Contents
ABSTRACT
Background
This study investigated the biosynthesis of Copper Oxide Nanoparticles (CuONPs) using an environmentally safe and sustainable approach based on Amaranthus tricolor extract.
Materials and Methods
The synthesized CuONPs were characterized using various analytical techniques, including X-ray Diffraction (XRD), scanning electron microscopy (SEM), and Fourier-Transform Infrared Spectroscopy (FTIR). The antioxidant activity of the CuONPs was assessed using different in vitro assays, including the (2,2-diphenyl-1-picrylhydrazyl) radical scavenging assay and cytotoxicity against MCF7 breast cancer cells.
Results
The presence of several functional groups such as amides, hydroxyl groups, alcohols, and alkenes was verified by Fourier-Transform Infrared (FTIR) analysis. Scanning Electron Microscopy (SEM) was used to analyze the morphology of the CuONPs produced from Amaranthus tricolor, which exhibited spherical CuONPs with distinct cell-spreading patterns. The elemental composition of the CuONPs was validated by X-ray Diffraction (XRD), which revealed typical peaks related to Copper (Cu) and Oxygen (O2). CuONPs demonstrated dose-dependent cytotoxicity against MCF7 breast cancer cells, with Inhibitory Concentration (IC50) values of 84.24 g/mL and 76.85 g/mL, respectively. These findings emphasize the potential of CuONPs as selective anticancer agents.
Conclusion
This work demonstrates the effective synthesis and diverse characteristics of CuONPs obtained from Amaranthus tricolor. These discoveries have broad implications in nanomedicine, antibacterial methods, and antioxidants. Furthermore, this study emphasizes the specific cytotoxicity of CuONPs against cancer cells, stressing their potential in targeted cancer therapy.
INTRODUCTION
Nanotechnology has recently attracted significant interest because of its immense potential to revolutionize various scientific fields, most notably medicine. Nanoparticles, characterized by their unique features, have garnered significant attention for their diverse range of uses, serving as the central focus of this transformative process. The enthusiasm for nanoparticle synthesis has been further enhanced by the emergence of environmentally friendly and sustainable approaches such as phytofabrication. Phytofabrication involves the incorporation of plant extracts into the manufacturing of nanoparticles, which contributes to their synthesis.1,2 Phytofabrication signifies the amalgamation of plant science and nanotechnology. This new methodology utilizes the intrinsic features of bioactive substances found in plant materials, such as leaves, stems, or roots, to effectively assist the production of nanoparticles via reduction and capping mechanisms.3 Bioactive compounds such as polyphenols, flavonoids, alkaloids, and other phytochemicals play a dual role in nanoparticle synthesis. First, they act as reducing agents by converting metal ions into nanoparticles. Second, they serve as capping agents, thereby stabilizing nanoparticles and preventing their agglomeration.4 Phytofabrication has many advantages, including a wide range of benefits. Primarily, this methodology exhibits ecological compatibility by circumventing the use of hazardous chemicals and energy-intensive procedures often associated with conventional methods of nanoparticle manufacturing.5 The decrease in the presence of dangerous substances not only mitigates environmental contamination but also contributes to the improved safety of researchers and the surrounding ecosystem. Furthermore, the incorporation of botanical extracts facilitates the advancement of sustainable practices, given that plants possess the inherent potential to regenerate, guaranteeing a steady supply of essential constituents for the synthesis of nanoparticles.6 Furthermore, this sustainable strategy is in accordance with the worldwide trend towards green and circular economies, making a valuable contribution to the responsible management of resources. In addition, phytofabrication is often cost-effective compared with conventional synthesis methods, making it more readily available for a broader spectrum of research and industrial implementation. Finally, it has been shown that these plant-mediated nanoparticles exhibit biocompatibility and safety characteristics, rendering them appropriate for use in biomedical contexts, thereby mitigating concerns pertaining to their potential toxicity. Amaranthus is a genus in the Amaranthaceae family that is significant in several ways. The plant family in question has a diverse array of species, among which, Amaranthus tricolor stands out because of its notable phytochemical makeup. Amaranth species have been deliberately developed and used as highly nutritious green vegetables, with some types yielding grains that are abundant in critical nutrients. In addition to its primary function as a dietary staple, Amaranthus has a rich historical background including several cultural traditions that have been used for medical purposes. The leaves of this plant are often acknowledged for their substantial concentration of bioactive components, including polyphenols, flavonoids, alkaloids, and vitamins, which significantly contribute to its medicinal efficacy.7–9 The field of phytochemistry encompasses the study of plant-derived chemical compounds, with a particular focus on their medicinal properties and potential therapeutic applications. The therapeutic importance of amaranthus can be attributed to its phytochemical makeup. The bioactive chemicals found in Amaranthus have been shown to possess antioxidant, anti-inflammatory, antibacterial, and antidiabetic activities, thus establishing it as a useful asset in traditional medicinal practices.10 Numerous studies have demonstrated the potential of Amaranthus extracts in the management of diseases associated with oxidative stress, including cardiovascular disorders and diabetes. This potential was attributed to the ability of the extract to scavenge free radicals and mitigate inflammation. Furthermore, amaranth leaves have been investigated for their potential as natural anti-cancer agents and their ability to promote wound healing, highlighting their diverse contributions to the field of healthcare.11–13
Copper Oxide Nanoparticles (CuO NPs) have become increasingly important in the field of healthcare because of their distinctive physicochemical characteristics. Nanoparticles have been shown to produce Reactive Oxygen Species (ROS), including superoxide radicals and hydrogen peroxide, which can cause oxidative stress in certain cells, with special emphasis on cancer cells. The potential of CuO Nanoparticles (NPs) in cancer treatment has been a subject of investigation. These NPs have been shown to induce apoptosis via the generation of Reactive Oxygen Species (ROS), alter the integrity of DNA, and interfere with signaling pathways that are essential for the survival and proliferation of cancer cells.14 The use of a multidimensional method renders CuO Nanoparticles (NPs) potential candidates for novel cancer therapeutics. The synthesis of copper oxide nanoparticles is facilitated by the use of Amaranthus tricolor extracts, which harness bioactive chemicals present in plants. This environmentally conscious technique eliminates the need for potentially harmful substances, and is in accordance with sustainable and ecologically responsible methodologies. The bioactive constituents of Amaranthus extracts, namely polyphenols and flavonoids, exhibit dual functionality as both reducing and capping agents in nanoparticle production. The capacity to assume a dual function facilitates the attainment of meticulous control over the dimensions, configuration, and durability of nanoparticles, thereby customizing them to suit certain therapeutic applications.15 CuO NPs generated by phytofabrication using Amaranthus tricolor extracts provide a unique synergy between natural plant chemicals and nanotechnology in this context. The nanoparticles exhibited the intrinsic bioactivity of Amaranthus, including antioxidant and anti-inflammatory characteristics, with the therapeutic capabilities of CuO NPs, notably in the context of cancer treatment. The integration of these novel elements has potential for the development of nanomedicine applications that are ecologically sustainable and biocompatible. This serves as a demonstration of the efficacy and environmental consciousness achieved through multidisciplinary research in the healthcare field.
