ABSTRACT
Exosomes are small nanovesicles that are produced through the fusion of multiple veins and plasma membranes, then escaping into adjacent body fluids. Considerable attention has been paid to them due to their potential as delivery vehicles for drugs. Exosomes play a key role in many physiological processes that occur both in healthy and ill states. The production of exosomes depends on the state of the disease, but the disease itself often serves the opposite function by promoting more cell damage and stress. Traditional drug delivery methods often face limitations in terms of specificity, targeted delivery and drug release kinetics. Exosomes have emerged as promising candidates for drug delivery due to their natural ability to selectively deliver cargoes to recipient cells. Exosomes are taken up through various mechanisms, including endocytosis and fusion with target cells. They can encapsulate poorly soluble drugs, enhancing their bioavailability and improving their therapeutic efficacy. Exosomes inspired Lipid Nanovesicles (Exo-LNVs) have shown promising results as drug delivery vehicles. Exosomes have considerable potential as sophisticated vehicle for the delivery of targeted drugs and genes due to their unique characteristics, including inherent stability, minimal immunity and exceptional ability to penetrate tissues and cells. Therapeutic interventions have the capacity to increase effectiveness, reduce side effects and increase patient compliance. Exosomes have the ability to transport various therapeutic by encapsulating different substrates such as nucleic acids, proteins and small molecules. Recent advancements in exosome-inspired lipid nanovesicles have opened up new possibilities for cell-specific drug delivery. These nanovesicles mimic the composition and structure of exosomes, which are naturally occurring extracellular vesicles released by cells. By incorporating therapeutic agents into the lipid nanovesicles, they can effectively target and deliver drugs to specific cells of interest. This review article aims to summarize the current literature on Exo-LNVs and discuss their potential as drug delivery vehicles. A systematic search was conducted to identify relevant studies and relevant data were extracted and analyzed. The review covers various aspects of Exo-LNVs, including their composition, preparation methods and applications in various disease conditions.
INTRODUCTION
The function of drug delivery systems in pharmaceutical science is crucial. Treatment agents are delivered to target cells or tissues through these methods, minimizing systemic toxicity and maximizing effectiveness by preventing non-specific distribution.1 Over the past decades, remarkable progress and innovations have been made in the field of Drug Delivery Systems (DDS) using nanocarriers and vehicles. Traditional approaches to the delivery of drugs, including oral administration, lead to the dispersion of drugs in the gastrointestinal tract, which results in reduced bioavailability and inconsistent absorption.2 To overcome these limitations, researchers have developed various drug delivery systems, including nanoparticles, liposomes, hydrogels and nanoparticles. Lipid nanovesicles, also known as liposomes, are spherical structures composed of lipid bilayers that can encapsulate and deliver various cargoes, including drugs.3 In recent times, DDS composed of our own cells or cell derivatives has emerged as a highly promising candidate for the future development of potent vectors capable of traversing physical and biological barriers, overcoming obstacles erected by the tumour microenvironment and possessing inherent targeting properties.4,5 The design of lipid nanovesicles has been inspired by Exosomes, small vesicles naturally released by cells. Exosomes have gained significant attention due to their potential applications in drug delivery due to their unique composition, targeting ability and biocompatibility.6
Cell-specific drug delivery holds immense significance in improving the therapeutic outcomes for various disorders. By targeting specific cells or tissues, drugs can be delivered directly to the site of action, bypassing the barriers encountered by conventional delivery systems. One way to achieve cell-specific drug delivery is to use cell-based drug delivery systems.7 These systems use cells such as mesenchymal stem cells and macrophages to deliver drugs to specific cells. This method offers many benefits, including reduced side effects, improved effectiveness and improved patient compliance. By reducing drug exposure to healthy tissues and organs, cell-specific drug delivery systems improve treatment selectivity and specificity, thus reducing the potential for side effects.8 Exosomes are membrane organelles of smaller size and are secreted endogenously by various cell types. Exosomes, which contain a variety of biomolecules, including lipids, proteins and genetic material, have a diameter of 30 to 150 nm and show their cell origin.9 Exosomes play a crucial role in cellular communication, facilitating the transportation of diverse cargo such as lipids, proteins and nucleic acids. They mediate communication between progenitor cells and neighboring or distant cells in response to external stimuli, contributing to the transfer of lipids, proteins and nucleic acids.10 Naturally released by cells in the nano- to micrometer range, Extracellular Vesicles (EVs) enclosed in fluid bilayers are inherent byproducts of normal cellular physiology and emerge as significant contributors in instances of aberrant cellular behavior.11 These nanovesicles mimic the size and structure of exosomes, allowing them to evade the immune system and avoid opsonization, enhancing the stability and efficacy of drug delivery. Cell-specific drug delivery has gained significant attention in recent years due to its potential to address the limitations of conventional drug delivery methods.12 By targeting specific cells or tissues, drugs can be delivered directly to the site of action, resulting in improved pharmacokinetics, bioavailability and therapeutic outcomes. This approach is particularly beneficial for diseases with limited treatment options, such as cancer and neurodegenerative disorders. Additionally, cell-specific drug delivery holds promise for personalized medicine, allowing the delivery of tailored therapies tailored to the unique characteristics of each patient.10,9
Exosomes and lipid nanovesicles
Extracellular Vesicles (EVs) are microvesicles derived from cells, which are composed mainly of lipids. These vesicles transport biologically active substances, including nucleic acids and proteins and facilitate intercellular communication. Exosomes are nanoscale extracellular organs that are essential for intercellular communication.8 Different cell types secrete these membrane structures in extracellular space, usually between 30 and 150 nanometers in diameter. Exosomes transport many bioactive molecules, including lipids, metabolites, proteins and nucleic acids (including RNA and DNA fragments).12 The process by which they originate consists of endosomal membranes that grow inside, resulting in the formation of multi-vesicular bodies that, when merged with the cell membrane, release exosomes.7 Exosomes function as intermediaries in intercellular communication and enable the transmission of biological data between cells.11 Their involvement in physiological processes and pathological conditions, such as immune response modulation, tumor progression and tissue regeneration, has sparked interest in harnessing their properties for therapeutic applications.13
Composition of Exosome-Inspired Lipid Nanovesicles
The effectiveness, compatibility with biological systems and the ability to target specific areas are heavily influenced by the design and composition of drug delivery systems.14 In the field of nanomedicine, lipid nanovesicles inspired by exosomes have emerged as a promising platform. These nanovesicles mimic the natural characteristics of exosomes, offering versatility and precise control in drug delivery.8 Understanding the intricate composition of these lipid nanovesicles is crucial for harnessing their potential in various therapeutic applications. At the core of exosome-inspired lipid nanovesicles is the lipid bilayer, a fundamental structural element shared with natural exosomes.12 This lipid bilayer serves as a protective shell encapsulating the cargo, providing stability and compatibility with biological environments. The choice of lipids for constructing this bilayer is a critical consideration, as it not only influences the physical properties of the nanovesicles but also dictates their interactions with target cells.23 The lipid composition typically includes phospholipids, cholesterol and sphingolipids, mirroring the diversity found in biological membranes.14 Phospholipids are essential building blocks, forming the basic structure of the lipid bilayer. Cholesterol, with its unique role in membrane fluidity and stability, contributes to the overall robustness of the nanovesicle. Sphingolipids, characterized by their role in cell signaling and recognition, further enhance the biomimetic nature of exosome-inspired lipid nanovesicles.25 The careful selection and combination of these lipids enable researchers to tailor the physical and chemical properties of the nanovesicles.15 The lipid composition determines crucial factors such as size, surface charge and stability, which in turn influence the pharmacokinetics and biodistribution of the nanovesicles in vivo. In addition to the lipid bilayer, the cargo encapsulated within exosome-inspired lipid nanovesicles contribute significantly to their therapeutic potential.9 These nanovesicles are engineered to encapsulate a diverse array of bioactive molecules, including small-molecule drugs, nucleic acids, proteins and imaging agents. This versatility allows for the development of multifunctional drug delivery systems that can address a spectrum of diseases. Surface modifications further enhance the functionality of exosome-inspired lipid nanovesicles.10 By incorporating specific ligands, antibodies, or peptides onto the surface, researchers can impart targeting capabilities to these nanovesicles.9 This targeted delivery approach is particularly advantageous in directing therapeutic agents to specific cell types or tissues, improving the precision and efficiency of drug delivery.2 The composition of exosome-inspired lipid nanovesicles extends beyond the physical components to include surface proteins and ligands that mimic the natural recognition and binding mechanisms observed in exosomes. This biomimetic approach enhances the nanovesicles’ ability to interact with target cells, facilitating cellular uptake and intracellular delivery of the encapsulated cargo.16,17
