ABSTRACT
Objectives
The present investigation was aimed to develop Liposome-Based Gel (LBG) for Eberconazole nitrate. The purpose was to administer the drug at a sustained rate through skin to improve bioavailability for longer period of time.
Materials and Methods
Thin film hydration technique was used for the preparation of EBZ loaded liposomes. Preliminary trials were conducted for the selection of type of lipid and its ratio with cholesterol along with the lipid:drug ratio. EBZ loaded liposomes was optimized by using central composite design with amount of phospholipid, cholesterol and lipid:drug ratio as independent variables along with vesicle size and %Entrapment Efficiency (%EE) as dependent variables. Each formulation was evaluated for vesicle size, polydispersity index, % entrapment efficiency, pH and zeta potential. Further, with an aim to provide enhanced patient compliance the optimized batch of EBZ loaded liposomes was transformed into Liposomal Based Gel (LBG).
Results and Conclusion
Results of all design batches showed nano size of the liposomal vesicles with good dispersion. The optimized batch of EBZ loaded liposome showed a vesicle size of 183.4 nm and 92.4% entrapment efficiency. TEM images of the optimized liposomal formulation showed well separated vesicles with narrow size distribution. The results of ex vivo skin permeation study of the optimized batch of EBZ loaded LBG revealed a remarkable improvement in the dissolution as compared to its conventional formulation. All these concluded LBG as one of the suitable approaches for developing topical formulation of poorly water-soluble drugs like eberconazole nitrate.
INTRODUCTION
The skin, which is the body’s outermost layer, serves as a barrier against pathogens and other foreign objects entering the body as well as shielding the wearer from hazardous environmental stimuli including light, heat, and radiation. The skin’s protective nature makes topical drug administration particularly hard. Hence, a number of unique ways have been adopted to boost percutaneous absorption and improve medication permeability over the skin.1 As a result, carrier systems must be developed to improve penetrability. These innovative drug delivery systems’ key tenets include localization, sustaining a release profile for a predetermined amount of time, and preserving stability at the site of action. Many formulations, including nanovesicles, liposomes, niosomes, micelles, and nanocrystals, were created for this aim. The targeted distribution of drugs to the dermal layer using liposomal formulations offers advantages for the controlled release of molecules with low permeability.2
Liposomes are spherical, nanoscale vesicles made up of a lipid bilayer and water molecules. As a result, a diverse range of active moieties may be encapsulated in liposomes due to their hydrophilic or hydrophobic nature. Liposomes have the advantage of being biocompatible due to their similarity to cellular membranes.3 The liposomes are dispersed in a gel matrix to create liposomal gel, which is semisolid dosage form for external use. A medication can be dispersed well in liposomal gel owing to its highly hydrophilic Three-Dimensional (3-D) network structure. The liposome gel has the benefit of being a liposome carrier in addition to having a distinct solution-gel transition property that makes it simple to create, simple to use, and have a strong affinity for skin tissue.4
Eberconazole Nitrate (EBZ) is an imidazole derivative, used topically in the treatment of superficial fungal infections. EBZ, 1-(2, 4-dichloro-10, 11-dihydro-5H-dibenzo [a, d] cyclohepten-5- yl)-1H-Imidazole nitrate, acts by inhibition of fungal lanosterol 14α-demethylase. This causes changes to its structure and activity, which prevents the fungus from developing. Since the infection is frequently restricted to the outermost layers of skin, topical antifungal medications are useful in the treatment of dermatophytoses. The most popular and preferred first line treatments for localized dermatophytoses are topical medicines with strong local bioavailability.5,6 The main aim was to enhance the permeation rate and improve the stability of EBZ when delivered through the skin.
MATERIALS AND METHODS
Materials
Eberconazole Nitrate was received as gift sample from Precise Chemipharma Pvt. Ltd., Navi Mumbai, India. PL 35% (Soya phosphatidylcholine grade with 35% purity), PL 97% (Soya phosphatidylcholine grade with 97% purity) and Lipoid (PL 90H, PL 90G Germany) were purchased from Himedia, Mumbai, India. Isopropyl alcohol and Chloroform were purchased from Chemdyes Corporation, Rajkot. Cholesterol was purchased from Suvidhinath Laboratories, Vadodara. HPLC grade Methanol and ethanol were purchased from Purvi Enterprises, Ahmedabad. Carbopol 940® was purchased from Alpha chemika, Mumbai. Commercially available Eberconazole cream was procured from local pharmacy for research purpose. Ultrapure distilled water was used all over the procedure. All other chemicals were used of HPLC or analytical grade.
Methods
Drug and Excipient compatibility studies
The compatibility between pure drug, cholesterol, and phosphatidylcholine was detected by FTIR analysis. The aim of this study was to test whether there is any interaction between the lipid carriers and drug. The compatibility was evaluated using an IR spectrophotometer (Bruker ALPHA-II, Japan.) The IR spectra was obtained from 4000 cm-1 to 400 cm-1 after approximately 5 mg of sample was fully mixed with 100 mg of potassium bromide (KBr) IR powder and compressed under vacuum for three minutes at a pressure of around 12,000 psi.7
Preparation of EBZ loaded liposomes
Eberconazole liposomes were prepared by thin film hydration technique using (Buchi, Rotary evaporator). Initially preliminary trials were conducted for the selection of the type of phospholipid, its ratio with cholesterol and also for the selection of the lipid:drug ratio. Accurately weighed amount of drug (EBZ), Phospholipid (PL) and Cholesterol (CH) were dissolved in a solvent mixture (chloroform: ethanol, 2:1 v/v) in 250 mL round-bottom flask. A thin layer of lipid film was formed on the wall of round-bottom flask by evaporating the organic solvent using a rotary film evaporator under reduced pressure. During the preparation process, the instrumental conditions such as temperature (55°C) and rotation speed (100 rpm) were maintained. The flask was kept under vacuum in desiccator to ensure complete removal of residual solvents. The obtained thin lipid film formed on the wall of the flask was then hydrated using phosphate buffer saline pH 7.4 solution at 55 ± 2°C. The resulting vesicle dispersion was vortexed for 5 min and then left undisturbed for 2–3 hr for complete process of hydration. The developed formulations were characterized with respect to carrier-specific parameters.8
Experimental design
In this study, central composite design was employed to optimize Eberconazole Nitrate Liposomes (EBZLs). In order to optimize, the amount of Phospholipid (PL 97%) (mg) (X1), Amount of Cholesterol (mg) (X2), and Lipid: drug ratio (X3) was selected as independent variables. Each factor was set at five levels +α, +1, 0, −1, and –α. fifteen formulations of EBZLs (B1–B15) were designed using Design Expert® software version 7.1.5. % Entrapment efficiency (R1) and vesicle size (R2) were taken as dependent response parameters. The actual values and coded values of different variables are given in Table 1.9 Fifteen formulations of EBZLs (B1–B15) were designed and given in Table 2.
Independent variables | Name of the variables | -α | -1 | 0 | 1 | +α |
---|---|---|---|---|---|---|
X1 | Amount of Phospholipid (PL 97%) (mg) | 200 | 341.49 | 550 | 758.11 | 900 |
X2 | Amount of Cholesterol (mg) | 20 | 34.19 | 55 | 75.81 | 90 |
X3 | Lipid: drug ratio | 10 | 18.11 | 30 | 41.89 | 50 |
Dependent variables | Constrains | |||||
R1 | Entrapment efficiency (%EE) | Maximum | ||||
R1 | Vesicle size (nm) | Minimum |
Selected level of independent and dependent variables.
