Contents
ABSTRACT
Aim
The study’s goal was to develop and characterize a new type of Fenoprofen tablet that can float.
Materials and Methods
Research on the drug’s performulation was carried out to evaluate its organoleptic qualities, solubility, melting point, and partition coefficient. Bulk and pharmaceutical formulations of the medication were evaluated using UV spectroscopy to identify Fenoprofen in accordance with ICH Guidelines. Fenoprofen floating tablets were made utilizing the direct compression method with different concentrations of HPMC K100 M, Xanthan gum, and guar gum. Furthermore, a QbD method was used to manufacture the Fenoprofen tablets. To find the optimal formulation of the solid dispersed drug material in Fenoprofen tablets, a 23-full factorial Design of Experiment (DoE) was carried out using Design-Expert 22.0.2.0 software.
Results
The produced tablets were subjected to a micromeritics research, which included both a pre- and post-compression phase. Using a USP Dissolution test device type II (Paddle), an in vitro dissolution research was conducted. There were three months of stability testing done at high temperatures. The research confirmed that Fenoprofen had a distinct odor and a white color look. Its melting point (MP) was 171°C, and it was soluble in DMSO without any difficulty. We measured 0.31gm/cm3 for the optimized formulation’s bulk density, 0.46gm/cm3 for the tapped density, 14.78 for the Carr index, 1.42 for the hausner ratio, and 30.17 degrees for the angle of repose. The optimized batch (F4) including polymers and excipients floated for a long duration and had a short lag time for buoyancy. There was a 99.12 percent drug release rate after 12 hours in in vitro dissolution tests.
Conclusion
The stability showing that formulation was stable for months of storage and minimal change was found in color, shape, appearance, drug content, in vitro dissolution studies and floating lag time value.
INTRODUCTION
In order to overcome the drawbacks of conventional drug delivery methods, researchers have developed the Innovative Drug Delivery System (NDDS).1 To accomplish the required pharmacological effects of a drug in a safe manner, a new technology called Non-traditional Drug Delivery Systems (NDDS) is being developed and implemented.2 Scientific site-targeting within the body has the potential to increase drug potency, regulate drug release, and produce a more sustained pharmacological effect. Recent therapeutic applications and formulation system development strategies to enhance drug delivery potential were discussed at NDDS.3
Due to their excellent efficacy and durability, NDDS have recently garnered much attention, especially in Gastrointestinal (GI) illnesses.4 The addition of NDDS into bilayer floating tablets has also increased the use of the FDDS.5,6 The FDDS approach to drug delivery is simple, inexpensive, and more practical than alternative approaches. The idea is to make the dosage form lighter than the fluids in the stomach so that it floats. Drug Delivery Systems (FDDS) rely heavily on polymers since they are the primary factor in determining stomach retention time and drug protection.7 The floating system utilizes polymers to direct medicine delivery to a specific area of the gastrointestinal tract, specifically the stomach.
Drugs that have weak solubility and limited stability in intestinal fluids can benefit from floating tablets, which were developed to keep the medicine in the stomach.8 Nonsteroidal Anti-Inflammatory Drugs (NSAIDs) include fenoprofen. Fenoprofen is prescribed for the treatment of a wide variety of painful conditions, including osteoarthritis, rheumatoid arthritis, and ankylosing spondylitis, as well as for the management of mild to moderate pain.9 Although it is absorbed fast after oral administration, considerable first-pass metabolism occurs. It causes nausea and gastrointestinal discomfort, among other GI adverse effects.10,11 Its half-life is only 2-3 hr.
Cellulose Ether (CE) is a polymer derived from cellulose that is widely distributed in the natural world. It’s a polymer that dissolves in water, too. Gupta et al. (2021)12 and Tudoroiu et al. (2021)13 note that they are also useful as a suspending agent, protective colloids, lubricants, emulsifiers, and surfactants. Hydrophilic Polyethylene Glycol (HPMC) is widely utilized in the pharmaceutical industry as a hydrophilic gel matrix and bio-adhesion material. The fact that it is safe to use, can be compressed easily, and can hold a lot of drugs has contributed to its widespread acceptance.14,15 It has the properties and potential uses of emulsification, bonding, thickening and adhering, suspension, gelation, and film formation because of its variation in molecular weight and viscosity. When developing products with controlled medication release, HPMC is an excellent polymer to use. Although it remains insoluble at all of the body’s pH levels, it expands in the presence of gastric juice. After being exposed to water, the material becomes permeable and can release modified drugs slowly over time.16,17 This makes it a good option for boosting patients’ adherence. This research was conducted to better disperse Fenoprofen into the body’s bloodstream and to create longer-acting tablets of the medicine. The drug release was also investigated in vitro.
MATERIALS AND METHODS
Pre-formulation studies
Organoleptic Properties
Human sense organs were used to evaluate organoleptic qualities. Color, smell, visual appeal, and other “organoleptic” factors were all taken into account.18
Solubility study
Fenoprofen’s solubility in various solvents was evaluated qualitatively in accordance with USP NF, 2007. An accurately weighed dose of drug (1 mg) was poured into a 10 mL test tube, and then diluted with the appropriate solvents (1 mL of methanol, ethanol, DMSO, chloroform, and acetone).
Melting Point
The open Capillary technique was used to determine the melting point. A little dose of the medication was sealed at one end of a capillary tube that was only 10-15 mm long and around 1 mm in diameter. To ensure that the samples were heated uniformly and gradually, the capillary containing the samples was suspended, and a thermometer was put to monitor the temperature. The melting point is determined by the observed temperature range in which the sample melts.
Partition coefficient
In n-Octanol:water system, drug partition coefficient was investigated. It was determined by taking 5mg of medication in two separating funnels with 20 mL of n-Octanol and 20 mL water. For balance, the separating funnel was shaken for 2 hr in a wrist action shaker. The drug in the aqueous phase was measured spectrophotometrically at 272 nm. Drug partition coefficient was estimated using this formula:
Analytical method development by UV
A process is considered validated if it has been shown, through careful documentation, to reliably and repeatedly yield the intended outcome, with the associated quality attributes and standards, over an extended period of time. Multiple metrics, including linearity, precision, intraday, intraday, ruggedness, and robustness, were used to verify the method’s validity.19
Preparation of standard stock solution
Fenoprofen, equivalent to around 5 mg, was weighed and placed in a 5 mL volumetric flask. A solution with a concentration of 1000 g/mL was prepared by diluting the solid in ethanol until the volume reached 5 mL. The standard stock solution concentration is 100 g/mL, which was achieved by taking 1 mL of the stock solution and diluting it to 10 mL with the appropriate solvent.
Lambda max
A concentration of 20 g/mL was prepared by transferring 2 mL of the aforesaid stock solution into a 10 mL volumetric flask and filling it up to the mark with solvent. UV-vis Spectrophotometer measurements were taken between 200 and 400 nm, with each solvent serving as a blank. Maximum absorbance (max) wavelength was determined.
Formulation of Fenoprofen floating tablets
Fenoprofen floating tablets were made utilizing the direct compression method with different concentrations of HPMC K100 M, Xanthan gum, and guar gum. Fenoprofen 600 mg per tablet was achieved by adjusting the tablet’s weight. A #80 mesh sieve was used to filter all of the particles. The necessary amount of medication and polymers were carefully combined. The final touches were the addition of talc for gliding and 2% magnesium stearate for lubrication. Using a tablet compression machine, the mixture was immediately compressed (punched) to create the tablets. The tablets now measure in at a more manageable 800 mg.20
Optimization of Fenoprofen tablet formulation
By imposing requirements (objectives) on both the response and the factors, an optimal formulation was achieved. To obtain a perfect formula, we used the Box-Behnken Design available in DESIGN EXPERT 22.0.2.0 (STAT-EASE) edition software. Because of the variable nature of the component mixture, 17 distinct formulation batches were created for testing. Numerous formulations were made and tested for all of the indicated outcomes. Analysis of Variance (ANOVA) was used to compare and contrast the two models to find out which one was more significant. A linear relationship between the response and the factor is indicated by a positive sign before the factor in polynomial equations, while a negative sign indicates the inverse relationship. The correlation between the independent [HPMC K100 M, Xanthan gum, and Guar gum] and dependent [Drug content, Floating lag time] variables was calculated across all 17 experiments.21
Pre-compression Evaluation Parameters
Bulk Density
A measured amount of powder that has been sieved through a mesh size of 40 and then carefully put into a graduated cylinder. The powder bed was then made uniform by pouring the powder into the graduated cylinder and not stirring the powder. The volume was then read from the cylinder’s graduation marks and converted to milliliters. The total measured space was referred to as the bulk volume. The formula was used to determine the density of the bulk material.22
Where M is the powder’s mass and Vb is its volume in bulk
Tapped Density
The identical measuring cylinder was used for both the bulk volume and the tap density measurement. The tap density apparatus was run for 500 taps at a rate of 300 taps per minute. After recording the initial volume as (Va), 750 taps were recorded as (Vb). If the discrepancy between Va and Vb is less than 2%, the tapped volume will be calculated based on Vb is.22 The formula for tapped density can be found below.
Where M is the powder’s mass and Vt is the powder’s tapped volume.