Role of Lipids in Drug Delivery Systems
The role of lipids in drug delivery systems is a multifaceted and critical aspect that significantly influences the design, functionality and efficacy of therapeutic nanoparticles.12 Lipids, as essential components of cell membranes, have been harnessed in the development of innovative drug delivery systems, including liposomes, micelles and more recently, exosome-inspired lipid nanovesicles.18 This deep dive into the role of lipids in drug delivery systems unravels the intricacies of their impact on the physicochemical properties, targeting capabilities and therapeutic outcomes of these nanocarriers. Lipids serve as the building blocks of the lipid bilayer that constitutes the structural foundation of drug delivery vehicles.7,19 The lipid bilayer imparts stability to these nanocarriers, preventing premature leakage of encapsulated therapeutic agents.2,20 The choice of lipids profoundly influences the membrane’s fluidity, permeability and responsiveness to environmental cues, crucial factors in ensuring effective drug delivery. Additionally, the lipophilic nature of many drugs aligns seamlessly with lipids, enabling their efficient encapsulation within the lipid bilayer or the hydrophobic core of lipid-based nanoparticles.12 The diversity in lipid composition allows for the tailoring of drug delivery systems to specific applications. For instance, the incorporation of cholesterol enhances the stability and rigidity of liposomes, contributing to prolonged circulation times in the bloodstream.21 On the other hand, the inclusion of unsaturated lipids introduces flexibility, influencing membrane fluidity and drug release kinetics. Such control over lipid composition empowers researchers to fine-tune drug delivery systems for optimal performance, considering factors like biocompatibility, biodegradability and release profiles.15,22
Methods of exosome-inspired lipid nanovesicle synthesis
Isolation and Purification of Exosomes
Exosomes typically consist of proteins, lipids and nucleic acids. In contrast to membranes of other Extracellular Vesicles (EVs), the exosome membrane primarily comprises lipid layers, encompassing cholesterol, sphingolipids, ceramide and diacylglycerol.23 The process of creating lipid nanovesicles, inspired by exosomes, initiates with the extraction and purification of exosomes. These natural vesicles serve as the structural blueprint for the production of these synthetic nanoparticles.24 Exosomes are extracted from biological sources, including cell culture supernatants and biofluids, during isolation. Ultracentrifugation, density gradient centrifugation and size exclusion chromatography are all common methods. These techniques, by leveraging the unique physical and biochemical properties of exosomes, allow them to separate from extracellular vesicles and cell waste.25 Purification is a critical step to obtain a homogeneous population of exosomes with minimal contamination. The choice of isolation and purification methods depends on the desired characteristics of the final lipid nanovesicles, including size, surface charge and cargo content.1,26 Additionally, advances in microfluidics and immunocapture techniques offer innovative approaches to enhance the efficiency and specificity of exosome isolation, contributing to the reproducibility and scalability of the synthesis process.15
Lipid Nanovesicle Formulation Techniques
Exosomes contain various nucleic acids, including double-stranded DNA (dsDNA), messenger RNAs (mRNAs) and microRNAs (miRNAs).27 To synthesize exosome-inspired lipid nanovesicles, it is necessary to formulate lipid bilayers that mimic the structural characteristics of natural exosomes once purified exosomes have been obtained.28 Lipid nanovesicle formulation techniques encompass a variety of methods that dictate the size, morphology and surface properties of the resulting nanoparticles.
Liposome Extrusion
Liposome extrusion is a powerful and versatile technique used in the synthesis of lipid nanovesicles; particularly liposomes.18 In this process, a lipid mixture is forced through a porous membrane using controlled extrusion, resulting in the formation of uniform-sized liposomes. This method provides precise control over the size distribution of liposomes, a crucial factor in ensuring reproducibility and optimizing their performance in drug delivery.29 By adjusting the pore size of the membrane and the number of extrusion cycles, researchers can tailor the size of liposomes to meet specific requirements.10 Liposome extrusion is favored for its simplicity, scalability and ability to produce nanoscale liposomes with enhanced stability, making it a cornerstone in the development of advanced drug delivery systems.30
Sonication
Sonication, a pivotal technique in lipid nanovesicle formulation, harnesses ultrasonic waves to disrupt lipid aggregates and create nanoscale vesicles.12 One common application of probe sonication involves inducing the creation of temporary pores or even the disintegration and subsequent reassembly. This method involves subjecting lipid dispersion to ultrasonic energy, inducing cavitation and shear forces that lead to the formation of smaller lipid vesicles.23 While sonication is effective for creating nanovesicles, it is particularly useful for applications where precise control over size distribution is not the primary concern. The loading efficiency is documented to be notably elevated. Surprisingly, the intense localized energy may result in heating and potential harm to agents in certain instances.31 This technique is advantageous for encapsulating therapeutic agents within the lipid bilayer, especially hydrophobic drugs. Although widely utilized, sonication may exhibit some limitations in achieving uniformity compared to more controlled methods like extrusion.32 Nonetheless, its simplicity and versatility make sonication a valuable tool in the synthesis of lipid nanovesicles for various drug delivery applications.16 Haney et al. (2020) found that the loading efficiency of Paclitaxel (PTX) in exosomes was higher when sonication was performed only with the “off” cooling period compared to the cooling of the whole treatment. This could be due to the slightly elevated temperature, which reduced the rigidity of the lipid layers. Furthermore, when pH approached the Isoelectric point (pI), DOX encapsulation was considerably improved. This was accompanied by a reduction in the charge and an increase in the hydrophobia of the drug load exosomes. The co-culture of the mixture at Room Temperature (RT) for an hour after sonication can be used to rebuild the exosome membrane and then improve its stability.33
Reverse Phase Evaporation
Reverse Phase Evaporation (RPE) is a sophisticated technique used in the synthesis of lipid nanovesicles, particularly in the development of drug delivery systems. In this method, lipids are emulsified in an organic solvent, creating a water-in-oil emulsion.28 Subsequent removal of the organic solvent through evaporation leads to the spontaneous formation of nanovesicles. RPE is advantageous for encapsulating hydrophobic drugs within the lipid bilayer and offers control over vesicle size and composition.19 This approach provides a stable and reproducible means of producing lipid nanovesicles with a consistent size distribution, making it suitable for large-scale manufacturing. RPE has proven to be a valuable tool in pharmaceutical research, enabling the design of drug delivery systems with enhanced drug encapsulation efficiency and controlled release kinetics.34
Microfluidic Technologies
Microfluidic technologies represent a revolutionary approach in the synthesis of exosome-inspired lipid nanovesicles. Leveraging microscale channels and controlled fluid flows, these technologies offer precise control over the self-assembly process, enabling the creation of homogeneous lipid nanovesicles.11 Microfluidic devices facilitate the manipulation of parameters such as flow rates, mixing conditions and lipid concentrations, ensuring reproducibility and scalability in the synthesis process. This innovative method enhances the uniformity of particle size distribution, a critical factor in optimizing drug delivery characteristics.22 Microfluidic technologies not only contribute to the efficiency of lipid nanovesicle synthesis but also hold promise for tailoring these nanocarriers to specific therapeutic applications, marking a significant advancement in the field of precision medicine and targeted drug delivery.35