Batch | X1 | X2 | X3 |
---|---|---|---|
B1 | -1 | -1 | -1 |
B2 | 1 | -1 | -1 |
B3 | -1 | 1 | -1 |
B4 | 1 | 1 | -1 |
B5 | -1 | -1 | 1 |
B6 | 1 | -1 | 1 |
B7 | -1 | 1 | 1 |
B8 | 1 | 1 | 1 |
B9 | -1.68 | 0 | 0 |
B10 | 1.68 | 0 | 0 |
B11 | 0 | -1.68 | 0 |
B12 B13 | 0 0 | 1.68 0 | 0 -1.68 |
B14 | 0 | 0 | 1.68 |
B15 | 0 | 0 | 0 |
Central composite design layout.
Evaluation Parameters
Vesicle size, polydispersity index and zeta potential
The average liposome diameter and the Polydispersity Index (PDI) of liposomes were measured by Malvern (Zetasizer, MALVERN (ZEN1690), UK) at a fixed scattering angle of 90° at 25°C. All formulations were diluted with distilled water (1:10 ratio) to ensure intensity adjustment. The same instrument was used to measure the zeta potential. A measurement of the repelling forces between vesicles is the zeta potential. Larger repulsive forces would prevent vesicles from aggregating and make them more stable.9,10
Percentage Entrapment Efficiency (% EE)
Centrifugation method was used to measure the entrapment efficiency of liposomes dispersions. Liposomes were centrifuged (REMI Elektrotechnik Ltd., India) at 20000 rpm for 1 hr at controlled temperature of 4°C. Supernatant containing unentrapped EBZ was withdrawn and measured UV spectrophotometrically (Shimadzu UV-1800i, Japan) at 208 nm against phosphate buffer (pH 7.4). The amount of EBZ entrapped in liposome was determined as follow:
Where Ci is initial concentration of drug used in formulating the liposomes and Cf is concentration of EBZ in supernant. The entrapment efficiency was obtained by repeating the experiment in triplicate and the values were expressed as mean standard deviation.11
Transmission Electron Microscopy (TEM)
A drop of liposomes dispersion was placed and adsorbed on microscopic carbon coated grids. These grids were subsequently negatively stained with 1% (w/v) aqueous solution of phosphotungstic acid, dried, and viewed under TEM (Talos F200i FEGTEM, Thermofisher, USA) at suitable magnifications, operating at an acceleration voltage of 200 kV.10
Preparation of drug loaded liposomes based gel
The viscosity played a vital role in increasing the drug retention on the skin since liposomes have a very low viscosity and cannot be held at the site of application for an adequate amount of time. Carbopol has been used in this study to increase the viscosity of liposomes, resulting in created liposomes-based gel that offers higher formulation application. Carbopols is regarded as a secure and non-irritating gelling agent, and there have been no reports of human sensitivity to it when applied topically. For this, Carbopol-940 was first dissolved in water and left aside for a sufficient amount of time to allow the polymer chains to fully hydrate and expand. EBZ loaded liposomal gel formulations were prepared by incorporation of optimized liposome dispersions into the structured vehicle of carbopol 940 with gentle mechanical mixing at 25 rpm for 10 min. Triethanolamine was added for neutralization. The same procedure was followed to prepare conventional gel containing EBZ.12,13
Evaluation of liposomes based gel
Physical examination
The EBZ Liposomal gel was prepared by the procedure mentioned and evaluated for color, odor and transparency.13
pH
The pH of the gel was recorded using digital pH meter (EZODO, Taiwan). Initially the pH electrode was calibrated and 1% aqueous solution of liposome-based gel was taken and electrode was immersed into the formulation till the fluctuation in readings stopped and a constant reading was obtained. This procedure was repeated three times and the result were recorded.14
Drug content
Liposomes based gel (1 g) was suspended in 100 mL solution of chloroform: methanol (2:1). The resultant dispersion was kept for 20 min for complete mixing with continuous agitation and filtered through a 0.45 μm membrane filter. The drug content was determined spectrophotometrically at 208 nm. Results were based on triplicate determination.15
Viscosity
Viscosity of prepared gel was measured by using rheometer (Brookfield Engineering laboratories, USA). Approximately 1g of liposome-based gel was taken and rotated at 5 rotations per minute with the help of spindle S62. The temperature was maintained at 25°C. Reading indicates the viscosity of the formulations in cps.16
Spreadability study
It was determined by wooden block and glass slide apparatus. Weights about 20g were added to the pan and the time were noted for upper slide (movable) to separate completely from the fixed slides. Spreadability was then calculated by using the formula:
Where,
S = Spreadability
M = Weight tide to upper slide L = Length of glass slide
T = Time taken to separate the slide completely from each other
Lesser the time taken for the separation of two slides, better the Spreadability.13,16
Ex vivo skin permeation study
Ex vivo skin permeation study was performed by using Franz diffusion cell with an effective diffusion area of 2.0 cm2. The excised goat skin sample was clamped between the donor and the receptor chamber of diffusion cell with the stratum corneum facing the donor chamber. Then, liposomal based gel containing drug equivalent to 10 mg of EBZ was placed onto the donor chamber. The receptor chamber was filled with phosphate buffer pH 7.4 containing 20% v/v PEG 400. The receptor medium was maintained at 37°C ± 0.5°C and stirred at 100 rpm throughout the experiment. For each experiment, 1 mL sample was withdrawn from the donor compartment at pre-determined time intervals (0.25, 0.50, 0.75, 1, 2, 3, 4, 5, 6, 7, 8, 9, 10, 11, 12, 24 hr) and same volume was replaced with fresh phosphate buffer pH 7.4. The absorbance of withdrawn sample was measured after appropriate dilution at 208 nm to estimate concentration of EBZ. Same procedure followed for conventional gel. The experiments were performed in triplicates, and the results were represented as the mean values of three runs. The Korsmeyer-Peppas model, the Higuchi model, the first-order and zero-order mathematical models were utilized to evaluate the kinetics and mechanism of drug release from the liposomes-based gel, and the best-fitted model was chosen based on high correlation coefficient (R) values for the release data.17
Stability study
The tendency of drug leakage, vesicle size, and extent of structural integrity of selected formulation was observed for a period of 3 months by storing the sealed formulation in glass vials (n=3) at two different temperatures, 4-8°C (Refrigerator; RF), 25±2°C (Room temperature; RT). To accomplish this, samples were taken out periodically and their drug content, vesicle size and physical changes were monitored by visual observations.18
RESULTS AND DISCUSSION
Compatibility study
FTIR (Fourier transform infrared) spectra were constructed to check the possible interaction between pure drug and liposomal components. The interpreted data were shown in Table 3. All the major peaks representing the characteristic functional groups seen in the spectra of pure drug (Figure 1a) were also remain present in its physical mixture (Figure 1b) which confirms the compatibility of the EBZ with cholesterol and phospholipid.
