Contents
ABSTRACT
The upgrading of useful biosensors having advantages in disease monitoring besides detection has been sped up by the demand for quick, cheap, portable, easy screening procedures. Bioanalytical devices such as Biosensors have a number of distinguishing benefits over conventional analytical techniques, including high accuracy and specificity, ease of handling, economic friendly, and the potential for downsizing and mass production. With an emphasis on electrochemical and optical sensors, this study examines current developments in the design, performance, and applications of biosensors. Due to its advantages of high sensitivity, specificity, and rapid analysis, electrochemical sensors have demonstrated a wide range of applications in biological detection whereas the optical biosensors covered in this article have enabled diagnosis of SARS-CoV-2, which highlighted the importance of creating swift and extremely sensitive diagnostic methods for quickly identifying infected patients using LSPR, SERS, FET, EC biosensors.
INTRODUCTION
In the biomedical, environmental, and food quality control, agricultural, and pharmaceutical industries, enzyme-based biosensors have become an important tool for qualitative and quantitative measurement of a variety of target analytes. In the healthcare field, biosensors have advantages such as quick, extra-laboratory analysis and significantly lower sample costs. They provide considerable advantages over traditional analytical approaches, including, accuracy, quicker sample preparation, and ease of handling, since they can be useful in the disease detection.
An all-encompassing definition of a biosensor is “a self-contained analytical device that integrates a biological component with a physicochemical device for the detection of an analyte of biological importance”. It contains a transducer that can turn this connection into a measurable signal and a biological recognition element that can specifically engage with a target molecule.
Chemical biosensors work by detecting the biological component that is unique to the analyte and steady when used and stored normally. Biosensors have utilized a wide range of recognition components, including receptors, nucleic acids, entire cells, and many enzymatic classes.
Typically, biosensors are categorized based on the transduction technique they employ. A huge number of chemical, physical, or biological interactions are transformed into an electrical signal by the transducer in biosensors. This has led to the development and characterization of optical, calorimetric, or acoustic biosensors; nonetheless, the electrochemical properties of transducers and analytes are what make biosensors so popular.
A small number of biosensors are currently on the market (such as sensors for diabetes monitoring), whereas the majority are still in the development phase. Traditional enzyme-based biosensing designs for in vivo detection have been covered in a number of excellent reviews. These designs include optical and electrochemical sensors.1–4
ELECTROCHEMICAL BIOSENSORS FOR BIOMEDICAL ANALYSIS
The electrochemical biosensor keeps track of the electrochemical signal that results from the interaction of the target molecules (analyte molecules) and the detecting probe. Different electrochemical techniques, such as potentiometry, amperometry, electrochemical impedance spectroscopy, and cyclic voltammetry, can be utilized with electrochemical biosensors depending on the target molecules and environment.5–8
In particular, composites, conducting polymers, biopolymers, and nanomaterials have been used to create electrochemical biosensors.9–15 An analyte, receptor, transducer, and recording system are components of electrochemical biosensors.16 Acetylcholinesterase, for instance, can be a receptor that functions as a biorecognition element for an analyte. These enzymes are able to generate electrons with recognized signals.
Drug-DNA interactions, disease and biomarker identification are all common uses for DNA biosensors. Due to their potential and economic availability, DNA biosensors are also chosen for gene identification.17–22
For the detection of microbes, viruses, lipoproteins, bacteria, and food pathogens, immunosensor-based biosensors are chosen. When creating electrochemical sensors, it is important to keep in mind that they can either make simultaneous or multiplex measurements or have enhanced selectivity. Their shelf life is between six months and a year, which is short or limited. Other substances can interfere with some electrochemical sensors. Knowing the substances that might interfere with the suggested sensor is crucial. The most popular electrochemical biosensors will be discussed in this review.
Electrochemical DNA Biosensor
Drugs, particularly those with anticancer, antiviral, and antibacterial properties, primarily target the cellular DNA. Covalent and non-covalent agents can be used to categorize DNA-interacting substances in general. Covalent bonding results in cell death and is irreversible. The non-covalent binding can alter DNA conformation, is reversible, and may result in DNA strand breakage. In order to build DNA-targeted medications and to understand how drugs interact with DNA, studies on this topic are essential.23–25