MATERIALS AND MATERIALS
Source of the plant and method of extraction
The leaves of Amaranthus tricolor were collected from a nearby area of Saveetha Medical College in Chennai, India. The verdant foliage was meticulously minced and thoroughly cleaned with distilled water to remove any soil or impurities, and then a mixture was prepared by combining 10 g of the purified leaves with 100 mL of distilled water, followed by grinding them into a homogeneous blend with a mortar and pestle. The resultant mixture was subjected to boiling at a temperature of 100°C for duration of 15 to 20 min. Subsequently, the mixture was filtered through Whatman filter paper No.1 to obtain a refined leaf extract derived from the leaves. The solution that had been filtered was kept at a temperature of 4°C using refrigeration in order to preserve it for future investigations.13
Synthesis and characterization of nanoparticles of copper oxide
Initially, the filtrate obtained from the plant extract and a precisely measured solution of Copper Sulfate Pentahydrate (CuSO4·5H2O) at a concentration of 1 M in water were mixed in a 1:1 ratio. The mixture was maintained in a heated magnetic agitator and boiled for 60 min at 80°C. Simultaneously, 1 M Sodium Hydroxide (NaOH) solution was added progressively until the formation of copper oxide nanoparticles was indicated by the development of a distinct blackish-brown color. Owing to the presence of Amaranthus tricolor extract, the resulting precipitate was meticulously rinsed with ethanol and distilled water to remove any remaining impurities. Following the protocol established by Sumitha et al.,16 the nanoparticles were subjected to dehydration at 60°C. The CuONPs produced using Amaranthus tricolor extract were extensively analyzed to determine their characteristics. The 200-800 nm wavelength range of the Shimadzu UV-visible spectrophotometer was used to evaluate the optical properties of the samples, which reflected the effects of the plant extract. FTIR analysis was conducted using a JASCO FTIR-4700 instrument in the wavelength range 4000-400 cm1, confirming the involvement of Amaranthus tricolor compounds in the synthesis. The unique contributions of the plant extract were also reflected in the average size of the CuONPs, determined by Dynamic Scattering using a Malvern Instruments Ltd. instrument. To perform crystallographic analysis, X-ray Diffraction (XRD) was performed on the finely powdered copper oxide nanoparticles that were generated. The experiment was conducted using a Rigaku MiniFlex-II Desktop X-ray diffractometer, with working conditions set at 30 kV and 15 mA, utilizing Cu K radiation. The diffracted intensities were measured at a sample rate of 4°/ min, with a sampling step of 0.05°C. The measurements covered 2θ angles ranging from 5 to 80°C. The contribution of the plant extract to the thermal stability of the nanoparticles was also evaluated using Thermal Gravimetric Analysis (TGA) and Differential Scanning Calorimetry (DSC), which were performed using TA Instruments SDT Q600 and DSC Q200, respectively.17
AntiBacterial Activity
Bacterial samples were collected from the Microbiology Laboratory at Saveetha Medical College to determine the antibacterial properties of A. tricolor leaf extract. Anti-bacterial activity was evaluated using both gram-positive bacteria (Staphylococcus aureus) and gram-negative bacteria (Pseudomonas aeruginosa), using the well diffusion technique according to the standards set by the Clinical and Laboratory Standards Institute (CLSI), as described by Padil et al.18 The substrate used in the experiment consisted of sterile Petri dishes containing Mueller-Hinton agar. Pure bacterial cultures at a concentration of 90 Colony-Forming Units per Milliliter (CFU/ mL) were uniformly distributed throughout the agar surface using a sterile cotton swab. Subsequently, wells were generated using a sterile well borer with a diameter of 8 mm. Different amounts of Copper Oxide Nanoparticles (CuONPs) (75, 100, and 125 μg/mL) were introduced into the wells. Streptomycin sulfate (10 mg/mL) was used as the positive control in all experiments. Subsequently, the test plates were subjected to incubation for duration of 24 hr under controlled conditions at a temperature of 37±2°C. Following the incubation period, the zones of inhibition were carefully assessed, yielding valuable information on the anti-bacterial effectiveness of the Copper Oxide Nanoparticles (CuONPs) against both gram-positive and gram-negative bacterial strains.19
Antioxidant activity
The synthesis and characterization of copper oxide nanoparticles were initiated by carefully mixing the filtrate derived from the plant extract with a precisely measured solution of Copper Sulfate Pentahydrate (CuSO4·5H2O), which had a concentration of 1 M in water, in an impeccably balanced 1:1 ratio. The antioxidant activity of Amaranthus tricolor was evaluated using the DPPH test technique, which is well-known in the field. The experimental approach outlined by Letchumanan et al.20 was used. To assess the antioxidant capacity, several quantities of leaf extracts and CuONPs were produced, spanning a range of 5 to 30 mg/mL. Next, 1 mL DPPH solution (0.1 mM) was added to each concentration. The resulting solution was incubated for 30 min in the dark. Following the designated incubation time, absorbance readings were quantified at a specific wavelength of 517 nm. The inhibition % was calculated using the following formula:
