ABSTRACT
Background
3D printing, also known as additive manufacturing, is a rapidly advancing technology in the life sciences sector. Several countries, including the USA, Canada and Singapore, have established regulations for 3D printing of pharmaceutical products. The growing demand for personalized medical devices presents a significant challenge to traditional manufacturing methods.
Materials and Methods
This study aims to provide a concise overview of the introduction, history and evolution of 3D printers, their development process and their role and guidelines and focus is on exploring regulatory parameters related to 3D printing in the United States, Canada and Singapore. The Food and Drug Administration (FDA) has issued guidance outlining its initial considerations for technical aspects specific to devices using additive manufacturing. The regulatory landscape of 3D-printed medical devices and biologics in the United States is discussed, emphasizing key challenges and considerations.
Results and Discussion
A comparative analysis in Selected counties was conducted across various aspects, including user needs, design input, performance requirements, design processes, functional requirements, design output, verification and validation, bio-printing regulatory perspectives, standardization and certification.
Conclusion
As additive manufacturing technology advances, 3D bio-printing has emerged as an efficient tool for regenerative medicine and tissue engineering, integrating computer-aided manufacturing into healthcare delivery. The applications of 3D printing in the healthcare field extend to creating and implementing patient-specific solutions.
INTRODUCTION
Continuing the trends of earlier years, there has been significant improvement in combinatorial production techniques and scientists have been investigating their uses in multiple domains within engineering and healthcare. This process transforms a digital 3D model into a tangible prototype without the need for detailed process planning, physical equipment, or Molds. This technology possesses a remarkable capacity to craft intricate prototype shapes using a range of materials, including nylon, polymers and also metals. It is proficient at producing biocompatible implants that fulfil structural criteria.1 3D printing falls under the category of combinatorial production and encompasses various forms of this manufacturing technique.2 While 3D printing and rapid creation are often used interchangeably, we shall use the term “3D printing” for simplicity.3,4 Furthermore, thanks to this technological advance, producers may now make intricately designed interior parts for their gadgets or gadgets that are tailored to a patient’s unique physiology. These capabilities have sparked considerable interest in applying 3-D printing to manufacture not only medical devices but also a variety of other products, ranging from food and household items to automotive components. In certain 3D Printing methods, up to 98% of the raw material is utilized in the final product.4,5
History
Charles Hull, one of the founders of 3D Systems, revolutionized the field by inventing “Stereolithography” in 1984, a technology that he received a patent for this work. Hull, who was a technologist and physicist, initially worked for a company manufacturing ultraviolet lamps that were applied as coatings on household surfaces. The spark of inspiration came when he conceived of a novel application for UV light: transforming digitally assisted design creations into 3D objects. He gained permission to use the company’s test centre during off-hours, particularly at nocturnal and on holidays. In his experimentation, Hull discovered that photo-polymers, acrylic substances, could solidify when exposed to UV light. Subsequently, he designed a machine equipped with a UV laser to engrave acrylic films into specific forms, stacking these films to form tangible objects. Developing the code that told a printer about how to etch the acrylic sheets was one of his main obstacles, which caused him to concentrate on rather simple forms. Hull made $100,000 in the year 1988 when he delivered his first 3D printer following years of invention and design.
The foundational concept behind combinatorial production can be traced back to natural occurrences, such as the gradual formation of minerals in underground rock formations like Stalactites and Stalagmites, where dripping water deposits thin layers over time. A more contemporary example is the common desktop printer, where distinct points of toner are added to create a duplicate. Similarly, a 3D printer only adds the material where required based on a digital file. In contrast, some traditional manufacturing processes often referred to as “Subtractive Manufacturing,” involve cutting away excess material to shape the desired part. This method may lead to substantial material wastage, with reported figures indicating as much as 30 pounds of waste for every pound of usable material, according to the Energy Department’s Oak Ridge National Lab.4 Initially, 3D Printing technology carved out its specialized role in the medical sector, primarily for applications such as dental implants and personalized prosthetics in the 1990s. As time progressed, researchers enhanced the technology, progressing to the point of growing tissues from a patient role personal cell and employing 3D-printed scaffolds for provision.
As technology continued to evolve, the medical field saw remarkable achievements, such as the creation of a miniature kidney and the pursuit of fully functioning organs without the need for scaffolds. In 2008, the first prosthetic leg was successfully produced using 3D printing. Notably, in 2012, a manufacturing company in Holland, Layer wise, 3D printed a jaw. Fast forward to today, 3D printers have become more affordable and widely utilized in hospitals, with the potential to produce vital human organs.4
What is 3D Printed Medical Device
Combinatorial production, also referred to as 3D printing, is the process by which three-dimensional objects are systematically constructed, utilizing raw materials guided by a digital blueprint.6 Three-dimensional (3D) printing falls under the category of combinatorial production, a method that creates an object by layering raw materials, typically based on a digital 3D file derived from medical images.5 This form of manufacturing technology, known as combinatorial production, entails the progressive deposition of materials such as powder, plastic, or metal to build a 3D model according to a Computer-Aided Design (CAD) model. Unlike traditional manufacturing methods, this approach entails the addition of materials layer by layer instead of the removal of material. In surgical contexts, this technology finds application in generating models that enhance comprehension of intricate patient pathologies and anatomical structures.1 This includes the design of more effective and patient-matched pharmaceutical products as well as bio-inspired medical devices.7 Within the realm of “medical 3D printing,” the creation of “3D-printed medical devices” has emerged as a significant area of focus. Within the identified five application types, “3D-printed in vitro medical devices” and “3D-printed implants” in the initial two categories can be classified as medical devices. Unlike the first two applications, which predominantly utilize non-living materials such as metals and polymers for 3D printing, the subsequent three applications frequently require the development of biomaterials and advancements in 3D bioprinting technologies. The production and use of these biologically derived products are currently neither swift nor fully developed due to the present state of technological advancement; they are predominantly in the research and exploration phase. Consequently, it is worthwhile to discuss the efficacy and regulatory aspects of 3D-printed medical devices.8 Broadly, the regulatory complexity associated with 3D-printed medical devices is not rooted in the enigmatic aspects of 3D Printing technology itself, but rather in the distinctive attributes of customization and the decentralized nature of this manufacturing process.9 The current and possible uses encompass:
Tailored 3D-printed medications, devices for drug delivery and implants.
Medical prosthetics.
Models of anatomical structures for surgical planning and training.
The creation of tissues and organs through bioprinting.10
A medical device’s functionality is independent of immune-mediated, pharmaceutical, chemical-based, or metabolic functions. The materials and goods used in medical devices may consist of one or more biological materials that need to be:
Conducting in vivo implantation or other research to assess the biomaterials’ biological compatibility.
Precautionary testing involves determining the device’s or clinical product’s local and systemic toxicity and
The clinical product or completed device’s effectiveness is tested.11
3D printed medical device life cycle administration
The comprehensive oversight of combinatorial production medical devices spans from ensuring quality in design and production to evaluating quality, conducting pre-market assessments and conducting post-market monitoring of the final product. The regulation and standardization of these medical devices remain a prominent and evolving topic. In recent years, there has been an encouraging improvement in acquiring significant knowledge in the standardization of medical devices that are created using 3D technology. The processes of quality control and performance evaluation are gradually advancing, accompanied by the introduction of regulatory regulations and guidance. Despite the initial complexity of these regulations, it is important to recognize that all aspects are interconnected.8 The overarching objective is the full life cycle management of combinatorial production medical devices, emphasizing the seamless integration of these processes, as shown in Figure 1.
