ABSTRACT
Background
Staphylococcus aureus is an adaptive and versatile microorganism that can cause a wide range of ailments, from intense and short-lived infections to persistent infections that are difficult to cure. Even though S. aureus infections could once be treated with ordinary antibiotics, the rise of drug-resistant organisms is currently a major issue. Numerous antibiotics were used to treat Staphylococcus aureus infections, but over time, the bacteria eventually developed resistance to multiple drugs. Since then, Methicillin-resistant Staphylococcus aureus (MRSA) strain-related nosocomial infections have increased in frequency. Recent advances in bioinformatics and silico screening have boosted our rate and chances of discovering medicinal metabolites.
Objectives
In this study, we understand and analyse the binding efficiency of Staphylococcus aureus C0673 with three existing antibiotics employing molecular docking studies.
Materials and Methods
The genomic sequence of Staphylococcus aureus C0673 is retrieved from the Ensemble bacteria database (GCA_00 0638495) and docked with three currently prescribed antibiotics, i.e., Gentamicin, Linezolid and Norfloxacin using HDOCK server.
Results and Discussion
In the present study Gentamicin, Linezolid and Norfloxacin effectively bind with Staphylococcus aureus C0673. Based on the docking score, the efficiency of the compound against the bacterial protein was assessed. Gentamicin shows higher binding affinity when compared to the other two compounds. Hence, Gentamicin can be considered an eligible candidate by combining with novel medicines to treat the Multi-Drug Resistant protein of Staphylococcus aureus.
Conclusion
From this research investigation, we conclude that multidrug resistant antibiotics efficiently bind with Staphylococcus aureus C0673. The results obtained from this study play a major role in the field of current bacterial informatics studies.
INTRODUCTION
Staphylococcus aureus is a very prevalent invasive bacterial infection that is known to colonize large areas of the human population, resulting in significant morbidity and mortality on a global scale.1 S. aureus is a significant human pathogen that causes an extensive range of infectious diseases in both healthcare and public settings. The Gram-positive pathogen is loaded with a slew of virulence factors that contribute to the formation of infections in hosts.2
Staphylococcus aureus is an adaptive and versatile microorganism that can cause a wide range of ailments, from intense and short-lived infections to persistent infections that are difficult to cure.3 By the late 1960s, Methicillin-Resistant Staphylococcus aureus (MRSA) was endemic in hospitals; but, in the 1990s, it quickly and unexpectedly spread to communities and is now widespread globally.4 In addition to the environment and typical human flora, S. aureus can be detected on healthy individual skin and mucous membranes, most commonly in the nose region. On healthy skin, S. aureus usually does not cause illness; however, if allowed to penetrate the bloodstream or internal tissues, these germs can cause a variety of potentially serious illnesses.1
Multiple antibiotics were administered to treat S. aureus infections, but gradually Multidrug resistance of S. aureus started to become more common. Since then, nosocomial infections due to Methicillin-Resistant Staphylococcus aureus (MRSA) strains have become fairly common. Microorganisms undergo mutational changes in their chromosomal DNA or RNA to survive, which confers resistance. One well-known example is S. aureus resistance to Methicillin. Multiple resistant strains of S. aureus have been developed through evolution.5