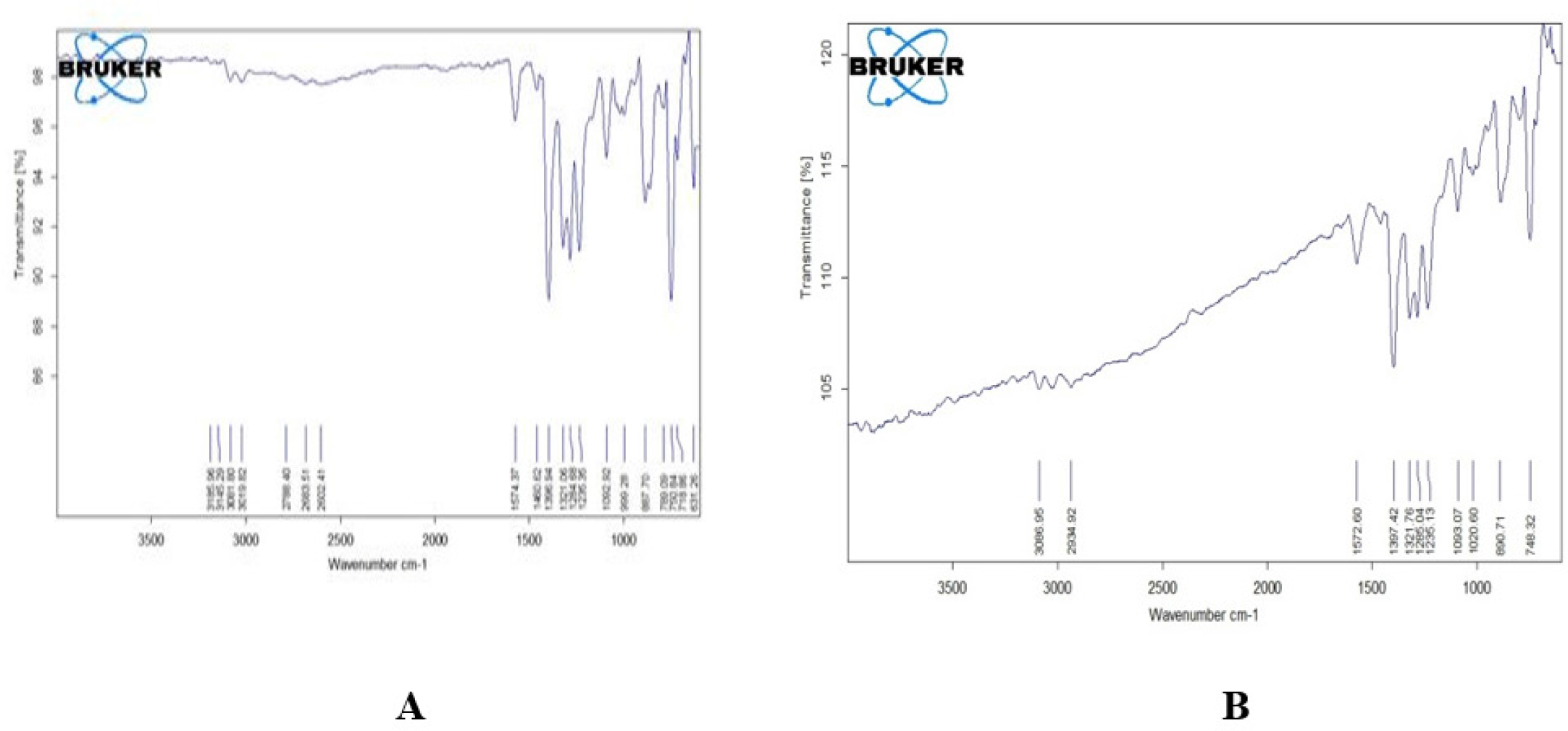
Figure 1:
FTIR spectra of (a) EBZ (b) Physical mixture.
Functional Group | Pure drug (cm-1) | Physical mixture (cm-1) |
---|---|---|
Aromatic C-H stretching | 3081.80 | 3086.80 |
Aromatic C-H bending | 750.84 | 748.32 |
Methyl group C-H bending | 1460.62 | 1397.42 |
Aromatic amine C-N stretching | 1284.68 | 1285.04 |
Cyclic alkenes C=C | 1574.37 | 1572.60 |
stretching | ||
Cyclic alkenes C=C | 887.70 | 890.71 |
bending | ||
Aryl benzene (chloro benzene) | 1092.92 | 1093.07 |
Interpretation of FTIR spectra.
Preliminary trials
EBZ loaded liposomes were prepared by the thin-film hydration method at different Phospholipid to cholesterol (PL: CH) ratio and lipid: drug ratio as shown in Table 4. This method is one of the simplest methods to prepare liposomes on a small scale in a research laboratory. For the selection of a main lipid for further liposome preparation, four different types of phospholipids (PL 35%, PL 97%, PL 90H and PL 90G) were taken in a ratio of 50:50 and 70:30 with Cholesterol (CH). Further to study the effect of lipid: drug ratio, the liposomes were prepared with lipid: drug ratio of 10:1 and 30:1. Likewise total 16 (P1 to P16) batches were prepared and evaluated for visual appearance, shape, and vesicle diameter and % entrapment efficiency. From the results shown in Table 4 it was found that PL 97% in a ratio of 70:30 with cholesterol and lipid: drug ratio of 30:1 form a stable small size vesicle with highest entrapment efficiency (78.8%) compare to all other formulations.
Batch | Type of lipid | PL: Ch | Lipid: drug | Visual Appearance | Shape | %EE | Vesicle diameter |
---|---|---|---|---|---|---|---|
P1 | 10:1 | Stable | Vesicular | 46.3 | Small | ||
P2 | 50:50 | 30:1 | Stable | Irregular, some Vesicular | 53.9 | Small to large medium | |
PL 35% | |||||||
P3 | 70:30 | 10:1 | Stable | Vesicular | 55.3 | Small to medium | |
P4 | 30:1 | Phase separation | — | —- | —- | ||
P5 | PL 97% | 50:50 | 10:1 | Stable | Vesicular | 62.5 | Small |
P6 | 30:1 | Stable | Vesicular | 63.4 | small | ||
P7 | 70:30 | 10:1 | Stable | Vesicular | 70.1 | small, few medium | |
P8 | 30:1 | Stable | Vesicular | 78.8 | Very small | ||
P9 | 50:50 | 10:1 | Stable | Vesicular | 54.4 | small | |
P10 | PL 90H | 30:1 | Phase separation | — | —- | —- | |
P11 | 70:30 | 10:1 | Stable | Vesicular | 59.2 | Small to medium | |
P12 | 30:1 | Stable | Non-Vesicular | 61.1 | Large medium | ||
P13 | PL 90G | 50:50 | 10:1 | Stable | Vesicular | 60.8 | small |
P14 | 30:1 | Phase separation | — | —- | —- | ||
P15 | 70:30 | 10:1 | Stable | Irregular, Few Vesicular | 67.3 | Small | |
P16 | 30:1 | Stable | Vesicular | 69.9 | Small | ||
P17 | 40:1 | Stable | Vesicular | 78.1 | Very small | ||
P18 | 50:1 | Stable | Vesicular | 81.6 | Very small | ||
P19 | PL 97% | 70:30 | 60:1 | Stable | Irregular, some Vesicular | 77.2 | Small to large medium |
P20 | 70:1 | Phase separation | — | — | — |
Preliminary trials for the selection of components and its levels.
Further four batches (P17 to P20) were prepared to study the effect and select the range of lipid: drug ratio. Here PL 97% in a ratio of 70:30 with cholesterol was kept constant for all four batches. From the evaluation results it was observed that as the lipid: drug ratio was increased, % entrapment efficiency was increased, and this effect was significant up to lipid: drug ratio of 50:1. Further increase in the lipid: drug ratio (60:1) lead to decrease in the %EE and above that (70:1) there was a complete phase separation in the liposomal formulation. Hence it was decided to optimize the lipid: drug ratio between 10:1 to 50:1.