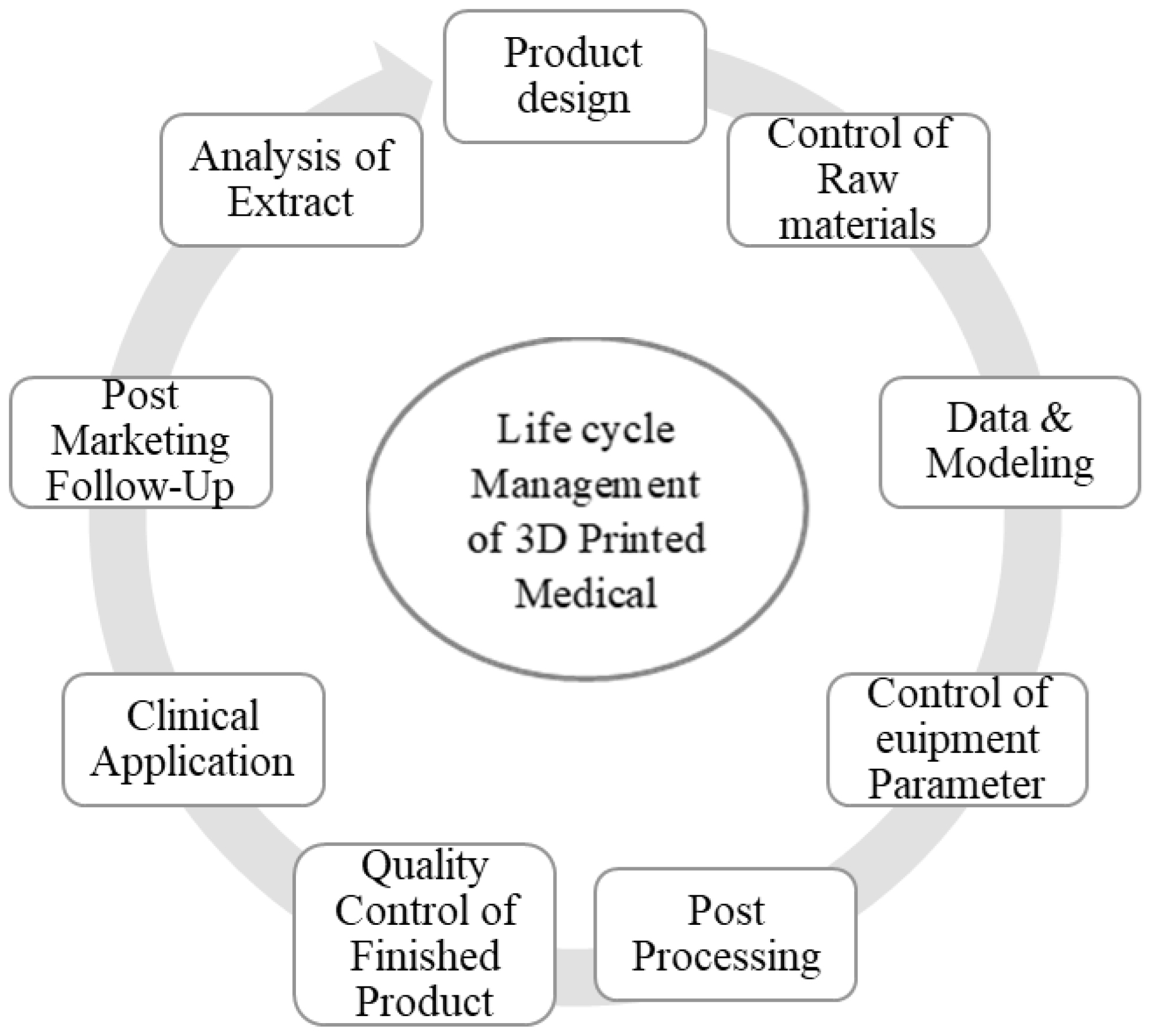
Figure 1:
Life cycle management of 3D-printed medical devices.
Medical Device Vs 3D-Printed Medical Devices
A medical device and a 3D printed medical device are both tools or instruments used in the field of healthcare, but they differ in terms of their manufacturing processes and some characteristics. Here’s a difference between a traditional medical device and a 3D printed medical device in Table 1.
Medical Device | 3D Printed Medical Device |
---|---|
These devices can be used for diagnosis, treatment, monitoring, or prevention of diseases and medical conditions. | The specific type of medical device that is manufactured using CAD and MRI, 3D Printing technology. |
Manufactured using various methods, including traditional manufacturing techniques like injection molding, machining and casting. | Produced using 3D Printing technology, where material is deposited layer by layer to build the final product. |
Mass-produced and may not be customized to individual patients. | Customized or patient-specific, designed according to the patient’s anatomy or unique medical needs. |
Medical devices can include a wide range of products such as orthopedic and cranial implants, surgical instruments and dental restorations. | Surgical guides, musculoskeletal implants, hearing aids, orthotics and dental devices. |
Produced in large quantities, which can help reduce the cost per unit. | 3D Printing can be cost-effective for small-batch or customized production. |
The Food and Drug Administration (FDA) released a long-awaited initial guidance document on 3D printed medical devices titled “Technical Considerations for Additive Manufactured Medical Devices.” This represented a pivotal advancement in fostering the innovation and utilization of 3D printing in healthcare. The draft guidance covers essential aspects, encompassing design, manufacturing and device testing considerations. While it may not address intricate 3D printing processes, point-of-care devices, or bioprinting involving cells or human tissues, it nevertheless represents substantial progress for the field of 3D printed medical devices by offering specific guidelines for design, manufacturing and testing.2
In the healthcare sector, 3D Printing holds significant appeal as a technology that can assist healthcare professionals, administrators and medical device manufacturers in various ways, such as:6–13
Visualizing and strategizing for intricate medical procedures.
Crafting customized or patient-specific devices.
Constructing devices with intricate internal and external designs using biocompatible materials.
Generating devices or supplies as required directly at the point of use.
Simplifying supply chain operations.
Minimizing inventory requirements.
Cutting down on labour expenses.
Creation of 3D objects by using combinatorial production Applications
The patient-specific analysis is crucial for acquiring precise medical imaging data for each patient. Various advanced imaging technologies such as Magnetic Resonance Imaging (MRI), modern Multi-Detector Computer Tomography (MDCT), CT scans, X-rays and 3D scanners offer rapid, high-resolution data, facilitating the creation of a detailed 3D representation of the patient’s anatomy.1 Additionally, the conversion of digital medical images into 3D physical models further enhances the understanding of the individual’s medical condition. This conversion takes place in three steps discussed in Table 2. Advanced medical imaging provides an accurate and more manageable diagnosis by capturing the data. Enhanced visualization is possible using 3-D reconstruction images produced by these scanning techniques. This results in more accurate diagnosis and improved surgical management”.14,15
Sl. No. | Steps used | Description |
---|---|---|
1 | Image acquisition | 3D medical images, characterized by high quality and resolution, are produced through the utilization of diverse scanning technologiesThe crucial step in this process is image acquisition, as the precision of the physical mode Accuracy depends on CT, MRI and 3D scanners. |
2 | Post-processing of images | With image afterward software, DICOM (Digital Imaging and Communications in Medicine) pictures are obtained for data rebuilding.It is beneficial for looking at bone features and providing specific details like joint alignments and fractures.CAD software transfers data into Standard Triangulation Language (STL) format and turns a three-dimensional model into a set of polygons.The STL format data file serves as the input for the 3D printing machine during component production.DICOM pictures are crucial for 3D printing technology as well as data from medical imaging particularly to the patient. |
3 | 3D printing | The 3D model is divided into “very thin” cross-sectional layers using CAD software. Layer after layer, a three-dimensional physical model is created using combinatorial manufacturing technology. |
3D Printed technologies
Various manufacturing methods can be employed to produce 3D-printed and bio-printed objects, typically characterized by a set of Following components.6–16
Images or other data that the design program can use.
Software is required for creating, modelling and transferring digital models into printer instructions on a computer.