Electrochemical DNA biosensors are a superb tool for the diagnosis of DNA thanks to modern technology and device downsizing. Usually, during electrochemical detection of DNA hybridization, a current at a fixed voltage is observed. In the realm of nucleic acid analysis, numerous efforts have been made to utilize the contemporary electrochemical methodologies. One of the best methods for finding cancer biomarkers early is to use DNA-based electrochemical biosensors.
DNA-based biosensors gather target DNA’s information in order to generate an electrical signal for that particular analyte to be examined. Different sorts of immobilization strategies exist achieve rapid, precise steadiness depending on the transducer’s qualities. The immobilization and hybridization processes are influenced by electrode surface modification using various nanoparticles. Due to its ability to identify little concentrations of oligonucleotides, single-base mismatches, DNA biosensors can quickly identify cancer biomarkers produced by malignant cells.
This section discusses particular DNA-based biosensor for functionally based identification of CYFRA 21-1 biomarker.
CYFRA 21-1 is regarded as a useful biomarker for the identification of NSCLC. As shown in Figures 2, (A-C) created a DNA-based biosensor for the detection of CYFRA21-1 using functionalized three-dimensional graphene and AgNPs (3D GF/AgNPs).
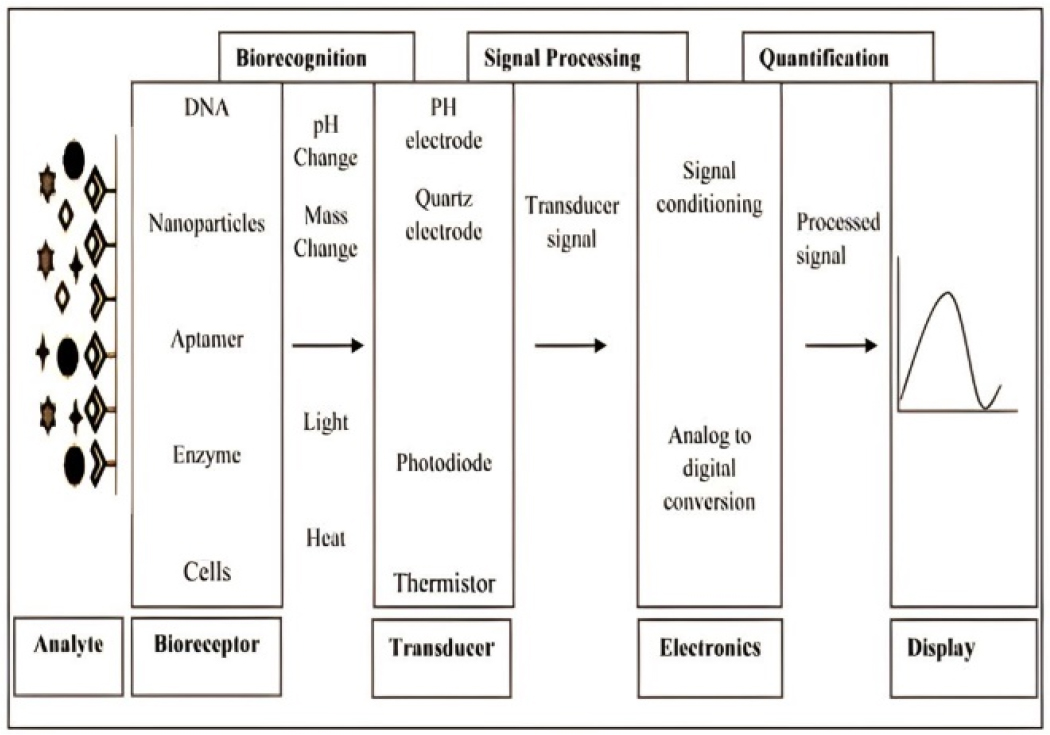
Figure 1:
Elements of Biosensor.4
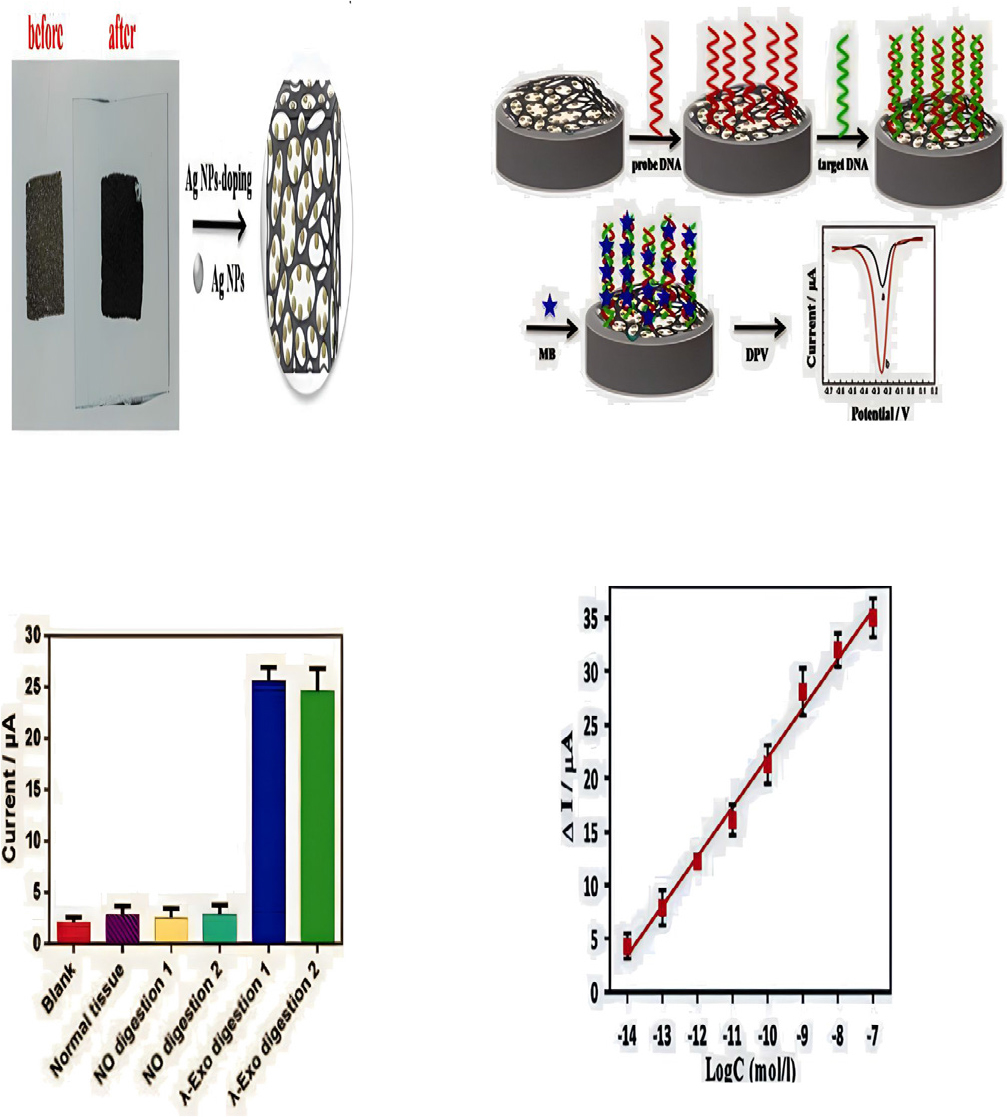
Figure 2:
A, B, C.
A probe DNA with the sequence 5′-S H-GAA GGGA GGAA T GGT GT CA GGGGC G-3′ was utilized to track the complementary DNA 5′-CGCCCCTGACACCATTCCTCC-CTTC. After PCR amplification, the isolated genomic DNA from a clinical sample was electrophoresed. Without any binding, lambda-Exo digestion verifies the desired DNA creation. In order to corroborate the detection of LC, the CYFRA21-1 biomarker develops a stronger signal and peak current in the presence of the MB indicator with a linear relationship at various concentrations (of normal tissue). To identify these indicators(biomarkers), blotting, and PCR-based techniques are widely used.26,27
A) Preparation of 3D/AgNPs and detection process of target DNA
Amperometric response compared to λ-exo digestion to capture PCR product.
Peak current vs. log of the concentration of target ssDNA by linear regression equation: ΔI (μA) = 68.15 + 4.623 log C (M).
Enzyme based Electrochemical Biosensor
In general, biosensors are categorized by the type of transducer they utilize or by the biological component they use, such as enzymes, nucleic acids, antibodies, or cells. Enzyme-based Electrochemical sensors are generally of low cost and show rapid results when compared to the other classic chromatographic and spectroscopic methods.28 A biosensor is an appliance having a transducer and a sensing component built right in. A bioreceptor plus a transducer that generates a response proportional to the concentration of the target substance make up an enzyme biosensor.29 Based on the quantity of the analyte, the enzyme preferentially interacts with the target analyte to produce an electrical signal. Leland C. Clark Jr.’s attempts to monitor oxygen in biological fluids markedly marked the beginning of the biosensor.30
In 1962, Clark proposed the idea of an enzyme-based instrument, which was realized as a clinical analyzer in 1975. The majority of conventional electrochemical enzyme biosensors are built around oxidoreductase enzymes and amperometric detection. An electrochemical setup is used to measure the redox, electron transfer process that occurs at the electrode surface and is related to the biocatalytic conversion of the target analyte. This electron transfer process generates current proportional to the amount of analyte present.31,32
Glucose, lactate, glutamate, urea, and cholesterol enzyme biosensors are the ones that are utilized the most in the clinical field. The analyte that has been investigated the most thoroughly is glucose. Due to its significance in the management of diabetes, it continues to get a great deal of recognition. This particular section concentrate on important progresses in enzyme-based biosensors, the majority of which have happened in the recent years. Commonly employed sensors for both practical and commercial application include glucose, lactate, and glutamate/glutamine. The primary step in the makeup of enzyme based electrochemical biosensor is the enzyme immobilization. There are various ways to immobilize enzymes, including physical adsorption, covalent attachment, imprisonment, and other methods like Covalent crosslinking as well as encapsulation.33–36 The literature’s observations on the use of electrochemical enzymatic biosensors with applications are compiled in Table 1.