Angle of repose
When calculating the angle of repose, the fixed funnel approach was used. Cones develop when powder is poured through a funnel onto a flat surface. It is called the angle of repose, and it is the angle formed by the sides of the cone and the horizontal. Because it indicates the point at which the interparticle attraction is stronger than the force of gravity on a particle, the angle is used as a proxy for the cohesiveness of the powder. The angle of repose is smaller for a powder that flows freely because the resulting cone will have shallower sides.22 By plugging in the measured numbers for the pile’s base radius “r” and height “h,” we were able to calculate the angle of repose.
Here, h = Height of pile,
r = Radius of pile,
⊖ = Angle of repose.
Hausner’sRatio
The measured and mixed powder was weighed, then transferred to the cylinder. After 300 taps, either a constant volume was recorded for the poured bulk volume (Vb) and the tapped volume (Vt), or the poured bulk density (Pb) and the tapped density (Pt) in g/mL were determined with the aid of a tap density tester. The Hausner ratio was determined by plugging the data for the bulk density and the tapped density into the following formula.
Percentage Compressibility (Carr’s Index)
Percentage
The percentage of compressibility, expressed as Carr’s Index, was determined by multiplying the ratio of the tapped density to the bulk density by 100. How to Determine a Carr’s Index (CI).
Post-compression Evaluation
General appearance
The color, shape, and overall look of the tablets are assessed once they have been formulated.22
Thickness
To measure the tablets’ thickness and diameter, a verniar caliper was employed. We utilized an average of 5 pills from each formulation type to get our results. The unit of measure is the mm.
Hardness
The “force required to break a tablet in the diametric compression test” is the standard measure of hardness. The ability to crush a tablet is synonymous with hardness. Tablets’ hardness determines how well they hold up throughout storage, shipping, and handling before to use. The Monsanto tablet hardness tester was used to get accurate results. The tablet was placed between two anvils of a hardness tester (Monsanto), and the force applied to them was increased incrementally until the tablet broke. The pressure at which the tablet breaks was measured using the clearly labeled scale. Kg/cm2 was the unit of measure for hardness. Lists the results of a hardness test.22
Weight variation
According to the protocol, 20 pills were weighed at random on an electric balance, and their average weight was then compared to the weights of the individual tablets.
Friability test
The capacity of tablets to survive abrasion during packaging, handling, and transit is measured by this test. Insufficiently cohesive tablet components are usually the cause of friability. The Roche Friabilator is a circular plastic chamber separated into 2-3 compartments, and the first step is to weigh and place 10 tablets within. Tablets are dropped from a height of 1.5 meters (4.9 feet) and the capsule rotates 100 times in 4 min. After then, the tablets undergo a second round of weighing.22 The percentage represents the difference in weight that was measured. It ought to be 1.0 or below. Table 17 reports an analysis of friability.
Where,
W1= weight of tablets before test,
W2 = weight of tablets after test.
Drug content
UV-visible spectrophotometric analysis is used to determine the total amount of medication present in the formulation. Phosphate buffer pH 7.4 was used to dissolve the formulation, which was then agitated for 5 min before enough of the buffer was added to bring the total amount to 100 mL. After 15 min, the solution was filtered using Whatman filter paper after being sonicated. We made dilutions of the solution and evaluated its absorbance spectrophotometrically at 272 nm against 0.01N HCl as a blank using a UV/visible spectrophotometer.
In vitro Buoyancy Test
The produced tablets were tested for buoyancy in a container of 200 mL 0.1 N HCl (pH 1.2, temp. 370.5°C) in a 250 mL beaker. Visual observation was used to determine the lag time and total buoyancy time from when the dosage form was introduced and when it became buoyant in the medium, as well as the floating durations of tablets. Total Floating Time (TFT) is the amount of time that elapses after a dosage form first floats to the surface of a medium, while Floating Lag Time (FLT) is also known as Buoyancy Lag Time (BLT).23
In vitro dissolution studies
The dissolution tests were performed in a phosphate buffer at pH 7.4 in a volume of 900 mL using a USP type II apparatus (USP XXIII Dissolution Test Apparatus). The dissolution medium was kept at 37±2°C throughout the experiment. At predefined intervals, 2 mL aliquots of sample were removed from the dissolution equipment and replaced with new dissolution medium. Following appropriate dilution in phosphate buffer pH 7.4, absorbance was measured at 272 nm using a UV/visible Spectrophotometer on the samples that were collected. The standard calibration curve was used to determine the percentage of medication release.
Stability studies
The tablet formulations were packed and placed in the stability test chamber for three months of accelerated stability testing at (30°C±2°C and 60 ± 5% RH) and (40°C±2°C and 70 ± 5% RH). The tablets were tested for organoleptic features as color, shape, appearance, drug content, in vitro dissolution studies, and floating lag time at 30, 45, 60, and 90 days (3 months). In compliance with International Conference on Harmonization (ICH) criteria, optimal formulation tablets were tested for stability under accelerated storage conditions for 3 months. The optimal formulation of 0 days was used to compare all results.
RESULTS AND DISCUSSION
Preformulation studies
Organoleptic properties
An evaluation of the API’s organoleptic qualities, including color, odor, appearance and state, was conducted. Fenoprofen was found white in color and had a characteristic odor. Fenoprofen exhibited the same color, appearance, and odor as the I.P. requirements for these characteristics. Preformulation studies of fenoprofen were in agreement with the findings reported by Hirsch et al., 1978.24
Solubility study
Fenoprofen’s solubility was tested in a wide range of volatile and nonvolatile liquids, including methanol, ethanol, chloroform, and acetone. Based on the findings, it can be concluded that the medication is soluble in ethanol and Dimethyl sulfoxide but insoluble in water.
Melting point study
The melting point of a substance can be calculated using the capillary method. Fenoprofen’s melting point was measured to be 171°C, which is within the acceptable range for this medication.
Determination of Partition coefficient
The drug’s partition coefficient in n-Octanol:water was studied. It is the proportion of the unionized drug that exists in the aqueous phase relative to the organic phase at equilibrium. The drug’s lipophilic and hydrophilic properties can be described using the partition coefficient. Lipophilic drugs have P values significantly higher than 1, while hydrophilic drugs have P values significantly lower than 1. Fenoprofen was reported to have partition coefficients of 3.47.
UV-spectroscopy analysis of Fenoprofen
UV-visible spectrophotometer (Shimadzu-1700) was used to determine wavelength (absorption maxima) of a substance. The wavelength of the Fenoprofen was found to be 272 nm (Figure 1).
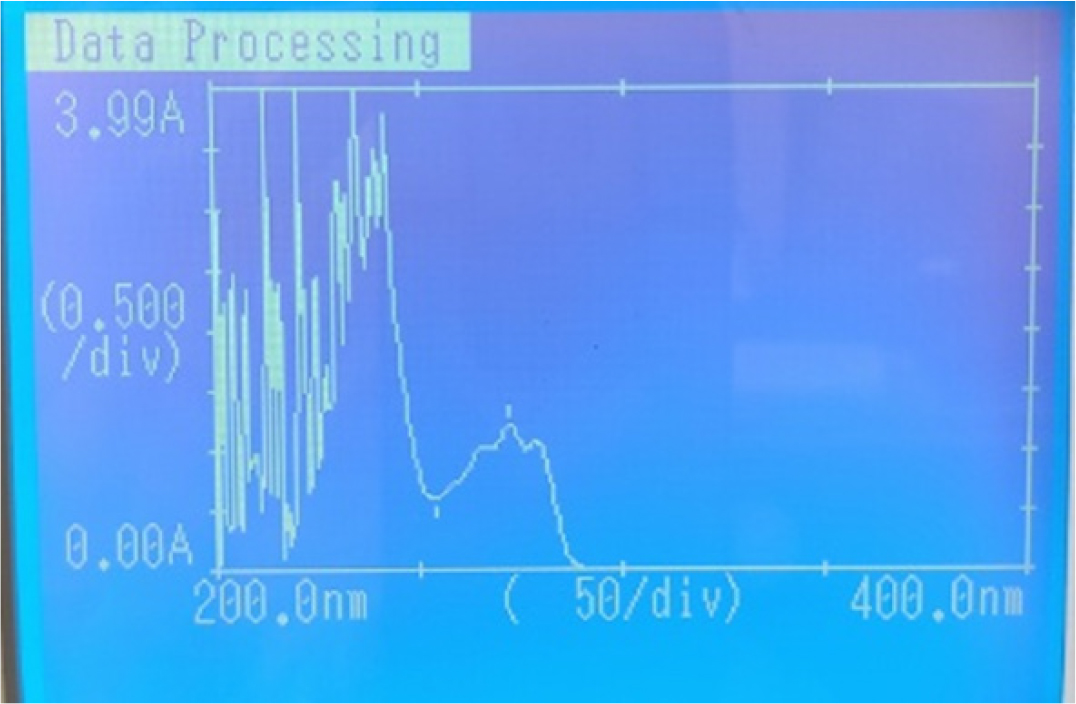
Figure 1:
UV-spectroscopy of Fenoprofen.