PL 35% (Soya phosphatidylcholine grade with 35% purity); PL 97% (Soya phosphatidylcholine grade with 97% purity); PL 90H (Phospholipon 90H with 90% purity); PL 90G (Phospholipon 90G with 90% purity); PL: Ch (ratio of Phospholipid to Cholesterol); %EE (Entrapment efficiency); P1 to P20 (Preliminary trial batch 1 to 20).
Experimental design
Preliminary investigations of the process parameters revealed that factors such as Amount of Phospholipid (mg) (X1), Amount of Cholesterol (mg) (X2) and Lipid: drug ratio (X3) exhibited significant influence on response variables; hence, they were utilized for further systematic studies. Formulation of liposomes was performed while the amount of Phospholipid, Cholesterol and Lipid: drug ratio was changed as independent operational variables, according to a three-factor central composite design. Five different parameters of liposomes were examined: Vesicle size (nm), %EE, Polydispersity index (PDI), Zeta Potential (mV) and pH. Results are shown in Table 5.
Batch | Vesicle size (nm) | %Entrapment Efficiency | Polydispersity index (PDI) | Zeta Potential (mV) | pH |
---|---|---|---|---|---|
B1 | 284.3±5.1 | 70.6±2.7 | 0.049 ± 0.05 | -22.1±1.5 | 6.8±0.4 |
B2 | 327.2±4.6 | 82.1±1.5 | 0.095 ± 0.06 | -27.0±1.2 | 6.9±0.2 |
B3 | 349.6±9.2 | 74.5±2.1 | 0.113 ± 0.04 | -26.5±1.6 | 6.6±0.2 |
B4 | 318.9±8.4 | 70.9±1.1 | 0.214 ± 0.05 | -32.4±1.3 | 6.9±0.3 |
B5 | 293.2±4.1 | 66.3±1.2 | 0.335 ± 0.05 | -26.8±2.0 | 6.7±0.3 |
B6 | 274.1±5.2 | 80.4±2.3 | 0.072 ± 0.06 | -29.1±1.1 | 7.0±0.2 |
B7 | 370.3±6.9 | 74.2±1.3 | 0.342 ± 0.04 | -31.5±2.7 | 6.9±0.1 |
B8 | 331.7±6.7 | 72.7±1.4 | 0.091 ± 0.06 | -23.3±2.7 | 6.6±0.4 |
B9 | 359.1±9.4 | 73.7±1.7 | 0.083 ± 0.05 | -19.3±2.2 | 7.0±0.3 |
B10 | 371.6±7.2 | 70±1.1 | 0.264 ± 0.03 | -28.3±1.7 | 6.9±0.2 |
B11 | 272.3±8.2 | 81.4±1.8 | 0.090 ± 0.06 | -25.3±2.1 | 6.7±0.2 |
B12 | 358.4±6.3 | 73±2.5 | 0.191 ± 0.03 | -26.8±2.5 | 7.1±0.3 |
B13 | 286.5±4.4 | 72±1.6 | 0.345 ± 0.05 | -31.7±1.5 | 6.8±0.3 |
B14 | 249.8±8.7 | 75.8±1.1 | 0.078 ± 0.04 | -28.5±2.0 | 7.0±0.2 |
B15 | 181.6±3.2 | 92.5±1.1 | 0.048 ± 0.02 | -31.3±1.8 | 6.9±0.4 |
Evaluation parameters of design batches of EBZ loaded liposomes.
The data clearly indicate the strong influence of X1, X2 and X3 on selected responses (vesicle size and %EE). The polynomial equations can be used to draw conclusions after considering the magnitude of coefficients and the mathematical sign carried: positive or negative. For vesicle size, coefficient b1, b3, b12, b13, and b23 were found to be insignificant, as p values were more than 0.05 and hence removed from the full model. For %EE, values of b1, b2, b3, b13 and b23 were insignificant and hence removed from the full model (Table 6). Table 7 shows the results of Analysis of Variance (ANOVA) performed to justify the removal of insignificant factors. The high values of correlation coefficients for vesicle size and %EE indicate a good fit. The tabulated (critical) values of F for vesicle size and %EE were found to be 0.606 (df = 5, 5) and 2.167 (df 5, 5) respectively. Moreover, calculated F value [4.093 (vesicle size) and 5.192 (%EE)] was found to be less than tabulated (critical) value, which suggests no significant difference between the full and reduced model.
Coefficient | %EE | Vesicle size (nm) | ||||
---|---|---|---|---|---|---|
FM | RM | FM | RM | |||
B0 | 91.0767 | 91.0765 | 182.809 | 182.8090 | ||
B1 | 0.6437 | — | -1.7955 | — | ||
B2 | -0.5347 | — | 24.650 | 24.6502 | ||
B3 | 0.3359 | — | -5.3028 | — | ||
B12 | -3.4 | -3.4 | -11.637 | — | ||
B13 | 0.925 | — | -8.737 | — | ||
B23 | 0.35 | — | 9.7125 | — | ||
B11 | -6.5895 | -6.5895 | 63.4697 | 63.4697 | ||
B22 | -5.7924 | -5.7923 | 45.7543 | 45.7543 | ||
B33 | -5.8632 | -5.86323 | 29.0310 | 29.0310 |
Summary of Multiple linear regression analysis.
DF | SS | MS | R2 | F | |
---|---|---|---|---|---|
Entrapment efficiency (%EE) | DF=(5,5) | ||||
Regression | Fcal =2.167 | ||||
FM | 9 | 416.733 | 46.303 | 0.9066 | Ftab =5.192 |
RM | 4 | 397.812 | 99.453 | 0.8654 | Fcal < Ftab |
Error | |||||
FM | 5 | 42.910 | 8.582 | ||
RM | 10 | 61.831 | 6.183 | ||
Vesicle size (nm) | DF=(5,5) | ||||
Regression | Fcal =0.606 | ||||
FM | 9 | 37794.16 | 4199.35 | 0.9719 | Ftab =4.093 |
RM | 4 | 34917.62 | 8729.405 | 0.8980 | Fcal<Ftab |
Error | |||||
FM | 5 | 1089.10 | 217.820 | ||
RM | 10 | 3965.649 | 396.56 |
Analysis of variance (ANOVA).
The data of all the 15 batches of central composite design were used to generate interpolated values using Design Expert® software version 7.1.5. Medium levels of X1, X2 and X3 were found to be favorable for lower value of vesicle size and high value of %EE. Multiple linear regression analysis (Table 6) also revealed positive value of coefficient b2 for vesicle size. This indicated that as amount of cholesterol (X2) was increased, there was a significant increase in vesicle size. Similarly positive values were obtained for coefficient b1 and b3 for %EE indicating increasing in entrapment efficiency with increasing in amount of phospholipid and lipid: drug ratio.
Influence of formulation composition factor on vesicle size and %EE
A strong influence of all the independent variables (amount of phospholipid, amount of cholesterol and lipid: drug ratio) was observed on vesicle size and %EE. The lowest value of vesicle size and highest value of %EE was observed with batch B15 which clearly indicates the number of liposomal components decreases the vesicle size and increase the %EE up to some level but after that level there is a negative effect on the required results for liposomes preparation. Response surface plot [Figures 2(a) and 2(b)] for %EE and vesicle size illustrated influence of all three factors.