Saponin-assisted permeation
Saponin-assisted permeation refers to the use of saponins, a class of naturally occurring amphiphilic glycosides, to enhance the permeability of biological membranes. Saponins are often derived from plant sources and are known for their ability to form complexes with cholesterol and other lipids present in cell membranes.36 In the context of drug delivery or molecular studies, saponin-assisted permeation serves as a valuable tool to overcome barriers such as the cell membrane or the blood-brain barrier. The interaction of saponins with lipid components in the membranes leads to the formation of pores or disruptions in the lipid bilayer, temporarily increasing membrane permeability.15 This process allows for improved delivery of drugs, genetic material, or other bioactive compounds into cells or tissues that might otherwise restrict their entry. Saponin-assisted permeation is employed in various biomedical applications, including enhancing the bioavailability of therapeutic agents and facilitating the delivery of macromolecules for research purposes.37
Incorporation of Therapeutic Agents
The incorporation of therapeutic agents into exosome-inspired lipid nanovesicles is a crucial step to transform these synthetic carriers into effective drug delivery systems. Various strategies are employed to load therapeutic cargo into the lipid nanovesicles while maintaining their structural integrity.38
Passive Encapsulation
Passive encapsulation is a pivotal strategy in drug delivery, involving the spontaneous incorporation of therapeutic agents into lipid-based nanovesicles during their formulation.39 In this process, drugs, whether hydrophobic or hydrophilic, become entrapped within the lipid bilayer or the hydrophobic core of the nanovesicles without the need for additional external forces.19 This method is particularly suitable for lipophilic compounds, as they seamlessly integrate into the lipid environment. However, for hydrophilic drugs, the efficiency of passive encapsulation may be lower.40 Despite this, passive encapsulation stands as a versatile and widely employed technique due to its simplicity and applicability, contributing to the creation of drug-loaded lipid nanovesicles with controlled release capabilities and enhanced therapeutic potential.11
Active Loading
Active loading is a sophisticated technique in drug delivery systems that involves the targeted and controlled incorporation of therapeutic agents into nanocarriers.22 This method leverages transmembrane gradients, such as pH or ion concentration differentials, to actively drive the uptake of drugs into nanoparticles. Unlike passive encapsulation, active loading enhances the loading efficiency, enabling a higher payload of therapeutic agents within the nanocarrier.41 This approach is particularly advantageous for both hydrophobic and hydrophilic compounds, ensuring optimal drug encapsulation while preserving the structural integrity of the nanocarrier.42 Active loading strategies contribute to the precision and efficacy of drug delivery systems, allowing researchers to tailor formulations for specific applications and optimize therapeutic outcomes in areas such as cancer treatment, where precise and controlled drug release is paramount.15
Post-Loading Modification
Post-loading modification is a strategic approach in the synthesis of lipid nanovesicles, allowing for the customization and optimization of therapeutic cargo after the initial formation of the nanoparticles.43 In this method, preformed lipid nanovesicles undergo modifications, typically through surface functionalization or conjugation, to incorporate therapeutic agents or targeting ligands.17 This approach offers versatility in tailoring the nanovesicles for specific applications, enabling the attachment of diverse therapeutic molecules or ligands to the nanoparticle surface.44 Post-loading modification enhances the adaptability of lipid nanovesicles, addressing challenges associated with loading efficiency and cargo compatibility.45 As a result, this technique plays a pivotal role in the development of advanced drug delivery systems, providing a platform for controlled release and targeted delivery in the field of nanomedicine.10
Recent developments in exosome-inspired lipid nanovesicles
Although there are reports of the use of exosomes as diagnostic instruments for diseases, the fascinating biological properties and functions of endogenous exosomes have motivated further scientific and clinical research. Exosomes are small vesicles secreted by immune cells, neurons and cancerous cells.21 The considerable interest in these vesicles stems from their potential as drugs delivery vehicles. Recently, scientists have made progress in the form of lipid nanovesicles inspired by Exosomes (ExoLNVs), whose aim is to duplicate the properties and operations of exosomes.46 These nanovesicles offer several advantages for drug delivery applications. Recent developments in exosome-inspired lipid nanovesicles have significantly advanced the field of targeted drug delivery.3,7 These nanovesicles, which mimic the natural properties of exosomes, hold great promise for enhancing the stability, targeting capacity and safety of drug delivery systems.47 Several engineering strategies have been employed to improve the performance of these nanovesicles, including enhanced stability, surface modification for targeted delivery, loading and release of therapeutic cargo and biocompatibility and safety considerations.15,6
Engineering Strategies for Enhanced Stability
One of the key challenges in utilizing exosomes for drug delivery is their limited stability and short circulation time in the blood. To overcome this limitation, researchers have employed engineering strategies to enhance the stability and circulation of Exosome-Inspired Lipid Nanovesicles (ExoLNVs).48 Lipid modification stands out as a crucial approach, involving the incorporation of synthetic lipids or modification of the lipid composition to optimize stability and prolong circulatory lifetimes. Additionally, the incorporation of biodegradable polymers into the lipid bilayer enhances both stability and targeting capabilities.17 Another innovative strategy involves encapsulating ExoLNVs within liposomes, creating hybrid delivery systems that combine the advantageous properties of both lipid nanovesicles and liposomes. These engineering strategies reflect a deep understanding of the intricate balance required for creating stable and effective drug delivery platforms.49
Surface Modification for Targeted Delivery
Targeting specific tissues or cells within the body is crucial for effective drug delivery using ExoLNVs. Surface modifications play a pivotal role in achieving targeted delivery by attaching specific ligands to the ExoLNV surface.50 Antibodies, aptamers and carbohydrates are commonly used ligands, each offering unique advantages. Antibodies, when conjugated to the ExoLNV surface, enable precise binding to specific receptors on target cells. Aptamers, synthetic RNA or DNA molecules, provide another avenue for targeted delivery by covalently attaching to the ExoLNV surface.10 Additionally, carbohydrates, such as sugars or lectins, grafted onto the ExoLNV surface facilitate targeted delivery through carbohydrate-lectin interactions. These surface modifications exemplify a deep understanding of molecular interactions, enabling the design of ExoLNVs with remarkable specificity in recognizing and interacting with target cells.21
Loading and Release of Therapeutic Cargo
Exosome-inspired lipid nanovesicles offer flexibility in loading and releasing therapeutic cargo, showcasing deep insights into optimizing drug delivery. Entrapment of small drug molecules within the lipid bilayer allows for controlled release and targeted delivery.9,51 Formulating ExoLNVs as syringe-injectable formulations facilitates parenteral administration, directly injecting them into the bloodstream. Polymer encapsulation, where therapeutic agents are encapsulated within biodegradable polymers incorporated into the lipid bilayer of ExoLNVs, enables controlled release and targeted delivery.52 These loading and release strategies demonstrate a profound understanding of nanocarrier dynamics, providing avenues for tailoring cargo delivery profiles to meet specific therapeutic requirements.53
Biocompatibility and Safety Considerations
Ensuring the biocompatibility and safety of ExoLNVs is paramount for their clinical translation, reflecting a comprehensive understanding of potential risks. Selective lipid incorporation involves choosing biocompatible lipid components to minimize potential toxicity and enhance overall biocompatibility.54 The incorporation of biodegradable polymers into the lipid bilayer further emphasizes safety, as these polymers contribute to the biocompatibility of ExoLNVs. Safety testing and extensive characterization studies play a critical role in assessing the safety profile of ExoLNVs before clinical application, demonstrating a thorough comprehension of the regulatory requirements and ethical considerations associated with advancing these nanovesicles toward clinical use.6,55 This commitment to safety underscores the deep knowledge guiding the development of ExoLNVs as safe and effective drug delivery systems.