Calibration curve
Least-squares linear regression analysis of the calibration curve verified the linearity of the suggested approach. By graphing absorbance against Fenoprofen concentration from 10-70 g/mL, we were able to derive the regression equation for Fenoprofen. In a concentration range of 10-70 ng/mL for the drug, a seven-point calibration curve was obtained. In the concentration range under study, the drug’s reaction was determined to be linear, with a linear regression equation of y = 0.005x + 0.050 and a correlation coefficient of R2 = 0.991 (Figure 2).
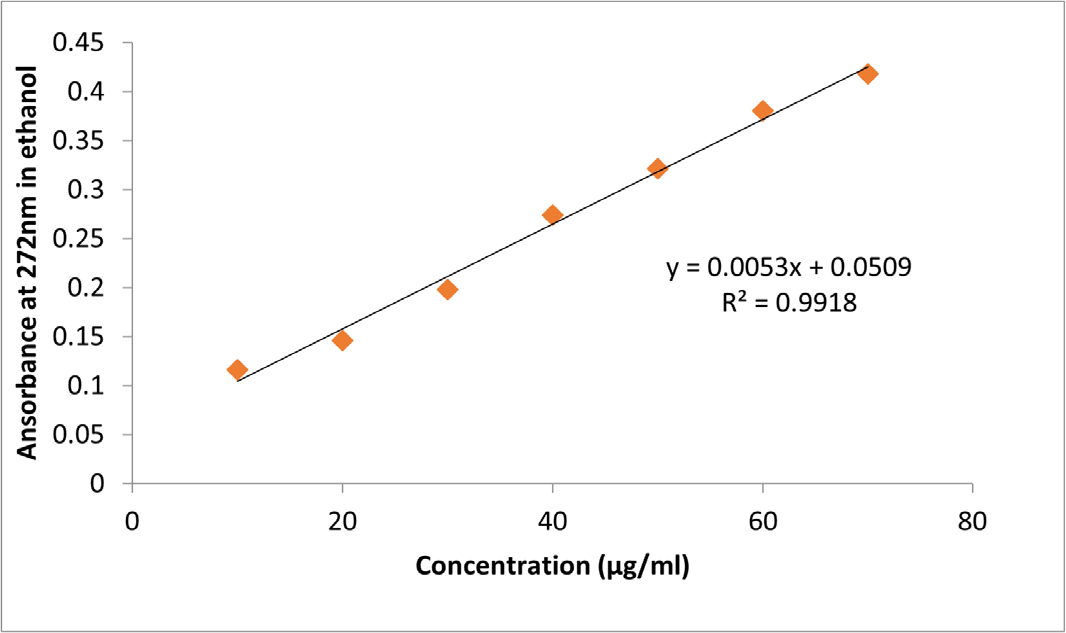
Figure 2:
Calibration curve of Fenoprofen.
Preparation and optimization of Fenoprofen floating tablets
Table 1 showing different batches of Fenoprofen floating tablets. The drug quantity was 600 mg in all batches. The quantity of HPMC K100 M, Xanthan gum and guar gum were vary between 30-50 mg, 20-40 mg and 20-40 mg, respectively. Formulation trails as per Box–Behnken design is shown in Table 5. The response of drug content and floating lag time was recorded as per three variables. The highest drug content was achieved for the formulation F13 i.e., 99.81%, however, floating lag time was highest for F12 i.e., 37.04 sec. The relationship between the independent and dependent variables, as well as all the results from the 17 runs.
Ingredients | F1 | F2 | F3 | F4 | F5 | F6 | F7 | F8 | F9 | F10 | F11 | F12 | F13 | F14 | F15 | F16 | F17 |
---|---|---|---|---|---|---|---|---|---|---|---|---|---|---|---|---|---|
Drug | 600 | 600 | 600 | 600 | 600 | 600 | 600 | 600 | 600 | 600 | 600 | 600 | 600 | 600 | 600 | 600 | 600 |
HPMC K100 M | 30 | 30 | 30 | 40 | 40 | 40 | 40 | 30 | 40 | 40 | 40 | 50 | 50 | 50 | 40 | 50 | 40 |
Xanthan gum | 30 | 40 | 30 | 30 | 20 | 30 | 30 | 20 | 30 | 40 | 20 | 20 | 30 | 30 | 40 | 40 | 30 |
guar gum | 40 | 30 | 20 | 30 | 40 | 30 | 30 | 30 | 30 | 20 | 20 | 30 | 40 | 20 | 40 | 30 | 30 |
Sod. bicarbonate | 30 | 30 | 30 | 30 | 30 | 30 | 30 | 30 | 30 | 30 | 30 | 30 | 30 | 30 | 30 | 30 | 30 |
Citric acid | 15 | 15 | 15 | 15 | 15 | 15 | 15 | 15 | 15 | 15 | 15 | 15 | 15 | 15 | 15 | 15 | 15 |
Magnesium Stearate (2%) | 16 | 16 | 16 | 16 | 16 | 16 | 16 | 16 | 16 | 16 | 16 | 16 | 16 | 16 | 16 | 16 | 16 |
Talc (2%) | 16 | 16 | 16 | 16 | 16 | 16 | 16 | 16 | 16 | 16 | 16 | 16 | 16 | 16 | 16 | 16 | 16 |
Lactose | q.s | q.s | q.s | q.s | q.s | q.s | q.s | q.s | q.s | q.s | q.s | q.s | q.s | q.s | q.s | q.s | q.s |
Total weight | 800 | 800 | 800 | 800 | 800 | 800 | 800 | 800 | 800 | 800 | 800 | 800 | 800 | 800 | 800 | 800 | 800 |
Pre-compression study of Fenoprofen
The regularity of the tablet’s weight could be affected by the powder’s flow, therefore it’s an important factor to consider. Therefore, the number of polymers needed to keep acceptable flow and compaction properties was calculated by measuring the flow properties of the powder blend of the optimized tablet formulation. The angle of repose, bulk density, tapped density, Carr’s index, and Hausner’s ratio were among the pre-compression parameters tested on the powder mix of the formulation. For a pre-compression investigation, 17 distinct Fenoprofen formulations were analyzed by measuring their bulk density, tapped density, Hausner’s ratio, Carr’s ratio, and angle of repose, among other parameters. F12 has the largest bulk density, with 0.39 g/cm3. The highest values for tapped density (0.37 g/ cm3), Hausner’s ratio (0.41 g/cm3), Carr’s ratio (16.08%), and angle of repose (31.47°) were all found in F14 (0.37 g/cm3), F13 (0.41 g/cm3), F8, and F2, respectively. Tapped density, floating lag time, Carr’s index, and Hausner’s ratio are some of the metrics whose results are displayed in Table 2.
Formulation | Bulk density (g/cm3) | Tapped density (g/cm3) | Hausner’s ratio | Carr’s ratio% | Angle of repose |
---|---|---|---|---|---|
F1 | 0.34 | 0.38 | 1.17 | 13.03 | 27.35 |
F2 | 0.32 | 0.46 | 1.16 | 13.01 | 31.47 |
F3 | 0.27 | 0.42 | 1.16 | 12.05 | 28.72 |
F4 | 0.31 | 0.42 | 1.14 | 14.08 | 29.85 |
F5 | 0.32 | 0.43 | 1.15 | 11.01 | 30.15 |
F6 | 0.29 | 0.47 | 1.16 | 12.04 | 30.18 |
F7 | 0.34 | 0.46 | 1.17 | 15.05 | 29.77 |
F8 | 0.34 | 0.41 | 1.14 | 16.08 | 30.07 |
F9 | 0.32 | 0.39 | 1.13 | 14.07 | 29.86 |
F10 | 0.35 | 0.41 | 1.17 | 13.08 | 26.97 |
F11 | 0.38 | 0.37 | 1.14 | 13.06 | 30.58 |
F12 | 0.39 | 0.41 | 1.14 | 14.12 | 29.72 |
F13 | 0.32 | 0.41 | 1.18 | 12.11 | 30.76 |
F14 | 0.37 | 0.47 | 1.16 | 13.01 | 29.42 |
F15 | 0.28 | 0.46 | 1.16 | 12.08 | 25.46 |
F16 | 0.27 | 0.46 | 1.12 | 14.18 | 30.56 |
F17 | 0.29 | 0.44 | 1.14 | 12.17 | 26.39 |
Post-compression evaluation of tablets
Post-compression factors including tablet appearance, weight fluctuation, hardness, thickness, friability, and drug content were compared between formulations. It was determined that the thickness of several formulations ranged from 3.2 mm to 4.4 mm. Hardness between range 3.3 – 4.3 kg/cm2, Friability between 0.68-0.77%, Weight variation between 797.41-804.59 mg. The drug content was found to be between 94.33-99.58% (Table 3). The flow properties of Fenoprofen tablets were determined to be within the range indicative of better flow property based on post-compression metrics such as bulk density, tapped density, Carr’s index, and Hausner’s ratio.