Multi-Drug Resistance (MDR) is a global issue that is wreaking havoc on health care. Due to constant exposure to antimicrobial medications, bacteria are becoming resistant to antibiotic treatments. Microbial infections have significantly grown during the past ten years, and this has resulted in a surge in resistance.6 Gentamicin a bactericidal aminoglycoside drug is effective against both gram-positive and gram-negative bacteria, however, it is more useful for treating severe gram-negative infections. Linezolid is a synthetic antibiotic used to treat Gram-positive aerobic bacterial infections. It is bactericidal against most isolates of streptococci and bacteriostatic against staphylococci and enterococci. Norfloxacin is a quinolone monocarboxylic acid that has broad-spectrum anti-bacterial action against most gram-negative and gram-positive bacteria.7–9
Treatment for S. aureus infections is largely dependent on the kind of infection and whether drug-resistant strains are present or not. The type of infection and other factors have a major influence in defining the course and type of treatment when antimicrobial therapy is necessary.10 S. aureus infections are commonly treated by primary care physicians, internists, nurse practitioners, and infectious disease specialists. Identifying and treating drug-resistant strains is the primary goal of treatment. Prevention of S. aureus infections remains challenging. Despite their best attempts, scientists have not been able to provide regular vaccination against infections caused by S. aureus. As a result, efforts have relied on infection control measures including handwashing practices, hospital decontamination procedures, and MRSA transmission prevention standards.11
In fact, millions of compounds may now be virtually screened in a reasonable amount of time owing to in silico methods, which also reduce early hit identification costs and raise the likelihood of discovering the intended therapeutic candidates. Drug development efforts can currently be assisted by several molecular modeling techniques, the majority of which can be categorized into structure-based and ligand-based approaches.12 One of the most well-known and effective structure-based in silico techniques for forecasting molecular interactions with biological targets is molecular docking. This is commonly accomplished by first estimating the ligand’s molecular orientation within the receptor, and then using a scoring function to determine how complementary the two are.13
Antibiotics are required for the management of Staphylococcus aureus infections. Most antibiotics used to treat staphylococcal infections aim to interfere with essential bacterial processes, including transcription, translation, DNA synthesis, and cell wall production. However, antibiotic resistance is a growing problem, and ineffective therapies have enormous financial and human implications.14
MATERIALS AND METHODS
Step wise methodology of the present study
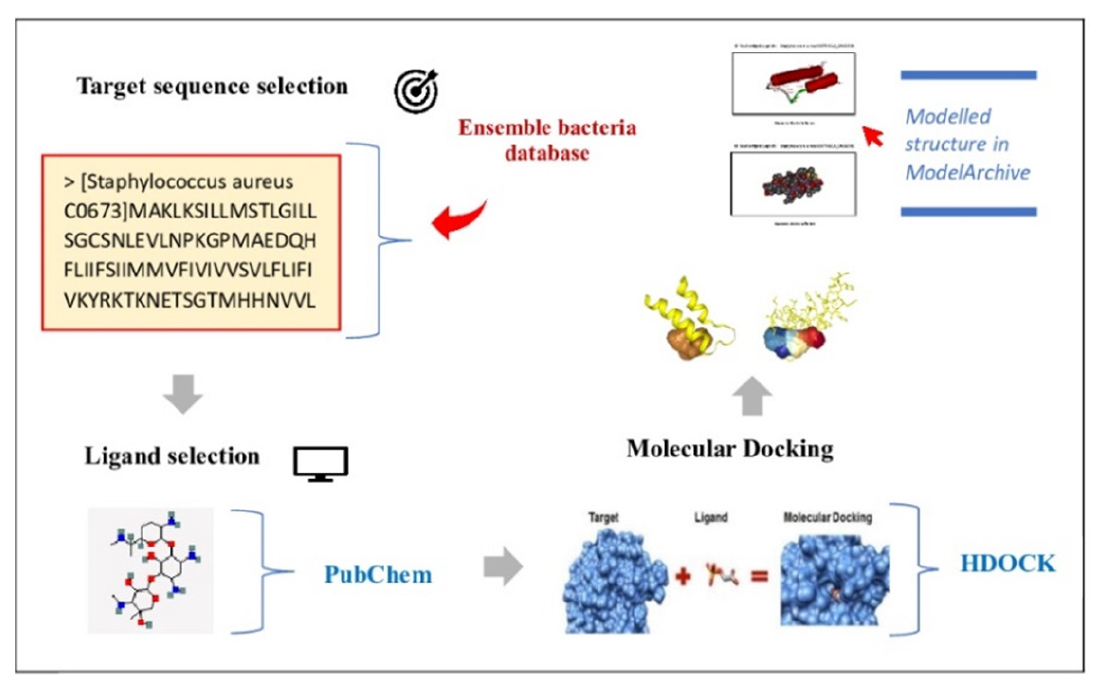
Micro-organism sequence selection
The genomic sequence of Staphylococcus aureus C0673 is retrieved from Ensemble bacteria database.15 (https://bacteria.ensembl.org/index.html) (GCA_00 0638495) for 3D modelling studies.
Ligand selection
Three chemical compounds (Gentamicin, Linezolid, Norfloxacin) with bactericidal properties were focused for molecular docking studies. Simplified molecular input line entry system (SMILES) format of the selected chemical compounds was retrieved from PubChem.16 (https://pubchem.ncbi.nlm.nih.go v/). Canonical SMILES (Simplified Molecular Input Line Entry System) of the three chemical compounds were deposited in Online SMILES Translator (https://cactus.nci.nih.gov/translate/) to get the PDB structure.
Molecular drug docking
Molecular docking is one of the most extensively used in silico drug discovery tools, investigating the optimum conformations of small molecules during interaction with target molecules. Three different chemical compounds and Staphylococcus aureus C0673 were subjected to docking tests.17 PDB file of the ligand and the receptor sequence (S. aureus) were submitted to HDOCK server18 (http://hdock.phys.hust.edu.cn/) to predicts the interaction between receptor and ligand molecules. HDOCK analysis also revealed data for receptor and ligand surface residues.