Applications of exosome-inspired lipid nanovesicles in cell-specific drug delivery
Exosome-inspired Lipid Nanovesicles (ExoLNVs) have emerged as promising vehicles for cell-specific drug delivery, demonstrating versatility in various therapeutic areas. Their unique properties make them particularly suitable for addressing challenges in precision medicine. Here’s a deep insight into their applications across different medical domains:
Cancer Treatment
Targeted Therapy
Exosome-inspired Lipid Nanovesicles (ExoLNVs) represent a transformative advancement in targeted cancer therapy.56 Exosomes exhibit considerable potential as nanocarriers for a wide range of therapeutic applications due to their exceptional capability of traversing biological barriers and navigating into tissue regions devoid of blood supply.57 Their surface modification capabilities enable precise targeting of cancer cells, a crucial aspect in overcoming the limitations of conventional treatments. By incorporating specific ligands, such as antibodies or aptamers, onto the surface of ExoLNVs, researchers achieve selective recognition and binding to cancer cell receptors.29 This targeted approach enhances drug delivery accuracy, reducing the likelihood of off-target effects and systemic toxicity. As the field progresses, the integration of patient-specific markers into ExoLNVs holds the potential to revolutionize cancer treatment by tailoring therapies to individual molecular profiles, maximizing efficacy and minimizing adverse effects. This precision-driven approach positions ExoLNVs at the forefront of the quest for more effective and personalized cancer therapies.10,58
Combination Therapy
Combination therapy represents a groundbreaking application of Exosome-inspired Lipid Nanovesicles (ExoLNVs) in the realm of precision medicine. The deep knowledge underlying this approach lies in the intricacies of designing multifaceted treatment strategies within a single nanocarrier.59 ExoLNVs, with their customizable lipid bilayer and surface modifications, provide an ideal platform for encapsulating and delivering a synergistic combination of therapeutic agents.13,2 The scientific rationale for combination therapy involves targeting multiple pathways or cellular mechanisms implicated in disease progression. By encapsulating different types of drugs-such as chemotherapy agents, RNA-based therapeutics, or immunomodulators-within ExoLNVs, researchers aim to achieve complementary and enhanced therapeutic effects. This not only addresses the heterogeneity of diseases like cancer but also mitigates the risk of drug resistance.1,60
Neurological Disorders
Blood-Brain Barrier Penetration
Blood-Brain Barrier (BBB) penetration is a critical challenge in treating neurological disorders and Exosome-Inspired Lipid Nanovesicles (ExoLNVs) demonstrate profound capabilities in overcoming this obstacle.15 The BBB, designed to protect the brain, restricts the entry of many therapeutic agents, limiting effective treatments for conditions like neurodegenerative diseases. ExoLNVs, owing to their unique structural and surface properties, address this challenge by exploiting advanced knowledge in nanomedicine.6 Surface modifications, such as ligands or peptides, enable targeted recognition of BBB-specific receptors, facilitating enhanced cellular uptake. Additionally, the inherent ability of ExoLNVs to mimic natural exosomes aids in navigating the complex microenvironment of the BBB.61 This targeted and efficient BBB penetration mechanism not only allows for the delivery of therapeutic cargo to the brain but also holds promise for revolutionizing the treatment landscape of neurological disorders, where precise drug delivery is paramount for therapeutic success.62
Neuroprotective Effects
Neuroprotective effects of Exosome-Inspired Lipid Nanovesicles (ExoLNVs) represent a transformative facet in addressing neurological disorders. These effects arise from the intricate interplay between ExoLNVs and neural cells, showcasing the depth of knowledge in neurobiology.1,63 The lipid composition and surface modifications of ExoLNVs contribute to their ability to modulate cellular responses, promoting neuronal survival and mitigating neurodegenerative processes. By mimicking the natural functions of exosomes, ExoLNVs engage in complex interactions with neural cells, fostering a microenvironment conducive to cell protection.43 This dual role, both as drug carriers and neuroprotective entities, signifies a comprehensive understanding of the intricate neurobiology involved. As a result, ExoLNVs emerge as promising candidates not only for precise drug delivery to the brain but also for their inherent potential to counteract neurodegenerative mechanisms, offering a multifaceted approach to tackling the challenges associated with neurological disorders.64
Other Therapeutic Areas
Inflammatory Diseases
In the realm of inflammatory diseases, Exosome-Inspired Lipid Nanovesicles (ExoLNVs) demonstrate an intricate understanding of immunomodulation and targeted drug delivery.33 Inflammatory diseases, characterized by dysregulated immune responses, pose complex challenges in treatment. ExoLNVs, through surface modifications and customizable cargo loading, present a sophisticated approach to modulate immune responses and deliver anti-inflammatory agents.65,32 The lipid bilayer and surface ligands of ExoLNVs can be engineered to interact selectively with immune cells, directing therapeutic cargo precisely to inflamed sites.22 This precision in targeting, coupled with the immunomodulatory properties inherited from natural exosomes, signifies a profound comprehension of the intricate dynamics of inflammatory processes. As a result, ExoLNVs offer a promising avenue for tailored interventions, presenting a potential paradigm shift in the treatment landscape of inflammatory diseases.66
Metabolic Disorders
In the context of metabolic disorders, Exosome-Inspired Lipid Nanovesicles (ExoLNVs) showcase a deep understanding of the complex interplay between lipid metabolism and disease pathophysiology.41 Metabolic disorders, encompassing conditions like diabetes and obesity, demand precise interventions to regulate metabolic pathways.17 ExoLNVs, with their tailored lipid composition and surface properties, offer a sophisticated platform for targeted drug delivery. By addressing specific cell types involved in metabolic processes, ExoLNVs can deliver therapeutic agents with exceptional precision.24,67 The ability to modulate lipid interactions and cellular responses reflects a profound knowledge of the molecular intricacies of metabolic pathways.40 As metabolic disorders often entail multifaceted dysregulations, the versatility of ExoLNVs in accommodating various cargo types positions them as innovative carriers for personalized and effective interventions in the realm of metabolic diseases.68
Infectious Diseases
In the realm of infectious diseases, Exosome-Inspired Lipid Nanovesicles (ExoLNVs) showcase a sophisticated approach to targeted drug delivery and combating pathogens. Infectious diseases, characterized by microbial invasions, necessitate precise therapeutic interventions to minimize collateral damage and enhance treatment efficacy.55 ExoLNVs, with their customizable surface modifications, enable the specific targeting of infected cells or pathogens. Ligand conjugation and engineered lipid compositions facilitate interactions with microbial surfaces, enhancing the selective delivery of antimicrobial agents.32 Moreover, the versatility of ExoLNVs in encapsulating diverse cargo types, from antiviral drugs to genetic material, presents a comprehensive strategy to combat infections.69 This nuanced understanding of the host-pathogen dynamics and the ability to tailor drug delivery responses to specific infectious agents position ExoLNVs at the forefront of innovative approaches for more effective, targeted and personalized treatments in infectious diseases.22
Gene therapy
In the landscape of gene therapy, Exosome-Inspired Lipid Nanovesicles (ExoLNVs) offer a nuanced and advanced platform for precise and effective delivery of genetic material.2 Exosomes, which possess the ability to safeguard nucleic acids against degradation, seem to be optimal vehicles for nucleic acids (such as siRNAs and miRNAs) utilised in gene therapy.70 Gene therapy, aiming to correct or replace defective genes, demands sophisticated delivery systems to navigate biological barriers and deliver therapeutic genes to target cells. ExoLNVs, with their biomimetic lipid bilayer and customizable surface modifications, provide a vehicle for encapsulating and delivering genetic payloads.71,5 The ability to mimic natural exosomes enhances cellular uptake and facilitates the transfer of genetic material, ensuring efficient and targeted gene delivery. CRISPR/Cas9 can be delivered to cells more efficiently by exosomes than by current vehicles, which rely primarily on immunogenicity.38 This deep understanding of molecular interactions and the complex dynamics of genetic interventions positions ExoLNVs as promising carriers for the advancement of gene therapy, offering a potential avenue for treating genetic disorders and advancing personalized medicine through precise and controlled genetic interventions.72 Different Advanced Exosome-Inspired Lipid Nanovesicles (ExoLNVs) of small molecules, proteins and genetic substances toward various therapeutic applications are shown in Figure 1.