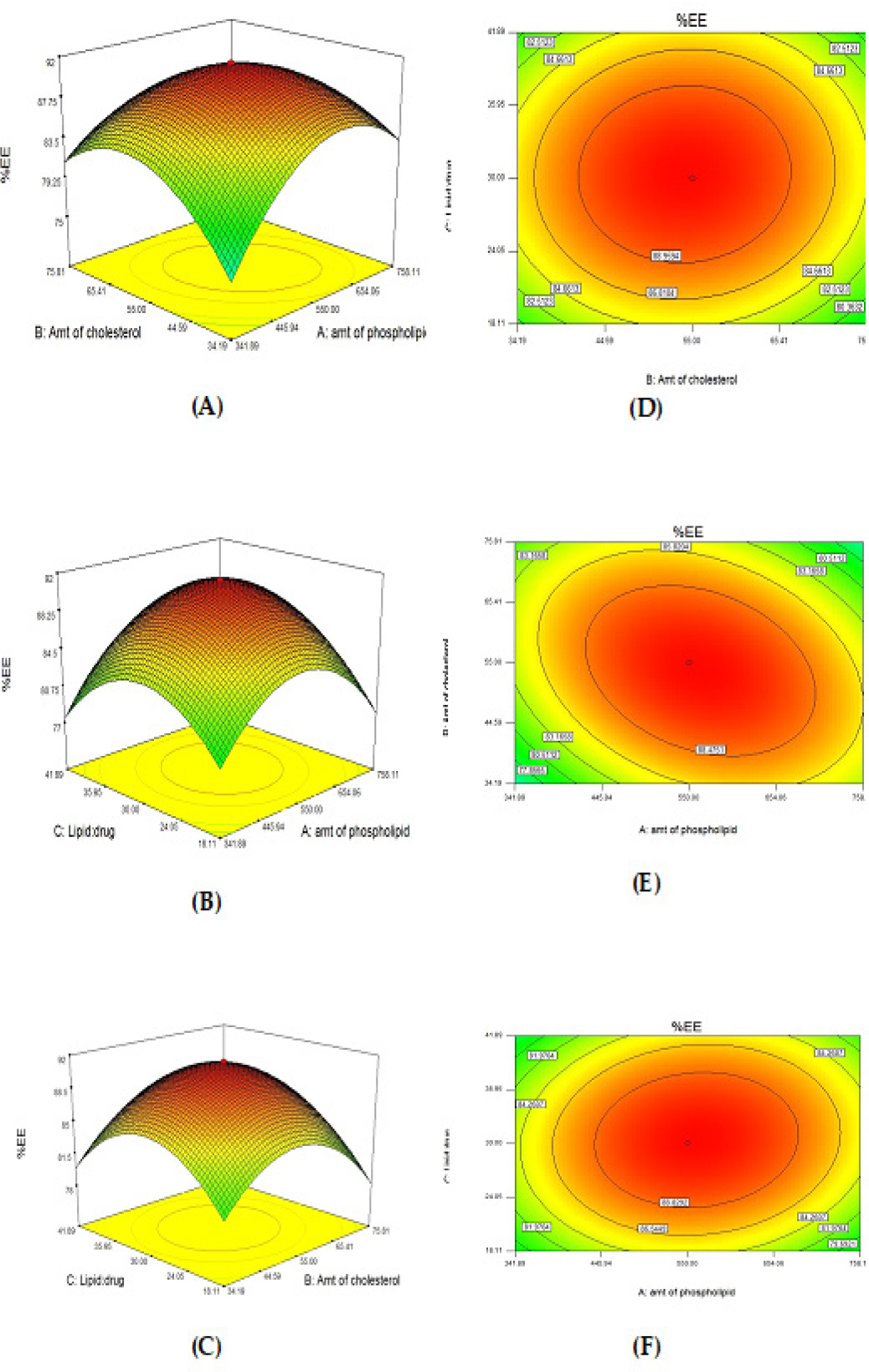
Figure 2(a):
Influence of formulation composition factors represented by response surface plots showing effect of variables A) X1 and X2, B) X1 and X3, C) X2 and X3; contour plots showing effect of variables D) X1 and X2, E) X1 and X3, F) X2 and X3 on %EE (Entrapment efficiency).

Figure 2(b):
Influence of formulation composition factors represented by response surface plots showing effect of variables A) X1 and X2, B) X1 and X3, C) X2 and X3; contour plots showing effect of variables D) X1 and X2, E) X1 andX3, F) X2 and X3 on %EE (Entrapment efficiency).
Optimized batch analysis
Optimized batch was selected on the basis of following criteria: maximum % EE and minimum vesicle size. The result of vesicle size and %EE was compared with that of computed values (Table 8). Overlay plot was generated (Figure 3) by using Design Expert® software version 7.1.5. To confirm the validity of design, optimized batch of liposomes was prepared experimentally using the same procedure. The result of vesicle size and %EE was compared with that of computed values. When both (experimentally obtained and theoretically computed) values were compared, % error was found to be less than <8% for both responses which suggested suitability of design applied.
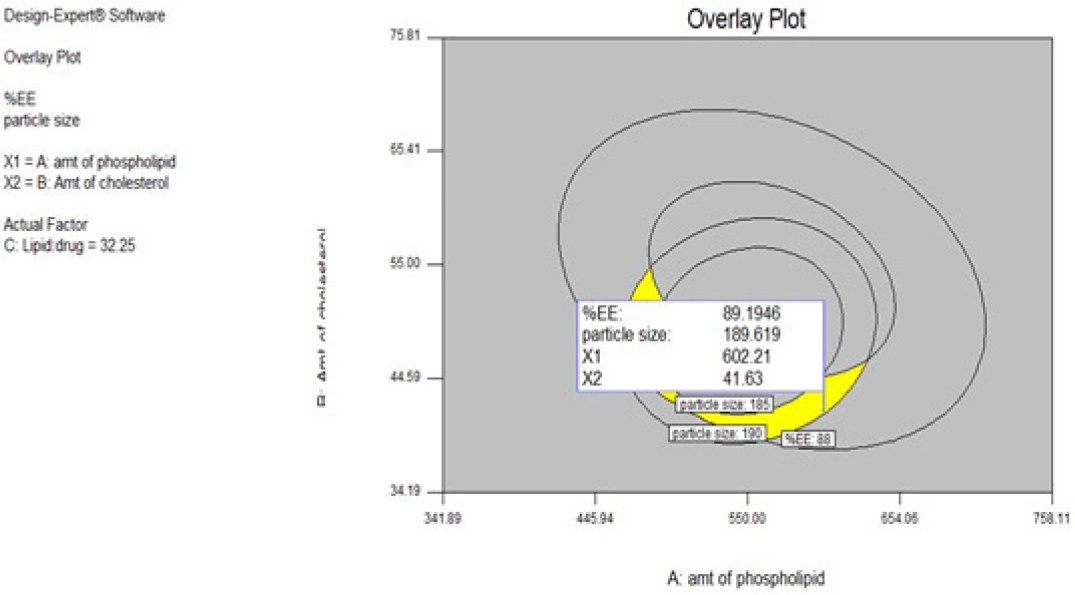
Figure 3:
Overlay plot for optimization of Liposomes dispersion.
Responses | Predicted value | Experimental Valuea | Relative error (%) |
---|---|---|---|
Vesicle size (nm) | 189.62 | 183.44 ± 4.16 | -3.28 |
%EE | 89.20 | 92.44 ± 0.14 | 3.59 |
Results of optimized batch of liposome dispersion.