Anticancer Evaluation of CuONPs on MCF7 Cells via MTT Assay
The MTT test was used to evaluate the anticancer efficacy of the biosynthesized Copper Oxide Nanoparticles (CuONPs) in the MCF7 breast cancer cell line. MCF7 cells were grown in appropriate medium and plated in 96-well plates. Different concentrations of Copper Oxide Nanoparticles (CuONPs) were introduced into the wells with the inclusion of the control samples. Following the incubation period, the culture medium was replaced and the MTT reagent was used to facilitate the formation of formazan crystals. The absorbance was measured and cell viability was calculated. The Inhibitory Concentration (IC50) was determined to assess the concentration at which 50% of cell viability was impeded. The present technique provides a thorough evaluation of the anticancer capabilities of CuONPs, elucidating their cytotoxic effects and potential as agents for combating breast cancer cells, specifically MCF7.21–23
Statistical analysis
The experiments were conducted in triplicate to ensure the robustness and dependability of the results. The precision of the measurements was reflected using mean values and standard deviations to communicate the data obtained from the experiments. The Inhibitory Concentration (IC50) values were determined using rigorous and exhaustive linear regression square analysis, which allowed for precise quantification of the concentration at which 50% of the observed effect or response was attained. Statistical analysis was performed to ensure the accuracy and significance of the IC50 values, which are essential for determining the biological activities and potential applications of the tested nanoparticles.
RESULTS
CuONP synthesis and characterization
The FTIR assay of the CuONPs revealed distinct peaks at specific wavenumbers such as 3372, 1972, 2048, and 575 cm-1. Notably, the absorption peak at 1972 cm-1 corresponds to the amide I bond of proteins, which is attributed to carbonyl elongation within proteins. In addition, the stretching vibrations of the Hydroxyl (OH) groups in alcohols and phenolic compounds accounted for the peak at 3372 cm-1 (Figure 1). FTIR analysis revealed the presence of various functional groups, including alcohols (OH stretching, H-bonding), alkenes (C=C stretching), and primary amines (NH bending). The prominent peak at 617 cm1 corresponds to the stretching vibration characteristic of CuO, providing additional evidence for the formation of CuO NPs. This observation is consistent with previous findings, highlighting the significance of the peak at 617 cm1, which represents the Cu-O bond in the monoclinic phase and indicates the formation of CuONPs.24 Praba et al.25 and Maruthupandy et al.26 also reported peaks at 618 cm1 and 480 cm1 corresponding to Cu-O stretching vibrations, further confirming the formation of CuONPs. In addition, CuONPs derived from various plant sources, such as the leaves of C. japonica and A. lunulatum, exhibit distinct peaks at 525 cm1, which correspond to Cu-O nanostructures.27 The results provided strong evidence that the phytosynthesized nanoparticles in this study are, in fact, CuONPs.

Figure 1:
Fourier-Transform Infrared (FTIR) Analysis of CuONPs.
In the context of SEM analysis, the visual examination of the morphology of the synthesized CuONPs provided intriguing insights. The A. multicolor-CuONPs had a spherical shape with distinct cell-spreading patterns, whereas the GS-CuONPs had a spherical morphology (Figure 2). These results are consistent with previous studies that have reported various morphologies of Copper Oxide Nanoparticles (CuONPs) produced from diverse origins. Rod-shaped nanoparticles were detected in a study conducted by Sankar et al.28 using C. papaya, but spherical agglomerates were created from S. indica leaves, as reported by Prasad et al.29 The influence of the original seed shape on the resultant nanoparticle morphology is a noteworthy aspect of the interaction between the precursor and the reducing agent. As the reaction progressed, the growth of crystal facets played a crucial role in shaping the nanoparticles, as documented in the literature.30 The size, shape, and surface chemistry of NPs have a significant impact on their potential applications in a variety of disciplines.31

Figure 2:
SEM Analysis of CuONPs Morphology.
In addition, XRD analysis confirmed the composition of the synthesized CuONPs, revealing Copper (Cu) and Oxygen (O2) peaks (Figure 3). Rehana et al.32 reported strong Cu peaks along with faint sulfur peaks when CuONPs were synthesized using medicinal plants. XRD analysis confirmed the elemental composition of the CuONPs and their formation. Overall, the evidence from color change, FTIR analysis, SEM morphology evaluation, and XRD analysis strongly supports the successful synthesis of CuONPs in this study, validating their formation and properties, which are crucial to their potential applications in various fields, including nanomedicine and healthcare.