Gene silencing
In the realm of gene silencing, Exosome-Inspired Lipid Nanovesicles (ExoLNVs) emerge as advanced carriers for the targeted delivery of small interfering RNA (siRNA) or antisense oligonucleotides.73 Gene silencing technologies aim to downregulate the expression of specific genes, presenting a promising avenue for treating various genetic disorders and cancers. ExoLNVs, with their biomimetic lipid composition and customizable surface properties, provide a sophisticated platform for encapsulating and delivering gene-silencing agents.43 Through surface modifications, such as ligand conjugation, ExoLNVs achieve targeted delivery to specific cells or tissues, enhancing the precision of gene silencing interventions. The inherent ability of ExoLNVs to mimic natural exosomes further contributes to their cellular uptake and intracellular delivery efficiency.66 This depth of understanding in molecular interactions positions ExoLNVs as innovative carriers for advancing gene silencing technologies, potentially revolutionizing the treatment landscape for conditions driven by aberrant gene expression.74 Overview of Exosome-Inspired Lipid Nanovesicles (ExoLNVs) in Cancer Therapy are shown in Table 1.
Cargo | Origin of Exosomes | Target Cancer Type | Loading Method | Administration Route | Outcome |
---|---|---|---|---|---|
Chemotherapeutic drugs | Human mesenchymal stem cells (MSCs). | Various (Customized). | Encapsulation in lipid bilayer. | Systemic injection. | Enhanced drug delivery, reduced side effects.11 |
siRNA | HEK293 cells. | Breast cancer, Lung cancer. | Entrapment in lipid bilayer. | Local injection. | Gene silencing, targeted cancer therapy.12 |
Immunomo dulators. | Dendritic cells. | Melanoma | Surface modification with ligands. | Intratumoral injection. | Improved immune response, tumor regression.13 |
Small molecules. | Cancer cell-derived exosomes. | Ovarian cancer. | Incorporation during synthesis. | Intraperitoneal injection. | Enhanced intracellular delivery, targeted treatment.14 |
mRNA | Red blood cell-derived exosomes. | Pancreatic cancer. | Syringe-inj ectable formulations. | Systemic injection. | Efficient delivery of genetic material, potential for personalized therapy.15 |
Antibody-drug conjugates | Platelet-derived exosomes. | Leukemia | Covalent attachment to surface. | Intravenous injection. | Specific targeting, reduced off-target effects.16 |
Nanoparticles | Macrophage-derived exosomes. | Prostate cancer. | Co-loading during vesicle formation. | Intravenous injection. | Synergistic drug delivery, prolonged circulation time.17 |
Peptide therapeutics. | Tumor cell-derived exosomes. | Colorectal cancer. | Surface functionalization. | Intratumoral injection. | Targeted delivery, enhanced therapeutic efficacy.18 |
Radioactive agents. | Fibroblast-derived exosomes. | Thyroid cancer. | Incorporation during biogenesis. | Intravenous injection. | Improved imaging and targeted radiotherapy.19 |
Antigen-presenting proteins. | B-cell-derived exosomes. | Lymphoma | Loading through membrane fusion. | Subcutaneous injection. | Enhanced antigen presentation, improved immune response.20 |
Overview of Exosome-Inspired Lipid Nanovesicles (ExoLNVs) in Cancer Therapy.
Future directions in Exosome-inspired Lipid Nanovesicles (ExoLNVs)
Improving the stability and shelf life of lipid nanovesicles
Advancing the stability and shelf life of ExoLNVs is pivotal for their successful translation into clinical applications.44 Future research may focus on refining lipid compositions, exploring innovative lipid modifications and incorporating stabilizing agents to mitigate challenges related to aggregation, degradation and environmental influences.75 Furthermore, advancements in storage techniques, such as lyophilization or cryopreservation, could enhance the long-term stability of ExoLNV formulations.56 Additionally, a deeper understanding of the physicochemical factors influencing stability will guide the development of robust formulations, ensuring the reproducibility and reliability required for pharmaceutical use.76
Enhancing the targeted delivery of lipid nanovesicles
Precision in drug delivery remains a critical goal for optimizing therapeutic outcomes. Future directions in enhancing targeted delivery involve refining surface modifications and ligand conjugation strategies to improve specificity for diseased cells.77 Incorporating advanced targeting moieties, such as aptamers or peptide ligands, could further enhance the selectivity of ExoLNVs.12 Moreover, the exploration of stimuli-responsive systems, triggered by specific cues within the microenvironment, may enable more precise release of therapeutic cargo at the target site.26 Microscale and nanoscale engineering approaches, including microfluidics and nanoengineering, may offer unprecedented control over the synthesis and customization of ExoLNVs, elevating their targeting capabilities.78
Developing combination therapies utilizing lipid nanovesicles
The future landscape of therapeutic interventions is likely to embrace the concept of combination therapies using ExoLNVs. Integrating multiple therapeutic agents within a single nanovesicle platform can address the complexity of diseases with multifaceted pathologies.79 Research may explore the synergistic effects of combining different drugs, including chemotherapeutics, gene therapies, or immunomodulators, within ExoLNVs.46 Strategies for co-delivery may involve optimizing loading techniques, controlling release kinetics and assessing the synergistic interactions between co-encapsulated agents.56 The customization of combination therapies using ExoLNVs holds promise for improving treatment efficacy, minimizing side effects and overcoming challenges associated with drug resistance, presenting a forward-looking direction in the development of advanced and multifunctional drug delivery systems.4,80
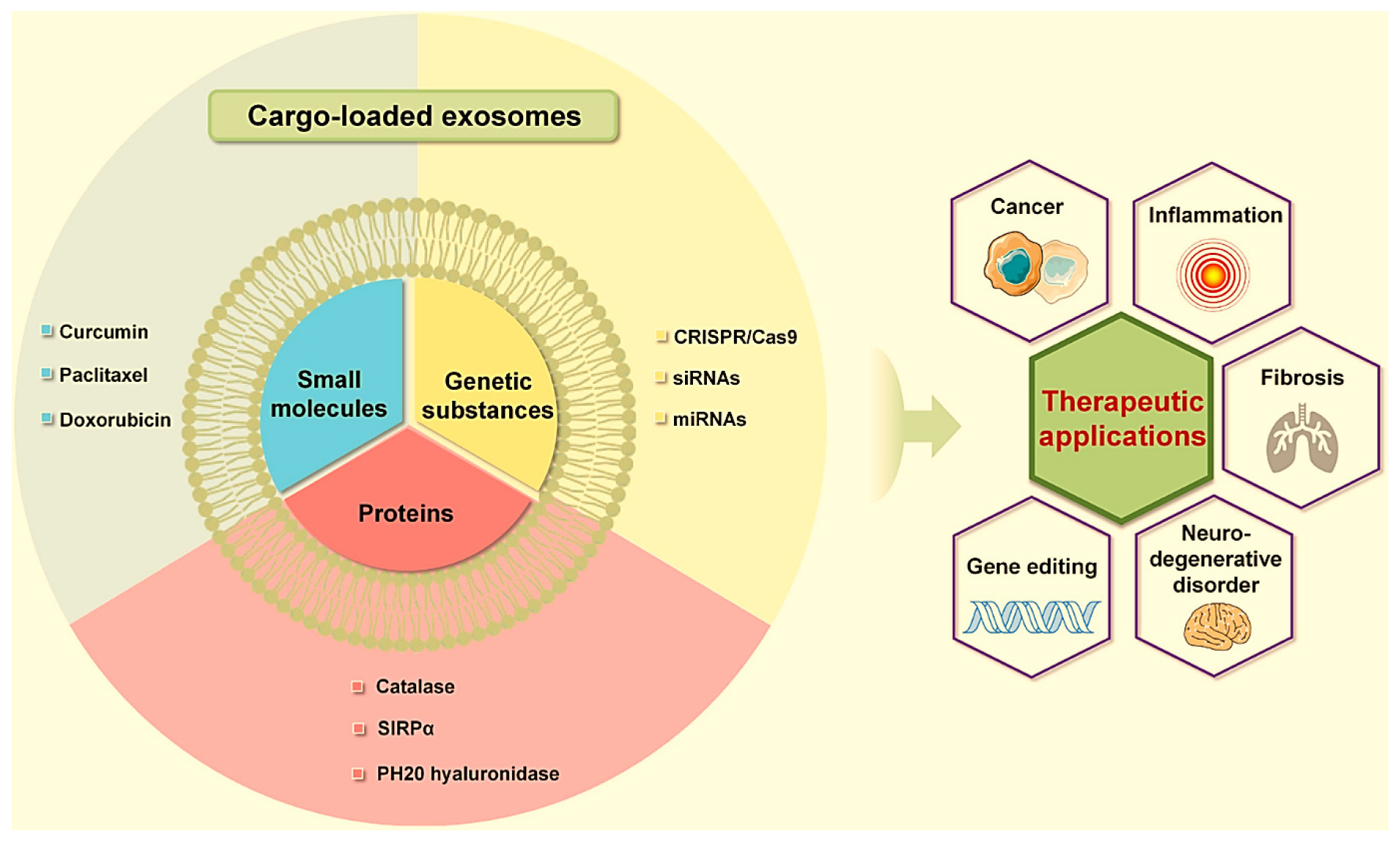
Figure 1:
Advanced Exosome-Inspired Lipid Nanovesicles (ExoLNVs) of small molecules, proteins and genetic substances toward various therapeutic applications.44
CONCLUSION
In the exploration of Exosome-Inspired Lipid Nanovesicles (ExoLNVs), significant findings underscore a transformative landscape in targeted drug delivery. Leveraging insights from natural exosomes, ExoLNVs exhibit versatility across applications, emphasizing their potential in cancer treatment through targeted and combination therapies, optimizing efficacy while minimizing side effects. The unique ability of ExoLNVs to traverse the blood-brain barrier holds promise for neurological disorders, not only facilitating precise drug delivery but also demonstrating inherent neuroprotective effects. Addressing inflammatory diseases, metabolic disorders, infectious diseases and gene therapy, ExoLNVs showcase a multifaceted prowess in targeted interventions. Future research aims to enhance stability and shelf life, refine targeted delivery strategies and develop combination therapies, offering a promising frontier. As ExoLNVs stand at the forefront of nanomedicine, their potential impact across various medical domains heralds a transformative era in the evolution of targeted and personalized medicine, necessitating interdisciplinary collaboration for successful translation from discovery to application.