A computer-assisted printer17,18
The right materials to stack to create the intended result Table 3. Lists common 3D printing production methods that are employed in therapeutic settings.5
3D Printing techniques | Description and Considerations |
---|---|
Vat Photopolymerization | |
Stereolithography (SLA). | The most ancient technique in 3D Printing involves the utilization of a laser that scans to examine a container holding fluid polymers that are photo-sensitive (resin). By the design data, it selectively freezes portions that cover the liquid’s edge. A movable construction scaffold slides to provide profundity to the material as each layer hardens. Software-generated reinforcements are necessary for this procedure and must be carried from the finished result.6-19 |
Powder-Bed Fusion | |
Laser sintering with selectivity (SLS). | Utilizing a laser or electron beam, a 2D cross-section is traced within a bed of finely textured thermoplastic powder, which consists of diverse materials such as nylon and metals. The powder is heated to a fusion point, causing it to bind together. After tracing each 2D slice, a fresh layer of powder is introduced to iterate the procedure until the desired object takes shape. When this method is employed on metal alloys, it is known as direct metal laser sintering. |
Laser melting that is selective (SLM). | In line with SLS, but with the powder being melted by the laser’s heat, resulting in a homogeneous component. When you are utilizing just one metallic powder, you can utilize it. Although pores cannot be regulated, the material is tougher. |
Heat sintering with selection (SHS). | Comparable to SLS, however sintering the powder is done with a thermal print head rather than a laser. It enables the printer to have a reduced footprint. |
Extrusion of Materials | |
Fabrication of fused filaments (FFF). | Creates an item by depositing numerous layers of heat-softened polymers heated from the filament using a computer-controlled extrusion nozzle. |
Material Swirling | |
Polyjet | Uses an inkjet head those travels in the x and y axes to deposit photopolymer using inkjet technology. After each layer has dried, further layers are printed on top of it and fused. Although products have a high resolution, they could not be as strong as other Techniques.6-19 |
3D Printing Software
The Development of rapid prototyping technology within the medical domain became feasible through the advancements in medical imaging techniques, image processing and reconstruction. Irrespective of the specific technology employed, the 3D Printing process typically involves standardized steps: 1) generating a Computer-Assisted Design (CAD) file, 2) converting it into a .stl file (uploaded to the printer equipment), 3) configuring the printer and finally, 4) producing the object. This manufacturing process is viewed as a comprehensive, fully computer-controlled procedure, eliminating intermediate production stages and substantial manual labor. This streamlined approach is a significant advantage, contributing to reduced time and cost in development and production, as any desired modifications to the final object can be implemented by adjusting the initial CAD file.7
Regulatory Considerations in the USA, Canada and Singapore
At present, 3D Printing is typically subject to the same regulations as medical devices in many nations. Nevertheless, being a novel and potentially transformative healthcare technology, 3D Printing introduces a variety of technical, legal and ethical complexities for the existing regulatory systems. This is because the traditional methods of verifying mechanical functionality may not be feasible or viable for personalized 3D-printed devices utilized in precision medicine.12–20
USA
Acknowledging the extensive array of 3D Printing applications, the FDA categorizes such as pharmaceuticals, biologics, or devices for medical use. The FDA evaluated over 100 3D printing devices that were available on the market by December 2017.6–21
The FDA initially issued guidance for 3D-printed medical devices in 2017, recognizing the specific design, production and testing needs involved. This guidance does not encompass bioprinting. The document addresses technical aspects related to quality systems, based on regulatory classifications and relevant guidelines applicable to the device, along with manufacturing considerations and the information necessary for regulatory notifications and submissions.22 Its purpose is to complement, rather than replace, other applicable regulatory directives for medical devices. The FDA also indicated that this guidance would adapt as our understanding advances regarding factors like unconventional manufacturing locations, supply chains, the use of biological printing materials and considerations for point-of- care devices.6–23
Role of FDA in 3D Printing
The Centre for Devices and Radiological Health (CDRH) at the FDA supervises companies involved in manufacturing, repackaging, relabelling, or importing medical devices for sale in the United States. In 2016, the FDA issued “Technical Considerations for Additive Manufactured Devices,” offering guidelines to manufacturers on producing devices utilizing 3D Printing techniques. Currently, the FDA is in the process of evaluating submissions for new 3D-printed medical devices to ensure their effectiveness and safety under regulation.24 This guidance is separated into two sections:
Design and Manufacturing Considerations fulfil the Quality System (QS) prerequisites for A device. These are discussed as part of meeting the Quality System requirements, which are determined by the device’s regulatory classification. Although the guidance includes manufacturing considerations, it is not meant to comprehensively cover all considerations or regulatory mandates necessary for establishing a quality system for device manufacturing.22–24
Device Testing Considerations outline the specific material requirements for 3D printed devices in various applications, including Humanitarian Device Exemption (HDE) submissions, de novo requests, Investigational Device Exemption (IDE) applications, premarket notification submissions (510(k)) and Premarket Approval (PMA) applications.7–24
Medical devices classification as per USFDA
The FDA has categorized around 17,000 distinct generic types of 3D printed devices, grouping them into 16 medical specialties or panels, as shown in Figure 2. These 17,000 generic types were each assigned to one of three regulatory classes based on the necessary level of control to guarantee their safety and effectiveness.25
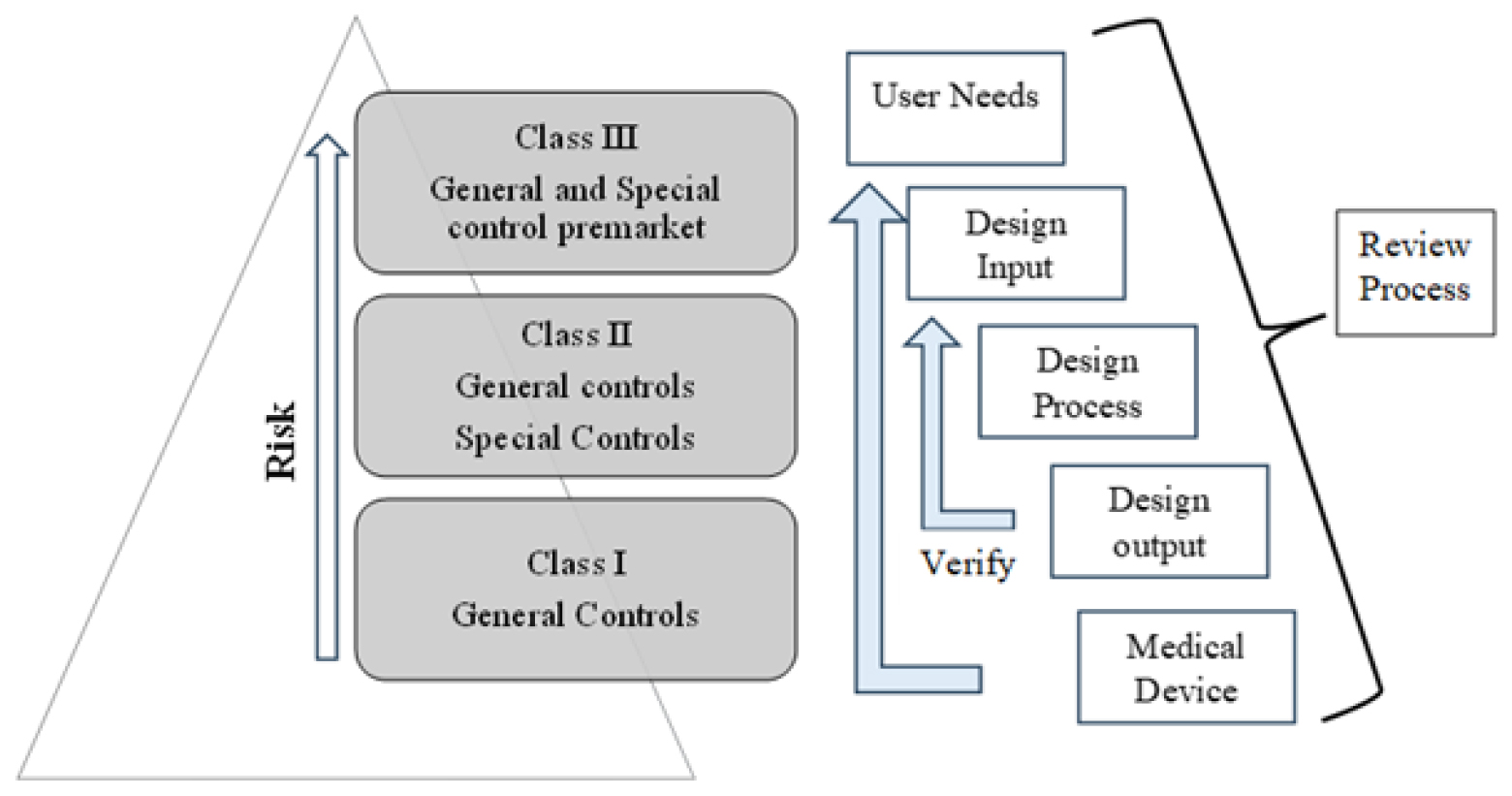
Figure 2:
Medical devices classification as per USFDA.
