ABSTRACT
Background
Many parts of the brain experience excruciating pain as a result of Alzheimer’s disease, a form of dementia. Cognitive processes and neuropsychiatric regulation are disrupted by the numerous neuropathologies that exist. The everyday activities, social and professional lives, and overall health of the individual are all substantially impacted by this ongoing deterioration. In this, we comprehend the advantages and practicality of phytoconstituents on the molecular targets of Alzheimer’s disease.
Materials and Methods
In order to write this review study, we used internet databases of publications including PubMed, Science Direct, Google Scholar, and Scopus.
Results
The use of pharmaceuticals has been a cornerstone in treating AD for a very long time. However, this strategy has numerous disadvantages. You must alter your treatment strategy now more than ever. Due to their advantages, which include decreased risk and negative effects from use, natural treatments made from plants are becoming increasingly widespread. The current situation study focuses on the anti-AD capabilities of phytoconstituents, sheds light on those currently engaged in clinical trials, and gathers data on their precise mechanisms of action in opposition to various neuropathologies associated with AD.
Conclusion
The phytoconstituents will undoubtedly contribute to the development of novel, safer AD treatments that are superior to the currently existing pharmaceutical options, whether taken alone or in combination.
INTRODUCTION
According to the observations of Auguste Deter, a 51 years old lady, German psychiatrist, and neuropathologist, Dr. Alois Alzheimer classified AD as a kind of dementia in Nov 1901. Alzheimer categorized the detected qualities as having “presenile dementia,” using the same fundamental ideas for empathetic AD.1
Clinical signs of AD are characterized by advanced memory harm, disorientation, language difficulties, cognitive deficiencies for example, deterioration of verdict as well as choices, and deficits in thinking. Other neuropsychiatric symptoms associated with AD include hallucinations, delusions, anxiety, agitation, depression, and apathy. This continuous deterioration in cognitive functioning significantly impairs people’s ability to perform basic everyday tasks, negatively affecting their capability to lead fulfilling personal and professional lives.2
Factors affecting the neuropathologies of Alzheimer’s disease
The neuropathologies linked to AD are a result of the damaging deviations in the anatomical brain organization. Cerebral nerves linked with cognitive performance are wiped out as a result. It also contributes to impaired synaptic activation in brain circuits. There is a comprehensive description of the neuropathologies connected to AD, Amyloid plaques, cerebral amyloid angiopathy, neurofibrillary tangles, glial responses, and neuronal and synaptic loss are all symptoms of Alzheimer’s disease.3 Tangled deposits and plaques include examples of diseased disturbances that have been linked to the buildup of improperly produced proteins both inside and outside neurons. Amyloid β (Aβ) and phosphorylated tau protein are the two primary proteins that cause these degenerative lesions. The phosphorylated tau protein is found in venation cells, whereas Aβ is found in the extracellular matrix of nerve cells. The development of the neurite depends on Aβ, which also enhances memory and shields the brain against harm, aging, and long-term potentiation.4 Anomalies are frequently caused by mutations in the genes that create the APP, PS1, and PS2, the operational units of the penultimate endo-protease in the sequence of its biosynthesis.5 Two main mechanisms by which APP is normally processed are the non-amyloidogenic and amyloidogenic pathways; one of these produces atypical Aβ, other one creates non-pathogenic, valuable amyloid products, like (sAPPα).6 The proteolytic enzymes α, β, and γ-secretases are principally responsible for these two methods’ digestion of APP.7 When the mutant tau protein accumulates intracellularly, (NFTs) and other enclosure builds are produced.8 Tau protein promotes tubulin assembly, which aids in the production of microtubules. The cytoskeletal elements known as microtubules are crucial for maintaining the shape and structure of the neuron.9 Tau’s ability to attach to tubulin is governed by its phosphorylation. Due to improper tau protein folding, AD develops when tau is unable to connect to microtubules.10 As a result, fibrillary formations of tau begin to accumulate inside the neurons. NFTs have the potential to cause neuronal death by obstructing the