Cite this article:
Sunkara SP, Kar NR, Kareemulla S, Sarma KN, Thool KU, Katual MK, et al. Recent Advancement in Exosome-Inspired Lipid Nanovesicles for Cell-Specific Drug Delivery. Int. J. Pharm. Investigation. 2024;14(4):1085-95.
ABBREVIATIONS
ExoLNVs | Exosomes inspired lipid nanovesicles |
---|---|
Evs | Extracellular vesicles |
DDS | Drug delivery systems |
dsDNA | Double stranded DNA |
mRNAs | Messenger RNAs |
miRNAs | Micro-RNA |
BBB | Blood-Brain Barrier |
References
- Théry C, Witwer KW, Aikawa E, Alcaraz MJ, Anderson JD, Andriantsitohaina R, et al. Minimal information for studies of extracellular vesicles 2018 (MISEV2018): A position statement of the International Society for Extracellular Vesicles and update of the MISEV2014 guidelines. J Extracell Vesicles. 2018;7(1):1535750 [PubMed] | [CrossRef] | [Google Scholar]
- Raposo G, Stoorvogel W. Extracellular vesicles: exosomes, microvesicles and friends. J Cell Biol. 2013;200(4):373-83. [PubMed] | [CrossRef] | [Google Scholar]
- Yang Y, Hong Y, Cho E, Kim GB, Kim IS. Extracellular vesicles as a platform for membrane-associated therapeutic protein delivery. J Extracell Vesicles. 2018;7(1):1440131 [PubMed] | [CrossRef] | [Google Scholar]
- Wang M, Zhao J, Zhang L, Wei F, Lian Y, Wu Y, et al. Role of tumor microenvironment in tumorigenesis. J Cancer. 2017;8(5):761-73. [PubMed] | [CrossRef] | [Google Scholar]
- Liu C, Su C. Design strategies and application progress of therapeutic exosomes. Theranostics. 2019;9(4):1015-28. [PubMed] | [CrossRef] | [Google Scholar]
- Sun YZ, Ruan JS, Jiang ZS, Wang L, Wang SM. Extracellular vesicles: A new perspective in tumor therapy. BioMed Res Int. 2018;2018:2687954 [PubMed] | [CrossRef] | [Google Scholar]
- Bhatt P, Kumar V, Subramaniyan V, Nagarajan K, Sekar M, Chinni SV, et al. Plasma modification techniques for natural polymer-based drug delivery systems. Pharmaceutics. 2023;15(8) [PubMed] | [CrossRef] | [Google Scholar]
- Bhatt P, Kumar V, Rastogi H, Malik MK, Dixit R, Garg S, et al. Functional and tableting properties of alkali-isolated and phosphorylated barnyard millet () starch. ACS Omega. 2023;8(33):30294-305. [PubMed] | [CrossRef] | [Google Scholar]
- Mulcahy LA, Pink RC, Carter DR. Routes and mechanisms of extracellular vesicle uptake. J Extracell Vesicles. 2014;3:24641 [PubMed] | [CrossRef] | [Google Scholar]
- Purushothaman A, Bandari SK, Liu J, Mobley JA, Brown EE, Sanderson RD, et al. Fibronectin on the surface of myeloma cell-derived exosomes mediates exosome-cell interactions. J Biol Chem. 2016;291(4):1652-63. [PubMed] | [CrossRef] | [Google Scholar]
- Soltani F, Parhiz H, Mokhtarzadeh A, Ramezani M. Synthetic and biological vesicular nano-carriers designed for gene delivery. Curr Pharm Des. 2015;21(42):6214-35. [PubMed] | [CrossRef] | [Google Scholar]
- Gutiérrez-Vázquez C, Villarroya-Beltri C, Mittelbrunn M, Sánchez-Madrid F. Transfer of extracellular vesicles during immune cell-cell interactions. Immunol Rev. 2013;251(1):125-42. [PubMed] | [CrossRef] | [Google Scholar]
- Haney MJ, Klyachko NL, Zhao Y, Gupta R, Plotnikova EG, He Z, et al. Exosomes as drug delivery vehicles for Parkinson’s disease therapy. J Control Release. 2015;207:18-30. [PubMed] | [CrossRef] | [Google Scholar]
- Whiteside TL. Immune modulation of T-cell and NK (natural killer) cell activities by TEXs (tumour-derived exosomes). Biochem Soc Trans. 2013;41(1):245-51. [PubMed] | [CrossRef] | [Google Scholar]
- Kawikova I, Askenase PW. Diagnostic and therapeutic potentials of exosomes in CNS diseases. Brain Res. 2015;1617:63-71. [PubMed] | [CrossRef] | [Google Scholar]
- Li X, Tsibouklis J, Weng T, Zhang B, Yin G, Feng G, et al. Nano carriers for drug transport across the blood-brain barrier. J Drug Target. 2017;25(1):17-28. [PubMed] | [CrossRef] | [Google Scholar]
- Sun D, Zhuang X, Xiang X, Liu Y, Zhang S, Liu C, et al. A novel nanoparticle drug delivery system: the anti-inflammatory activity of curcumin is enhanced when encapsulated in exosomes. Mol Ther. 2010;18(9):1606-14. [PubMed] | [CrossRef] | [Google Scholar]
- Prada I, Meldolesi J. Binding and fusion of extracellular vesicles to the plasma membrane of their cell targets. Int J Mol Sci. 2016;17(8):1296 [PubMed] | [CrossRef] | [Google Scholar]
- El Andaloussi S, Lakhal S, Mäger I, Wood MJ. Exosomes for targeted siRNA delivery across biological barriers. Adv Drug Deliv Rev. 2013;65(3):391-7. [PubMed] | [CrossRef] | [Google Scholar]
- Bhatt P, Kumar A, Shukla R. Nanorobots recent and future advances in cancer or dentistry therapy-A review. Am J Pharm. 2019;9(3):321-31. [CrossRef] | [Google Scholar]
- Bhatt P, Kumar V, Rastogi H, Malik MK, Dixit R, Garg S, et al. Functional and tableting properties of alkali-isolated and phosphorylated barnyard millet () starch. ACS Omega. 2023;8(33):30294-305. [PubMed] | [CrossRef] | [Google Scholar]
- Valapala M, Vishwanatha JK. Lipid raft endocytosis and exosomal transport facilitate extracellular trafficking of annexin A2. J Biol Chem. 2011;286(35):30911-25. [PubMed] | [CrossRef] | [Google Scholar]
- Das CK, Jena BC, Banerjee I, Das S, Parekh A, Bhutia SK, et al. Exosome as a novel shuttle for delivery of therapeutics across biological barriers. Mol Pharm. 2019;16(1):24-40. [PubMed] | [CrossRef] | [Google Scholar]
- Kim H, Wang SY, Kwak G, Yang Y, Kwon IC, Kim SH, et al. Exosome-guided phenotypic switch of M1 to M2 macrophages for cutaneous wound healing. Adv Sci (Weinh). 2019;6(20):1900513 [PubMed] | [CrossRef] | [Google Scholar]
- Reif S, Elbaum-Shiff Y, Koroukhov N, Shilo I, Musseri M, Golan-Gerstl R, et al. Cow and human milk-derived exosomes ameliorate colitis in DSS murine model. Nutrients. 2020;12(9):2589 [PubMed] | [CrossRef] | [Google Scholar]