Formulation | Thickness (mm) | Hardness kg/cm2 | Friability (%) | Weight variation (mg) | Drug Content (%) |
---|---|---|---|---|---|
F1 | 3.2 | 4.1 | 0.75 | 797.41 | 96.46 |
F2 | 3.8 | 4.2 | 0.76 | 800.15 | 98.51 |
F3 | 3.3 | 3.9 | 0.75 | 803.24 | 96.71 |
F4 | 4.1 | 3.8 | 0.76 | 801.54 | 97.78 |
F5 | 3.5 | 3.8 | 0.72 | 803.15 | 95.92 |
F6 | 3.4 | 3.8 | 0.68 | 801.61 | 98.78 |
F7 | 3.4 | 4.0 | 0.76 | 800.77 | 97.45 |
F8 | 4.2 | 4.1 | 0.73 | 804.59 | 98.56 |
F9 | 3.6 | 3.5 | 0.72 | 800.57 | 98.01 |
F10 | 4.3 | 3.3 | 0.69 | 798.85 | 99.58 |
F11 | 4.2 | 3.6 | 0.68 | 801.64 | 97.38 |
F12 | 3.9 | 3.4 | 0.71 | 797.54 | 94.33 |
F13 | 4.1 | 4.3 | 0.73 | 802.06 | 97.81 |
F14 | 3.3 | 3.3 | 0.77 | 801.34 | 96.25 |
F15 | 3.3 | 3.6 | 0.74 | 798.24 | 99.13 |
F16 | 3.3 | 3.5 | 0.73 | 800.57 | 97.45 |
F17 | 4.4 | 4.2 | 0.74 | 803.76 | 97.38 |
In vitro buoyancy and floating test
The pills did not dissolve when submerged in a 0.1N HCl solution at 37°C with a pH of 1.2. Buoyancy Lag Time (BLT) and Total Floating Time (TFT) were both found to be satisfactory for the mixture of polymers and excipients. Figure 3 displays that the buoyancy lag times for formulations F1 through F17 ranged from 24.65 sec to 37.04 sec. It was highest for F12 i.e., 37.04 sec. Floating time was ranged between 10.05-12.42 hr. F14 showed floating time of 12.42 hr (Figure 4).
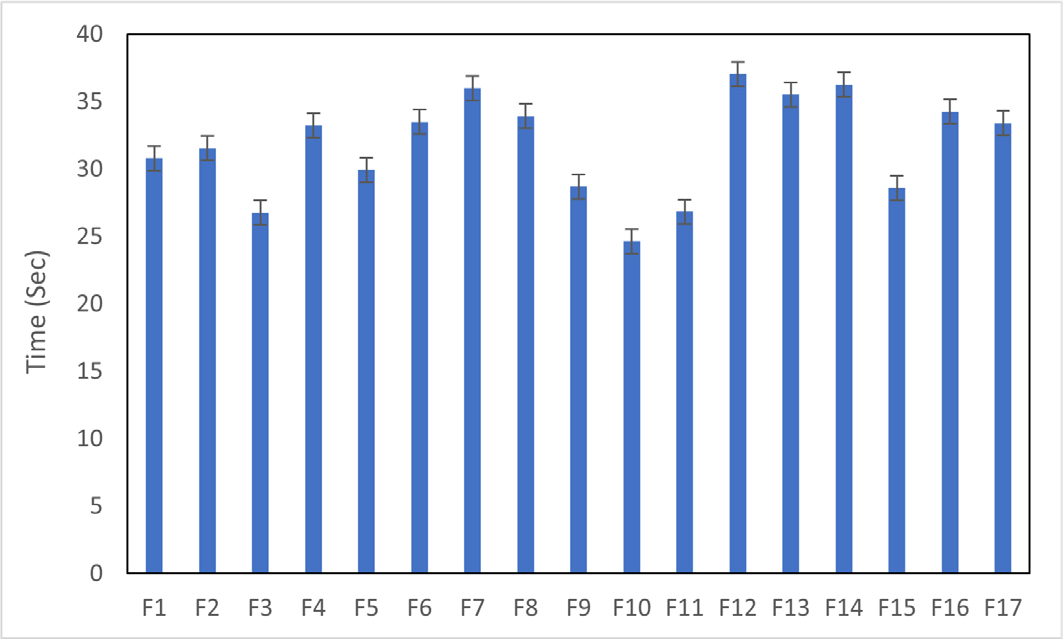
Figure 3:
In vitro buoyancy time of prepared tablets.
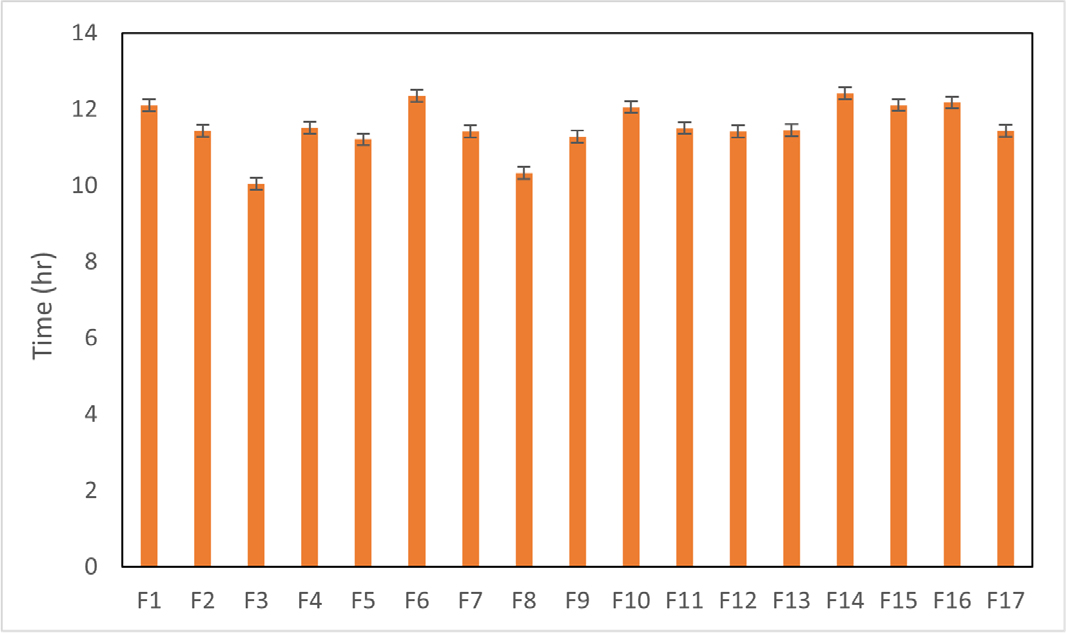
Figure 4:
In vitro floating time of prepared tablets.
In vitro dissolution studies
In vitro dissolution study of prepared tablets in shown in Table 4. The dissolution of tablets was observed for 12 hr. It was found that nearly all the tablet formulations were ~99% dissolved upto 12 hr.
Time (hrs) | F1 | F2 | F3 | F4 | F5 | F6 | F7 | F8 | F9 | F10 | F11 | F12 | F13 | F14 | F15 | F16 | F17 |
---|---|---|---|---|---|---|---|---|---|---|---|---|---|---|---|---|---|
30 min | 12.23 | 18.29 | 19.52 | 23.28 | 19.23 | 19.95 | 19.6 | 19.38 | 15.39 | 18.29 | 18.29 | 19.23 | 16.22 | 18.29 | 16.24 | 12.17 | 18.29 |
1 | 28.36 | 26.19 | 27.42 | 48.91 | 27.13 | 27.85 | 27.5 | 27.28 | 31.52 | 26.19 | 26.19 | 38.13 | 32.35 | 26.19 | 32.37 | 27.21 | 26.19 |
2 | 35.61 | 33.72 | 34.95 | 61.11 | 34.66 | 35.38 | 35.03 | 34.81 | 38.77 | 33.72 | 33.72 | 44.72 | 39.6 | 33.72 | 39.62 | 30.94 | 33.72 |
3 | 41.84 | 41.21 | 42.44 | 33.14 | 42.15 | 42.87 | 42.52 | 42.3 | 45 | 41.21 | 41.21 | 53.21 | 45.83 | 41.21 | 45.85 | 31.96 | 41.21 |
4 | 46.25 | 53.54 | 54.77 | 51.82 | 54.48 | 55.2 | 54.85 | 54.63 | 49.41 | 53.54 | 53.54 | 67.14 | 50.24 | 53.54 | 50.26 | 40.96 | 53.54 |
5 | 52.36 | 61.19 | 62.42 | 67.62 | 62.13 | 62.85 | 62.5 | 62.28 | 55.52 | 61.19 | 61.19 | 71.62 | 56.35 | 61.19 | 56.37 | 46.88 | 61.19 |
6 | 61.84 | 74.34 | 75.57 | 79.45 | 75.28 | 76.02 | 75.65 | 75.43 | 65.26 | 74.34 | 74.34 | 76.54 | 65.83 | 74.34 | 65.85 | 56.23 | 74.34 |
7 | 69.22 | 83.24 | 84.47 | 85.63 | 84.18 | 84.9 | 84.55 | 84.33 | 72.38 | 83.24 | 83.24 | 86.67 | 73.21 | 83.24 | 73.23 | 60.53 | 83.24 |
8 | 79.27 | 91.29 | 92.52 | 89.06 | 92.23 | 92.95 | 92.6 | 92.38 | 82.43 | 91.29 | 91.29 | 89.16 | 83.26 | 91.29 | 83.28 | 68.94 | 91.29 |
9 | 83.51 | 96.27 | 97.5 | 93.86 | 97.21 | 96.93 | 97.58 | 97.36 | 86.67 | 96.27 | 96.27 | 93.46 | 87.5 | 94.27 | 87.52 | 76.83 | 93.27 |
10 | 87.21 | 98.03 | 99.2 | 96.62 | 97.75 | 97.34 | 96.38 | 99.07 | 90.37 | 96.91 | 98.81 | 96.53 | 99.2 | 96.67 | 91.22 | 88.19 | 96.89 |
11 | 97.88 | 99.78 | 99.6 | 99.06 | 98.79 | 98.07 | 98.86 | 99.12 | 98.37 | 99.25 | 99.83 | 99.06 | 99.6 | 97.26 | 99.89 | 9686 | 98.37 |
12 | 99.05 | 99.86 | 99.87 | 99.22 | 99.02 | 99.57 | 98.98 | 99.32 | 99.11 | 99.9 | 99.98 | 99.23 | 99.8 | 99.76 | 99.97 | 99.01 | 99.09 |
Optimization of Fenoprofen tablet formulation
Constraints (goals) were placed on both the dependent (response) and independent (factors) variables to arrive at the optimum formulation. Table displays the limitations on the possible answers and contributing factors. Using DESIGN EXPERT 22.0.2.0 (STAT-EASE), we were able to narrow down the optimal formulation options to a single program. Due to the experimental character of this component mixture, 17 distinct formulation batches have been produced (when counting construction centers). Numerous formulation lots were made, as indicated, and evaluated based on the given replies. Analysis of Variance (ANOVA) is used to compare the model to another model and determine its significance. A positive sign before a factor in a polynomial equation indicates a linear relationship between the response and the factor, while a negative sign indicates the opposite. The relationship between independent and dependent variables was analyzed, along with all the results from 17 separate runs.