Compound | Electrode type | Method | Enzyme | Sensitivity | Linear range | LOD | Application | References |
---|---|---|---|---|---|---|---|---|
Atrazine | MWCNTs/PVA-SPG/Tyr/SPE | CV | Tyrosinase | NS | 0.5-20.0 ppm | 0.3PPM | Water sample | 39 |
Capsaicin | PAL/Nafion/MWCNTs/Pt-E | DPV | Phenylalanine ammonialyase enzyme | NS | NS | 0.1863PPM | Real sample | 40 |
Carbaryl | GCE/rGO/AChE | DPV | AchE | NS | NS | 1.9 nM | Fruit sample | 41 |
Catechol | Lac/GA/PANI/GCE | CV | Laccase | 706.7mA.L moL-1 | 3.2* 10-6-1.96* 10-5M | 2.07*10-6 M | Buffer | 42 |
Catechol | Lac/ZnS: Ni/ZnS/GCE | CV | Laccase | NS | 0.1-100 μM | 35Nm | Water sample | 43 |
Cholesterol | Ag/GO&CHER&CHOD/AuNPs/SPE | LSV | CHOD CHER | 0.084μAcm-2 | 0.01-5000 PPM | 0.001PPM | Buffer | 44 |
Cholesterol | ChEt-ChOx/ZnO-CuO/ITO/Glass bioelectrode | CV | ChOX ChEt | 760mA cm-2 μM | 0.5-12 Mm | NS | Human serum sample | 45 |
D-Alanine | DAAO/PTCA/MWCNTs/GCE | CV | DAAO | NS | 1.0*10-81.0*10-3 M | 3.3*10-9 M | Real sample | 46 |
An electrochemical biosensor for the identification of organophosphorus pesticides was proposed by Zhang and colleagues on the basis of GCE-MWCNT–(PEI/DNA)2/OPH/AChE.37 On this sensor, the paraoxon responses were investigated using CV at a LOD of 0.5 μM. Using CV and UV-vis spectrophotometry, they reported on the production, optimization, and characterization of a biosensor (Figure 3).38
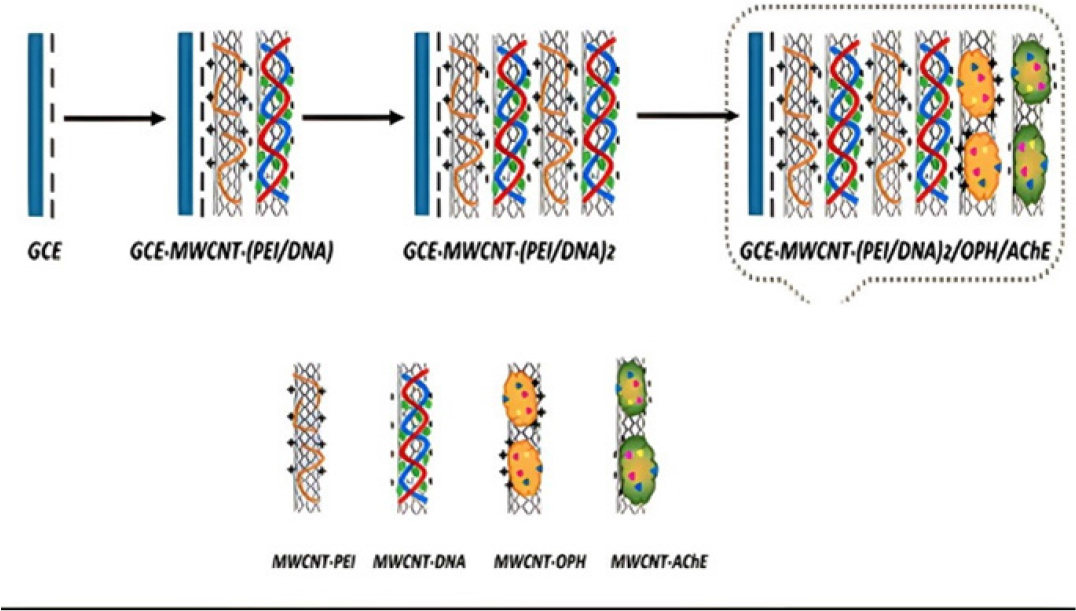
Figure 3:
Identification of paraoxon as organophosphate and carbaryl as non-organophosphate compounds on GCE for the makeover of enzyme-based biosensor.
Immunosensor based Electrochemical Biosensors
A specific target analyte, Antigen (Ag), is detected by the creation of a stable immunocomplex between Antigen and Antibody as a capture agent (Ab), which produces a quantifiable signal provided by a transducer in an immunosensor, a type of affinity solid-state based biosensor.47,48 An affinity biosensor known as an immunosensor relies on interactions between an antigen and a particular antigen that has been immobilised on a transducer surface. Depending on the type of signal, electrochemical immunosensors can be classified as amperometric, potentiometric, conductometric, or impedimetric based on computation of an electrical signal captured by an transducer. One of the immunoassay techniques is the immunosensors, which have all the fundamental performance features of immunoassays. The biological recognition area, the physico-chemical transducer, and the electrical impulse generator are the three components that make up the immunosensor.
Immunosensors can be created for heart illnesses, autoimmune diseases, cancer biomarkers, and more. A Label-Free Electrochemical Immunosensor for Detection of Tumor Necrosis Factor based on Fullerene-Functionalized Carbon Nanotubes/Ionic Liquid (C60-CNTs-IL) was created by Mazloum-Ardakani et al. in their paper (Figure 4). Additionally, the label-free electrochemical immunosensor that was developed proved successful. AFP, a biomarker for liver, ovarian, and testicular cancer, was discovered by Chen and colleagues using a one-step electrochemical immunoassay. The antigen-antibody complex was formed by the immobilisation of horseradish peroxidase-anti-AFP on a nanogold-functionalized graphene interface, and HRP was then used to catalyze the reduction of H2O2 in the solution. The Detection Limit (LOD) for AFP was 0.05 ng/mL, with a linear range of 0.1-200 ng/mL.49
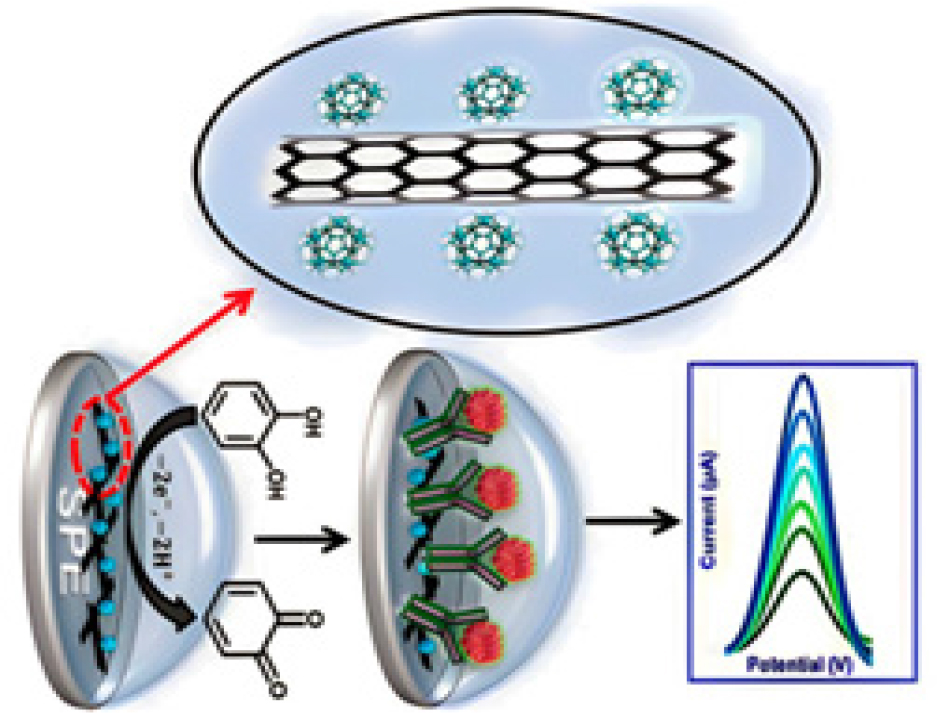
Figure 4:
Diagrammatic illustration of label-free electrochemical immunosensor based on Fullerene-Functionalized Carbon Nanotubes/Ionic Liquid.
Besides in here, we provide an update on the electrochemical immunosensors used in clinical study as of recent publications (Table 2).
Biomarker | Type of illness | Immunosensor | Electrochemical technique | Linear range | LOD | References |
---|---|---|---|---|---|---|
PSA | Prostate | HRP-modified magnetic particles labelled anti-PSA antibodies.
AuNPs-modified pyrolytic graphite disc electrode. |
Amperometry | 4-10 ng.mL-1 | 0.5 pg.mL-1 | 50 |
PSA | Prostate | Anti-PSA/MWCNTs/IL/GCE. | Differential pulse voltammetry | 0.2-1.0 ng. mL-1
1-40.0 ng. mL-1 |
20 pg. mL-1 | 51 |
PSA IL-6 | Prostate
Rheumatoid Arthritis Systemic Lupus Erythematosus |
AuNPs-microfluidic 8-electrode SPCE array. | Amperometry | 0.23pg.mL-1 PSA
0.30 pg. mL-1 IL-6 |
– | 52 |
PSA hCG | Prostate Cancer
Ovarian Testicular Trophoblastic Cancers |
Porous membrane-coated 2-electrode gold array. | Amperometry | NS | 0.4μg.L-1 PSA
2.5 UL-1 hCG |
53 |
PSA IL-8 | Prostate
Rheumatoid Arthritis, Inflammatory Bowel Disease, Psoriasis, Acute Respiratory Distress Syndrome |
16-electrode SPCE array. | Amperometry | – | 5pg.mL-1 PSA
8 pg. mL-1 IL-8 |
54 |
PthA | Citrus Bacterial Cancer Disease | AuNP/PB/CILE/GCE. | Square wave voltammetry | 0.03-100.00 nM | 0.01nM | 55 |
TNF-α | Rheumatoid Arthritis | PA+PAA/GCE. | Amperometry | 0.02-200.00 ng.mL-1 | 0.01ng.mL-1 | 56 |
TNF-α | Rheumatoid Arthritis | K3[Fe(CN)6]-CHT/GA/NA/Mouse anti-human TNF-α | Cyclic voltammetry | 0.02-34.00 ng.mL-1 | 10.0 pg.mL-1 | 57 |
TNF-α | Rheumatoid Arthritis | C60-fMWCNT-IL | Differential pulse voltammetry | 5.0-75.00 pg.mL-1 | 2.0 pg.mL-1 | 58 |
OPTICAL BIOSENSORS
In order to perform a qualitative and quantitative analysis of the target, the optical sensors primarily examine the optical signals produced when target and the detection element are combined. They then directly transform these signals through transducer in real-time. They are widely employed in various fields because of their accuracy, simplicity of maintenance and quick detection.59
The 2019 Coronavirus Disease (COVID-19) outbreak for one more time highlighted importance in creating swift and extremely sensible diagnostic methods for fast detection of patients who are infected.