- Zhu W, Huang L, Li Y, Zhang X, Gu J, Yan Y, et al. Exosomes derived from human bone marrow mesenchymal stem cells promote tumor growth in vivo. Cancer Lett. 2012;315(1):28-37. [PubMed] | [CrossRef] | [Google Scholar]
- Alqahtani MS, Kazi M, Alsenaidy MA, Ahmad MZ. Advances in oral drug delivery. Front Pharmacol. 2021;12:618411 [PubMed] | [CrossRef] | [Google Scholar]
- Ismail R, Bocsik A, Katona G, Gróf I, Deli MA, Csóka I, et al. Encapsulation in polymeric nanoparticles enhances the enzymatic stability and the permeability of the GLP-1 analog, liraglutide, across a culture model of intestinal permeability. Pharmaceutics. 2019;11(11):599 [PubMed] | [CrossRef] | [Google Scholar]
- Zhang R, Qin X, Kong F, Chen P, Pan G. Improving cellular uptake of therapeutic entities through interaction with components of cell membrane. Drug Deliv. 2019;26(1):328-42. [PubMed] | [CrossRef] | [Google Scholar]
- Peng Y, Chen L, Ye S, Kang Y, Liu J, Zeng S, et al. Research and development of drug delivery systems based on drug transporter and nano-formulation. Asian J Pharm Sci. 2020;15(2):220-36. [PubMed] | [CrossRef] | [Google Scholar]
- Charoenviriyakul C, Takahashi Y, Morishita M, Matsumoto A, Nishikawa M, Takakura Y, et al. Cell type-specific and common characteristics of exosomes derived from mouse cell lines: yield, physicochemical properties and pharmacokinetics. Eur J Pharm Sci. 2017;96:316-22. [PubMed] | [CrossRef] | [Google Scholar]
- Vader P, Mol EA, Pasterkamp G, Schiffelers RM. Extracellular vesicles for drug delivery. Adv Drug Deliv Rev. 2016;106(A):148-56. [PubMed] | [CrossRef] | [Google Scholar]
- Zhu X, Badawi M, Pomeroy S, Sutaria DS, Xie Z, Baek A, et al. Comprehensive toxicity and immunogenicity studies reveal minimal effects in mice following sustained dosing of extracellular vesicles derived from HEK293T cells. J Extracell Vesicles. 2017;6(1):1324730 [PubMed] | [CrossRef] | [Google Scholar]
- Li J, Chen X, Yi J, Liu Y, Li D, Wang J, et al. Identification and characterization of 293T cell-derived exosomes by profiling the protein, mRNA and microRNA components. PLOS ONE. 2016;11(9) [PubMed] | [CrossRef] | [Google Scholar]
- Shao J, Zaro J, Shen Y. Advances in exosome-based drug delivery and tumor targeting: from tissue distribution to intracellular fate. Int J Nanomedicine. 2020;15:9355-71. [PubMed] | [CrossRef] | [Google Scholar]
- Smyth T, Kullberg M, Malik N, Smith-Jones P, Graner MW, Anchordoquy TJ, et al. Biodistribution and delivery efficiency of unmodified tumor-derived exosomes. J Control Release. 2015;199:145-55. [PubMed] | [CrossRef] | [Google Scholar]
- Qiao L, Hu S, Huang K, Su T, Li Z, Vandergriff A, et al. Tumor cell-derived exosomes home to their cells of origin and can be used as Trojan horses to deliver cancer drugs. Theranostics. 2020;10(8):3474-87. [PubMed] | [CrossRef] | [Google Scholar]
- Bhatt P, Singh S, Alfuraiji N, Al-Snafi AE. Crispr CAS9: A new technology to modify genome-A review. Open J Syst Demonstr. J. 2022;8(4):208-15. [PubMed] | [CrossRef] | [Google Scholar]
- Singh S, Bhatt P, Kumar V, Singh NP. Advances in flavonoids for human health and prevention of diseases. 2024:23-46. [PubMed] | [CrossRef] | [Google Scholar]
- Munich S, Sobo-Vujanovic A, Buchser WJ, Beer-Stolz D, Vujanovic NL. Dendritic cell exosomes directly kill tumor cells and activate natural killer cells via TNF superfamily ligands. Oncoimmunology. 2012;1(7):1074-83. [PubMed] | [CrossRef] | [Google Scholar]
- Pitt JM, André F, Amigorena S, Soria JC, Eggermont A, Kroemer G, et al. Dendritic cell-derived exosomes for cancer therapy. J Clin Invest. 2016;126(4):1224-32. [PubMed] | [CrossRef] | [Google Scholar]
- Viaud S, Terme M, Flament C, Taieb J, André F, Novault S, et al. Dendritic cell-derived exosomes promote natural killer cell activation and proliferation: a role for NKG2D ligands and IL-15Ralpha. PLOS ONE. 2009;4(3) [PubMed] | [CrossRef] | [Google Scholar]
- Simhadri VR, Reiners KS, Hansen HP, Topolar D, Simhadri VL, Nohroudi K, et al. Dendritic cells release HLA-B-associated transcript-3 positive exosomes to regulate natural killer function. PLOS ONE. 2008;3(10) [PubMed] | [CrossRef] | [Google Scholar]
- Andre F, Escudier B, Angevin E, Tursz T, Zitvogel L. Exosomes for cancer immunotherapy. Ann Oncol. 2004;15(Suppl 4) [PubMed] | [CrossRef] | [Google Scholar]
- Sharma SK, Bhatt P, Asdaq SM, Alshammari MK, Alanazi A, Alrasheedi NS, et al. Combined therapy with ivermectin and doxycycline can effectively alleviate the cytokine storm of COVID-19 infection amid vaccination drive: A narrative review. J Infect Public Health. 2022;15(5):566-72. [PubMed] | [CrossRef] | [Google Scholar]
- Singh S, Pankaj B, Nagarajan K, Singh P. N, Bala V. Blockchain with cloud for handling healthcare data: A privacy-friendly platform. Mater Today. 2022;62:5021-6. [CrossRef] | [Google Scholar]
- Ahamed S, Bhatt P, Sultanuddin SJ, Walia R, Haque MA, InayathAhamed SB, et al. An Intelligent IoT enabled Health Care Surveillance using Machine Learning. 2022 [CrossRef] | [Google Scholar]
- Bhatt P, Singh S, Kumar Sharma S, Rabiu S. Development and characterization of fast dissolving buccal strip of frovatriptan succinate monoydrate for buccal delivery. Int J Pharm Investig. 2021;11(1):69-75. [CrossRef] | [Google Scholar]
- Al-Snafi AE, Singh S, Bhatt P, Kumar V. A review on prescription and non-prescription appetite suppressants and evidence-based method to treat overweight and obesity. GSC Biol PharmSci. 2022;19(3):148-55. [CrossRef] | [Google Scholar]
- Singh S, Bhatt P, Alfuraiji N, Thuwaini MM, Snafi AE. Cardiovascular comorbidity of COVID-19 disease: a review. WJPMR. 2022;8(4):216-25. [CrossRef] | [Google Scholar]
- Malik MK, Bhatt P, Kumar T, Singh J, Kumar V, Faruk A, et al. Significance of chemically derivatized starch as drug carrier in developing novel drug delivery devices. Nat Prod J. 2022:12 [CrossRef] | [Google Scholar]