3D macromolecular visualization
Following molecular drug docking studies of three chemical compounds- Gentamicin, Linezolid and Norfloxacin with Staphylococcus aureus C0673, visualization of their 3D structure was done using molecular visualization tool called Discovery Studio.
RESULTS
Gentamicin, Linezolid and Norfloxacin compounds were docked with the modelled Staphylococcus aureus C0673 as shown in Table 1 in order to analyse the binding efficiency. Among the top 10 binding models generated by HDOCK server the one with the highest docking score as shown in Table 1 were chosen as it has more Binding affinity towards the Receptor. Docking results clearly shows that Gentamicin compound shows higher binding affinity with docking score of -122.78 kcal/mol in comparison to Linezolid and Norfloxacin with docking score of -106.71 kcal/mol, -103.85 kcal/mol respectively. The score simulates the potential energy change that could occur when the protein and ligand bind. Accordingly, a very negative score indicates a strong binding, whereas a number that is less negative or even positive indicates a weak or non-existent binding.19
Ligand | Receptor | Docking score (ACE) |
---|---|---|
Gentamicin | Staphylococcus aureus C0673 | -122.78 kcal/mol |
Linezolid | Staphylococcus aureus C0673 | -106.71 kcal/mol |
Norfloxacin | Staphylococcus aureus C0673 | -103.85 kcal/mol |
HDOCK a novel web server of template-based modeling and free docking server was employed in this study for docking Gentamicin, Linezolid and Norfloxacin compounds with the modelled Staphylococcus aureus C0673 as seen in Figures 1, 4 and 7 also illustrating drug binding affinities of Ligand and Receptor as seen in Tables 2, 3 and 4. Following the docking study, the 3-Dimensional (3D) structure of the ligand and receptor was viewed in molecular visualization tool called Discovery Studio as seen in Figures 2 and 3 for Gentamicin and Staphylococcus aureus C0673, Figures 5 and 6 for Linezolid and Staphylococcus aureus C0673, Figures 8 and 9 for Norfloxacin and Staphylococcus aureus C0673. Previous studies have proved the efficiency of HDOCK server.20–25
Ligand interface residue(s) | Receptor-ligand interface residue pair(s) |
---|---|
PHE 10A 3.406 | 0 – 10A 3.406 |
PHE 13A 2.691 | 0 – 13A 2.691 |
PHE 14A 2.531 | 0 – 14A 2.531 |
PHE 17A 2.866 | 0 – 17A 2.866 |
THR 19A 3.358 | 0 – 19A 3.358 |
ILE 24A 4.878 | 0 – 24A 4.878 |
ILE 25A 3.401 | |
Receptor interface residue(s) 0 2.531 |
Ligand interface residue(s) | Receptor-ligand interface residue pair(s) |
---|---|
TRP 3A 3.244 | 0-3A 3.244 |
MET 4A 4.165 | 0-4A 4.165 |
VAL 6A 4.120 | 0-6A 4.120 |
CYS 7A 1.751 | 0-7A 1.751 |
PHE 10A 2.782 | 0-10A 2.782 |
PHE 14A 4.477 | 0-14A 4.477 |
ILE 25A 3.006 | 0-25A 3.006 |
VAL 28A 3.095 | 0-28A 3.095 |
ALA 32A 3.257 | 0-32A 3.257 |
CYS 33A 4.936 | 0-33A 4.936 |
VAL 35A 2.804 | 0-35A 2.804 |
Receptor interface residue(s) 0 1.751 |
Ligand interface residue(s) | Receptor-ligand interface residue pair(s) |
---|---|
TRP 3A 2.949 | 0-3A 2.949 |
MET 4A 3.129 | 0-4A 3.129 |
VAL 6A 4.200 | 0-6A 4.200 |
CYS 7A 1.858 | 0-7A 1.858 |
PHE 10A 4.827 | 0-10A 4.827 |
ALA 32A 2.818 | 0-32A 2.818 |
CYS 33A 3.077 | 0-33A 3.077 |
MET 34A 4.823 | 0-34A 4.823 |
VAL 35A 2.845 | 0-35A 2.845 |
Receptor interface residue(s) 0 1.858 |
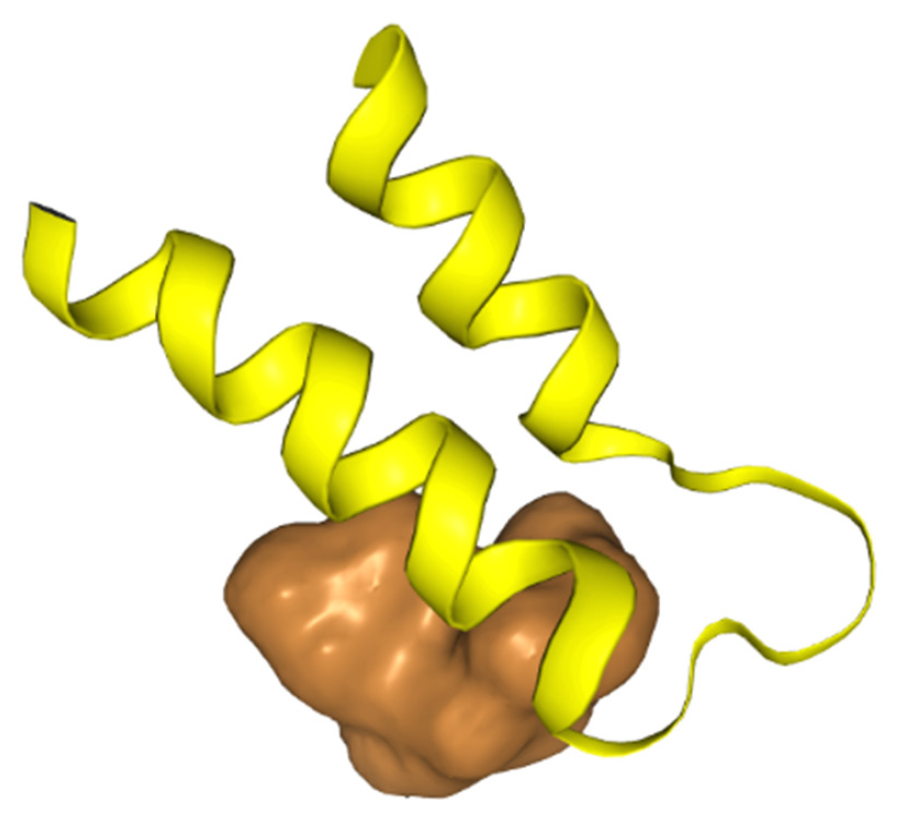
Figure 1:
Molecular docking studies of Gentamicin and Staphylococcus aureus C0673 illustrating drug binding affinities of Ligand and Receptor. Ligand (Gentamicin) indicated in brown colour surface style and Receptor (Staphylococcus aureus C0673) indicated in yellow colour.
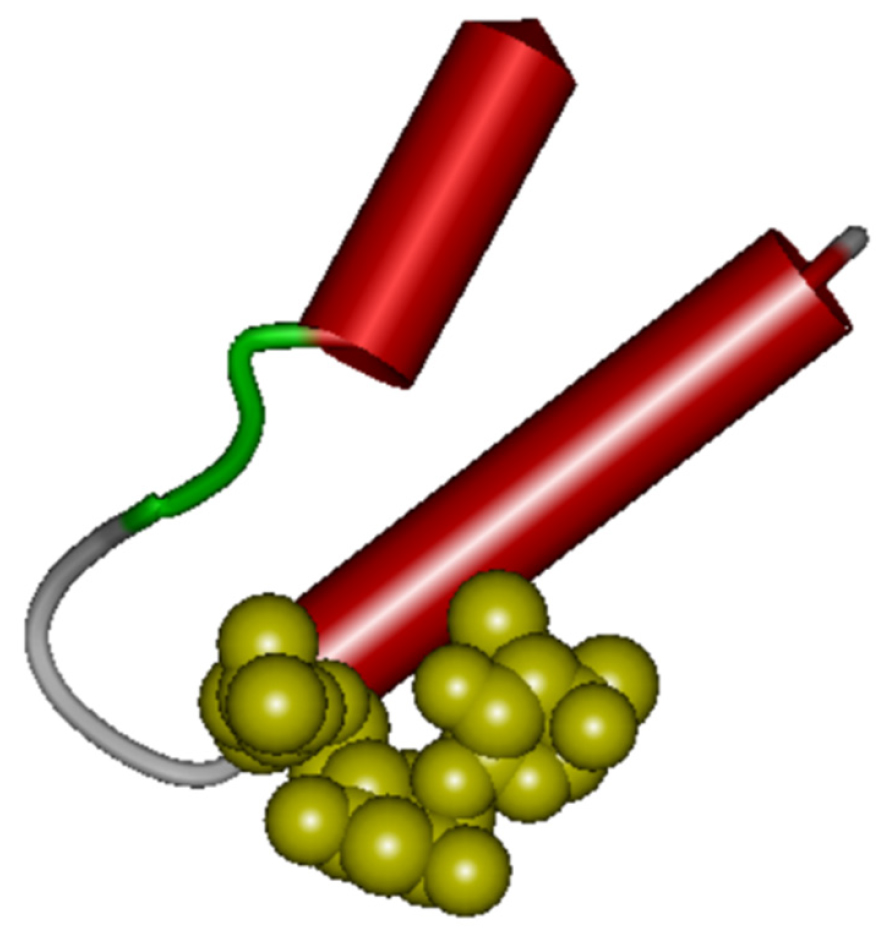
Figure 2:
Schematic model view of Gentamycin and Staphylococcus aureus C0673 viewed with visualization tool-Discovery studio.