Regulatory Requirements for 3D Printed Medical Devices
It guides the type of information needed in;
Pre-market Notification submissions [510(k)];
Pre-market Approval (PMA) applications;
Humanitarian Device Exemption (HDE) applications;
De novo application and;
Investigational Device Exemption (IDE) applications for a 3D printed device.4
Methods for 3D Printing Medical Devices
Device Design
A design is generated and verified by employing digital models of predetermined dimensions or by utilizing digital models tailored to an individual’s specific anatomy.
Software Workflow
The digital design of the device is transformed into a printable file, which is then transmitted to the printer. This file typically segments the design into layers, incorporates support materials for better printing and instructs the printer on where to create the device on the printing platform, readying it for the printing process. Additionally, the 3D printer may necessitate some adjustments in preparation for producing diverse designs, involving settings for the material, design type and intended application.23
Control of Materials
For the production of consistently high-quality devices using 3D printing, it is essential to utilize high-quality materials that adhere to uniform specifications. To ensure this, procedures, requirements and agreements called material controls, which need verification for each material batch, are defined among suppliers, buyers and material end-users.
Printing
The item is produced according to the design conditions provided in the document.
Afterward Processing
Once the printing process is finished, additional procedures may be carried out on the device or component, which can involve tasks like clearing away any remaining debris, controlled cooling, also known as annealing and possibly other actions like piercing, hurtful, smoothing and decontamination.
Method Assessment and Confirmation
After the production, it is possible to individually inspect certain device or component attributes to ensure they function correctly and conform to standards. This is particularly relevant for geometric characteristics, which can be swiftly and non-invasively examined. When it is not feasible to individually test other functional features, like mechanical strength, either due to the risk of destroying the item during testing or due to impracticality, manufacturers confirm the effectiveness of their procedures before beginning production. Process validation guarantees that a manufacturing process will yield products that meet specific standards, provided that designated processing parameters are supervised and regulated.
Testing
Testing procedures and their outcomes are presented to the FDA to demonstrate that the device complies with regulatory prerequisites and is reasonably safe and efficient for its intended purpose. Each device or category of device is associated with its specific tests, which can be derived from FDA Guidance documents, global standards, or internal quality controls. Devices created through 3D Printing typically adhere to the same regulatory standards as conventional medical devices.
Elements of 3D-Printed Devices
The FDA usually grants clearance or approval for final medical devices, rather than endorsing individual materials for broad application in the production of medical devices, including those used in creating 3D printed devices, As shown in Table 4. For instance, the FDA has granted approval for spinal implants crafted from titanium alloy, but the FDA doesn’t assess or grant a universal endorsement for the use of titanium in medical devices. The materials employed in developing medical products are assessed in the context of the FDA’s evaluation of the safety and efficacy of the medical product for its intended purpose.23
Sl. No. | Name | Year of approval |
---|---|---|
1 | DENTCA Receives FDA approval for world’s first material for 3D printed Denture. | Aug 10, 2015 |
2 | GRiTT 3D creating custom 3D Printed Sports mouthguards. | Feb 23, 2015 |
3 | OssDsign AB | OssDsign AB |
4 | E-Denture | Jul 4, 2017 |
The FDA assesses a material in the context of the final device and its designated purpose. It examines whether the intended use and technological attributes of the device, including the materials used, are reasonably safe and effective or essentially comparable to the safety and efficacy of a legally marketed device. If this is the case, the FDA grants approval or clearance for specific devices and their intended uses. However, this does not extend to granting approval or clearance for the manufacturer to employ the same materials in other devices.
Devices that incorporate novel materials may not automatically necessitate the FDA’s more stringent premarket review procedure, referred to as the PMA review. In reality, devices featuring new materials can be granted clearance via the 510(k) premarket notification process, as long as the use of the new material does not introduce unique concerns regarding safety or efficacy. The submission must also establish that the new material is, at the very least, as safe and effective as those found in a comparable legally marketed device.
Materials Used in Printed Dental Devices
The FDA grants authorization for specific specially engineered materials intended for use in dental equipment, including those produced through 3D printing techniques. These materials are approved for particular purposes as if they were fully developed devices. These specific materials are considered ready for use by healthcare professionals and are designed to match individual patients or are customized during care. Examples encompass dental restorative and prosthetic tools like direct filling resins, dental cement, denture resins, orthodontic retainers, night guards, crowns, bridges, inlays and onlays. When approving these devices, the FDA typically mandates testing the material’s performance in its final form to confirm that it possesses the necessary physical and material attributes for its intended use.4–23 It’s crucial to understand that the FDA has only approved these materials for particular designated purposes, such as “to create a denture base” or “to repair structural defects in teeth.” The FDA doesn’t grant clearance for materials to be used in an unrestricted range of intended uses. Each engineered material is cleared for the production of a specific device, with distinct physical characteristics and for a particular indication of use. For instance, a device material approved for a particular intended use like “tooth shade resin material” doesn’t automatically have FDA clearance for any other application, such as “Endosseous dental implant abutment.”23
CANADA
In Canada, the Medical Devices Regulations apply to medical devices manufactured using 3-D Printing (3-DP). Health Canada initiated the development of guidance in August 2018 for manufacturers seeking licenses for 3-DP medical devices.26 A preliminary guidance document was made available for public comment in October 2018, with the final guidance anticipated in spring 2019. Some stakeholder groups have publicly shared feedback on the issued guidance. The guidance specifically targets manufacturers, including hospitals producing 3-DP devices for external distribution, of Class III and Class IV implantable medical devices.27 It serves as a supplementary resource to the existing evidence requirements for all Class III and Class IV devices. According to the latest draft guidance from Health Canada, the agency has not provided specific instructions regarding the use of certain types of medical devices that are unique or tailored to individual patients. These include third-party software, custom-designed devices, patient-specific anatomical models, devices created at the point of care and those incorporating biological elements.28 While this draft guidance represents an initial stance on 3-dP policies in Canada, it is expected to develop alongside advancements in technology over time.29
“Evidence to Support Inserted Medical Devices Made with 3D Printing” This paper provides guidelines to help producers and regulatory bodies prepare licensing applications for medical equipment made using 3D printing technology.30 The draft guidance from Health Canada emphasizes that the production of 3D printed devices poses unique considerations for manufacturers. In addition to the standard data required for the approval of all Class III and Class IV medical devices, manufacturers of 3D printed medical devices may need to provide extra information for approval.29
This includes:
Specification of starting materials, additives and the 3D printed technique used in production.
A statement indicating whether or not the gadget is fully 3D printed.
Inclusion of a design philosophy that explains the rationale for choosing 3D printing as the manufacturing approach.