Despite sensitivity and specificity requirements being met RT-PCR diagnostic procedures, there are certain inherent drawbacks with time-consuming.
Its application possibilities are restricted by sophisticated machinery and experienced operators. Optical biosensors based on nanomaterials attracted a lot of attention for the detection of SARS-CoV-2. The development of optical biosensors for SARS-CoV-2 diagnosis, including colorimetric, Electrochemical (EC), Quartz Crystal Microbalance (QCM), Field Effect Transistor (FET)-based, localized surface plasmon resonance (LSPR), Surface Enhanced Raman Scattering (SERS), and fluorescence-based biosensors.60,61 However, LSPR, FET, EC, and SERS biosensors have been used often in pandemic which is mainly highlighted in the below article.
Plasmonic Biosensors
In both life science and pharmaceutical research, these biosensors play an important role in characterizing and measuring bio-analytical targets. These biosensors are very sensitive, devoid of tagging which are suitable for a variety of therapeutically relevant analytes that are present to be targeted. These are utilized to identify SARS-CoV antibodies using a macromolecule of amino acids made by combining a SARS coronaviral surface antigen with gold-binding polypeptides in a genetical format.62
Viral antibodies can be found at the nanomolar level. The bioassay was performed using a portable SPR device. In order to identify patients who have been inoculated against SARS-CoV-2 and strategically help vaccine development efforts, the immune system responds to the sensor’s exposure to SARSCoV-2 by producing antibodies at levels that can be identified and tracked. By precisely identifying the antibodies, we can support the creation of the vaccine and assess those who have developed immunity to SARS-CoV-2.63
The multi-functional plasmonic biosensor built by the joint effects of Plasmonic Photothermal (PPT) and Localized Surface Plasmon Resonance (LSPR) has also been shown by Wang’s research team to have encouraging COVID-19 diagnosing capabilities. By hybridizing nucleic acid, two-dimensional gold Nanoislands (AuNIs) and complementary DNA receptors may identify specific sequence from virus, as shown in Figure 5A. The creation of plasmonic heat on the same surface of the AuNIs when they began shining at their plasmonic resonance frequency greatly improved detecting capabilities. The in situ hybridization temperature can be raised by the locally generated PPT heat, which then permits differentiation of two identical gene sequences. In identification of specific virus, our multi functionalized LSPR sensor has demonstrated excellent sensing capability.64
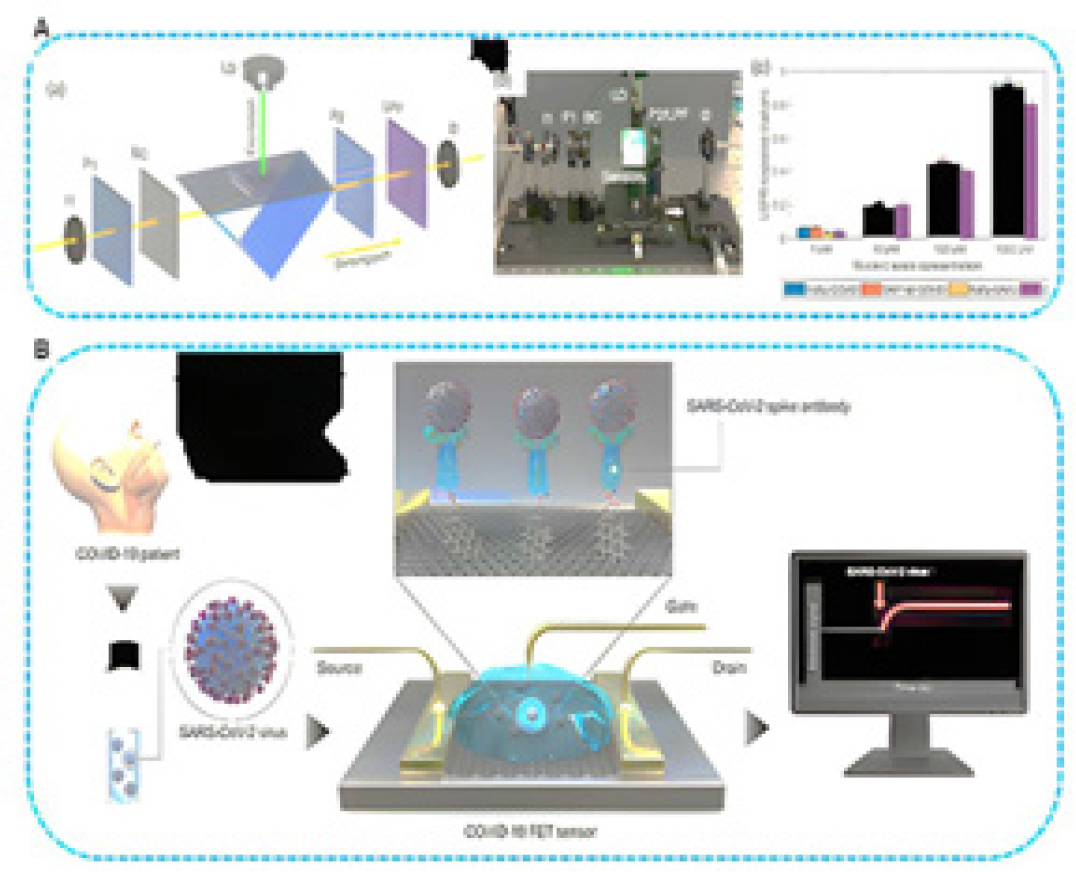
Figure 5:
(A) (a) Pattern (b) experimental arrangement of multi-functional PPT enhanced LSPR biosensor, (c) Concentrations of several viral oligos measured using LSPR biosensor, (B) picture of FET-based biosensor.
FET based Biosensors
Field-effect transistor dependent biosensing platforms provide wide range of intriguing advantages compared to current diagnostic methods, including the capability of being extremely accurate and to instantaneously identify low volume containing analytes. These could be used for point-of-care assays, clinical analysis, and on-site diagnostics.65 Due to its capacity to detect adjacent surface fluctuations and serve as an ideal sensing platform, graphene, which has carbon atoms of hexagonal orientation exposed on its surface and is electronically conductive, high charge mobility has proven to be extremely detectable in sensing systems. So these graphene-based FET biosensors are crucial for performing highly sensitive immunological diagnosis.
A system depending on this phenomenon for identifying the coronavirus in clinical specimens has been successfully created by Sea and colleagues as illustrated in Figure 5B.66 In order to create the biosensor, the graphene sheets of the FET were coupled with particular antibodies against the SARS-CoV-2 spike protein. Antigen protein, a self-cultured virus, and nasopharyngeal swab samples from COVID-19 pneumonia patients were used to assess the biosensor’s sensing capacity.
The SARSCoV-2 spike protein could be detected by the FET biosensor at 1 fg/mL in phosphate-buffer saline and 100 fg/mL in clinical transport medium.