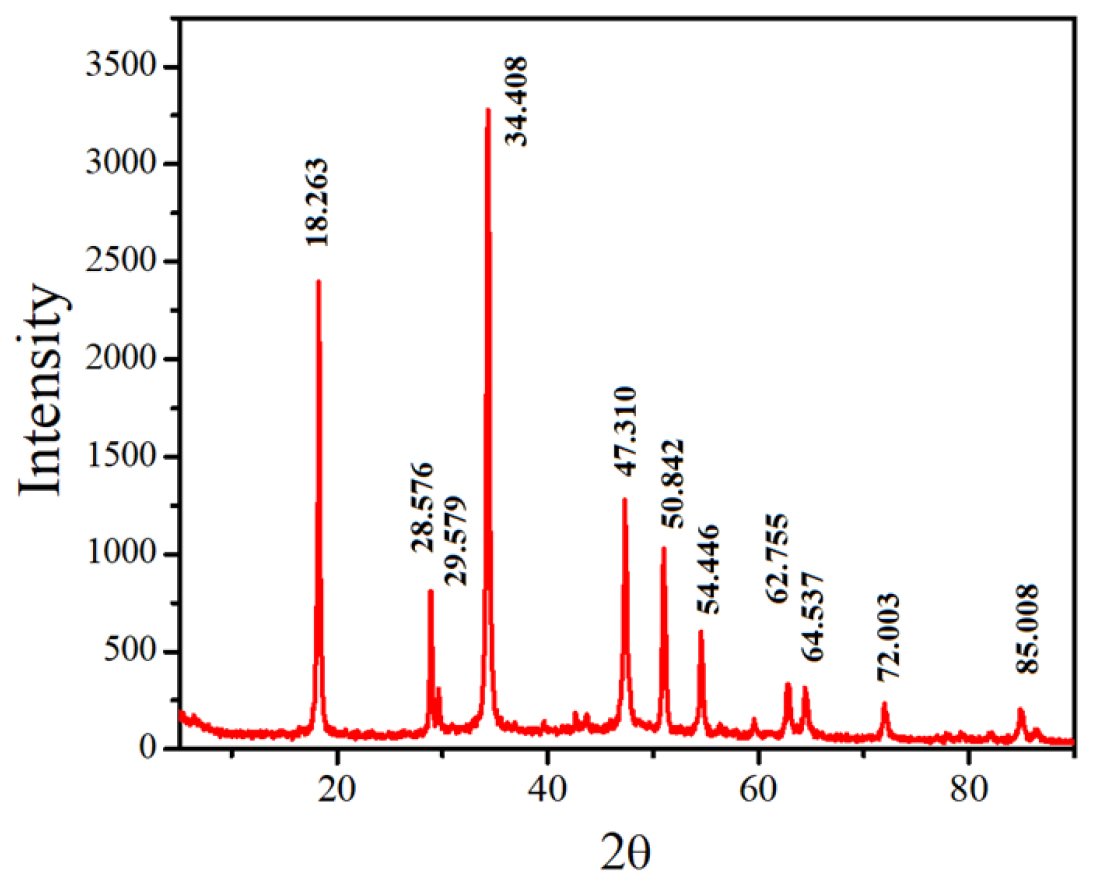
Figure 3:
XRD Analysis of A. tricolor-CuONPs.
Anti-bacterial activity
Anti-bacterial activity was evaluated by measuring the zone of inhibition on the bacterial culture plates, presented in Millimeters (mm) (Figure 4). The ethanolic extract of Amaranthus tricolor exhibited a modest inhibitory effect on bacterial growth compared with the positive control (p<0.05). Interestingly, the Gram-positive bacterium Streptococcus aureus displayed greater sensitivity to the tested plant extract than the gram-negative bacterium Pseudomonas aeruginosa. This observation is consistent with the findings of AlMamun et al.33 These results underscore the dual functionality of the constituent components of Amaranthus tricolor, not only demonstrating antiradical properties but also exhibiting antibacterial activity. Consequently, the whole red amaranth plant has emerged as a promising source of natural antioxidants and essential nutrients with significant potential for promoting human health.
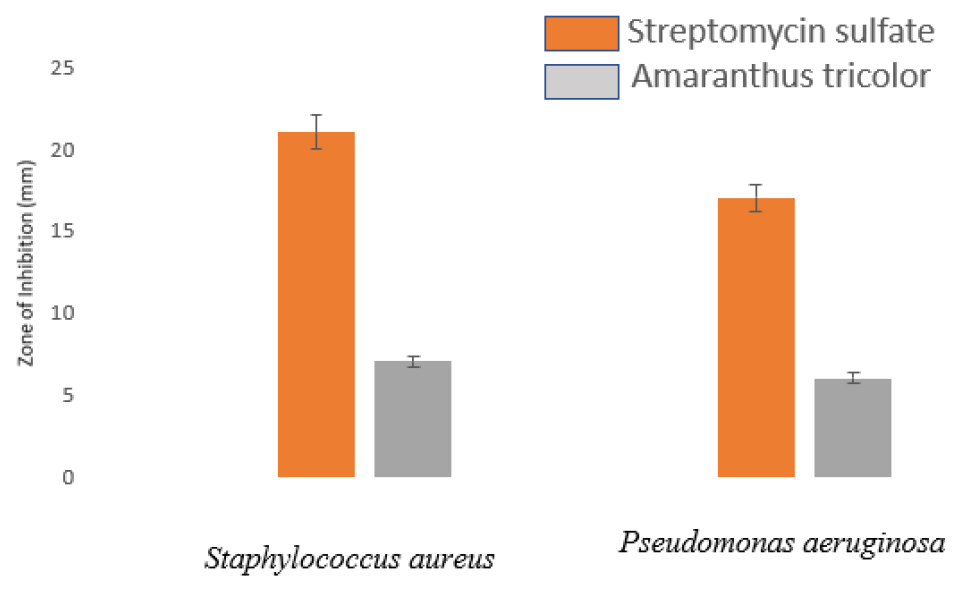
Figure 4:
Anti-bacterial activity of green synthesized CuONPs using A. tricolor.