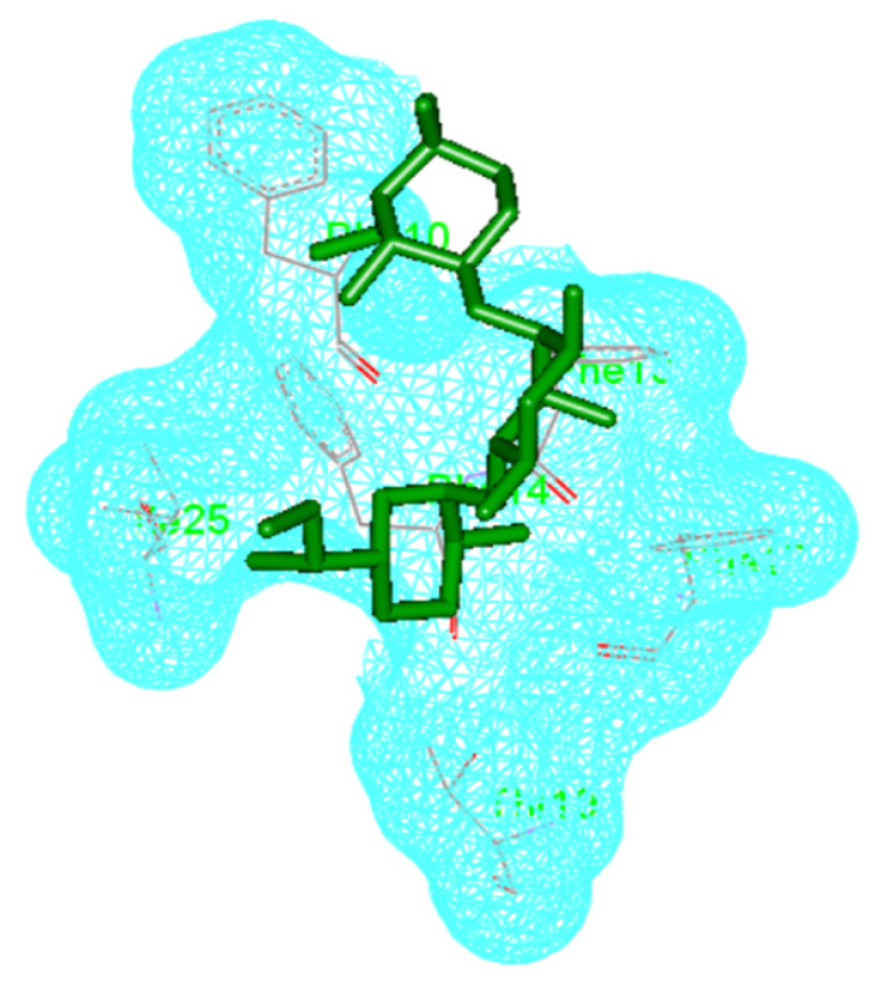
Figure 3:
3D structure of Gentamicin and Staphylococcus aureus C0673 illustrating surface around ligand viewed with visualization tool-Discovery studio.
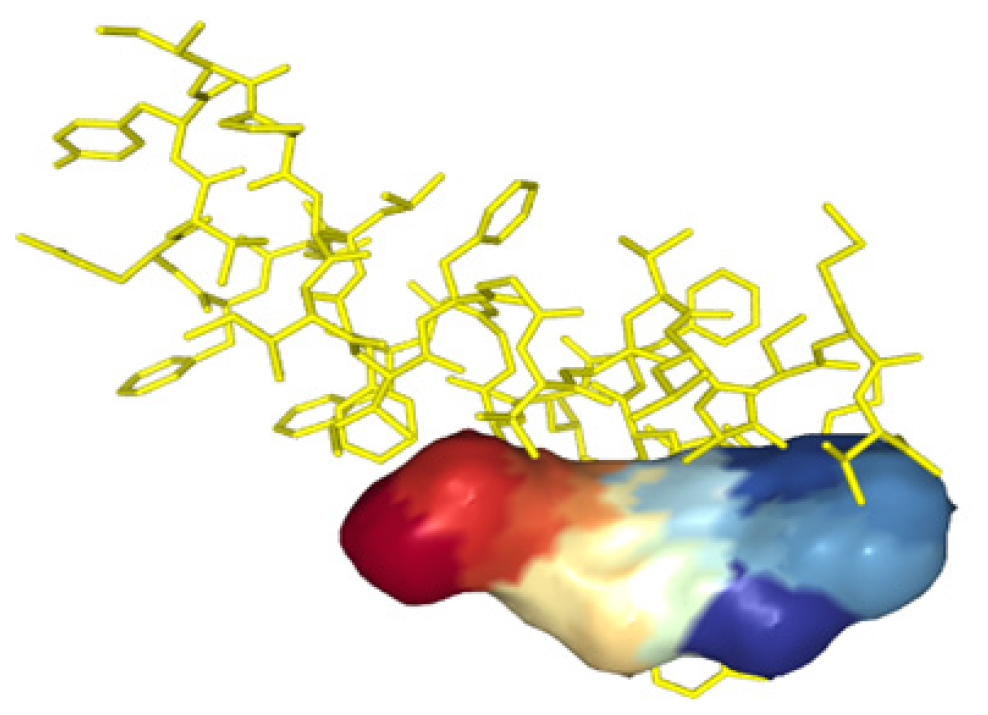
Figure 4:
Molecular docking studies of Linezolid and Staphylococcus aureus C0673 illustrating drug binding affinities of Ligand and Receptor. Ligand (Linezolid) indicated in rainbow coloured surface style and Receptor (Staphylococcus aureus C0673) indicated in yellow colour.