EC Biosensors
Because of its simplicity, low cost, ease of downsizing, and ability to be manufactured in large quantities, electrochemical biosensors have drawn the interest of scientists. Additionally, they can be used at the point of care in clinics or at homes.67,68 Early diagnosis is the sole method for controlling and combating the COVID-19 virus since there is no vaccination or specialized medication available for its treatment. Figure 6A shows the architecture of a multiplexed electrode array. The created EC sensor successfully used in spiked nasal samples, and influenza A and B did not cause any measurable interference. Patricia Abad-Valle and colleagues created a further straightforward, affordable, and user-friendly EC Geno sensor on gold films for the detection of the SARS-CoV gene. Square wave voltammetry was used by the Geno sensor to reach a response criterion of 6 pM for sequencing this DNA.69
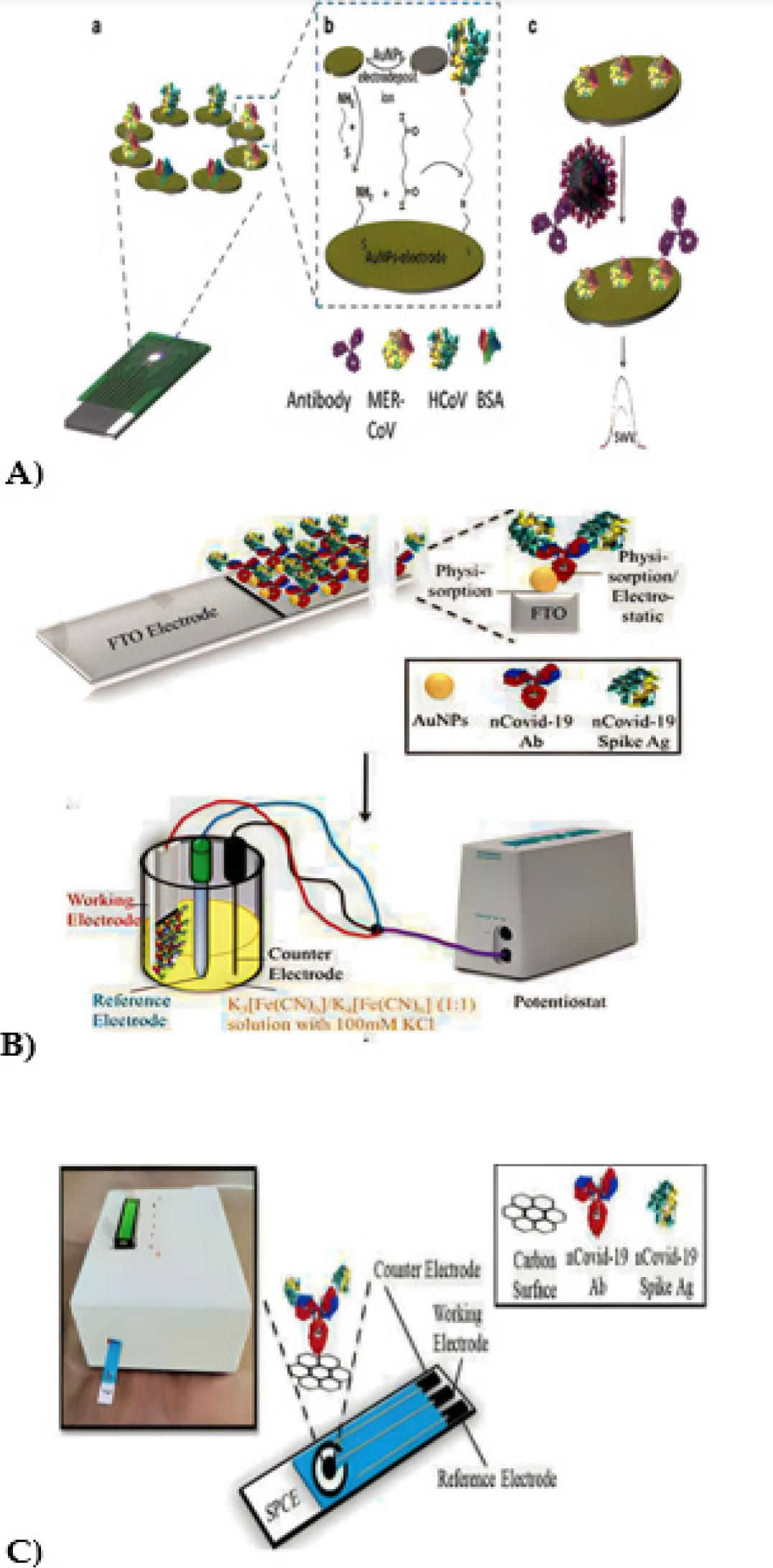
Figure 6:
(A) Chip array, immunosensor fabrication process and virus identification (B) FTO electrode modification process (C) Diagram of electrochemical eCovSens device.
Gandhi’s research team has created a custom made biosensing device (eCovSens) that is available for purchase to diagnose COVID-19 Ag. In order to create a potentiostatic biosensor, a Fluorine-doped Tin Oxide electrode (FTO) was embellished with AuNPs and bound with the COVID-19 Ab as shown in Figure 6B. Screen printed carbon electrode was immobilized with COVID-19 Ab to analyse the difference in conductivity, shown in picture 6C, in order to build eCovSens.70 Both the FTO immunosensor and the SPCE biosensor shown exceptional detection in the region ranging 1 fM to 1 M for COVID-19 Ag detection. In spiked saliva specimens, the detection limits for the eCovSens and potentiostatic devices were 90 fM and 120 fM, respectively. Within 10–30 s, the eCovSens gadget as-fabricated can detect COVID-19 Ag. Because of its improved selectivity and specificity, this platform can be utilized as a backup diagnostic tool to find COVID Silver traces in saliva from patients. The eCovSens gadget can be a useful diagnostic tool because it is highly affordable, portable, needs very little electricity (1.3-3 V), and can even be powered by batteries.
SERS-based Biosensors
The accurate measurement of analyte utilizing Surface Enhanced Raman Scattering-coded nano particles by replacing colloidal gold to detect response has drawn the researchers’ undivided attention to SERS-based biosensors. SERS tags consist 3 main parts: an Gold/Silver nanoparticle that serves as a Raman enhanced substrate, adsorbed Raman reporter dyes that generate distinctive Surface Enhanced Raman Scattering response, and certain antibodies that bind targets. Figure 7 illustrates how Wang et al. constructed a Surface Enhanced Raman Scattering dependent biosensor to concurrently identify the presence of two viruses, such as Influenza A (H1N1) and Human Adenovirus (HAdV), using Fe3O4@Ag nanoparticles as magnetic SERS nanotags.71 For H1N1 and HAdV, respectively, the small amounts of detection calculated using SERS-based biosensors were 50.0 pfu/mL and 10.0 pfu/mL. The biosensor’s sensitivity was 2000 times greater than that of the widely used colloidal gold strip approach. Without any pretreatment, the suggested biosensor can be used directly for the examination of living material. Additionally, a porous carbon substrate coated with Ag nanoparticles was used to build a SERS-based biosensor for the detection of three distinct viral types.72 For these three viruses, the lowest feasible concentration that our biosensor could detect was 1 107 copies/mL. The distinction of virus was made possible by the SERS spectra.