Maintenance and cleaning records for printers, validation of consistent performance, accuracy in reproducing patient-specific images and validation of printer-material combinations.
Validation of processes for the removal and potential reuse or recycling of layering materials.
Requirement for verification and validation of software used in design and printing.
Biocompatibility testing on finished 3-DP devices.
Validation of processes for post-processing, including the removal of residues and excess layering material, as well as the sterilization of 3-DP devices. This should demonstrate the minimization of bioburden and consideration of how sterilization may impact the final product.30
Licence Applications for Class III and IV 3D printed insertable device
Device description
A detailed depiction of the finalized device is necessary, accompanied by a photograph or illustration clearly labelling all efficient apparatuses. It should be made clear in the gadget’s specification if a specific element or the complete device is made via 3D printing. Moreover, the device specification in the licensing application for 3D printed devices should include information regarding the beginning material.31 This information should encompass:
Reference to pertinent materials values.
The initial material’s chemical makeup, such as with any applicable certifications for testing.
Processing ingredients or additives used in the raw material should be identified.
A brief explanation of the 3D printing technique used (which might include lasers melting, straight metal lasers melting and powdered bed fusing) should also be included in the license application. This should be complemented by an overview of the printing process, incorporating post-printing processing steps. The overview of the manufacturing process should also identify quality control procedures.31
Design ideology
In the case of 3D printed gadgets (i.e., patient-matched gadgets, gadgets with complicated shapes and/or unusual sizes), the creative approach may involve a debate and/or the decision to employ the use of 3D printing as a manufacturing technique.
Licence amendments
A license amendment application becomes necessary when there is a reasonable expectation that a substantial alteration will impact the security and efficacy of a finalized gadget. The standards for non-3D printed items can be utilized to determine what constitutes a major change in a 3D printed device. The Guidance for the Interpretation of Major Change of a Medical Device provides further information on Health Canada’s definition of major changes in a medical device.31
Examples of alterations associated with 3D Printing may encompass, for instance, but are not restricted to:
Adding a 3D element printed to improve one that was already authorized (such as adding a porous surface layer).
Combining a 3D printed component with an already-existing, suitable part (such as implants for teeth, attachments, or structures).
Modifications to the material or its requirements.
Modifications to afterward procedures (such as treatment of the surface and heat treatments) that may affect the finished device.
Modifications to the material-printer setup.
Software-related modifications that may influence the final device.
Safety and Effectiveness
Pre-clinical studies
The most recent, completely produced device, comprising all post-printing operations, sanitation and sterilizing activities, must undergo pre-clinical performance assessments. Each assessment must have an in-depth report that includes details regarding the test’s aim, the samples examined, an assessment of the experiment’s specimens’ worst-case setup based on measurements and includes (like gaps, encourages and permeable areas), acceptance requirements and their rationale, adherence to international regulations, test findings and an examination or analysis of the findings concerning the test goal. For 3D printed devices, the analysis of the worst-case device configuration for preclinical testing may involve referencing or summarizing:
Verification is connected to the process that takes into account the location, size and orientation of the device within the printer build space.
Procedure-related examinations including several build cycles to address printing process uniformity and repeatability.
Verification of powder reprocessing while considering possible impacts on melting characteristics and layer bridging.
Shelf life
Further considerations regarding the shelf life of 3D printed devices may involve:
Impact on the extensive stability of materials, particularly concerning between layers bonding and uniformity across build layers.
Possible periodic modifications to the physiology of the patient for devices made specifically for each patient.
Shelf-life considerations for raw materials, where applicable.
Program verification and approval
A description, maybe in the way of a diagram, of the software-based workflows specific to patients’ gadgets should be included in the licensing request. This should include the steps from the collecting of medical images to segmentation and design modifications for the final print creation. It is recommended that producers offer the medical professional in charge of taking pictures explicit instructions about the specifics of the picture collection. Before moving on to the next steps of the device design and printing process, the manufacturer should confirm that these variables are appropriate after obtaining patient photos.
Biocompatibility tests
In the case of 3D printed devices, relying on bio-compatible evidence from a beforehand approved (non-3D printed) device is typically deemed inadequate to substantiate the biocompatibility of 3D printed devices, given the variations in the manufacturing processes. Biocompatibility testing must be performed on the device representing the worst-case scenario. The evaluation of the worst-case scenario for biocompatibility should also take into account the potential presence of residual support material.
Animal studies
Animal studies are commonly employed to evidence of the safety and efficacy of interventions in humans. When dealing with a 3D printed device featuring a unique design, material, or intended purpose, it may be necessary to conduct animal studies. For instance, these studies could be utilized to substantiate claims of effectiveness concerning improved bony in growth in a porous structure or coating produced through 3D printing.
Clinical studies
For a 3D printed item with a unique design, material, or intended purpose, item-specific clinical information can be needed. When evaluating the safety and efficacy of a 3D printed device, the clinical performance of a comparable non-3D printed device may be used in the absence of lengthy clinical data for that particular device. The licensing proposal is examined separately to determine whether further, device-specific clinical evidence is required.31
SINGAPORE
In Singapore, the Health Sciences Authority (HSA) oversees the regulation of 3D-printed devices within the existing regulatory structure designed for various medical devices categorized into four risk classes. Nevertheless, amid the COVID-19 pandemic, a concise set of guidelines concerning the 3D printing of vital medical devices has been released online. This guidance is directed towards organizations contemplating the local 3D Printing of essential devices as a response to heightened demand and disruptions in the global supply chains.32
Regulatory controls are adjusted according to the assigned risk classification of a medical device. Like other medical devices, 3D-printed devices are also categorized into four risk classes based on an internationally recognized risk classification system. The risk class is determined by factors such as the device’s nature, invasiveness, duration of contact and intended purposes. Class A 3D-printed medical devices encompass external prosthetics, face shields and nasopharyngeal swabs. Examples of higher-risk Class B 3D-printed medical devices include ventilator tubes and connectors, while Class C involves dental or orthopaedic implants and Class D includes cranial implants and heart valves.33
Current Development in Singapore
The use of 3D printing in medical applications is a growing area globally and it’s likely that Singapore has continued to explore and implement such technologies, As shown in Table 5.
Stage of diffusion | Clinical applications |
---|---|
Investigational | Custom implants. |
Newly entered | 3D-printed devices for COVID-19, anatomical models, surgical guide. |
Nearly established | – |
Established | – |
As per the Economic Development Board, Singapore is actively working towards establishing a significant research and development hub for biomedical innovations. Recognizing the transformative potential of 3D printing technology in manufacturing processes, the country has initiated efforts to harness its benefits. In 2015, the National Combinatorial Production Innovation Cluster (NAMIC) was established to provide infrastructure support, promote collaboration and expedite the application and adoption of 3D printing. Under this overarching initiative, 3D Printing in medical technologies has been identified as a key and growing area within recent Innovation Cluster programs. NAMIC consists of three combinatorial production translation and capability development research hubs, with the National University of Singapore’s Centre for Combinatorial Production serving as the primary centre. The focus of this centre is to introduce innovative products to the healthcare sector, aiming to enhance patient outcomes through groundbreaking 3D Printing technology tailored for personalized patient treatments. Collaborating with multidisciplinary expertise from the National University of Singapore and clinicians from the National University Hospital, the Centre specializes in five main areas of expertise.32
Surgical Instruments, Simulation and Prosthetics;
Restorative Repair and Implants;
3D Bio-printing for Tissue Repair;
AM Enabled Medicine; and
Oral Health and Craniofacial Applications.
The Health Sciences Authority (HSA) has released Regulatory guidelines for 3D-printed medical devices this guideline offers clarification on Three-Dimensional (3D)-printed medical devices, along with the regulatory approach and requirements governing these devices. It is important to note that this guideline reflects HSA’s current perspective, existing policies and established practices. It should not be interpreted as the introduction of new regulatory controls for these medical devices.32
Types of medical devices commonly manufactured using 3D printing:
Non-invasive prosthetics (such as prosthetic limbs)
Dental devices,
Surgical tools and guides,
Implantable devices (such as orthopaedic implants).