Effect of formulation variables on Drug content
When the design formulation findings were fitted into many models, a linear model was significant for drug content (F = 3.45, P = 0.0484). In this model, the drug content was significantly changed by factors A and B (polymers), but not by component C (the amount of Guar gum). This is the model equation: Table 6 shows the formula for calculating the number of drugs in a sample: drug content = +97.46 -0.5500 A + 1.15 B -0.1650 C. Figure 5 depicts a three-dimensional response surface map that helps to explain the impact of components A and B.
Run | Factor 1 A: HPMC K100 M (mg) | Factor 2 B: Xanthan gum (mg) | Factor 3 C: Guar gum (mg) | Response 1 Drug content | Response 2 Floating lag time (Sec.) |
---|---|---|---|---|---|
1 | 30 | 30 | 40 | 96.46 | 30.79 |
2 | 30 | 40 | 30 | 98.51 | 31.54 |
3 | 30 | 30 | 20 | 96.71 | 26.78 |
4 | 40 | 30 | 30 | 97.78 | 33.23 |
5 | 40 | 20 | 40 | 95.2 | 29.93 |
6 | 40 | 30 | 30 | 98.78 | 33.5 |
7 | 40 | 30 | 30 | 97.45 | 36.01 |
8 | 30 | 20 | 30 | 98.56 | 33.92 |
9 | 40 | 30 | 30 | 98.01 | 28.71 |
10 | 40 | 40 | 20 | 99.58 | 24.65 |
11 | 40 | 20 | 20 | 97.38 | 26.83 |
12 | 50 | 20 | 30 | 94.33 | 37.04 |
13 | 50 | 30 | 40 | 97.81 | 35.52 |
14 | 50 | 30 | 20 | 96.25 | 36.27 |
15 | 40 | 40 | 40 | 99.13 | 28.61 |
16 | 50 | 40 | 30 | 97.45 | 34.27 |
17 | 40 | 30 | 30 | 97.38 | 33.41 |
Source | Sum of Squares | df | Mean Square | F-value | p-value | |
---|---|---|---|---|---|---|
Model | 13.22 | 3 | 4.41 | 3.45 | 0.0484 | Significant |
A-HPMC K100 M | 2.42 | 1 | 2.42 | 1.90 | 0.1918 | |
B-Xanthan gum | 10.58 | 1 | 10.58 | 8.29 | 0.0129 | |
C-Guar gum | 0.2178 | 1 | 0.2178 | 0.1707 | 0.6863 | |
Residual | 16.59 | 13 | 1.28 | |||
Lack of Fit | 15.32 | 9 | 1.70 | 5.35 | 0.0606 | Not significant |
Pure Error | 1.27 | 4 | 0.3180 | |||
Cor Total | 29.81 | 16 |
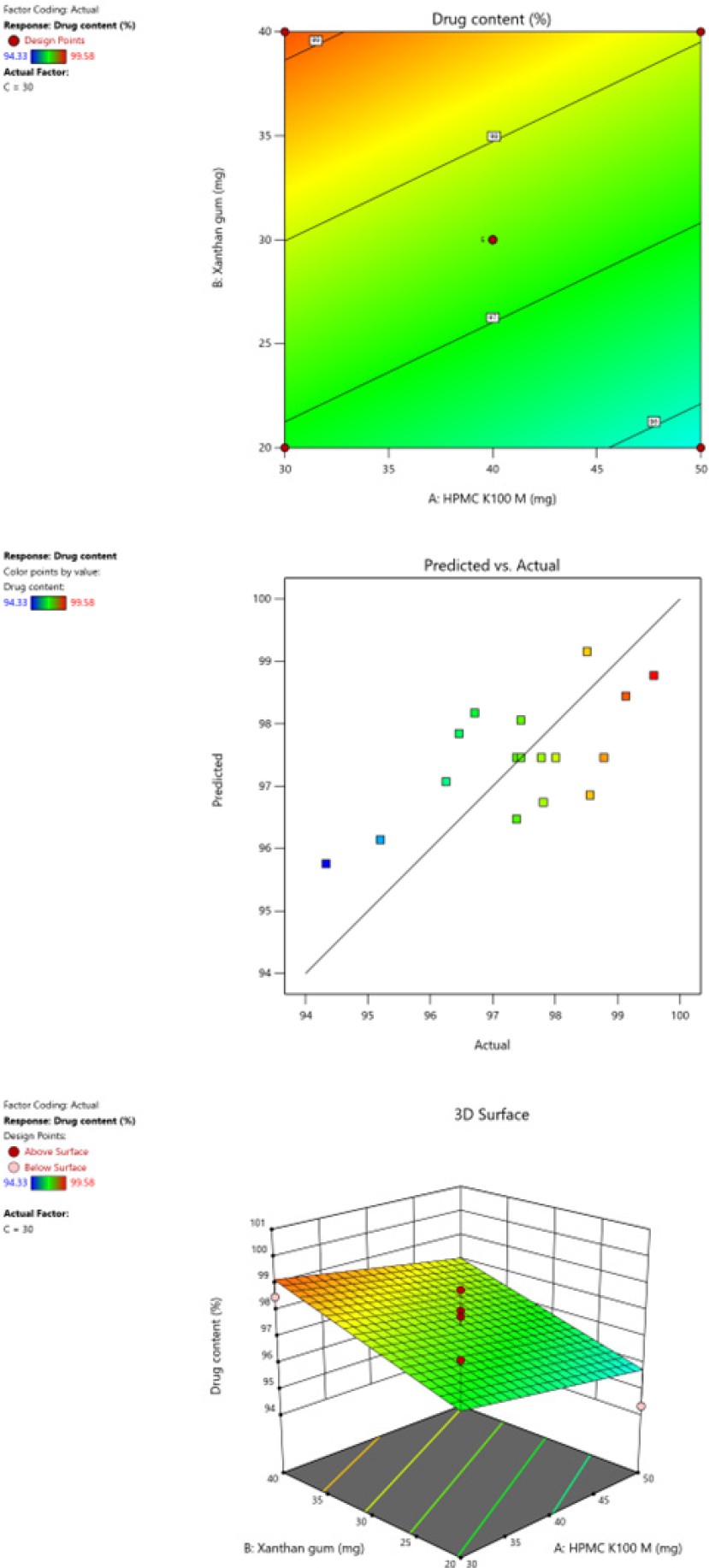
Figure 5:
Response surface plot showing combined effect of xanthan gum and HPMC K100 M on drug content of formulation.
Sum of squares is Type III – Partial
A Model F-value of 3.45 indicates statistical significance. An F-value this high could only arise from random chance 4.84% of the time.
Model terms are statistically significant when their p-values are less than 0.0500. Here, the model term B is particularly important. Model terms are not significant if their p-values are bigger than 0.1000. If your model has a large number of meaningless terms (except those necessary to support hierarchy), you may benefit from performing a model reduction.
A Lack of Fit F-value of 5.35 indicates a 6.06% possibility that such a significant number could be attributed to random chance alone. Inadequate model fit is undesirable. Ibis low likelihood (below 10%) raises some red flags.