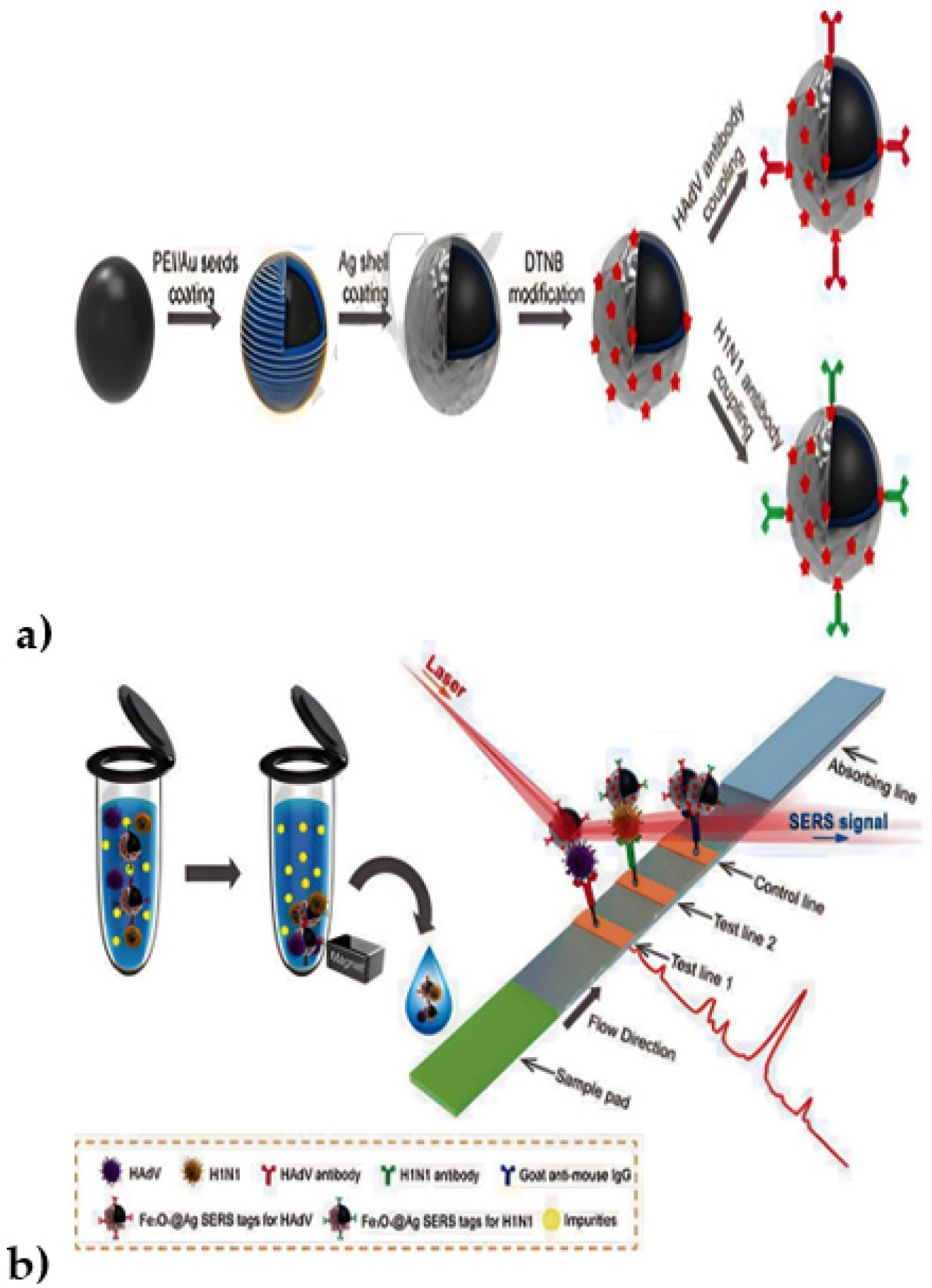
Figure 7:
(a) Antibody-modified Fe3O4@Ag nanotags preparation, (b) Brief illustration of magnetic SERS-based biosensor for the identification of respiratory viruses.
CONCLUSION
Biosensor technology has a number of distinct and significant advantages over conventional analytical techniques, including real-time operation, low limits of detection, greater sensitivity, decreased cost, simple operation, and downsizing. Without prior separation or derivatization, simultaneous detection and quantification are possible. This review illustrated the diverse range of applications for electroanalytical biosensors. Despite the increased use of disposable electrodes, change in electrodes has received more attention because of larger surface area. The approval processes prove that they work with actual samples. Biosensors based on DNA could be used to examine medication interactions, analyze genes, and perform hybridization. Researchers have favored voltammetric and amperometric methods. Immunosensors are utilized in the detection in addition to the measurement of variable range of samples as a result of the quick development of immunological agents and technology. Wider applications of simple, affordable, and dependable immunosensing devices have been developed, including extensive screening programs. Electrochemical biosensor research has been expanding in recent years. Electrochemical biosensors will soon be used more extensively in the medical fields among others. Furthermore, the development of electrochemical biosensors is intimately tied to wireless real-time data collecting. Biosensors and biochips are tools that can be used at the Point of Care (POC). Information regarding potential future developments in molecular diagnostics will be provided through the improvement of DNA biosensor. It is conceivable that PCR-free DNA biosensors will soon be available thanks to the industry’s rapid advancements in electrochemical biosensing.
Since the pandemic’s start, spread of coronavirus has been witnessed throughout the world. Additionally, the virus is always evolving in a sneakier way, posing issues with temporal persistence and globalization. To stop the spread of viruses, it is crucial to create technologies for quick virus detection and diagnosis. An excellent option for the identifying this virus is optical biosensors. The significance of these biosensors is particularly stressed in the detection of coronavirus. For asymptomatic patients, everyone should have easy access to in-house biosensor instruments in detection of virus in people. Their capacity to detect the target virus antigen quickly, on-site, and with great sensitivity can ultimately lead to an faster detection of covid. In addition to the busiest places, including airports and hospitals, they can scan people there. In order to detect viruses, the use of nanoparticles in conjunction with electrochemical diagnostic techniques is promising. Nanomaterials and nanotechnologies should evolve in order to create better biosensors that can detect virus antigens with good sensitivity and selectivity.
References
- Wilson GS, Hu YB. Enzyme based biosensors for measurements. Chem Rev. 2000;100(7):2693-704. [PubMed] | [CrossRef] | [Google Scholar]
- Borisov SM, Wolfbeis OS. Optical biosensors. Chem Rev. 2008;108(2):423-61. [PubMed] | [CrossRef] | [Google Scholar]
- Van Dorst B, Mehta J, Bekaert K, Rouah-Martin E, De Coen W, Dubruel P, et al. Recent advances in recognition elements of food and environmental biosensors: a review. Biosens Bioelectron. 2010;26(4):1178-94. [PubMed] | [CrossRef] | [Google Scholar]
- Jia WZ, Wang K, Xia XH. Elimination of electrochemical interferences in glucose biosensors. TrAC Trends Anal Chem. 2010;29(4):306-18. [CrossRef] | [Google Scholar]
- Chen C, Xie Q, Yang D, Xiao H, Fu Y, Tan Y, et al. Recent advances in electrochemical glucose biosensors: a review. RSC Adv. 2013;3(14):4473-91. [CrossRef] | [Google Scholar]
- Soldatkina OV, Soldatkin OO, Kasap BO, Kucherenko DY, Kucherenko IS, Kurc BA, et al. A novel amperometric glutamate biosensor based on glutamate oxidase adsorbed on silicalite. Nanoscale Res Lett. 2017;12(1):260 [PubMed] | [CrossRef] | [Google Scholar]
- Park J, Lee W, Kim I, Kim M, Jo S, Kim W, et al. Ultrasensitive detection of fibrinogen using erythrocyte membrane-draped electrochemical impedance biosensor. Sens Actuators B. 2019;293:296-303. [CrossRef] | [Google Scholar]
- Mahato K, Kumar A, Maurya PK, Chandra P.. Shifting paradigm of cancer diagnoses in clinically relevant samples based on miniaturized electrochemical nanobiosensors and microfluidic devices. Biosens Bioelectron. 2018;100:411-28. [PubMed] | [CrossRef] | [Google Scholar]
- Yoo EH, Lee SY. Glucose biosensors: an overview of use in clinical practice. Sensors (Basel) May pp. 2010;10(5):4558-76. [PubMed] | [CrossRef] | [Google Scholar]
- Bahadır EB, Sezgintürk MK. Applications of commercial € biosensors in clinical, food, environmental, and biothreat/biowarfare analyses. Anal Biochem. 2015;478:107-20. [PubMed] | [CrossRef] | [Google Scholar]
- Kong RM, Zhang XB, Chen Z, Tan W.. AptamerAssembled nanomaterials for biosensing and biomedical applications. Small. 2011;7(17):2428-36. [PubMed] | [CrossRef] | [Google Scholar]
- Tavakolian-Ardakani Z, Hosu O, Cristea C, Mazloum-Ardakani M, Marrazza G.. Latest trends in electrochemical sensors for neurotransmitters: a review. Sensors (Basel). 2019;19(9):2037 [PubMed] | [CrossRef] | [Google Scholar]
- Pumera M, Sánchez S, Ichinose I, Tang J. Electrochemical nanobiosensors. Sens Actuators B.. 2007;123(2):1195-205. [CrossRef] | [Google Scholar]
- Grieshaber D, MacKenzie R, Vor Reimhult E € os. Electrochemical Biosensors – Sensor Principles and Architectures. Sensors (Basel). 2008;8(3):1400-58. [PubMed] | [CrossRef] | [Google Scholar]
- Wei D, Bailey MJA, Andrew P, Ryhänen T.. Electrochemical biosensors at the nanoscale. Lab Chip. 2009;9(15):2123-31. [PubMed] | [CrossRef] | [Google Scholar]
- Khanmohammadi A, Aghaie A, Vahedi E, Qazvini A, Ghanei M, Afkhami A, et al. Electrochemical biosensors for the detection of lung cancer biomarkers: a review. Talanta. 2020;206:120251 [PubMed] | [CrossRef] | [Google Scholar]