Based on the risk categorization given to the device, medical devices developed using 3D printing technology comply with the present regulatory framework and regulations that belongs to those manufactured using conventional manufacturing methods. The Essential Principles of Safety and Performance, as described in GN-16: Guidance on Essential Principles for Safety and Performance of Medical Devices must be followed by 3D-printed medical devices. Despite its manufacturing process, regulatory controls are based on the intended application/indications for use and technological features, working within the current risk-based framework for medical devices.
Prospects and challenges of 3D Printed Medical device
Prospects of 3D Printed Medical device
There is great potential in tapping the AM Technology, namely.19
Localization of production
The design of the product or device may be modified to better suit the needs and preferences of local users.”
Democratization of 3D printing
As 3D Printing technology becomes more widely available and mainstream, it is likely that 3D printers, components, attachments, materials and supplies will become more accessible to the general public, much like traditional office and household items such as printers.
Advancement of 3D Printing technology
Numerous research groups are actively investigating 3D Printing technology and materials, coupled with the introduction of new scientific journals dedicated to 3D printing. This collective effort indicates an undeniable trajectory toward the rapid advancement of the technology, making it applicable to a myriad of practical uses. Furthermore, as the patents of the original inventors of 3D Printing technologies expire, innovation is accelerating, benefiting researchers worldwide. Intense competition among various manufacturers typically results in more affordable products, suggesting the likelihood of more economical, sophisticated and user-friendly 3D printers in the future.
Challenges of 3D Printed Medical device
Legal/patent issues
With 3D Printing technology, individuals or organizations can reproduce or violate the exclusive rights held by other companies over their original designs without proper authorization. While this may result in legal disputes and consequences later on, there are instances where such actions might be seen as justifiable in times of urgent need.
Ethics
In the testing process for medical products, human participation is typically required, which means that informed consent must be obtained from individuals involved in these tests.
Safety
When it comes to using materials for long-term, invasive medical procedures, questions about their biocompatibility-or how well they interact with the body without causing adverse effects frequently arise. However, another crucial concern is the sterilization process, particularly when these devices are reused multiple times. Ensuring that the materials used can be properly sterilized and remain free from contamination is essential for patient safety and the overall success of the procedure.
Materials testing
There are particular guidelines for the raw materials, manufacturing processes and quality control tests required for 3D-printed components. While the FDA acts as the ultimate authority, these standards ensure greater dependability over extended periods (ISO and ASTM).
Cost
Currently, the cost of producing goods using 3D printers remains relatively high per unit. However, this technology may become more competitive when scaled up for large-scale manufacturing operations or when using cheaper raw materials such as pellets rather than filaments, as shown in Figure 3.
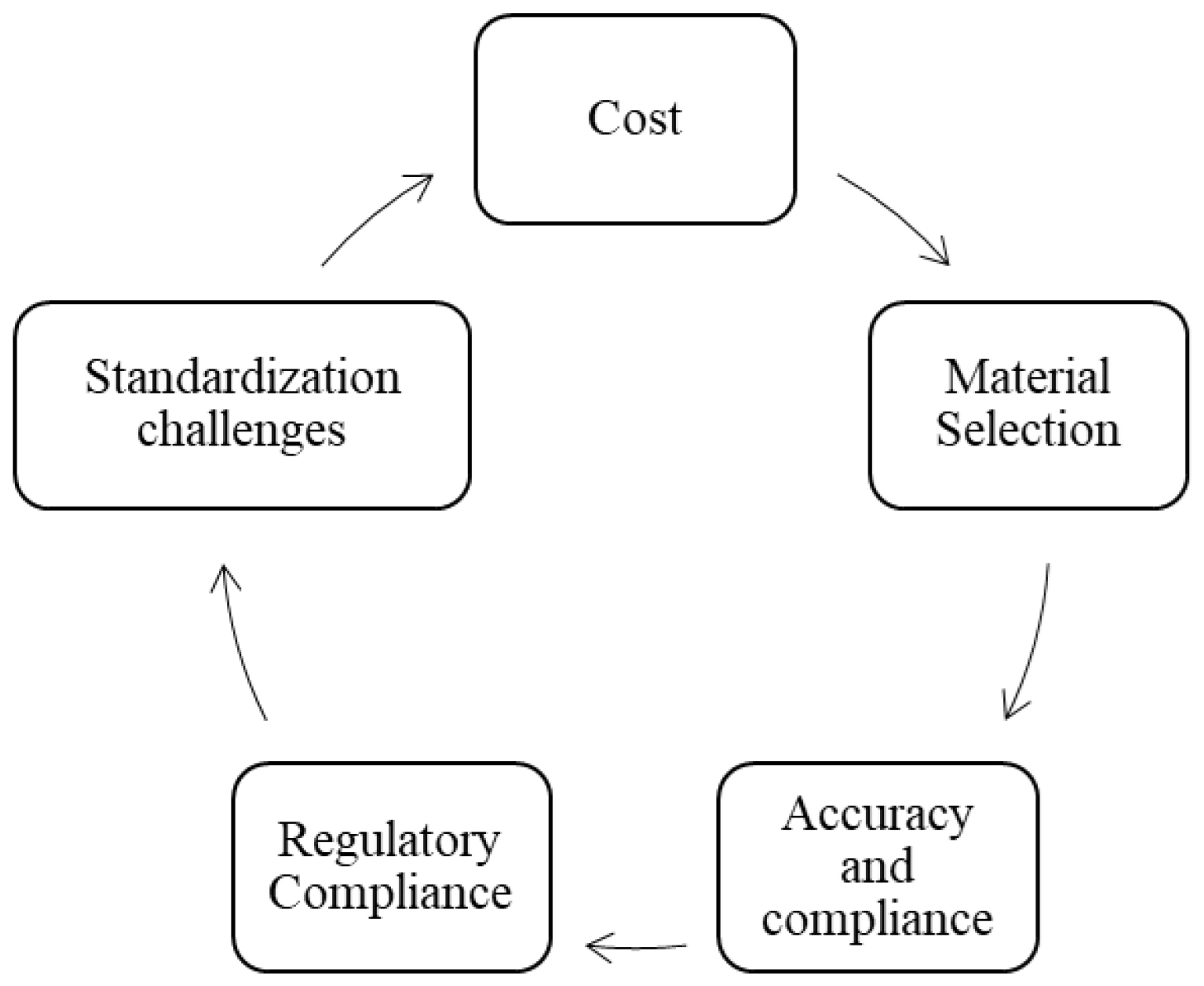
Figure 3:
Challenges of 3D Printing in Medical Sectors.33
Materials durability/robustness
The inherent properties of 3D-printed materials, such as strength and durability, are often influenced by their layered structure, which is characteristic of 3D Printing techniques. Furthermore, the numerous variables that can be adjusted during the 3D Printing process, including temperature, pressure and material composition, can significantly impact the final mechanical performance and overall characteristics of the printed part.
Future directions of 3D printed medical devices
Nowadays, 3D Printing is generally thought of as an innovative and flexible manufacturing technique because of its huge potential to manufacture implants with a wide range of complex structures. Moving forward, despite the difficulties we are currently confronting, additional advances can be made to improve implants’ therapeutic effectiveness and the advantages they provide both patients and society.28 Sometimes the resolution of the clinic image dataset used for 3D Printing or reconstruction is insufficient, which makes it difficult to meet the minimum criteria for further processing. To improve the amount and quality of the raw image datasets, the proper data augmentation algorithm can be used. Deep learning models have been developed with Convolutional Neural Networks (CNNs) and Generative Adversarial Networks (GANs).34 Improving printing configurations for 3D Printing is still a difficulty; however, it is growing increasingly crucial in customized orthopaedic implant applications. Here, Artificial Neural Networks (ANNs) and Machine Learning (ML) can be used to identify intricate designs and determine the connection between process parameters and the end-product attributes of the printed component.35 It has been effectively employed to reduce surface roughness with the most effective 3D printing process parameters by combining ANN models and swarm optimization particle methods.28–36
While the USA, Canada and Singapore have similar requirements regarding quality management systems and risk management for 3D printed medical devices, differences exist in the regulatory pathways, approval processes and specific standards recognized and as differences in the specific requirements and processes for obtaining regulatory approval, as shown in Table 6.