Evaluation of liposomes
Vesicle size analysis, polydispersity index, pH and Zeta potential measurement
The vesicle size of the liposomes is a crucial factor because it determines the rate and extent of the drug release as well permeation of drug through the skin. The vesicle size of the liposomes of all design batches was shown in Table 1. The vesicle size of optimized batch of liposome dispersion was found to be 183.4 ± 4.16 nm (Figure 4). This low value of vesicle size would definitely provide larger surface area leading to ease in drug transfer through the SC (Stratum Corneum) of skin. The Polydispersity Index (PDI) indicates the uniformity of vesicle size distribution within the formulation. The PDI value of optimized batch of liposome dispersion was found to be 0.459 indicating uniformity of globule size within the liposomes and also indicate that the liposomes were monodispersed to polydispersed. The zeta potential of all batches was found to be in the ranges between -19.3±2.2 mV and -32.4±1.3 mV. Since there were no positively charged molecules present, the negative charge of each formulation demonstrated the relationship of soya lecithin molecules at the liposomal surface. The zeta potential of optimized batch of liposome dispersion was found to be -30.5 ± 0.14 mV which justify the stability of the optimized formulation.19 pH values of all batches were found to be nearer to the skin pH (7.4) which is very important in accordance to the skin irritation in topical formulation. The pH of optimized batch of liposome dispersion was found to be 6.8±0.3.
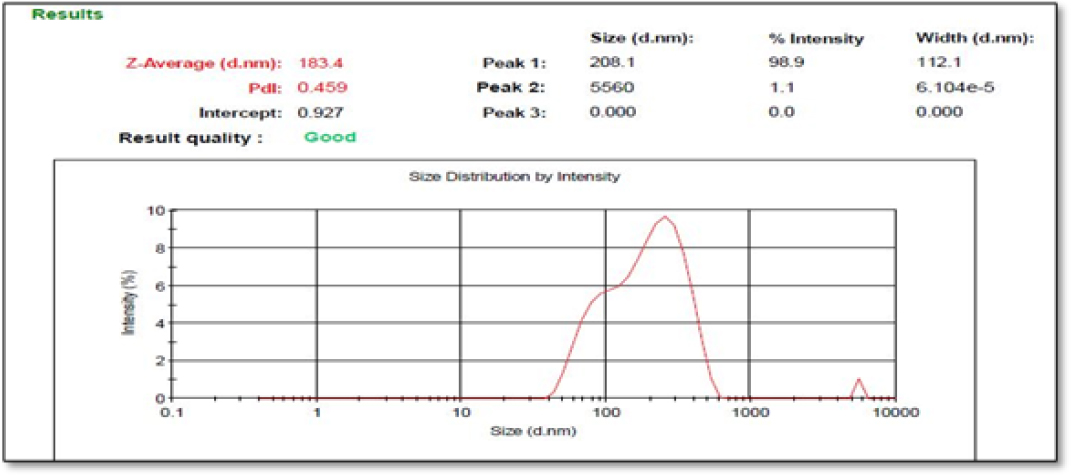
Figure 4:
Vesicle size analysis of optimized liposomal formulation.
%EE
The determination of %EE is a crucial factor when it comes to liposomes because it might have an impact on the medication release and skin penetration. In the present study, the observed %EE for all design batches were in the range of 66.30–92.5%. The %EE of optimized batch of liposomes dispersion was found to be 92.4 ± 0.14%.
Transmission Electron Microscopy (TEM)
To examine the shape of the optimized liposomal formulation, TEM study was performed. The outcomes are shown in Figure 5. The well-separated vesicles with narrow size distribution are visible in the TEM image of the optimized liposomal formulation with EBZ loading. The absence of aggregation is depicted in Figure 5. All of the visible vesicles were smaller than 200 nm, making them very well suited for topical application.
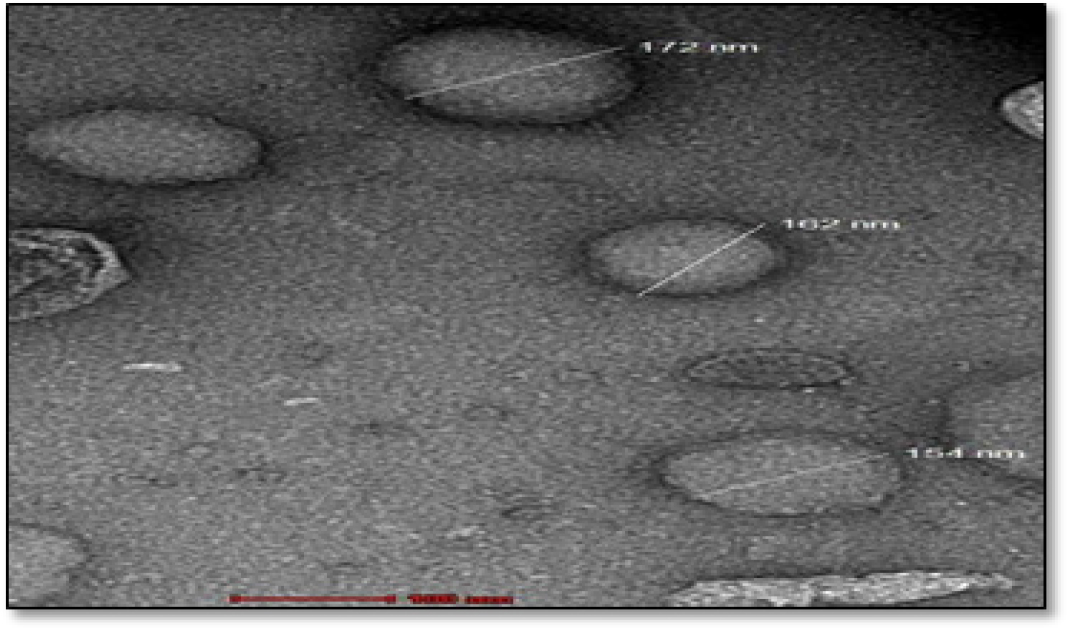
Figure 5:
TEM study of EBZ loaded liposomal formulation
Preparation of drug loaded liposomes based gel
Liposomes are challenging to apply to skin because of their decreased viscosity. Therefore, they are tried to be gelled using appropriate gelling agent for the ease of application. The amount of gelling agent used in gel preparation was also optimized.
The effects of three different Carbopol 940 concentrations were examined. Carbopol 940 was found to make a very hard, stiff gel at a concentration of 1.5% but was unable to produce an acceptable gel at a concentration of 0.5%. Only Carbopol 940 at a concentration of 1% w/w was able to produce gel (Figure 6) consistency without affecting the EBZ liposomes’ architecture and thicken the liposomes.
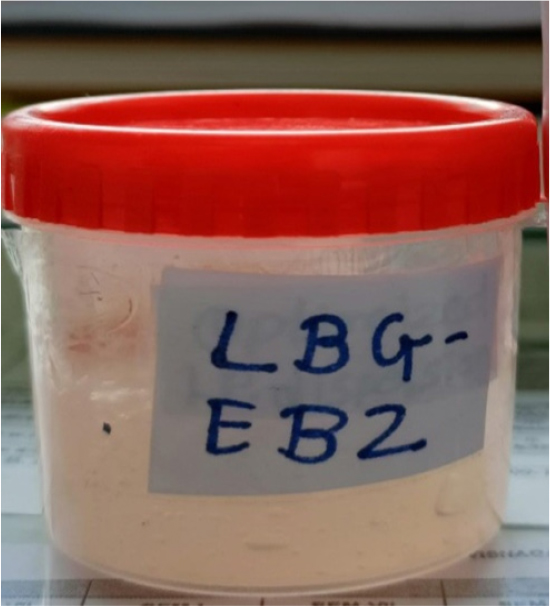
Figure 6:
1% w/w Liposome Based Gel of Eberconazole Nitrate.