- Farzin L, Shamsipur M, Samandari L, Sheibani S.. Advances in the design of nanomaterial-based electrochemical affinity and enzymatic biosensors for metabolic biomarkers: a review. Mikrochim Acta. 2018;185(5):276 [PubMed] | [CrossRef] | [Google Scholar]
- Blair EO, Corrigan DK. A review of microfabricated electrochemical biosensors for DNA detection. Biosens Bioelectron. 2019;134:57-67. [PubMed] | [CrossRef] | [Google Scholar]
- Cui F, Zhou Z, Zhou HS. Review—measurement and analysis of cancer biomarkers based on electrochemical biosensors. J Electrochem Soc.. 2020;167(3):037525 [CrossRef] | [Google Scholar]
- Wang J. Electrochem. Nucleic Acid Biosens. 2002;469:63-71. [CrossRef] | [Google Scholar]
- Shahabadi N, Abbasi AR, Moshtkob A, Hadidi S. Design, synthesis and DNA interaction studies of new fluorescent platinum complex containing anti-HIV drug didanosine. J Biomol Struct Dyn. 2020;38(10):2837-48. [PubMed] | [CrossRef] | [Google Scholar]
- Nemčeková K, Labuda J, Milata V, Blaškovičová J. Sochr JInteraction of DNA and mononucleotides with theophylline investigated using electrochemical biosensors and biosensing. Bioelectrochemistry. 2018;123:182-9. [PubMed] | [CrossRef] | [Google Scholar]
- Sohrabi N, Valizadeh A, Farkhani SM, Akbarzadeh A. Basics of DNA biosensors and cancer diagnosis. Artif Cells Nanomed Biotechnol. 2016;44(2):654-63. [PubMed] | [CrossRef] | [Google Scholar]
- Kowalczyk A.. Trends and perspectives in DNA biosensors as diagnostic devices. Curr Opin Electrochem. 2020;23:36-41. [CrossRef] | [Google Scholar]
- Topkaya SN, Cetin AE. Investigation of metal ion effect on specific DNA sequences and DNA hybridization. Electroanalysis. 2020;32(1):112-8. [CrossRef] | [Google Scholar]
- Derkus B, Ozkan M, Emregul KC, Emregul E.. Single frequency analysis for clinical immunosensor design. RSC Adv. 2016;6(1):281-9. [CrossRef] | [Google Scholar]
- Chen M, Wang Y, Su H, Mao L, Jiang X, Zhang T, et al. Threedimensional electrochemical DNA biosensor based on 3D graphene-Ag nanoparticles for sensitive detection of CYFRA21-1 in non-small cell lung cancer. Sens Actuator B.. 2018;255:2910-8. [CrossRef] | [Google Scholar]
- Stroble JK, Stone RB, Watkins SE. An overview of biomimetic sensor technology. Sens Rev. 2009;29(2):112-9. [CrossRef] | [Google Scholar]
- Soropogui KM, Jameel AT, Salim WWAW. EnzymeBased biosensors for electrochemical detection of pesticides–A mini review. IJEEI. 2018;6:161-71. [CrossRef] | [Google Scholar]
- . Array. Chem Rev. Array;100(80):2693-704. [CrossRef] | [Google Scholar]
- Schuhmann W.. Amperometric enzyme biosensors based on optimised electron-transfer pathways and non-manual immobilisation procedures. J Biotechnol. 2002;82(4):425-41. [PubMed] | [CrossRef] | [Google Scholar]
- Heller A, Feldman B.. Electrochemical glucose sensors and their applications in diabetes management. Chem Rev. 2008;108(7):2482-505. [PubMed] | [CrossRef] | [Google Scholar]
- [PubMed] | [CrossRef] | [Google Scholar]
- [PubMed] | [CrossRef] | [Google Scholar]
- Kurbanoglu S, Ozkan SA, Merkoçi A.. Nanomaterials-based Enzyme electrochemical biosensors operating through inhibition for biosensing applications. Biosens Bioelectron. 2017;89(2):886-98. [PubMed] | [CrossRef] | [Google Scholar]
- Zhang Q, Xu Q, Guo Y, Sun X, Wang X.. Acetylcholinesterase biosensor based on the mesoporous carbon/Ferroferric oxide modified electrode for detecting organophosphorus pesticides. RSC Adv. 2016;6(29):24698-703. [CrossRef] | [Google Scholar]
- Zhang Y, Arugula MA, Wales M, Wild J, Simonian AL. A novel layer-by-layer assembled multi-enzyme/CNT biosensor for discriminative detection between organophosphorus and non-Organophosphrus pesticides. Biosens Bioelectron. 2015;67:287-95. [PubMed] | [CrossRef] | [Google Scholar]
- Tortolini C, Bollella P, Antiochia R, Favero G, Mazzei F.. Inhibition-based biosensor for atrazine detection. Sens Actuat B. 2016;224:552-8. [CrossRef] | [Google Scholar]
- Sabela MI, Mpanza T, Kanchi S, Sharma D, Bisetty K.. Electrochemical sensing platform amplified with a nanobiocomposite of L-phenylalanine ammonia-lyase enzyme for the detection of capsaicin. Biosens Bioelectron. 2016;83:45-53. [PubMed] | [CrossRef] | [Google Scholar]
- da Silva MKL, Vanzela HC, Defavari LM, Cesarino I. Determination of carbamate pesticide in food using a biosensor based on reduced graphene oxide and acetylcholinesterase enzyme. Sens Actuat B.. 2018;277:555-61. [CrossRef] | [Google Scholar]
- Nazari M, Kashanian S, Rafipour R. Laccase immobilization on the electrode surface to design a biosensor for the detection of phenolic compound such as catechol. Spectrochim Acta A Mol Biomol Spectrosc. 2015;145:130-8. [PubMed] | [CrossRef] | [Google Scholar]
- Wang Y, Qu J, Li S, Qu J.. Catechol biosensor based on ZnS:Ni/ZnS quantums dots and laccase modified glassy carbon electrode. J Nanosci Nanotechnol. 2016;16(8):8302-7. [CrossRef] | [Google Scholar]
- Huang Y, Tan J, Cui L, Zhou Z, Zhou S, Zhang Z, et al. Graphene and Au NPs co-mediated enzymatic silver deposition for the ultrasensitive electrochemical detection of cholesterol. Biosens Bioelectron. 2018;102:560-7. [PubMed] | [CrossRef] | [Google Scholar]
- Batra N, Tomar M, Gupta V.. ZnO-CuO composite matrix based reagentless biosensor for detection of total cholesterol. Biosens Bioelectron. 2015;67:263-71. [PubMed] | [CrossRef] | [Google Scholar]
- Q. , Huang Y, Lin X, Zhu S, Fu Y.. Highly sensitive D-alanine electrochemical biosensor based on functionalized multi-walled carbon nanotubes and D-amino acid oxidase. Biochem Eng J. 2016;113:1-6. [CrossRef] | [Google Scholar]
- Ghindilis AL, Atanasov P, Wilkins M, Wilkins E.. Immunosensors: electrochemical sensing and other engineering approaches. Biosens Bioelectron. 1998;13(1):113-31. [PubMed] | [CrossRef] | [Google Scholar]
- Morgan CL, Newman DJ, Price CP. Immunosensors: technology and opportunities in laboratory medicine. Clin Chem. 1996;42(2):193-209. [PubMed] | [CrossRef] | [Google Scholar]
- Chen H, Zhang B, Cui Y, Liu B, Chen G, Tang D., et al. One-step electrochemical immunoassay of biomarker based on nanogold-functionalized graphene sensing platform. Anal Methods. 2011;3(7):1615-21. [CrossRef] | [Google Scholar]