Parameters | United States | Canada | Singapore |
---|---|---|---|
Regulatory Authority | USFDA (CDER and CDRH). | Health Canada | Health Science Authority. |
Classification | Class III | Class III and Class IV | Class II, III and IV |
Guideline for 3 Printing medical device. | Technical Considerations for Additive Manufactured Medical Devices. | Supporting Evidence for Implantable Medical Devices Manufactured by 3D Printing Licensing Requirements for Implantable Medical Devices Manufactured by 3D Printing. | Regulatory guideline for 3D-printed medical devices. GN-16 Guidance on Essential Principles for safety and performance of medical device. |
Regulatory Requirements. | FDA 21 CFR 820, Premarket Approval (PMA), humanitarian device exemption (HDE) applications, De Novo requests and investigational device exemption (IDE), pre-market notification (510(k). | Medical Device Establishment License, Additive manufacturing file (AMF) Format, Pre-market license Application. | Singapore Medical Device Register (SMDR), Product registration license Application. |
Approval timeline | Depending on the classification of the device, the approval process can be gathered from several months to years. | Based on risk assessment, approval times may also differ, however they are generally shorter than in the USA. | Although they may vary, approval processes are frequently quick in comparison to larger regulatory bodies. |
Biocompatibility | Required (the data must be included in 510K or PMA). | Required for worst-case device (the data must be included in the medical device license application). | Required (the data must be included in the Product registration license). |
Database | Manufacturer and User Facility Device Experience (MAUDE). | Medical Devices Active Licence Listing (MDALL). | Singapore Medical Device Register (SMDR). |
Design Input | A patient-matched device designed from the patient’s medical image. | Patient anatomy and adaptable medical devices are essential components in patient-matched AM devices. | Custom-made medical devices and Patient-matched medical devices. |
Functional Requirements | Operational qualification, Installation Qualification. | Operation Qualification (OQ). | Operation Qualification. |
Performance Requirements | Additive Manufacturing and Hot Isotactic Process. | 1) accepted imaging techniques; file different types; patient implantation or gating requirements; scanning settings; and image reconstruction parameters. Laser sintering, direct metal laser sintering and powder bed fusion. | Depending on the additive manufacturing and post-manufacturing processes. laser sintering, direct metal laser sintering and stereolithography. |
Design Process | Standard design | Devices with complex geometry and/or non-standard sizing Medical image acquisition to segmentation and design manipulation. | Depending on the additive manufacturing and post-manufacturing processes. |
Design output | Development and documentation. | Not available | Not available |
Verification | Non-destructive evaluations. | Destructive Evaluation. | Destructive Evaluation. |
Validation | Process validation. | Cleaning and sterilization processes. | Depending on the device type it may include: tensile strength, fatigue, abrasive wear, etc. |
Bioprinting law regulatory perspective. | IP, regulation of distribution, premarket restrictions, control mechanism | as per the requirements of ISO 10993-1:2009 | Not Applicable |
Quality Standards | Compliance with FDA’s Quality System Regulation (QSR). | The Canadian Medical Devices Regulations, which include ISO 13485 certification, must be followed by manufacturers. | In besides complying with the Good Distribution Practice for Medical Devices, ISO 13485 certification is commonly required. |
Certification | Good Manufacturing Practices (GMP), ISO 13485, ISO 14971. | ISO 10993, ISO 13485. | ISO 10993 |
Labelling Information | Device identification, the intended use, the material used and any special handling instructions. Developed by consensus standards, device-specific guidance materials and applicable regulations. | Determine or monitor the device, the name and address of the manufacturer, the manufacturing date and the parts of the material that were used. | The intended use, special handling instructions, device identification, name and address of the manufacturer and manufacture date. |
Availability and Adoption | Although 3D-printed medical devices are appropriately accessible acceptance may vary based on the specific product and the area of the United States. | According to the region and type of healthcare facility, 3D-printed medical devices may not be widely available or adopted in Canada. | Proactively promoting the application of 3D printing in healthcare to increase the accessibility of such instruments. |
CONCLUSION
Currently, Medical devices manufactured using the 3D method are subject to the same regulations as those produced using traditional manufacturing techniques. Utilizing CT and MRI, a 3D image of bones can be reconstructed and transformed into a bone prototype layer by layer, proving effective in the medical field. The global market for 3D printing medical devices is anticipated to grow from US$0.84 billion in 2017 to US$1.88 billion by 2022, with a compound annual growth rate (CAGR) of 17.5%. While the potential of 3D printing in developing personalized drug products is evident, it is crucial to diversify machines for appropriate pharmaceutical applications. Successful production methods require collaboration between the pharmaceutical industry (for large-scale filament production) and digital pharmacies (for printing medicine based on patient-specific prescriptions). To ensure the adoption of Different 3D printing techniques for medical product fabrication, it is essential to implement appropriate regulatory guidelines, although some guidelines have been established by regulatory bodies such as the US FDA, Canada and Singapore.
Cite this article:
Bhise MG, Patel L, Patel K. 3D Printed Medical Devices: Regulatory Standards and Technological Advancements in the USA, Canada and Singapore-A Cross-National Study. Int. J. Pharm. Investigation. 2024;14(3):888-902.
ACKNOWLEDGEMENT
The authors are grateful to the Parul Institute of Pharmacy, Parul University, Vadodara, Gujarat, India for providing guidance and support for this Article.