The observed antibacterial activity of Amaranthus tricolor aligns with a growing body of research, highlighting the therapeutic potential of plant-based compounds against microbial pathogens. The presence of phytochemicals, such as polyphenols, flavonoids, and alkaloids, in Amaranthus tricolor may contribute to its antibacterial properties. These compounds have demonstrated the ability to interfere with microbial cell walls, disrupt cellular functions, and inhibit bacterial growth, thus offering potential as natural antibacterial agents. Furthermore, the differential sensitivity of Gram-positive and Gram-negative bacteria to the plant extract suggests that the antibacterial mechanism may involve specific interactions with bacterial cell structures or membranes.34 The exploration of Amaranthus tricolor as a source of both anti-radical and anti-bacterial agents underscores the multifaceted health benefits of this plant and highlights its potential for use in various therapeutic and nutritional applications. These findings contribute to a broader understanding of the pharmacological properties of Amaranthus tricolor and their significance in natural health care.
Antioxidant activity
The DPPH (2,2-diphenyl-1-picrylhydrazyl) assay is a widely used method for evaluating the antioxidant potential of compounds by measuring their ability to neutralize free radicals. In this study, the DPPH assay was used to compare the radical scavenging capabilities of the biosynthesized Copper Oxide Nanoparticles (CuONPs) and an aqueous extract of A. multicolor (Amaranthus tricolor). The IC50 value of this assay is a crucial parameter because it indicates the concentration at which 50% of free radicals are neutralized. A lower IC50 value indicates a higher antioxidant capacity. In the DPPH assay, CuONPs demonstrated superior radical-scavenging properties compared to plant extracts. The IC50 value of the aqueous extract of A. muticolor was 15 0.89 mg/mL, indicating a moderate antioxidant potential. The IC50 value of the CuONPs was significantly lower (5 mg/mL). This significant disparity in IC50 values demonstrates the superior antioxidant effectiveness of CuONPs in neutralizing free radicals (Figure 5). Several factors may contribute to the increased antioxidant activity of CuONPs. CuONPs have a high surface area and unique physicochemical properties that allow them to interact with and neutralize free radicals effectively. In addition, their nanoscale size increases their bioavailability and cellular absorption, thereby enhancing their antioxidant effects. Copper ions on the surface of nanoparticles may also play a role in scavenging free radicals via redox reactions. Additionally, phytofabrication processes involving plant extracts may result in the synthesis of CuONPs with tailored surface chemistry, thereby rendering them more effective radical scavengers. The bioactive compounds in the plant, such as polyphenols and flavonoids, may contribute to the antioxidant potential of the CuONPs. These compounds can function as capping and stabilizing agents during the nanoparticle synthesis, influencing their antioxidant properties.35

Figure 5:
Antioxidant Potential of A. tricolor Leaf Extracts and CuONPs.
CuONPs from A. tricolor Extract Cytotoxic to MCF7 Breast Cancer Cells
Evaluation of the cytotoxicity of Copper Oxide Nanoparticles (CuONPs) synthesized with A. tricolor extract against the MCF7 breast cancer cell line revealed a dose-dependent effect in the current study. The results demonstrated that as the concentration of CuONPs increased, their cytotoxic effects on MCF7 cells also increased. The IC50 values for the respective CuONP concentrations, which were determined to be 84.24 g/mL and 76.85 g/mL (Figure 6), further corroborated this dose-dependent relationship. These results demonstrated the potential of CuONPs as selective cytotoxic agents against breast cancer cells, particularly MCF7 cells. The dose-dependent cytotoxic effect observed in this study is consistent with prior research, indicating that CuONPs possess intriguing anti-cancer properties.36 The mechanism of cytotoxicity is multifaceted and involves multiple pathways. CuONPs elicit oxidative stress in cancer cells through the production of Reactive Oxygen Species (ROS), which can result in cellular damage and apoptosis. In addition, CuONPs may interfere with essential cellular processes such as DNA replication and repair, resulting in cell death. These results are consistent with those of previous studies that demonstrated the cytotoxic effects of CuONPs on numerous cancer cell lines, including breast cancer cells. In addition, the capacity of CuONPs to exhibit selective cytotoxicity against cancer cells while sparing healthy cells is crucial for their potential therapeutic application. This selectivity is a result of the unique size and surface properties of CuONPs, which enable them to preferentially target cancer cells. This selectivity can reduce collateral injury to benign tissues, which is a significant benefit of cancer therapy.37