Evaluation of Liposomes Based Gel (LBG)
The gels prepared were translucent and homogenous with absence of lumps. Due to the presence of gelling agent the viscosity for the LBG got enhanced as compared to liposomes. The optimized batch of EBZ loaded LBG showed the value of viscosity as 174.41 cps and that of conventional gel was 261.21 cps. The percentage drug content of the LBG was found to be 98.92 ±0.54% and that of conventional gel was 86.78 ±1.25%. pH measurements of LBG system were 6.6 ±0.28 and that of conventional gel was 6.9±0.4. Therefore, the pH of both prepared gels was within the required range and was considered to be safe and non-irritant for topical application. The Spreadability is important for uniform and ease of application of topical preparation from patient compliance point of view. Spreadability of LBG was 33.96 gm.cm/sec and that of conventional gel was 14.88 gm.cm/sec. It indicates that LBG was easily spreadable with small amount of shear as compared to conventional gel.4
Ex vivo skin permeation study
The ex vivo skin permeation profiles of EBZ loaded liposomal based gel and conventional gel performed through excised skin of goat were shown in Figure 7. A steady increase of EBZ concentration in the receptor chambers with time was observed which support the sustained release of drug at site of application. The significantly higher value of drug release after 24 hr 77.55% for the optimized batch of EBZ loaded LBG as compared to its corresponding conventional gel 29.35% suggested marked improvement in release rate. The better penetration associated with LBG was might be due to the presence of phospholipid-constituents which modified the skin layer and enhanced the drug permeation, whereas in the case of conventional gel this form of interaction was not present. Thus, this ex vivo study for 24 hr revealed that the prepared LBG was better in facilitating the drug penetration and sustained release of EBZ.8,20,21 Kinetic modelling of drug release data was investigated to analyze the drug release mechanism from the dosage form. The R2 value of zero-order (Figure 8), first order, Higuchi and Korsmeyer-Peppas model were found to be 0.967, 0.714, 0.894 and 0.957 respectively. The zero-order shows the best fit model with the highest R2 value.22
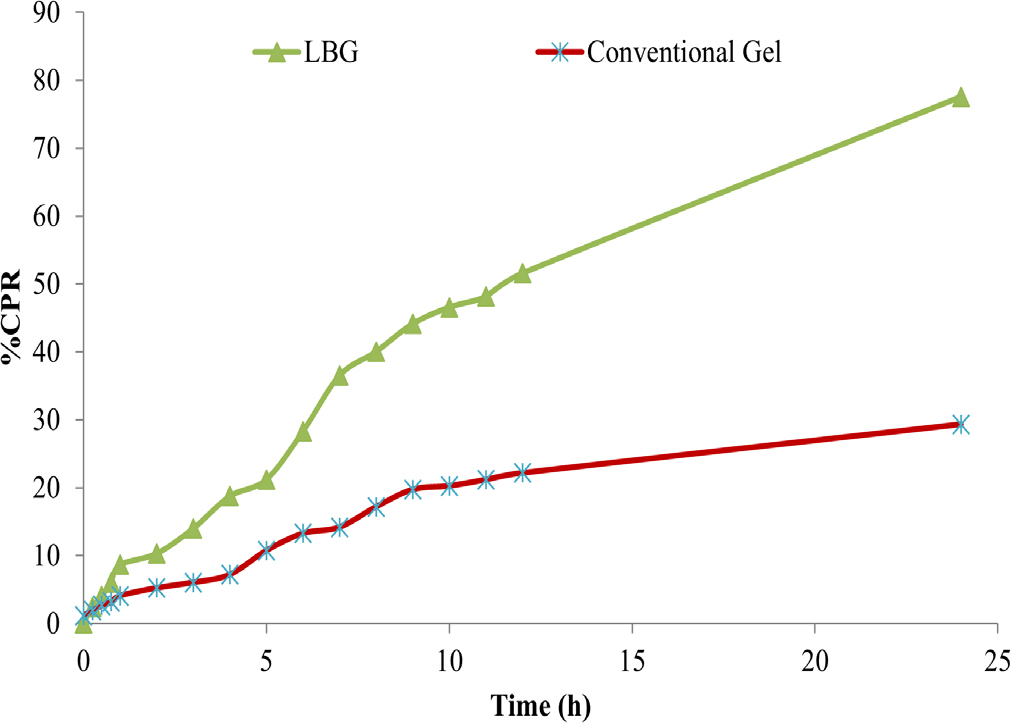
Figure 7:
Percentage cumulative amount of Eberconazole nitrate permeated through goat skin over a period of 24 hr.
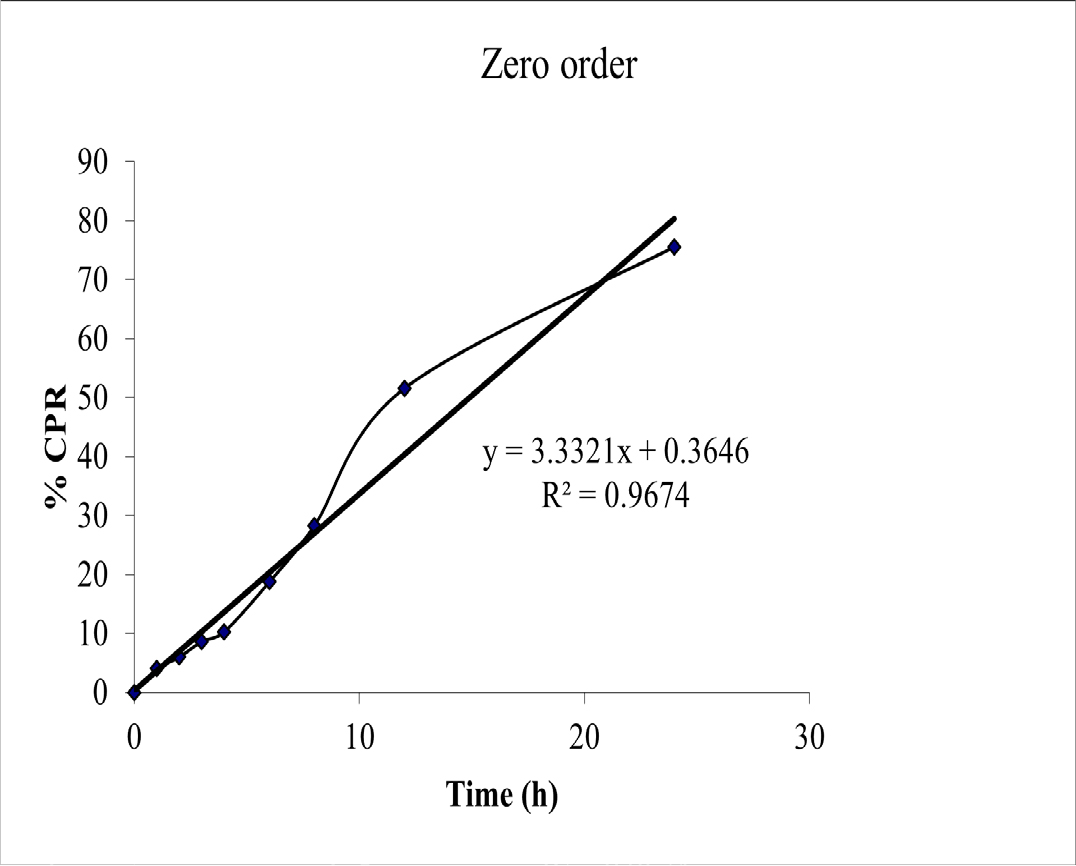
Figure 8:
Kinetic modeling of drug release data of Eberconazole nitrate loaded LBG.