Effect of formulation variables on Floating lag time
When the design’s specified formulations were entered into a number of models, it was discovered that the quadratic model was significantly better in predicting the Floating lag time, with a F value of 3.68 and a P value of 0.0500. This is the model equation: Time delay while floating = +32.97 + 2.51 A – 1.08 B + 1.29 C – 0.0975 AB – 1.19 AC + 0.2150 BC + 3.03 A2 – 1.81 B2 -3.66 C2. The analysis of variance data is presented in Table 7. Numerically optimized solutions were produced from the analysis and optimization of these experimental results. A solution was chosen at random from the numerical optimization results, labeled as optimized formulation, and taken into account (Figure 6). Predicted and experimental findings are presented, demonstrating how closely they align. It was found that the observed reaction was quite near to the response predicted by the software.
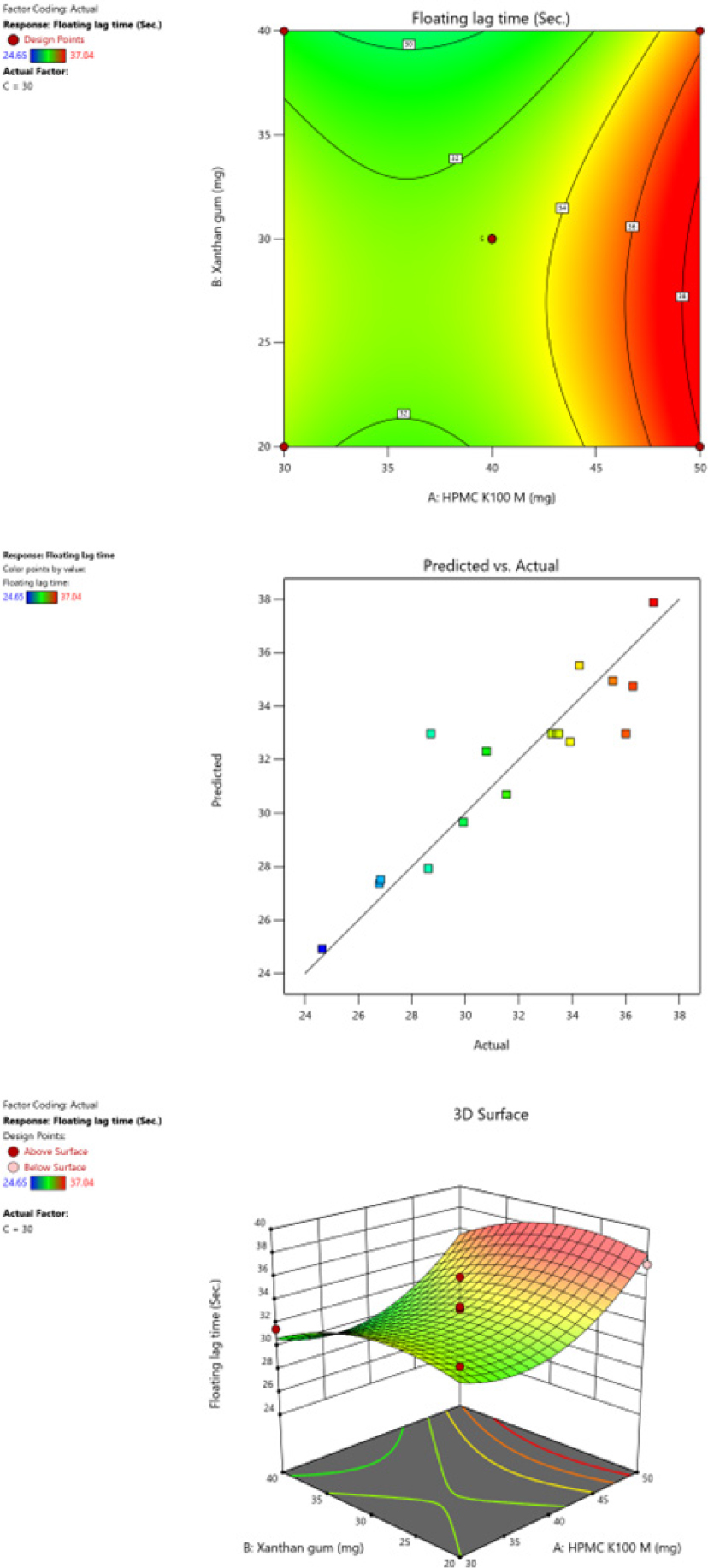
Figure 6:
Response surface plot showing combined effect of HPMC K 100 M and xanthan gum on Floating lag time of formulation.
Source | Sum of Squares | df | Mean Square | F-value | p-value | |
---|---|---|---|---|---|---|
Model | 183.61 | 9 | 20.40 | 3.68 | 0.0500 | Significant |
A-HPMC K100 M | 50.35 | 1 | 50.35 | 9.08 | 0.0196 | |
B-Xanthan gum | 9.35 | 1 | 9.35 | 1.69 | 0.2353 | |
C-Guar gum | 13.31 | 1 | 13.31 | 2.40 | 0.1653 | |
AB | 0.0380 | 1 | 0.0380 | 0.0069 | 0.9363 | |
AC | 5.66 | 1 | 5.66 | 1.02 | 0.3459 | |
BC | 0.1849 | 1 | 0.1849 | 0.0333 | 0.8603 | |
A2 | 38.60 | 1 | 38.60 | 6.96 | 0.0335 | |
B2 | 13.75 | 1 | 13.75 | 2.48 | 0.1594 | |
C2 | 56.39 | 1 | 56.39 | 10.17 | 0.0153 | |
Residual | 38.83 | 7 | 5.55 | |||
Lack of Fit | 10.90 | 3 | 3.63 | 0.5204 | 0.6908 | Not significant |
Pure Error | 27.93 | 4 | 6.98 | |||
Cor Total | 222.45 | 16 |
Sum of squares is Type III – Partial
There is statistical significance in the model, since the F-value is 3.68. A big F-value is extremely unlikely to be the result of random chance; the probability is only 5.00%.
Significance of model terms is indicated by p-values less than 0.0500. A, A2, and C2 all play important roles as model terms here. The model terms are not significant if their p-values are bigger than 0.1000. Model reduction is useful if your model contains a large number of meaningless terms (excluding those necessary to maintain hierarchy).
With an F-value of 0.52 for Lack of Fit, this is not statistically different from the pure error. An F-value for Lack of Fit this high has a 69.08% likelihood of being due to random chance. An insignificant mismatch between the data and the model is ideal.
Evaluation parameter of optimized formulation
After compression, the optimal formulation was tested for quality indicators like tablet color, shape retention, hardness, thickness, friability, disintegration time, and medication content. The capsule-shaped, white pills were a product of careful formulation. The design of each tablet was sleek and sophisticated. The size and shape of the tablets were standardized according to the formulation’s thickness once it had been optimized. The vernier caliper measurements for the entire batch of tablets showed a consistent range of 3.6 mm. The findings suggested that the shape and size of the formulation was consistent throughout. The formulation’s hardness of 4.4 kg/cm2 demonstrates the tablets’ robust mechanical properties. A total of 800 mg was used in the finished pill. Tablets from this formulation have weight discrepancies that are well within IP’s tolerance levels. Every tablet formulation was found to have a friability percentage of 0.74%. The results showed that the mechanical resistance of tablets was high across the board, with no formulation failing to meet the standard by a margin of more than 1%. With a medication content percentage of 98.54%, the formulation is guaranteed to have a constant dose of the active ingredient. Based on the outcomes, it seems the formulation is acceptable as per IP standards. When tablets were immersed in a 0.1N HCl solution at a pH (1.2) temperature of 37°C, they floated and stayed afloat without dissolving, indicating that their formulation was optimized. Buoyancy Lag Time (BLT) and Total Floating Time (TFT) were both satisfactory in the optimized batch containing polymers and excipients.