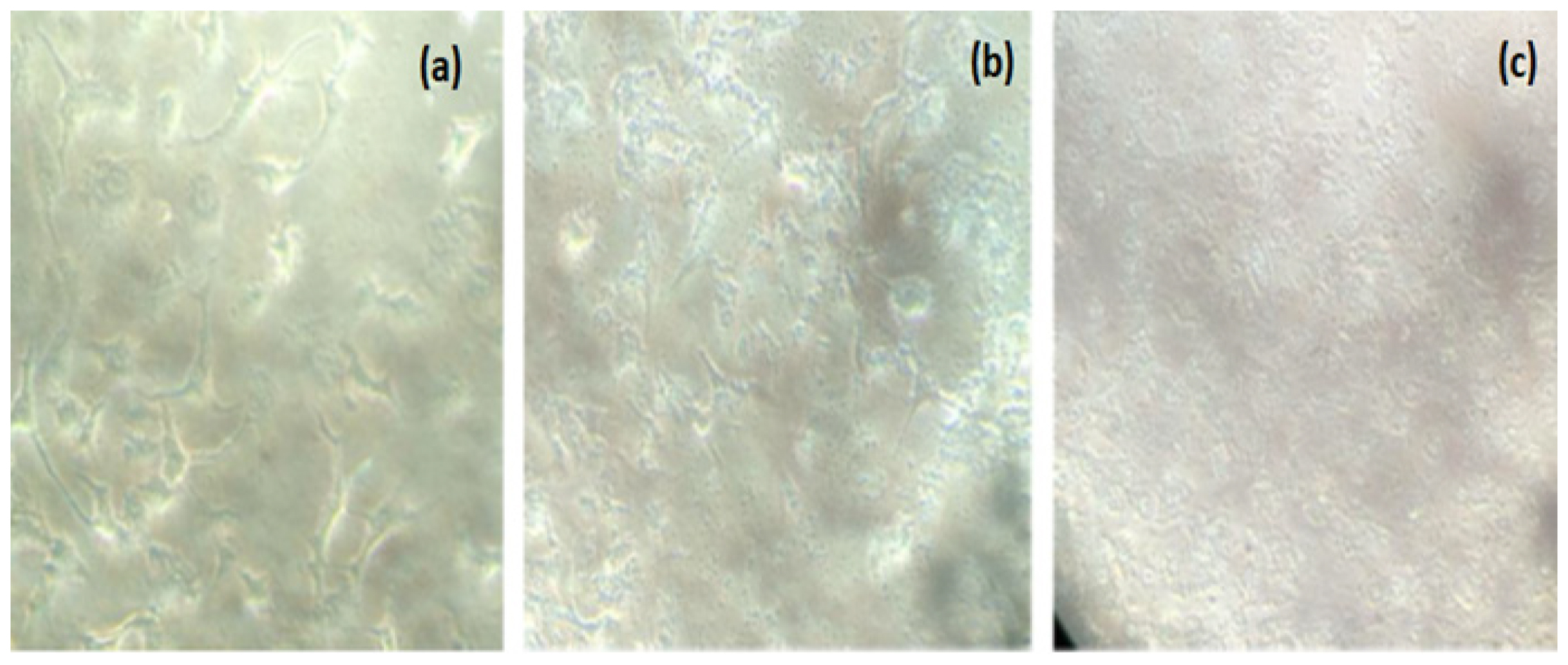
Figure 6:
Cell Morphology Observations in MTT Assay.
DISCUSSION
The synthesized CuONPs from Amaranthus tricolor is reported to FTIR, XRD, SEM analysis, cytotoxic effect to MCF7 breast cancer cells, antibacterial and antioxidant activity. In FTIR analysis, the synthesized CuONPs exhibited distinct peaks at 525 cm1, which correspond to Cu-O nanostructures.27 The results provided strong evidence that the phytosynthesized nanoparticles in this study are CuONPs. The XRD analysis confirmed the elemental composition of the CuONPs and their formation. In the SEM analysis, the growth of crystal facets played a crucial role in shaping the nanoparticles. The presence of phytochemicals, such as polyphenols, flavonoids, and alkaloids, in Amaranthus tricolor may contribute to its antibacterial properties. The bioactive compounds in the plant, such as polyphenols and flavonoids, may contribute to the antioxidant potential of the CuONPs. These compounds can function as capping and stabilizing agents during the nanoparticle synthesis, influencing their antioxidant properties. In addition, the capacity of CuONPs to exhibit selective cytotoxicity against cancer cells while sparing healthy cells is crucial for their potential therapeutic application.
CONCLUSION
In conclusion, the phytofabrication process employing extracts from Amaranthus tricolor has shown impressive results for the production and characterization of Copper Oxide Nanoparticles (CuONPs). The effective synthesis of CuONPs was strongly supported by several key findings. First, the solution shifted from blue to brownish-black, indicating that a chemical change occurred. Additionally, different peaks were observed in the FTIR spectra, suggesting the presence of specific functional groups associated with the CuONPs. Furthermore, various morphologies were identified via SEM analysis, indicating the production of CuONPs of various shapes and sizes. Finally, the confirmation of the elemental composition by XRD provides further evidence for the successful synthesis of CuONPs. Nanoparticles have significant potential in many applications, including nanomedicine and healthcare, owing to their distinctive physicochemical characteristics and therapeutic capabilities. Moreover, research has emphasized the many advantages of Amaranthus tricolor, not only as a provider of CuONPs but also as a substantial repository of innate antioxidants and antimicrobial substances. The antimicrobial efficacy against both Gram-positive and Gram-negative bacteria highlights the medicinal capacity of this plant to combat microbial diseases. Moreover, the exceptional radical-scavenging capacity of Copper Oxide Nanoparticles (CuONPs), as shown by the DPPH test, underscores their efficacy as powerful antioxidants. This may be related to their unique nanoscale characteristics and interactions with free radicals. Furthermore, examination of the cytotoxic properties of Copper Oxide Nanoparticles (CuONPs) on MCF7 breast cancer cells demonstrated a correlation between the administered dosage and the observed impact. Notably, the nanoparticles have shown considerable promise as agents that selectively induce cell death in cancerous cells. This discovery holds great promise for prospective use of these findings in the field of cancer treatment. The ability of these substances to generate oxidative stress and disrupt vital cellular mechanisms may be of significant importance in the fight against cancer. This study provides empirical evidence supporting an environmentally conscious and sustainable method of utilizing plants to synthesize Copper Oxide Nanoparticles (CuONPs). Additionally, it highlights the wide range of potential therapeutic applications of Amaranthus tricolor, positioning it as a valuable asset in the fields of nanotechnology, healthcare, and natural medicine. The cumulative results provide a foundation for further investigation and use of CuONPs and Amaranthus tricolor across diverse scientific fields and sectors, potentially yielding significant advancements in healthcare and environmental preservation.
Cite this article
Jaculin C, Viswanathan D, Govindasamy R. Phytofabricated Copper Oxide Nanoparticles from Amaranthus tricolor Leaf Extract: Assessing Antioxidant and Anticancer Potentials. Int. J. Pharm. Investigation. 2024;14(2):509-16.
ACKNOWLEDGEMENT
The authors are grateful to Saveetha Medical College Hospital and Saveetha Dental College and Hospital (SIMATS), Saveetha University, Chennai, for their support throughout my research.