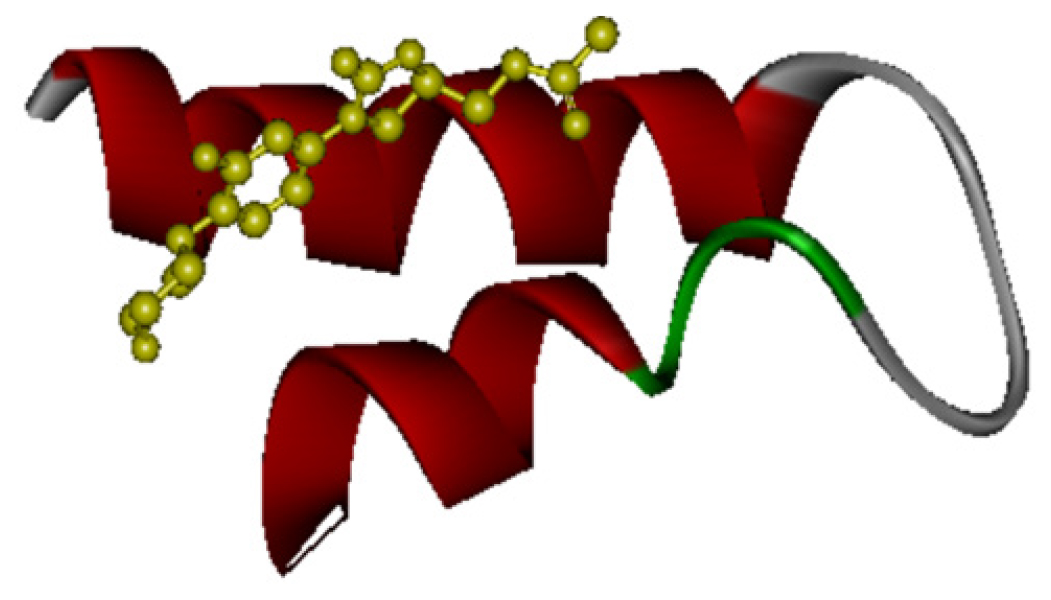
Figure 5:
Solid ribbon model view of Linezolid and Staphylococcus aureus C0673 viewed with visualization tool-Discovery studio.
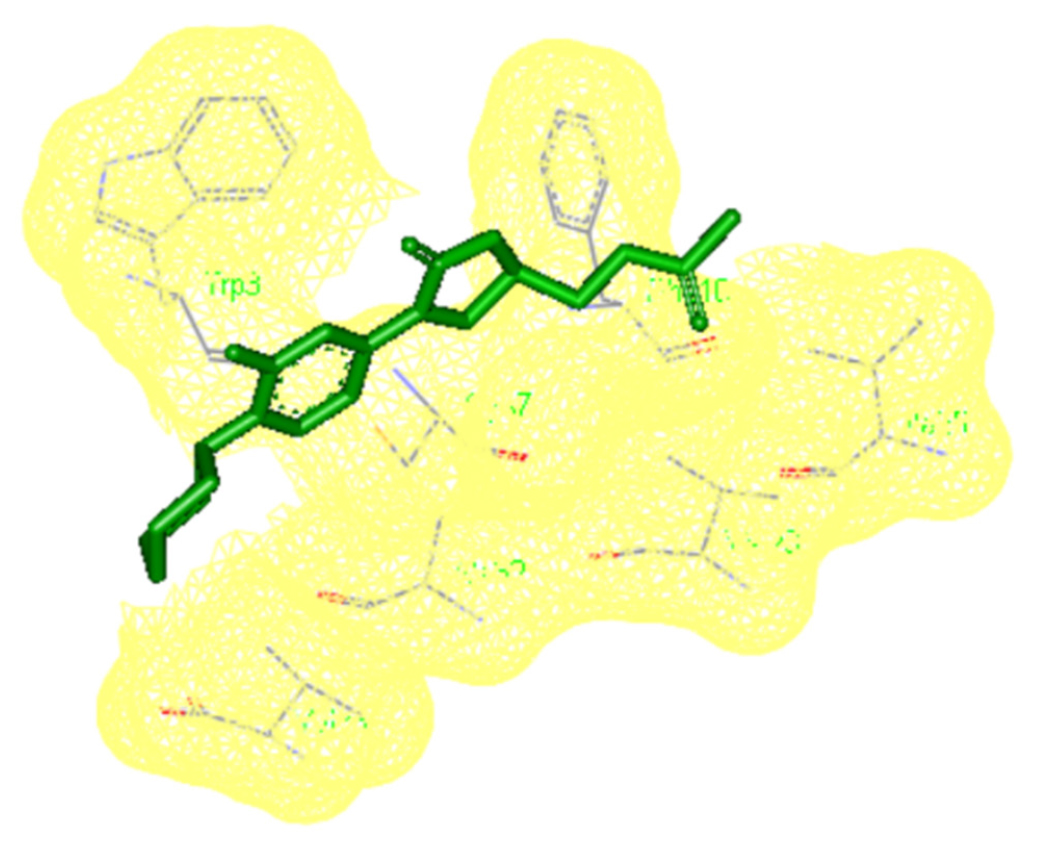
Figure 6:
3D structure of Linezolid and Staphylococcus aureus C0673 illustrating surface around ligand viewed with visualization tool-Discovery studio.
