRef] | [Google Scholar]
- Venkatesh J, Chowdary MS, Haritha D, Prasad VV, Reddy VV. Reverse phase high performance liquid chromatographic estimation of atazanavir and ritonavir in pharmaceutical dosage form. Asian J Res Chem. 2013;6(9):855-8. [CrossRef] | [Google Scholar]
- Pawar DS, Dole M, Sawant S, Salunke JM. Development and validation of RP-HPLC method for the simultaneous estimation of atazanavir sulphate and ritonavir in bulk and formulations. Int J Pharm Pharm Sci. 2013;5(Suppl 3):905 [CrossRef] | [Google Scholar]
- Dey S, Subhasis Patro S, Suresh Babu N, Murthy PN, Panda SK. Development and validation of a stability-indicating RP-HPLC method for estimation of atazanavir sulfate in bulk. J Pharm Anal. 2017;7(2):134-40. [PubMed] | [CrossRef] | [Google Scholar]
- Cateau E, Tournier N, Dupuis A, Le Moal G, Venisse N. Determination of atazanavir in human plasma using solid-phase extraction and high-performance liquid chromatography. J Pharm Biomed Anal. 2005;39(3-4):791-5. [PubMed] | [CrossRef] | [Google Scholar]