ABBREVIATIONS
CuO NPs | Copper oxide nanoparticles |
---|---|
FTIR | Fourier-transform infrared |
SEM | Scanning electron microscope |
XRD | X-ray diffraction |
DPPH | Diphenyl picryl hydrazyl |
MCF7 | Michigan Cancer Foundation -7 |
ROS | Reaxtive Oxygen Species |
CuSO4.5H2O | Copper Sulfate Pentahydrate |
NaOH | Sodium Hydroxide |
TGA | Thermal Gravimetric Analysis |
DSC | Dofferential Scanning Calorimetry |
CLSI | Clinical and Laboratory Standards Institute |
CFU | Colony Forming Unit |
MTT | Dimethylthiazol Diphenyl Tetrazolium bromide |
IC50 | Half-maximal Inhibitory Concentration. |
References
- Shi J, Votruba AR, Farokhzad OC, Langer R. Nanotechnology in drug delivery and tissue engineering: from discovery to applications. Nano Lett. 2010;10(9):3223-30. [PubMed] | [CrossRef] | [Google Scholar]
- Haleem A, Javaid M, Singh RP, Rab S, Suman R. Applications of nanotechnology in the medical field: A brief review. Global Health. 2023;7(2):70-7. [CrossRef] | [Google Scholar]
- Ahmad B, Shireen F, Rauf A, Shariati MA, Bashir S, Patel S, et al. Phyto-fabrication, purification, characterization, optimisation, and biological competence of nanosilver. IET Nanobiotechnol. 2021;15(1):1-18. [PubMed] | [CrossRef] | [Google Scholar]
- Zuhrotun A, Oktaviani DJ, Hasanah AN. Biosynthesis of gold and silver nanoparticles using phytochemical compounds. Molecules. 2023;28(7):3240 [PubMed] | [CrossRef] | [Google Scholar]
- Bhardwaj B, Singh P, Kumar A, Kumar S, Budhwar V. Eco-friendly greener synthesis of nanoparticles. Adv Pharm Bull. 2020;10(4):566-76. [PubMed] | [CrossRef] | [Google Scholar]
- Chu EW, Karr R. Reference module in life sciences. In book Encyclopedia of biodiversity. 2017;3:278-96. [PubMed] | [CrossRef] | [Google Scholar]
- Khanam UKS, Oba S. Bioactive substances in leaves of two amaranth species, Amaranthus tricolor and A. hypochondriacus. Can J Plant Sci. 2013;93(1):47-58. [CrossRef] | [Google Scholar]
- Sarker U, Oba S. Nutrients, minerals, pigments, phytochemicals, and radical scavenging activity in Amaranthus blitum leafy vegetables. Sci Rep. 2020;10(1):3868 [PubMed] | [CrossRef] | [Google Scholar]
- Baraniak J, Kania-dobrowolska M. The dual nature of amaranth-functional food and potential medicine. Foods. 2022;11(4):618 [PubMed] | [CrossRef] | [Google Scholar]
- Kumari S, Elancheran R, Devi R. Phytochemical screening, antioxidant, antityrosinase, and antigenotoxic potential of Amaranthus viridis extract. Indian J Pharmacol. 2018;50(3):130-8. [PubMed] | [CrossRef] | [Google Scholar]
- Khandaker L, Ali MB. S, Oba U. Total polyphenol and antioxidant activity of red amaranth (Amaranthus tricolor L.) as affected by different sunlight level. J Jpn Soc Hortic Sci. 2008;77(4):395-401. [CrossRef] | [Google Scholar]
- Srivastava R. An updated review on phyto-pharmacological and pharmacognostical profile of Amaranthus tricolor: a herb of nutraceutical potentials. J Pharm Innov. 2017;6(6):124 [CrossRef] | [Google Scholar]
- Sarker U, Oba S. Protein, dietary fiber, minerals, antioxidant pigments and phytochemicals, and antioxidant activity in selected red morph Amaranthus leafy vegetable. PLOS ONE. 2019;14(12):e0222517 [PubMed] | [CrossRef] | [Google Scholar]
- Naz S, Gul A, Zia M. Toxicity of copper oxide nanoparticles: a review study. IET Nanobiotechnology. 2020;14(1):1-13. [PubMed] | [CrossRef] | [Google Scholar]
- Begum S, Esakkiraja A, Asan S, Muthumari M, Raj G. Green synthesis of copper oxide nanoparticles using Catharanthus roseus Leaf extract and their antibacterial activity. Int J Sci Res Multidiscip Stud. 2019;5(8):21-7. [PubMed] | [CrossRef] | [Google Scholar]
- Sumitha S, Vidhya RP, Subalakshmi M, Shanmuga Prasad K. Leaf extract mediated green synthesis of copper oxide nanoparticles using Ocimum tenuiflorum and its characterisation. Int J Chem Sci. 2016;14(1):435-40. [PubMed] | [CrossRef] | [Google Scholar]
- Amjad R, Mubeen B, Ali SS, Imam SS, Alshehri S, Ghoneim MM, et al. Green synthesis and characterization of copper nanoparticles using Fortunella margarita Leaves. Polymers. 2021;13(24):4364 [PubMed] | [CrossRef] | [Google Scholar]
- Thekkae Padil VVT, Černík M. Green synthesis of copper oxide nanoparticles using gum karaya as a biotemplate and their antibacterial application. Int J Nanomedicine. 2013;8:889-98. [PubMed] | [CrossRef] | [Google Scholar]
- Chandrasekar A, Vasantharaj S, Jagadeesan NL, Shankar SN, Pannerselvam B, Bose VG, et al. Studies on phytomolecules mediated synthesis of copper oxide nanoparticles for biomedical and environmental applications. Biocatal Agric Biotechnol. 2021;33(1):101994 [CrossRef] | [Google Scholar]