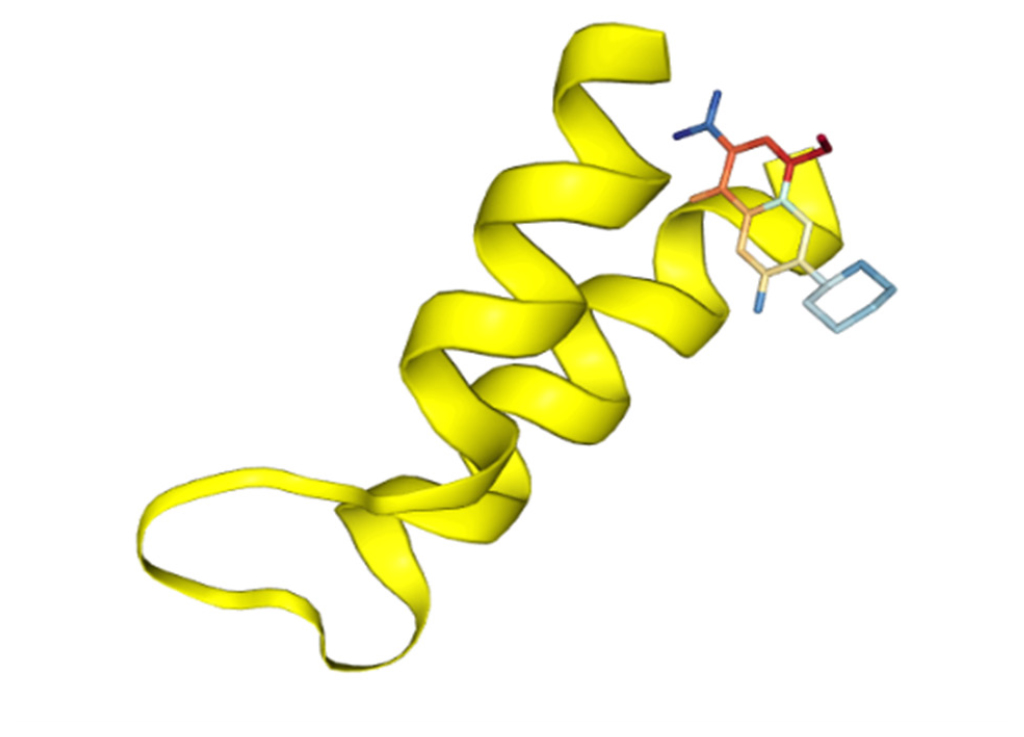
Figure 7:
Molecular docking studies of Norfloxacin and Staphylococcus aureus C0673 illustrating drug binding affinities of Ligand and Receptor. Ligand (Norfloxacin) indicated in rainbow coloured licorice style and Receptor (Staphylococcus aureus C0673) indicated in yellow colour.
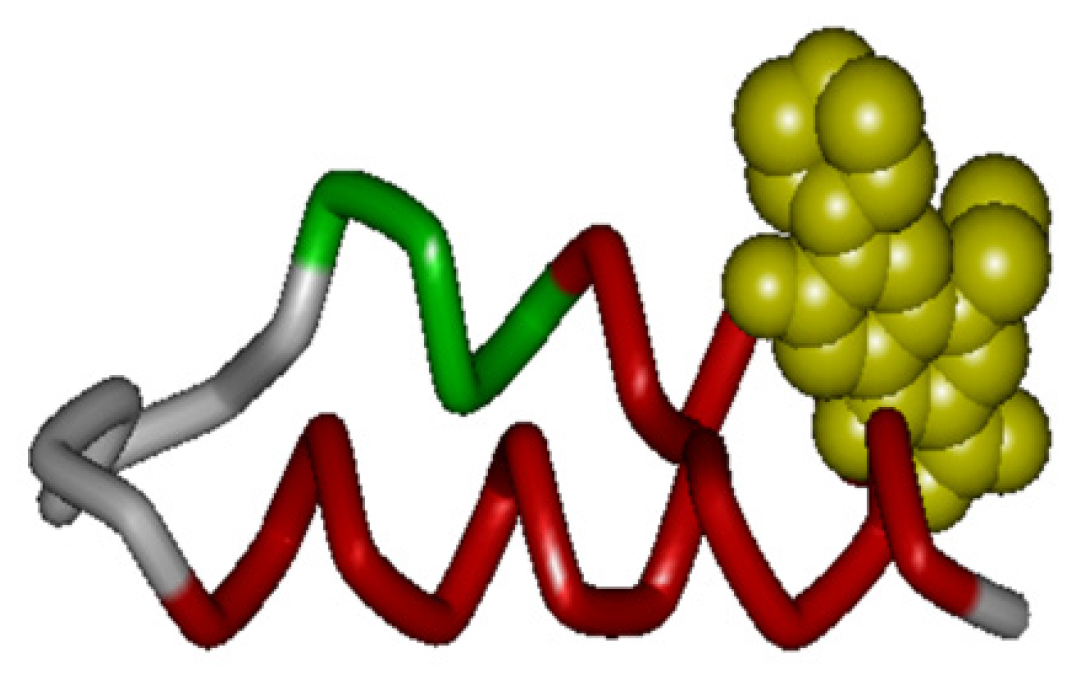
Figure 8:
Tube model view of Norfloxacin and Staphylococcus aureus C0673 viewed with visualization tool-Discovery studio.
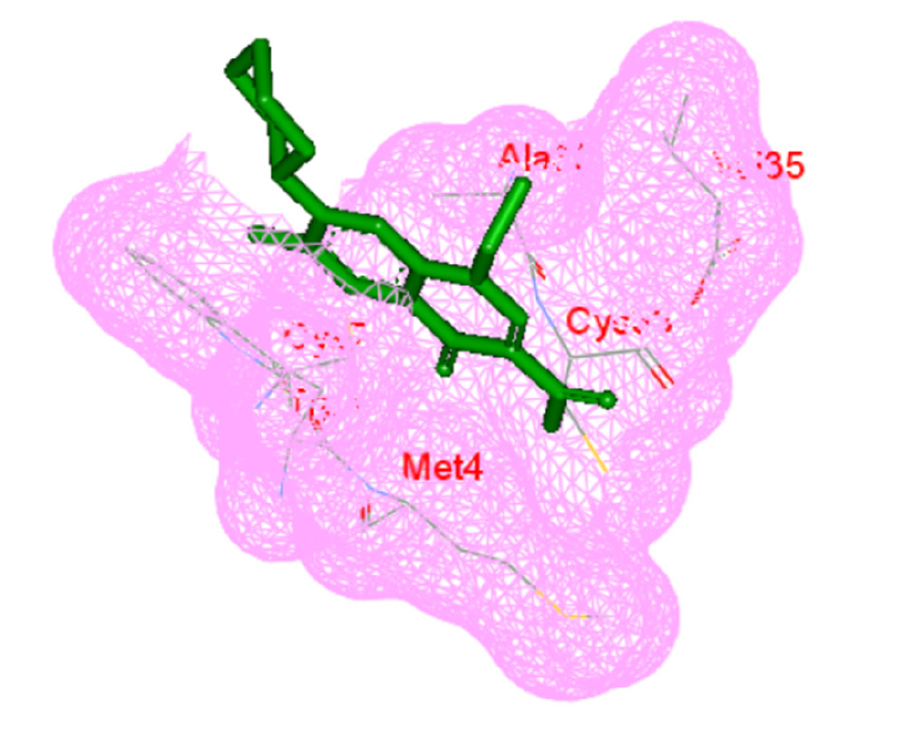
Figure 9:
3D structure of Norfloxacin and Staphylococcus aureus C0673 illustrating surface around ligand viewed with visualization tool-Discovery studio.