Stability study
The point of stability testing is to give proof on how the medicinal product contents Active Pharmaceutical Fixing (API) changes regarding time affected by various environmental variables. Optimized liposomes and LBG were found to be stable as both the formulations do not exhibit concentration change in drug, aggregation, vesicle disruption, phase separation and organoleptic characteristics.
CONCLUSION
The present study concluded the suitability of experimental design for design and development of liposomes as carriers for topical delivery of poorly water-soluble drug, EBZ. Independent variables of process parameters such as amount of phospholipid, cholesterol and lipid: drug ratio had an intense effect on the vesicle size and entrapment efficiency. The optimized batch of liposomal formulation was found to have nano sized vesicles with good morphological characters. The EBZ loaded liposomal formulation was successfully converted to gel using Carbopol 940 as gelling agent. The marked improvement in the ex vivo investigation compared to its conventional gel formulation is highly attributed to the reduction in the size of vesicles with increase in effective surface area and high entrapment efficiency. However, it further requires in vivo investigations before commercialization of the formulation.
References
- Shukla T, Upmanyu N, Agrawal M, Saraf S, Saraf S, Alexander A., et al. Biomedical applications of microemulsion through dermal and transdermal route. Biomed Pharmacother. 2018;108:1477-94. [PubMed] | [CrossRef] | [Google Scholar]
- Marwah M, Badhan RKS, Lowry D.. Development of a novel polymer-based carrier for deformable liposomes for the controlled dermal delivery of naringenin. J Liposome Res. 2022;32(2):181-94. [PubMed] | [CrossRef] | [Google Scholar]
- Eroğlu İ, Aslan M, Yaman Ü, Gultekinoglu M, Çalamak S, Kart D, et al. Liposome-based combination therapy for acne treatment. J Liposome Res. 2020;30(3):263-73. [PubMed] | [CrossRef] | [Google Scholar]
- Shi J, Ma F, Wang X, Wang F, Liao H.. Formulation of liposomes gels of paeonol for transdermal drug delivery by Box-Behnken statistical design. J Liposome Res. 2012;22(4):270-8. [PubMed] | [CrossRef] | [Google Scholar]
- Moodahadu-Bangera LS, Martis J, Mittal R, Krishnankutty B, Kumar N, Bellary S, et al. Eberconazole-pharmacological and clinical review. Indian J Dermatol Venereol Leprol. 2012;78(2):217-22. [PubMed] | [CrossRef] | [Google Scholar]
- Vamsi Krishna M, Ali Mahgoub Idris S, Madhavi G, Jalachandra Reddy B, Sowhardhra M, Gowri Sankar D, et al. Study on dissociation equilibria of eberconazole nitrate in micellar media by spectrophotometry. Int J Pharm Chem.. 2019;5(5):48 [CrossRef] | [Google Scholar]
- . Design, formulation, and characterization of liposomal-encapsulated gel for transdermal delivery of fluconazole. Asian J Pharm Clin Res.. 2018;11(8):417-24. [CrossRef] | [Google Scholar]
- Wadhwa S, Singh B, Sharma G, Raza K, Katare OP. Liposomal fusidic acid as a potential delivery system: a new paradigm in the treatment of chronic plaque psoriasis. Drug Deliv. 2016;23(4):1204-13. [PubMed] | [CrossRef] | [Google Scholar]
- Keshri L, Pathak K.. Development of thermodynamically stable nanostructured lipid carrier system using central composite design for zero order permeation of econazole nitrate through epidermis. Pharm Dev Technol. 2013;18(3):634-44. [PubMed] | [CrossRef] | [Google Scholar]
- Sabeti B, Noordine MI, Javar HA, Davoudi ET, Kadivar A. Characterization of diclofenac liposomes formulated with palm oil fractions. Trop J Pharm Res.. 2014;13(2):185-90. [CrossRef] | [Google Scholar]
- Mitkari BV, Korde SA, Mahadik KR, Kokare CR. Formulation and evaluation of topical liposomal gel for fluconazole. Indian J Pharm Educ Res.. 2010;44(4):324-33. [CrossRef] | [Google Scholar]
- Sasikala A, Tarakeswari VI. Anti-inflammatory flexible liposomal gel for treatment of rheumatoid arthritis. J Pharm Adv Res.. 2021;4(8):1334-45. [CrossRef] | [Google Scholar]
- Singh AS, Vengurlekar PR, Rathod SU. Design, development and characterization of liposomal neem gel. Int J Pharm Sci Res.. 2014;5(4):140-8. [CrossRef] | [Google Scholar]
- Dandagi PM, Pandey P, Gadad AP, Mastiholimath VS. Formulation and evaluation of microemulsion based luliconazole gel for topical delivery. Indian J Pharm Educ Res.. 2020;54(2):293-301. [CrossRef] | [Google Scholar]
- Shankar D, Gajanan S, Suresh J, Dushyant G. Formulation and evaluation of luliconazole emulgel for topical drug delivery. Int Res J Sci Eng.. 2018;3:85-9. [CrossRef] | [Google Scholar]
- Kaur LP. Topical gel: a recent approach for novel drug delivery. Asian J Biomed Pharm Sci.. 2013;3(17):1 [CrossRef] | [Google Scholar]
- Rao S, Barot T, Rajesh KS, Jha LL. Formulation, optimization and evaluation of microemulsion based gel of butenafine hydrochloride for topical delivery by using simplex lattice mixture design. J Pharm Investig.. 2016;46(1):1-12. [CrossRef] | [Google Scholar]
- Shishu N, Aggarwal N.. Preparation of hydrogels of griseofulvin for dermal application. Int J Pharm. 2006;326(1-2):20-4. [PubMed] | [CrossRef] | [Google Scholar]
- Amin SG, Shah DA, Dave RH. Formulation and evaluation of liposomes of fenofibrate prepared by thin film hydration technique. Int J Pharm Sci Res.. 2018;9(9):3621-7. [PubMed] | [CrossRef] | [Google Scholar]
- Wang W, Shu GF, Lu KJ Xu XL, Sun MC, Qi J. Flexible liposomal gel dual-loaded with all-trans retinoic acid and betamethasone for enhanced therapeutic efficiency of psoriasis. J Nanobiotechnology. 2020;18(1):1-4. [PubMed] | [CrossRef] | [Google Scholar]
- Dragicevic-Curic N, Winter S, Stupar M, Milic J, Krajišnik D, Gitter B, et al. Temoporfin-loaded liposomal gels: viscoelastic properties and skin penetration. Int J Pharm.. 2009;373(1-2):77-84. [PubMed] | [CrossRef] | [Google Scholar]
- Vani GN, Alagusundaram M, Chandrasekar KB. Formulation and optimization and characterization of olanzapine liposome. Int J Appl Pharm.. 2021;13(5):109-14. [CrossRef] | [Google Scholar]