In vitro drug (dissolution) kinetics study of optimized formulation
In vitro drug release from the best formulation is shown in Table 8. There was a 99.12% medication release up to 12 hr. At 12 hr, the drug’s log cumulative release percentage was 1.996%. The following graphs were generated for the kinetic analysis: cumulative percent drug release versus time (zero order kinetic models); log cumulative percent drug remaining versus time (first order kinetic models); cumulative percent drug release versus square root of time (Higuchi model); log cumulative percent drug release versus log time (Korsmeyer-Peppas model). Figure 7 through Figure 10 displays all plots. Constant drug release from a drug delivery system, regardless of concentration, is described using zero-order kinetic models. The improved formulation’s zero-order graph demonstrated a constant drug release from the tablet; the zero-order model’s R2 value was 0.880. When the rate of release depends on the concentration of the system, a first order kinetic model is used to characterize the release. The first order kinetic model yielded the following results: y = -0.236x + 2.411 R2 = 0.805. The constraints of drug delivery and transportation are modeled after the Higuchi equation. The formula for the Higuchi model was determined to be y = 28.12x + 2.933R2 = 0.992. Korsmeyer and Peppas’s kinetic model produced the following results: y = 1.124x + 0.927 R2 = 0.544. Drug dissolution or release experiments were performed in vitro utilizing specialized equipment. Phosphate buffer, pH 7.4, was used for the in vitro drug dissolution testing of the improved formulation. The projected findings were confirmed by the improved formulation’s 99.12% drug release within 12 hr. The R2 value may be seen in row 36 of the table above. The Highuchi kinetic model was found to have the best match, as measured by the coefficient
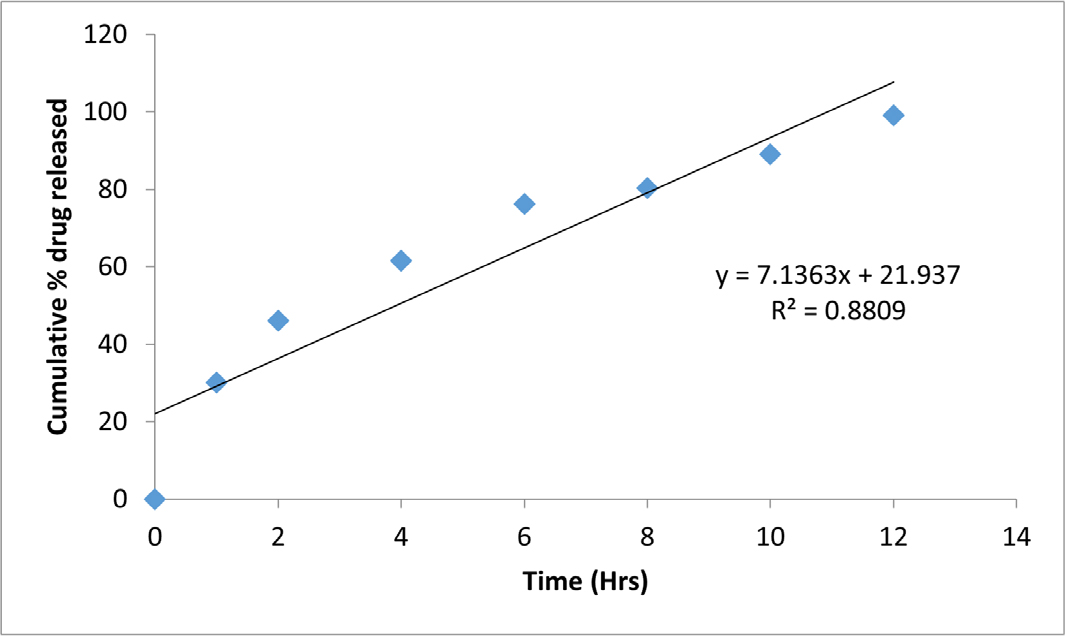
Figure 7:
Zero order kinetic model for fenoprofen tablets.
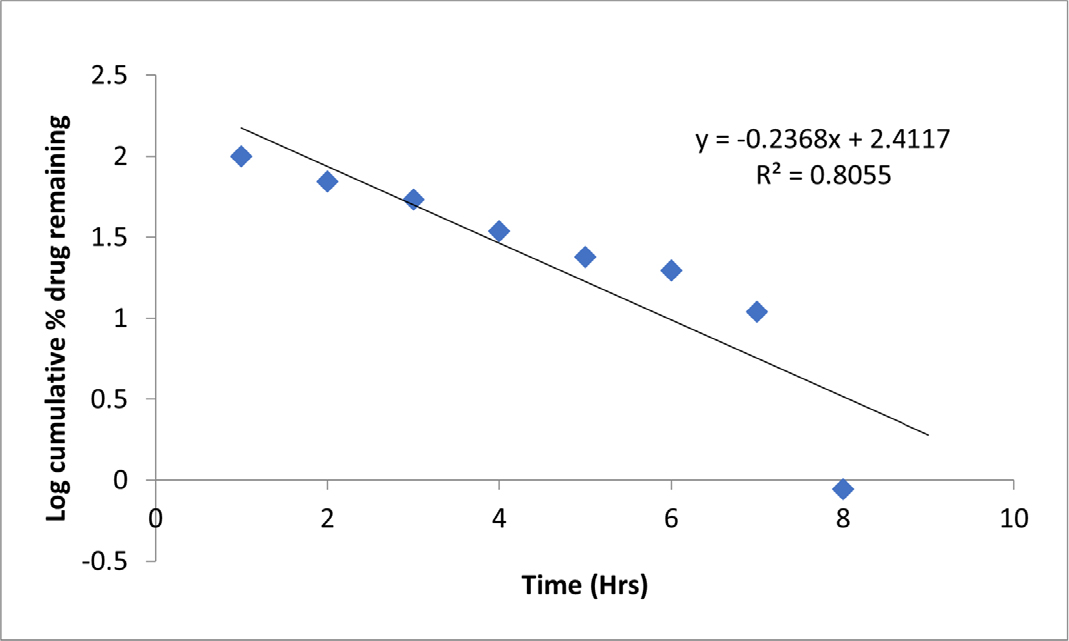
Figure 8:
First Order kinetic model for fenoprofen tablets.
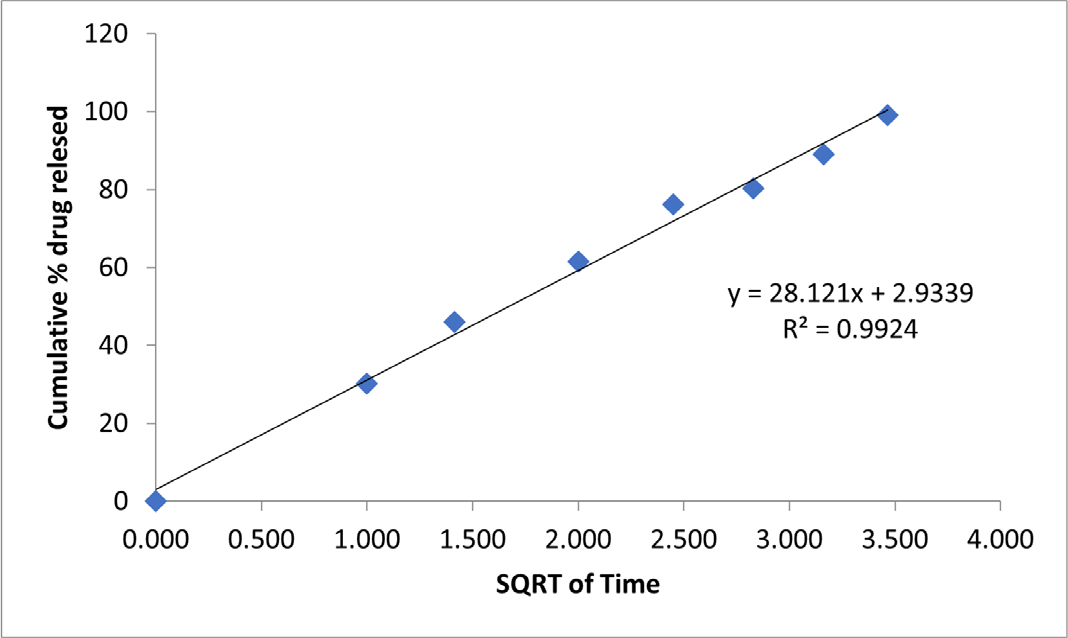
Figure 9:
Higuchi model for fenoprofen tablets.
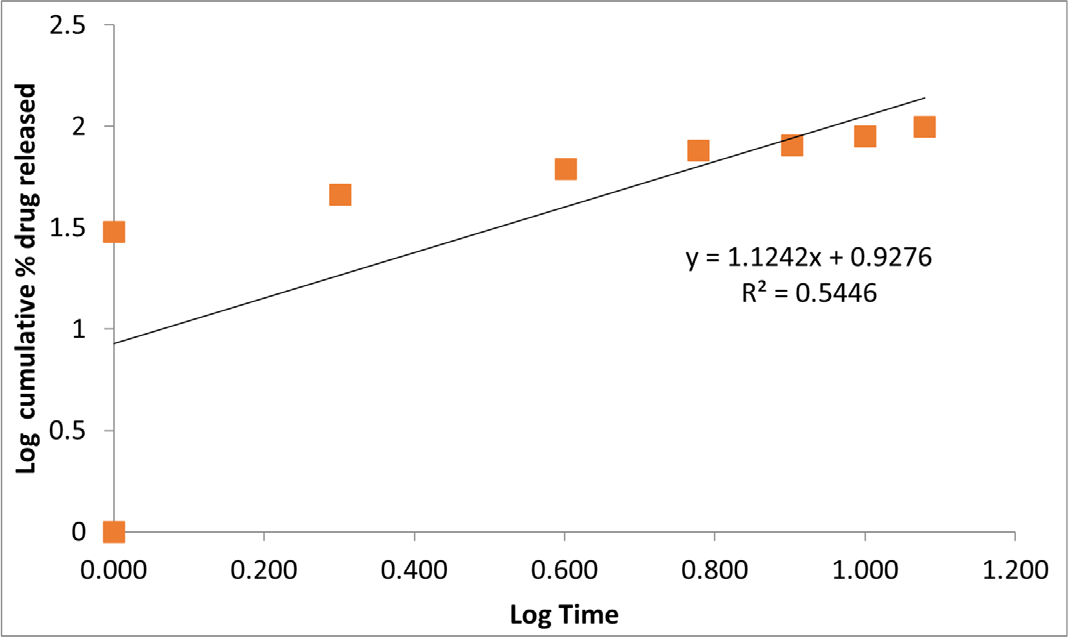
Figure 10:
Korsmeyer peppas for fenoprofen tablets.