DISCUSSION
Gentamicin has bactericidal activity against aerobic gram-negative bacteria, making it a viable treatment choice for a variety of common infections.26 Gentamicin active transport across the gram-negative membrane is oxygen dependent. Aminoglycosides are inefficient against anaerobic bacteria because they require oxygen to develop.27 Linezolid inhibits bacterial growth by blocking the first stages of protein synthesis, a method of action unique to this class of medicines. The medication is authorized for the management of specific gram-positive infections, such as drug-resistant forms of pneumococcus, staphylococcus, and enterococcus.28 Norfloxacin is an antibacterial agent that works by binding to DNA gyrase, an enzyme that allows DNA strands to unwind and duplicate one double helix into two, hence stopping bacterial DNA reproduction. Notably, the medication has a 100-fold higher affinity for bacterial DNA gyrase than for human DNA gyrase.29
Computational approaches that ‘dock’ small molecules into the structures of macromolecular targets and ‘score’ their potential complementarity to binding sites are widely used in hit detection and lead optimization.13 The subject of in silico techniques in computer-aided drug design, which integrates the concepts of molecular biology, biochemistry, and biotechnology, is becoming more and more well-known as a cutting-edge, economical tool for developing drugs for a variety of acute illnesses.30
Recent discoveries by scientists have fundamentally altered our knowledge of the structure and function of cells. Most significantly, these discoveries have led to the theory that proteins may interact with one another and modify their functions in response to internal situations. Proteins have been shown to assemble into complexes by forming short- or long-term bonds with other proteins or ligands. A protein surface location known as a binding site is where the binding takes place. Protein interactions regulate how proteins operate and ensure their own self-regulation, which is essential for the proper operation of biological systems.31–33
Molecular Drug docking studies for Luteolin bioactive compound and Spike glycoprotein of SARS-CoV-2 showed that the intramolecular electrostatic force is based on the binding affinity between the ligand-receptor complexes indicating that the selected drug compound can act as an efficient inhibitor for the target corona virus protein.34 Protein function depends on binding cavities on protein surfaces since these are often the locations where proteins attach to other biological macromolecules like proteins and nucleic acids or tiny molecules like metabolites and drugs.35,36
CONCLUSION
Globally, the threat to public health posed by diverse microbial species resistance to various antibiotics is growing at an alarming rate.6 The overall results clearly elucidate that the results from docking the drugs (Gentamicin, Linezolid, Norfloxacin) potentially inhibits the hydrophobic regions of the novel predicted structure of the multi-drug resistant target protein of Staphylococcus aureus. The modelled structures and docking information have been submitted in ModelArchive. From this research investigation, we conclude that multidrug resistant antibiotics efficiently bind with Staphylococcus aureus C0673. The results obtained from this study play a major role in the field of current bacterial informatics studies.
Cite this article
Grace H, Leelavathi D, Zashumo KJ. Molecular Drug Docking of Multi Drug Resistant Antibiotics (Gentamicin, Linezolid and Norfloxacin) with Staphylococcus aureus C0673 by Implementing Computational Approach. Int. J. Pharm. Investigation. 2024;14(2):392-8.
ACKNOWLEDGEMENT
The authors acknowledge the help extended by Dr. Balaji Munivelan, Ph.D., CEO and Senior Bio-informatician, ABS Geno-informatics, Chennai, for his contribution towards in silico drug docking studies.
The authors acknowledge the help extended by Dr. Balaji Munivelan, Ph.D., CEO and Senior Bio-informatician, ABS Geno-informatics, Chennai, for his contribution towards in silico drug docking studies.
ABBREVIATIONS
MRSA | Methicillin-resistant Staphylococcus aureus |
---|---|
SMILES | Simplified molecular input line entry system |
PDB | Protein Data Bank |
MDR | Multi-Drug Resistance |
References
- Lowy FD. Staphylococcus aureus infections. N Engl J Med. 1998;339(8):520-32. [PubMed] | [CrossRef] | [Google Scholar]
- Gnanamani A, Hariharan P, Paul-Satyaseela M. Staphylococcus aureus: overview of Bacteriology, Clinical Diseases, Epidemiology, antibiotic Resistance and Therapeutic Approach [internet]. Frontiers in Staphylococcus aureus; 2017. InTech. [CrossRef] | [Google Scholar]
- Löffler B, Tuchscherr L. Staphylococcus aureus toxins: promoter or handicap during infection?. Toxins. 2021;13(4):287 [PubMed] | [CrossRef] | [Google Scholar]
- DeLeo FR, Chambers HF. Reemergence of antibiotic-resistant Staphylococcus aureus in the genomics era. J Clin Invest. 2009;119(9):2464-74. [PubMed] | [CrossRef] | [Google Scholar]
- Mukherjee R, Priyadarshini A, Pati Pandey R, Samuel Raj V. Antimicrobial resistance in Staphylococcus aureus insights into drug resistance in Staphylococcus aureus. IntechOpen. 2021 [CrossRef] | [Google Scholar]
- Tanwar J, Das S, Fatima Z, Hameed S. Multidrug resistance: an emerging crisis. Interdiscip Perspect Infect Dis. 2014;2014:541340 [PubMed] | [CrossRef] | [Google Scholar]
- National Center for Biotechnology Information. [retrieved Jul 1, 2023];PubChem compound summary for CID 3467, gentamicin. 2023 [PubMed] | [CrossRef] | [Google Scholar]