- Letchumanan D, Sok SPM, Ibrahim S, Nagoor NH, Arshad NM. Plant-based biosynthesis of copper/copper oxide nanoparticles: an update on their applications in biomedicine, mechanisms, and toxicity. Biomolecules. 2021;11(4):564 [PubMed] | [CrossRef] | [Google Scholar]
- Mahmood RI, Kadhim AA, Ibraheem S, Albukhaty S, Mohammed-Salih HS, Abbas RHMK, Nayef UM, AlMalki FA, Sulaiman GM, Al-Karagoly H, et al. Biosynthesis of copper oxide nanoparticles mediated Annona muricata as cytotoxic and apoptosis inducer factor in breast cancer cell lines. Sci. Rep. 2022;12(1):16165 [PubMed] | [CrossRef] | [Google Scholar]
- Sathiyavimal S, Vasantharaj S, Bharathi D, Saravanan M, Manikandan E, Kumar SS, et al. Biogenesis of copper oxide nanoparticles (CuONPs) using Sida acuta and their incorporation over cotton fabrics to prevent the pathogenicity of Gram negative and Grampositive bacteria. J Photochem Photobiol B. 2018;188:126-34. [PubMed] | [CrossRef] | [Google Scholar]
- Vanathi P, Rajiv P, Sivaraj R. Synthesis and characterization of Eichhornia-mediated copper oxide nanoparticles and assessing their antifungal activity against plant pathogens. Bull Mater Sci. 2016;39(5):1165-70. [CrossRef] | [Google Scholar]
- Nagore PB, Ghoti AJ, Salve AP, Mane KG. RETRACTED ARTICLE: green synthesis of luminescent copper oxide nanoparticles using ginger lily leaves extract. J Inorg Organomet Polym Mater. 2020;30(10):4229 [CrossRef] | [Google Scholar]
- Lakshmi praba K, Jayashree M, Shakila Banu K, Gino A. Kurian. Green and chemically synthesized copper nanoparticles-A preliminary research towards its toxic behaviour. Int J Pharm Pharm Sci. 2015;7(13):156-60. [CrossRef] | [Google Scholar]
- Maruthupandy M, Zuo Y, Chen JS, Song JM, Niu HL, Mao CJ, et al. Synthesis of metal oxide nanoparticles (CuO and ZnO NPs) via biological template and their optical sensor applications. Appl Surf Sci. 2017;397:167-74. [CrossRef] | [Google Scholar]
- Sarkar J, Chakraborty N, Chatterjee A, Bhattacharjee A, Dasgupta D, Acharya K, et al. Green synthesized copper oxide nanoparticles ameliorate defence and antioxidant enzymes in Lens culinaris. Nanomaterials (Basel). 2020;10(2):312 [PubMed] | [CrossRef] | [Google Scholar]
- Sankar R, Manikandan P, Malarvizhi V, Fathima T, Shivashangari KS, Ravikumar V, et al. Green synthesis of colloidal copper oxide nanoparticles using Carica papaya and its application in photocatalytic dye degradation. Spectrochim Acta A Mol Biomol Spectrosc. 2014;121:746-50. [PubMed] | [CrossRef] | [Google Scholar]
- Prasad KS, Patra A, Shruthi G, Chandan S. Aqueous extract of Saraca indica leaves in the synthesis of copper oxide nanoparticles: finding a way towards going green. J Nanotechnol. 2017;1:1-6. [PubMed] | [CrossRef] | [Google Scholar]
- Salzemann C, Lisiecki I, Urban J, Pileni MP. Anisotropic copper nanocrystals synthesized in a supersaturated medium: nanocrystal growth. Langmuir. 2004;20(26):11772-7. [PubMed] | [CrossRef] | [Google Scholar]
- Albanese A, Tang PS, Chan WCW. The effect of nanoparticle size, shape, and surface chemistry on biological systems. Annu Rev Biomed Eng. 2012;14:1-16. [PubMed] | [CrossRef] | [Google Scholar]
- Rehana D, Mahendiran D, Kumar RS, Rahiman AK. Evaluation of antioxidant and anticancer activity of copper oxide nanoparticles synthesized using medicinally important plant extracts. Biomed Pharmacother. 2017;89:1067-77. [PubMed] | [CrossRef] | [Google Scholar]
- Al-Mamun MA, Husna J, Khatun M, Hasan R, Kamruzzaman M, Hoque MKMF, et al. Assessment of antioxidant, anticancer and antimicrobial activity of two vegetable species of Amaranthus in Bangladesh. BMC Complement Altern Med. 2016;16:1-11. [PubMed] | [CrossRef] | [Google Scholar]
- Sarker U, Islam MT, Rabbani MG, Oba S. Variability in total antioxidant capacity, antioxidant leaf pigments and foliage yield of vegetable amaranth. J Integr Agric. 2018;17(5):1145-53. [CrossRef] | [Google Scholar]
- Baliyan S, Mukherjee R, Priyadarshini A, Vibhuti A, Gupta A, Pandey RP, et al. Determination of antioxidants by DPPH radical scavenging activity and quantitative phytochemical analysis of Ficus religiosa. Molecules. 2022;27(4):1326 [PubMed] | [CrossRef] | [Google Scholar]
- Duan H, Wang D, Li Y. Green chemistry for nanoparticle synthesis. Chem Soc Rev. 2015;44(16):5778-92. [PubMed] | [CrossRef] | [Google Scholar]
- Mahmood RI, Kadhim AA, Ibraheem S, Albukhaty S, Mohammed-Salih HS, Abbas RH, et al. Biosynthesis of copper oxide nanoparticles mediated Annona muricata as cytotoxic and apoptosis inducer factor in breast cancer cell lines. Sci Rep. 2022;12(1):16165 [PubMed] | [CrossRef] | [Google Scholar]