Sl. No. | Time (Min.) | cumulative % drug released | % drug remaining | Square root time | log Cumulative % drug remaining | log Cumulative % drug released | log time |
---|---|---|---|---|---|---|---|
1. | 0 | 0 | 100 | 0.000 | 2.000 | 0.000 | 0.000 |
2. | 1 | 30.12 | 69.88 | 1.000 | 1.844 | 1.479 | 0.000 |
3. | 2 | 46.01 | 53.99 | 1.414 | 1.732 | 1.663 | 0.301 |
4. | 4 | 61.56 | 38.44 | 2.000 | 1.585 | 1.789 | 0.602 |
5. | 6 | 76.16 | 23.84 | 2.449 | 1.377 | 1.882 | 0.778 |
6. | 8 | 80.31 | 19.69 | 2.828 | 1.294 | 1.905 | 0.903 |
7. | 10 | 89.08 | 10.92 | 3.162 | 1.038 | 1.950 | 1.000 |
8. | 12 | 99.12 | 0.88 | 3.464 | -0.056 | 1.996 | 1.079 |
Stability study
Optimized Formulation was stability tested for three months at 30°C±2°C and 60% RH and 40°C±2°C and 70 ±5% RH, per ICH recommendations. At accelerated stability conditions, tablets with the optimized formulation were physically and chemically stable for 3 months. Table 9 shows test and other stability study results at various time periods.
Sl. No. | Time (Days) | 30°C±2°C and 60 ± 5% RH | 40°C±2°C and 70 ±5% RH | ||||
---|---|---|---|---|---|---|---|
Drug content (%) | Floating Lag Time (Sec.) | Drug release (%) 12 hr | Drug content (%) | Floating Lag Time (Sec.) | Drug release (%) 12 hr | ||
1. | 0 | 99.02 | 30.27 | 99.12 | 99.02 | 30.27 | 99.12 |
2. | 30 | 98.94 | 30.25 | 99.15 | 98.04 | 30.26 | 99.11 |
3. | 45 | 99.12 | 30.27 | 99.01 | 98.95 | 30.27 | 99.08 |
3. | 60 | 99.00 | 30.18 | 98.97 | 98.98 | 30.18 | 99.97 |
4. | 90 | 99.14 | 30.20 | 99.16 | 99.14 | 30.21 | 99.07 |
CONCLUSION
The direct compression method was used to make fenoprofen floating tablets with HPLC and xanthan gum as polymers at optimum concentrations. The present study found that tablets containing Fenoprofen alone are unable to effectively control its release for 12 hr, but that this problem is solved by combining the drug with polymers, which helped in increasing the viscosity of the formulation in the dissolution medium. In terms of how well each formulation-controlled drug release, it was determined that the F4 formulation, which included 40% HPMC, was the most effective. According to the results, formulation F4 demonstrates the ideal swelling properties and drug release kinetics, particularly in the form of zero-order release and floating behavior.
Cite this article:
Sikarwar GS, Gupta MK. Formulation Development and Optimization of Fenoprofen Floating Tablet Using QbD Approach. Int. J. Pharm. Investigation. 2024;14(1):76-88.
ACKNOWLEDGEMENT
We are thankful to the institute for providing all facilities required during conducting this experiment.
ABBREVIATIONS
QbD | Quality by design |
---|---|
DoE | Design of Experiment |
MP | Melting point |
NDDS | Novel drug delivery system |
GI | Gastrointestinal |
NSAIDs | Nonsteroidal anti-inflammatory drugs |
CE | Cellulose ether |
HPMC | Hydroxy Propyl Methyl Cellulose |
ANOVA | Analysis of variance |
ICH | International Conference on Harmonization |
BLT | Buoyancy lag time |
TFT | Total floating time |
References
- Tushar N, Sonawane PD, Dhangar SD, Patil AZ, Shaikh. Short review on novel dosage form. Asian J Pharm Technol. 2021;11(4):301-3. [Google Scholar]
- Trucillo P. Drug carriers: classification, administration, release profiles, and industrial approach. Processes. 2021;9(3):470 [CrossRef] | [Google Scholar]
- Mahomoodally MF, Sadeer N, Edoo M, Venugopala KN. The potential application of novel drug delivery systems for phytopharmaceuticals and natural extracts-current status and future perspectives. Mini Rev Med Chem. 2021;21(18):2731-46. [PubMed] | [CrossRef] | [Google Scholar]
- Adepu S, Ramakrishna S. Controlled drug delivery systems: current status and future directions. Molecules. 2021;26(19):5905 [PubMed] | [CrossRef] | [Google Scholar]
- Namdev A, Jain D. Floating drug delivery systems: an emerging trend for the treatment of peptic ulcer. Curr Drug Deliv. 2019;16(10):874-86. [PubMed] | [CrossRef] | [Google Scholar]
- Chaudhary S, Dua JS, Prasad DN. Recent development in floating drug delivery system: an overview. J Drug Deliv Ther. 2022;12(1):185-93. [CrossRef] | [Google Scholar]
- Hsu YT, Kao CY, Ho MH, Lee SP. To control floating drug delivery system in a simulated gastric environment by adjusting the Shell layer formulation. Biomater Res. 2021;25(1):31 [PubMed] | [CrossRef] | [Google Scholar]
- Hua S. Advances in oral drug delivery for regional targeting in the gastrointestinal tract – influence of physiological, pathophysiological and pharmaceutical. Front Pharmacol. 2020;11:524 [PubMed] | [CrossRef] | [Google Scholar]
- Traa MX, Derry S, Moore RA. Single dose oral fenoprofen for acute postoperative pain in adults. Cochrane Database Syst Rev. 2011;2011(2) [PubMed] | [CrossRef] | [Google Scholar]
- Narwariya SS, Jain S, Jadon A, Soni M. A review on development and evaluation of mouth dissolving anti-inflammatory tablet containing fenoprofen. AJPTR. 2019;9(2):248-67. [CrossRef] | [Google Scholar]
- Narwariya SS, Jain S. Physicochemical anti-inflammatory effect of Tablet containing fenoprofen. Res J Pharm Technol. 2022;15(10):4413-5. [CrossRef] | [Google Scholar]
- Gupta B, Mishra V, Gharat S, Momin M, Omri A. Cellulosic polymers for enhancing drug bioavailability in ocular drug delivery systems. Pharmaceuticals (Basel). 2021;14(11):1201 [PubMed] | [CrossRef] | [Google Scholar]
- Tudoroiu EE, Dinu-Pîrvu CE, Albu Kaya MG, Popa L, Anuţa V, Prisada RM, et al. An overview of cellulose derivatives-based dressings for wound-healing management. Pharmaceuticals (Basel). 2021;14(12):1215 [PubMed] | [CrossRef] | [Google Scholar]
- Seddiqi H, Oliaei E, Honarkar H, Jin J, Geonzon LC, Bacabac RG, et al. Cellulose and its derivatives: towards biomedical applications. Cellulose. 2021;28(4):1893-931. [CrossRef] | [Google Scholar]
- Borandeh S, van Bochove B, Teotia A, Seppälä J. Polymeric drug delivery systems by additive manufacturing. Adv Drug Deliv Rev. 2021;173:349-73. [PubMed] | [CrossRef] | [Google Scholar]
- Yoshida T, Lai TC, Kwon GS, Sako K. pH- and ion-sensitive polymers for drug delivery. Expert Opin Drug Deliv. 2013;10(11):1497-513. [PubMed] | [CrossRef] | [Google Scholar]
- Li CL, Martini LG, Ford JL, Roberts M. The use of hypromellose in oral drug delivery. J Pharm Pharmacol. 2005;57(5):533-46. [PubMed] | [CrossRef] | [Google Scholar]
- Desu P, Vaishnavi G, Divya K, Lakshmi U, Kumar P. An overview on preformulation studies. 2019:1399-407. [PubMed] | [CrossRef] | [Google Scholar]
- Deepika J, Bhavana AR, Nidhi S. Analytical method development and validation of UV-visible spectrophotometric method for the estimation of saxagliptin in gastric medium. Glob J Pharmaceu Sci. 2020;8(2) [PubMed] | [CrossRef] | [Google Scholar]
- SP , Yamsani M v R. formulation and evaluation of fenoverine floating tablets. Asian J Pharm Clin Res. 2021;14:175-80. [PubMed] | [CrossRef] | [Google Scholar]
- Prajapati S, Patel L, Patel C. Floating matrix tablets of domperidone formulation and optimization using simplex lattice design. Iran J Pharm Res. 2011;10(3):447-55. [PubMed] | [Google Scholar]
- Patil R, Pande V, Sonawane R. Nano and microparticulate chitosan based system for formulation of carvedilol rapid melt Tablet. Adv Pharm Bull. 2015;5(2):169-79. [CrossRef] | [Google Scholar]
- Mohan Sahu C, Margret CR, Vaidya Shivani TDK, Sarvesh S, Ingale SJ, Singhai AK, et al. Design and buoyancy evaluation of floating tablets of diltiazem hydrochloride. Res J Pharm Technol. 2012;5(3):337-41. [CrossRef] | [Google Scholar]
- Hirsch CA, Messenger RJ, Brannon JL. Fenoprofen: drug form selection and preformulation stability studies. J Pharm Sci. 1978;67(2):231-6. [PubMed] | [CrossRef] | [Google Scholar]