- National Center for Biotechnology Information. [retrieved Jul 1, 2023];PubChem compound summary for CID 441401, linezolid. 2023 [PubMed] | [CrossRef] | [Google Scholar]
- National Center for Biotechnology Information. [retrieved Aug 26, 2023];PubChem compound summary for CID 4539, norfloxacin. 2023 [PubMed] | [CrossRef] | [Google Scholar]
- Tong SY, Davis JS, Eichenberger E, Holland TL, Fowler VG. Staphylococcus aureus infections: epidemiology, pathophysiology, clinical manifestations, and management. Clin Microbiol Rev. 2015;28(3):603-61. [PubMed] | [CrossRef] | [Google Scholar]
- Taylor TA, Unakal CG. [Updated 2022: 2023];Staphylococcus aureus infection. StatPearls [Internet]. [PubMed] | [CrossRef] | [Google Scholar]
- Pinzi L, Rastelli G. Molecular docking: shifting paradigms in drug discovery. Int J Mol Sci. 2019;20(18):4331 [PubMed] | [CrossRef] | [Google Scholar]
- Kitchen DB, Decornez H, Furr JR, Bajorath J. Docking and scoring in virtual screening for drug discovery: methods and applications. Nat Rev Drug Discov. 2004;3(11):935-49. [PubMed] | [CrossRef] | [Google Scholar]
- Vestergaard M, Frees D, Ingmer H. Antibiotic resistance and the MRSA problem. Microbiol Spectr. 2019;7(2) [PubMed] | [CrossRef] | [Google Scholar]
- Kersey PJ, Staines DM, Lawson D, Kulesha E, Derwent P, Humphrey JC, et al. Ensembl Genomes: an integrative resource for genome-scale data from non-vertebrate species. Nucleic Acids Res. 2012;40(Database issue):D91-7. [PubMed] | [CrossRef] | [Google Scholar]
- Kim S, Chen J, Cheng T, Gindulyte A, He J, He S, et al. PubChem 2023 update. Nucleic Acids Res. 2023;51(D1):D1373-80. [PubMed] | [CrossRef] | [Google Scholar]
- Meng XY, Zhang HX, Mezei M, Cui M. Molecular docking: a powerful approach for structure-based drug discovery. Curr Comput Aided Drug Des. 2011;7(2):146-57. [PubMed] | [CrossRef] | [Google Scholar]
- Yan Y, Tao H, He J, Huang SY. The HDOCK server for integrated protein-protein docking. Nat Protoc. 2020;15(5):1829-52. [PubMed] | [CrossRef] | [Google Scholar]
- Malmir N, Fard NA, Mgwatyu Y, Mekuto L. Cyanide hydratase modification using computational design and docking analysis for improved binding affinity in cyanide detoxification. Molecules. 2021;26(6):1799 [PubMed] | [CrossRef] | [Google Scholar]
- Remmert M, Biegert A, Hauser A, Söding J. HHblits: lightning-fast iterative protein sequence searching by HMM-HMM alignment. Nat Methods. 2011;9(2):173-5. [PubMed] | [CrossRef] | [Google Scholar]
- Pearson WR, Lipman DJ. Improved tools for biological sequence comparison. Proc Natl Acad Sci U S A. 1988;85(8):2444-8. [PubMed] | [CrossRef] | [Google Scholar]
- Sievers F, Wilm A, Dineen D, Gibson TJ, Karplus K, Li W, et al. Fast, scalable generation of high-quality protein multiple sequence alignments using Clustal Omega. Mol Syst Biol. 2011;7(1):539 [PubMed] | [CrossRef] | [Google Scholar]
- Larkin MA, Blackshields G, Brown NP, Chenna R, McGettigan PA, McWilliam H, et al. Clustal W and Clustal X version 2.0. Bioinformatics. 2007;23(21):2947-8. [PubMed] | [CrossRef] | [Google Scholar]
- Martí-Renom MA, Stuart AC, Fiser A, Sánchez R, Melo F, Sali A, et al. Comparative protein structure modeling of genes and genomes. Annu Rev Biophys Biomol Struct. 2000;29:291-325. [PubMed] | [CrossRef] | [Google Scholar]
- Berman HM, Westbrook J, Feng Z, Gilliland G, Bhat TN, Weissig H, et al. The Protein Data Bank. Nucleic Acids Res. 2000;28(1):235-42. [PubMed] | [CrossRef] | [Google Scholar]
- Phillips I, Eykyn S, King BA, Jenkins C, Warren CA, Shannon KP, et al. The in vitro antibacterial activity of nine Aminoglycosides and spectinomycin on clinical isolates of common Gram-negative bacteria. J Antimicrob Chemother. 1977;3(5):403-10. [PubMed] | [CrossRef] | [Google Scholar]
- Chaves BJ, Tadi P. Gentamicin. StatPearls [Internet]. 2023 [PubMed] | [Google Scholar]
- Ament PW, Jamshed N, Horne JP. Linezolid: its role in the treatment of gram-positive, drug-resistant bacterial infections. Am Fam Phys. 2002;65(4):663-70. [PubMed] | [Google Scholar]
- Goldstein EJ. Norfloxacin, a fluoroquinolone antibacterial agent. Classification, mechanism of action, and in vitro activity. Am J Med. 1987;82(6B):3-17. [PubMed] | [CrossRef] | [Google Scholar]
- Browne RB, Goswami N, Borah P, Roy JD. Computational approaches for evaluation of isobavachin as potential inhibitor against t877a and w741l mutations in prostate cancer. J Biomol Struct Dyn. 2023;41(6):2398-418. [PubMed] | [CrossRef] | [Google Scholar]
- Phizicky EM, Fields S. Protein-protein interactions: methods for detection and analysis. Microbiol Rev. 1995;59(1):94-123. [PubMed] | [CrossRef] | [Google Scholar]
- Jones S, Thornton JM. Principles of protein-protein interactions. Proc Natl Acad Sci U S A. 1996;93(1):13-20. [PubMed] | [CrossRef] | [Google Scholar]
- Konc J, Janežič D. Protein binding sites for drug design. Biophys Rev. 2022;14(6):1413-21. [PubMed] | [CrossRef] | [Google Scholar]
- Grace HVM, Zashumo KJ, Leelavathi D. Plant derived compound-luteolin promising role against SARS-CoV-2 protein. Research Journal of Agricultural Sciences [an international journal]. 2022;13(05):1449-53. [PubMed] | [CrossRef] | [Google Scholar]
- Morris GM, Lim-Wilby M. Molecular docking. Methods Mol Biol. 2008;443:365-82. [PubMed] | [CrossRef] | [Google Scholar]
- Zashumo KJ, Leelavathi D, Grace H. AntiObesity Property of Indian Tulsi Plant (Ocimum sanctum) using in silico Docking Techniques. Biological Forum-An International Journal. 2023;15(2):09-14. [PubMed] | [CrossRef] | [Google Scholar]