- Pankaj . Anti-cancer cyclodextrin nanocapsules based formulation development for lung chemotherapy. J Pharm Res Int. 2021:54-63. [CrossRef] | [Google Scholar]
- Pankaj . Cyclodextrin modified block polymer for oral chemotherapy. J Pharm Res Int. 2021:21-9. [CrossRef] | [Google Scholar]
- Singh S, Kumar Sharma S, Mehrotra P, Bhatt P, Kaurav M. Blockchain technology for efficient data management in healthcare system: opportunity, challenges and future perspectives. Mater Today. 2022;62:5042-6. [CrossRef] | [Google Scholar]
- Malik MK, Bhatt P, Singh J, Kaushik RD, Sharma G, Kumar V, et al. Preclinical safety assessment of chemically cross-linked modified mandua starch: acute and sub-acute oral toxicity studies in Swiss albino mice. ACS Omega. 2022;7(40):35506-14. [PubMed] | [CrossRef] | [Google Scholar]
- Kaur T, Singh S. Controlled release of bi-layered malvidin tablets using 3D printing techniques. J Pharm Res Int. 2021:70-8. [CrossRef] | [Google Scholar]
- Bhatt P, Singh S, Sharma SK, Kumar V. Blockchain for healthcare systems. 2021:97-113. [CrossRef] | [Google Scholar]
- Singh S, Bhatt P, Sharma SK, Rabiu S. Blockchain for healthcare systems. 2021:61-79. [CrossRef] | [Google Scholar]
- Malik MK, Kumar V, Singh J, Bhatt P, Dixit R, Kumar S, et al. Phosphorylation of alkali extracted mandua starch by STPP/STMP for improving digestion resistibility. ACS Omega. 2023;8(13):11750-67. [PubMed] | [CrossRef] | [Google Scholar]
- Bhatt P, Kumar A, Shukla R. Nanorobots recent and future advances in cancer or dentistry therapy-A review. Am J Pharm. 2019;9(3):321-31. [CrossRef] | [Google Scholar]
- Sharma SK, Bhatt P. Controlled release of bi-layered EGCG tablets using 3D printing techniques. J Pharm Res Int. 2021:5-13. [CrossRef] | [Google Scholar]
- Kumar A, Bhatt P, Mishra N. Irritable bowel syndrome with reference of alosetron hydrochloride and Excipient profile used in the manufacturing of alosetron tablet-A review. J Chem Pharm Sci. 2019;12(3):71-8. [CrossRef] | [Google Scholar]
- Baskar C, Ramakrishna S, Daniela La Rosa A. Encyclopedia of green materials. 2022 [CrossRef] | [Google Scholar]
- Bhatt P, Singh S, Kumar V, Nagarajan K, Mishra SK, Kumar Dixit P, et al. Artificial intelligence in pharmaceutical industry: revolutionizing drug development and delivery. Chin J Artif Intell. 2023:02 [CrossRef] | [Google Scholar]
- Sahu KK, Kaurav M, Bhatt P, Minz S, Pradhan M, Khan J, et al. Nanotechnological aspects for next-generation wound management. 2024:101-30. [CrossRef] | [Google Scholar]
- Bhatt P, Kumar V, Subramaniyan V, Nagarajan K, Sekar M, Chinni SV, et al. Plasma modification techniques for natural polymer-based drug delivery systems. Pharmaceutics. 2023;15(8):2066 [PubMed] | [CrossRef] | [Google Scholar]
- Malik MK, Kumar V, Singh J, Bhatt P, Dixit R, Kumar S, et al. Phosphorylation of alkali extracted mandua starch by STPP/STMP for improving digestion resistibility. ACS Omega. 2023;8(13):11750-67. [PubMed] | [CrossRef] | [Google Scholar]
- Kaurav M, Kanoujia J, Gupta M, Goyal P, Pant S, Rai S, et al. In-depth analysis of the chemical composition, pharmacological effects, pharmacokinetics and patent history of mangiferin. Phytomed Plus. 2023;3(2):100445 [CrossRef] | [Google Scholar]
- Goyal C, Bhatt P, Rawat S, Kumar Sharma V, Rani Ahuja M. Estimation of shelf-life of Balachaturbhadrika syrup containing different sweetening agents. Res J Pharm Technol. 2022:5078-83. [CrossRef] | [Google Scholar]
- Ahamed S, Bhatt P, Sultanuddin SJ, Walia R, Haque MA, InayathAhamed SB, et al. An Intelligent IoT enabled Health Care Surveillance using Machine Learning. 2022 [CrossRef] | [Google Scholar]
- Ahmed V, Sharma S, Bhatt P. Formulation and evaluation of sustained release tablet of diltiazem hydrochloride. Int J Pharm Sci Res. 2020;11(5):2193-8. [CrossRef] | [Google Scholar]
- Bhatt P. Mouth dissolving tablets challenges, preparation strategies with a special emphasis on losartan potassium-A review. World J Pharm Pharm Sci. 2018;7(9):271-87. [CrossRef] | [Google Scholar]
- Wang X, He L, Huang X, Zhang S, Cao W, Che F, et al. Recent progress of exosomes in multiple myeloma: pathogenesis, diagnosis, prognosis and therapeutic strategies. Cancers. 2021;13(7):1635 [PubMed] | [CrossRef] | [Google Scholar]
- Campanella C, Caruso Bavisotto C, Logozzi M, Marino Gammazza A, Mizzoni D, Cappello F, et al. On the choice of the extracellular vesicles for therapeutic purposes. Int J Mol Sci. 2019;20(2):236 [PubMed] | [CrossRef] | [Google Scholar]
- Feng D, Zhao WL, Ye YY, Bai XC, Liu RQ, Chang LF, et al. Cellular internalization of exosomes occurs through phagocytosis. Traffic. 2010;11(5):675-87. [PubMed] | [CrossRef] | [Google Scholar]
- Mulcahy LA, Pink RC, Carter DR. Routes and mechanisms of extracellular vesicle uptake. J Extracell Vesicles. 2014;3:24641 [PubMed] | [CrossRef] | [Google Scholar]
- Clayton A, Turkes A, Dewitt S, Steadman R, Mason MD, Hallett MB, et al. Adhesion and signaling by B cell-derived exosomes: the role of integrins. FASEB J. 2004;18(9):977-9. [PubMed] | [CrossRef] | [Google Scholar]
- Kaur S, Singh SP, Elkahloun AG, Wu W, Abu-Asab MS, Roberts DD, et al. CD47-dependent immunomodulatory and angiogenic activities of extracellular vesicles produced by T cells. Matrix Biol. 2014;37:49-59. [PubMed] | [CrossRef] | [Google Scholar]
- Long KB, Beatty GL. Harnessing the antitumor potential of macrophages for cancer immunotherapy. Oncoimmunology. 2013;2(12) [PubMed] | [CrossRef] | [Google Scholar]
- Kamerkar S, LeBleu VS, Sugimoto H, Yang S, Ruivo CF, Melo SA, et al. Exosomes facilitate therapeutic targeting of oncogenic KRAS in pancreatic cancer. Nature. 2017;546(7659):498-503. [PubMed] | [CrossRef] | [Google Scholar]