- Mani V, Chikkaveeraiah BV, Patel V, Gutkind JS, Rusling JF. Ultrasensitive immunosensor for cancer biomarker proteins using gold nanoparticle film electrodes and multienzyme-particle amplification. ACS Nano. 2009;3(3):585-94. [PubMed] | [CrossRef] | [Google Scholar]
- Salimi A, Kavosi B, Fathi F, Hallaj R.. Highly sensitive immunosensing of prostate-specific antigen based on ionic liquid-carbon nanotubes modified electrode: application as cancer biomarker for prostate biopsies. Biosens Bioelectron. 2013;42:439-46. [PubMed] | [CrossRef] | [Google Scholar]
- Chikkaveeraiah BV, Mani V, Patel V, Gutkind JS, Rusling JF. Microfluidic electrochemical immunoarray for ultrasensitive detection of two cancer biomarker proteins in serum. Biosens Bioelectron. 2011;26(11):4477-83. [PubMed] | [CrossRef] | [Google Scholar]
- Meyerhoff ME, Duan C, Meusel M.. Novel nonseparation sandwich-type electrochemical enzyme immunoassay system for detecting marker proteins in undiluted blood. Clin Chem. 1995;41(9):1378-84. [PubMed] | [CrossRef] | [Google Scholar]
- Wan Y, Deng W, Su Y, Zhu X, Peng C, Hu H, et al. Carbon nanotube-based ultrasensitive multiplexing electrochemical immunosensor for cancer biomarkers. Biosens Bioelectron. 2011;30(1):93-9. [PubMed] | [CrossRef] | [Google Scholar]
- Haji-Hashemi H, Norouzi P, Safarnejad MR, Larijani B, Habibi MM, Raeisi H, et al. Sensitive electrochemical immunosensor for citrus bacterial canker disease detection using fast Fourier transformation square-wave voltammetry method. J Electroanal Chem. 2018;820:111-7. [CrossRef] | [Google Scholar]
- Yin Z, Liu Y, Jiang LP, Zhu JJ. Electrochemical immunosensor of tumor necrosis factor α based on alkaline phosphatase functionalized nanospheres. Biosens Bioelectron. 2011;26(5):1890-4. [PubMed] | [CrossRef] | [Google Scholar]
- Weng S, Chen M, Zhao C, Liu A, Lin L, Liu Q, et al. [Fe(CN) 6 as signal for facile and sensitive determination of tumor necrosis factor-alpha]. Sens. Actuators B Chem. Label-Free Electrochem Immunosensor Based K3. 2013;184:1-7. [CrossRef] | [Google Scholar]
- Mazloum-Ardakani M, Hosseinzadeh L, Khoshroo A Label. Label-free electrochemical immunosensor for detection of tumor necrosis factor α based on fullerene-functionalized carbon nanotubes/ionic liquid. J Electroanal Chem. 2015;757:58-64. [CrossRef] | [Google Scholar]
- Eletxigerra U, Martinez-Perdiguero J, Merino S.. Disposable microfluidic immuno-biochip for rapid electrochemical detection of tumor necrosis factor alpha biomarker. Sens Actuators B. 2015;221:1406-11. [CrossRef] | [Google Scholar]
- Say R, Özkütük EB, Ünlüer ÖB U, Ersöz A ˘gura ˘g A.. Nano Anti-tumor Necrosis Factor-alpha based potentiometric sensor for tumor necrosis factor-alpha detection. Sens Actuators B. 2015;209:864-9. [CrossRef] | [Google Scholar]
- Du D, Zou Z, Shin Y, Wang J, Wu H, Engelhard MH, et al. Sensitive immunosensor for cancer biomarker based on dual signal amplification strategy of graphene sheets and multienzyme functionalized carbon nanospheres. Anal Chem.. 2010;82(7):2989-95. [PubMed] | [CrossRef] | [Google Scholar]
- Qian J, Dai H, Pan X, Liu S.. Simultaneous detection of dual proteins using quantum dots coated silica nanoparticles as labels. Biosens Bioelectron. 2011;28(1):314-9. [PubMed] | [CrossRef] | [Google Scholar]
- Abdelhadi D, Benjamin C, Maryam Hojjat J, Vincent T, Julien C, Keisean S, et al. A rapid and quantitative serum test for SARS-CoV-2 antibodies with portable surface plasmon resonance sensing. 2020 [PubMed] | [CrossRef] | [Google Scholar]
- Qiu G, Gai Z, Tao Y, Schmitt J, Kullak-Ublick GA, Wang J., et al. Dual-functional plasmonic photothermal biosensors for highly accurate severe acute respiratory syndrome coronavirus 2 detection. ACS Nano. 2020;14(5):5268-77. [PubMed] | [CrossRef] | [Google Scholar]
- Liu J, Chen X, Wang Q, Xiao M, Zhong D, Sun W, et al. Ultrasensitive monolayer MoS2 Field-Effect transistor based DNA sensors for screening of Down syndrome. Nano Lett.. 2019;19(3):1437-44. [PubMed] | [CrossRef] | [Google Scholar]
- Seo G, Lee G, Kim MJ, Baek SH, Choi M, Ku KB, et al. Rapid detection of COVID-19 Causative Virus (SARS-CoV-2) in human nasopharyngeal swab specimens using Field-Effect transistor-based biosensor. Array. 2020;14(4):5135-42. [PubMed] | [CrossRef] | [Google Scholar]
- Asif M, Aziz A, Wang H, Wang Z, Wang W, Ajmal M, et al. Superlattice stacking by hybridizing layered double hydroxide nanosheets with layers of reduced graphene oxide for electrochemical simultaneous determination of dopamine, uric acid and ascorbic acid. Mikrochim Acta. 2019;186(2):61 [PubMed] | [CrossRef] | [Google Scholar]
- Asif M, Aziz A, Dao AQ, Hakeem A, Wang H, Dong S, et al. Real-time tracking of hydrogen peroxide secreted by live cells using MnO2 nanoparticles intercalated layered doubled hydroxide nanohybrids. Anal Chim Acta. 2015;898:34-41. [PubMed] | [CrossRef] | [Google Scholar]
- Asif M, Aziz A, Ashraf G, Wang Z, Wang J, Azeem M, et al. Facet-inspired core-shell gold nanoislands on metal oxide octadecahedral heterostructures: high sensing performance toward sulfide in biotic fluids. ACS Appl Mater Interfaces. 2018;10(43):36675-85. [PubMed] | [CrossRef] | [Google Scholar]
- Abad-Valle P, Fernández-Abedul MT, Costa-García A.. Genosensor on gold films with enzymatic electrochemical detection of a SARS virus sequence. Biosens Bioelectron. 2005;20(11):2251-60. [PubMed] | [CrossRef] | [Google Scholar]
- Mahari S, Roberts A, Shahdeo D, Gandhi S. eCovSens-ultrasensitive novel in-house built printed circuit board based electrochemical device for rapid detection of nCovid-19. Array. 2020 [PubMed] | [CrossRef] | [Google Scholar]
- Wang C, Wang C, Wang X, Wang K, Zhu Y, Rong Z, et al. Magnetic SERS strip for sensitive and simultaneous detection of respiratory viruses. ACS Appl Mater Interfaces. 2019;11(21):19495-505. [PubMed] | [CrossRef] | [Google Scholar]
- Luo Z, Chen L, Liang C, Wei Q, Chen Y, Wang J., et al. Porous carbon films decorated with silver nanoparticles as a sensitive SERS substrate, and their application to virus identification. Microchim Acta. 2017;184(9):3505-11. [CrossRef] | [Google Scholar]