ABBREVIATIONS
USA | United State America |
---|---|
FDA | Food and Drug Administration |
3D | Three Dimensional |
UV | Ultra Violet |
CAD | Computer-Aided Design |
MDCT | Multi-Detector Computer Tomography |
CT | Computer Tomography |
MRI | Magnetic Resonance Images |
STL | Standard Triangulation Language |
DICOM | Digital Imaging And Communication In Medicine |
CDRH | Centre For Devices And Radiological Health |
SLS | Laser Sintering Selectivity |
SLM | Selective Laser Melting |
SHS | Heat Sintering Selection |
FFF | Fabrication Of Fused Filaments |
HDE | Humanitarian Device Exemption |
PMA | Premarket Approval |
IDE | Investigational Device Exemption |
HAS | Health Sciences Authority |
NAMIC | National Combinatorial Production Innovation Cluster |
ASTM | American Society For Testing And Materials |
ISO | International Standard Organization |
GAN | Generative Adversarial Networks |
ANN | Artificial Neural Networks |
MDALL | Medical Devices Active Licence Listing |
MAUDE | Manufacturer And User Facility Device Experience |
SMDR | Singapore Medical Device Register |
References
- Javaid M, Haleem A. Additive manufacturing applications in orthopaedics: a review. J Clin Orthop Trauma. 2018;9(3):202-6. [PubMed] | [CrossRef] | [Google Scholar]
- Zhou H, Bhaduri SB. Biomaterials in translational medicine. 2019:269-89. [PubMed] | [CrossRef] | [Google Scholar]
- Ace overview for new and emerging health. Technologies 3D Printing and Its Clinical Applications. 2020 [PubMed] | [CrossRef] | [Google Scholar]
- Prasanthi Nori L, Manikiran SS. An outlook on regulatory aspects of 3D printing in pharmaceutical and medical sectors. Curr Trends Pharm Pharm Chem. 2022;4(3):98-108. [CrossRef] | [Google Scholar]
- Durfee WK, Iaizzo PA. Engineering in medicine: advances and challenges. 2018:527-43. [CrossRef] | [Google Scholar]
- Ford C, Dunfield L, Magega I, Globerman K, Carver T, Proctor G, et al. An overview of clinical applications of 3-D printing and bioprinting. 2019 [CrossRef] | [Google Scholar]
- Madla CM, Trenfield SJ, Goyanes A, Gaisford S, Basit AW. 3D printing technologies, implementation and regulation: an overview. AAPS Adv Pharm Sci S. 2018:21-40. [CrossRef] | [Google Scholar]
- Jin Z, He C, Fu J, Han Q, He Y. Balancing the customization and standardization: exploration and layout surrounding the regulation of the growing field of 3D-printed medical devices in China. Biodes Manuf. 2022;5(3):580-606. [PubMed] | [CrossRef] | [Google Scholar]
- Horst A, McDonald F. Uncertain but not unregulated: medical product regulation in the light of three-dimensional printed medical products. 3D Print Addit Manuf. 2020;7(5):248-57. [PubMed] | [CrossRef] | [Google Scholar]
- Choonara YE, Du Toit LC, Kumar P, Kondiah PP, Pillay V. 3D-printing and the effect on medical costs: A new era?. Expert Rev Pharmacoecon Outcomes Res. 2016;16(1):23-32. [PubMed] | [CrossRef] | [Google Scholar]
- Schuh JC, Funk KA. Compilation of international standards and regulatory guidance documents for evaluation of biomaterials, medical devices and 3-D printed and regenerative medicine products. Toxicol Pathol. 2019;47(3):344-57. [PubMed] | [CrossRef] | [Google Scholar]
- [cited Jan 04 2019];The 3 Rs of 3D printing: FDA’s role. Silver Spring, (MD): U.S. Food and Drug Administration. 2016 Available fromhttps://www.fda.gov/ForCo nsumers/ConsumerUpdates/ucm533992.htm
[PubMed] | [CrossRef] | [Google Scholar] - Zema L, Melocchi A, Maroni A, Gazzaniga A. Three-dimensional printing of medicinal products and the challenge of personalized therapy. J Pharm Sci. 2017;106(7):1697-705. [PubMed] | [CrossRef] | [Google Scholar]
- Vaishya R, Vijay V, Krishnan M, Agarwal AK. Fallacies of CT based component size prediction in total knee arthroplasty – Are patient specific instruments the answer?. J Clin Orthop Trauma. 2018;9(1):34-9. [PubMed] | [CrossRef] | [Google Scholar]
- Srivastava A, Aggarwal AN, Mishra P, Bhateja D. Femoral fracture acting as an “ominous masquerade” in a 7-year-old child. J Clin Orthop Trauma. 2016;7(Suppl 1):27-9. [PubMed] | [CrossRef] | [Google Scholar]
- Jagadeesh V. Medical applications of 3D printing. Pharma Times. 2018;50(5):9-17. [PubMed] | [CrossRef] | [Google Scholar]
- Vinck I, Vijverman Dewallens A, Vollebregt E, Broeckx Dewallens N, Wouters Dewallens K, Piёt M, et al. Available fromhttp://www.kce.fgov.be
Responsible Use of High-Risk Medical Devices: the Example of 3D Printed Medical Devices [Internet]. - ECRI Institute. 3-D printing in medicine: an introduction. Health Devices. 2018 [PubMed] | [CrossRef] | [Google Scholar]
- Advincula RC, Dizon JR, Chen Q, Niu I, Chung J, Kilpatrick L, et al. Additive manufacturing for COVID-19: devices, materials, prospects and challenges. MRS Commun. 2020;10(3):413-27. [PubMed] | [CrossRef] | [Google Scholar]
- Adamo JE, Grayson WL, Hatcher H, Brown JS, Thomas A, Hollister S, et al, et al. Regulatory interfaces surrounding the growing field of additive manufacturing of medical devices and biologic products. J Clin Transl Sci. 2018;2(5):301-4. [PubMed] | [CrossRef] | [Google Scholar]
- US Food and Drug Administration. [cited Mar 14 2019];Statement by FDA Commissioner Scott Gottlieb, M.D., on FDA ushering in new era of 3D printing of medical products; provides guidance to manufacturers of medical devices. 2017 https://wwwAvailable fromhttp://fda.gov/NewsEvents/Newsroom/PressAnnouncement s/ucm587547.htm
[PubMed] | [CrossRef] | [Google Scholar] - CDRH. Technical Considerations for Additive Manufactured Medical Devices Guidance for Industry and Food and Drug Administration Staff Preface Public Comment [Internet]. Available fromhttp://www.regulations.gov
[PubMed] | [CrossRef] | [Google Scholar] - Center for Devices, Radiological Health. [cited 2023 Dec 9];3D Printing of medical devices [Internet]. U.S. Food and Drug Administration. FDA. 2023 Available fromhttps://www.fda.gov/medical-devices/products-and-medical-procedures/3d-printing-medical-devices
[PubMed] | [CrossRef] | [Google Scholar] - Bhat S, Venkatesh M, Pramod Kumar TM. Comparison of 3D printing in USA, Europe and Australia and IPR. [PubMed] | [CrossRef] | [Google Scholar]
- Rust B, Tsaponina O, Maniruzzaman M. 7 recent innovations in additive manufacturing across industries: 3D printed products and FDA’s perspectives. 2019 [PubMed] | [CrossRef] | [Google Scholar]
- Belhadj B, Kaabi F. Mathématiques cours et exercices corrigés. [PubMed] | [CrossRef] | [Google Scholar]
- Health Canada. Draft guidance document-licensing requirements for implantable medical devices manufactured by 3D printing. 2018 https://www. Available fromhttp://canada.ca/en/health-canada/services/drugs-health-products/medical-devices /activities/announcements/notice-licensing-requirements-implantable-3dprinting/draft-guidance-document.html
- Wu Y, Liu J, Kang L, Tian J, Zhang X, Hu J, et al. An overview of 3D printed metal implants in orthopedic applications: present and future perspectives. Heliyon. 2023;9(7):e17718 [PubMed] | [CrossRef] | [Google Scholar]
- Mulero A. AdvaMed calls for revisions to Health Canada guidance on 3D-printed implantable devices. Reg Focus. 2019:1 [PubMed] | [CrossRef] | [Google Scholar]
- Canada.ca. [cited Dec 9 2023]. Available fromhttps://www.canada.ca/en/health-canada/services/drugs-health-products/medical-devices/activities/announc ements/notice-licensing-requirements-implantable-3d-printing/draft-guidance-document.html
- Available fromhttps://www.canada.ca/en/health-canada/services/drugs-health-products/medical-devices/application-information/guidance-documents/3d-licensing-requirements/document.html
- Ace overview for new and emerging health. Technologies 3D Printing and Its Clinical Applications. 2020 [PubMed] | [CrossRef] | [Google Scholar]
- Mamo HB, Adamiak M, Kunwar A. 3D printed biomedical devices and their applications: a review on state-of-the-art technologies, existing challenges and future perspectives. J Mech Behav Biomed Mater. 2023;143:105930 [PubMed] | [CrossRef] | [Google Scholar]
- Mahmood MA, Visan AI, Ristoscu C, Mihailescu IN. Artificial neural network algorithms for 3D printing. Materials (Basel). 2020;14(1):1-29. [PubMed] | [CrossRef] | [Google Scholar]
- REGULATORY GUIDELINE FOR 3D-PRINTED MEDICAL DEVICES medical devices branch. 2021 [PubMed] | [CrossRef] | [Google Scholar]
- Shirmohammadi M, Goushchi SJ, Keshtiban PM. Optimization of 3D printing process parameters to minimize surface roughness with hybrid artificial neural network model and particle swarm algorithm. Prog Addit Manuf. 2021;6(2):199-215. [CrossRef] | [Google Scholar]