transmission of the substances required for their survival and regular operation.11 Another well-known factor in AD is oxidative stress. More oxidative stress markers and persistent glycation products are present in NFT and Aβ deposits. The pathogenesis of AD is made worse by elevated levels of substances that are chemically reacting in the body.12 The study also suggests that a rise in Aβ-aggregation causes an increase in ROS, which causes mitochondrial damage-induced oxidative stress to follow.11 References favor oxidative injury before Aβ-pathology.13 Increased oxidative reactive substances in the brain exacerbate AD pathogenesis.14 These cellular alterations could be visualized In the dual scattering of their various biological aggregates, they used the ToF-SIMS visualization technology, giving critical new data about the cellular microenvironment as AD develops.15 The down-regulation of anti-apoptotic proteins and the active activation of particular apoptotic factors result in the usual neuronal loss associated with AD. Aberrant ABB processing, which results in abnormal calcium ions, is one of the factors that contribute to neuronal disorders and apoptosis.16 Acetylcholine activity abnormalities have been observed in the early stages of AD, and these impairments have been linked to memory loss and negatively impact both cognitive and non-cognitive functioning.17 IL-1β, IL-6, and TNF-α levels are elevated, pro-inflammatory genes are activated, and neuroinflammation results.18 Moreover, it has been observed that AD patients have glial activation, increased nuclear factor kappa B (NF-ĸB) levels, and (TLR2 and TLR4).19
Brain regions impacted by AD
Several brain regions are impacted by AD, and one region may be significantly impaired while the surrounding region is unaffected.20 The brain problems caused by AD have a distinctive pattern, which sets it apart after further neurodegenerative illnesses.21 Explicit intellect regions affected in AD include the Hippocampus, Parahippocampus, Amygdala, Cingulate cortex, Medial Septal Nucleus, Subiculum, Nucleus Basalis Meynert, Locus Coeruleus, Nucleus Accumbens, Entorhinal cortex, Perirhinal cortex, Orbitofrontal Cortex, Prefrontal cortex, and Raphe Nuclei.22 In 1991 and 1997, respectively, Heiko and Eva Braak provided a stage-by-stage explanation of the development of NFTs and senile plaques.23 Although offering a different senile plaque progression staging in 2002.24
Drugs used to treat Alzheimer’s disease at the moment
AChE, oxidative stress, inflammatory pathways, hormone therapy, and excess blood lipids are all aspects to think about, and other conditions are all targets for medication.25 Some of the current pharmacological treatments for AD. Cholinesterase inhibitors like galantamine, rivastigmine, and donepezil are used as the preliminary stripe of resistance in contrast to AD. Memantine is also utilized for severe or moderate AD.26 Sertraline, fluvoxamine, fluoxetine, paroxetine, citalopram, mirtazapine, venlafaxine, risperidone, ziprasidone, duloxetine and quetiapine, olanzapine, aripiprazole are just a few of the antidepressants that are used to treat depression in AD patients.27 Many use benzodiazepines to lessen the agitation and anxiety caused by AD. There are several more drugs available to address the many symptoms of AD. Research into new AD treatments has been prompted by the existing ineffectiveness of available medications.28 Recent studies have mostly therapy centered on A, ABB, and tau passing through different stages of a clinical study. These pharmaceutical treatments come with a variety of negative effects.29 The development of therapeutic drugs with fewer side effects should therefore receive more attention.30 The best alternative is composed of plant-based products, which also have fewer disadvantages and can be very helpful.31 Numerous phytochemicals were studied and correlated to the characteristics of AD.32
Phytochemicals for the treatment of ALzheimer’s Disease
Aβ targeted phytoconstituents
To target the Aβ peptides, the phytoconstituents addressed in this section frequently modify their APP and secretase activities. See in Table 1 for the list of the phytoconstituents.
Tau antagonistic phytoconstituents
Various antagonistic phytoconstituents can be current AD treatments. The following Table 2 lists a few phytoconstituents that target tau.
Plant | Component/ extract | Activity |
---|---|---|
Curcuma longa | Curcumin | Predicaments to tiny Aβ species and successfully prevents Accretion, fibril production, and self-assembly of Aβ.33 Diminishes the amount of the senile plaques and undoes the structural alterations in the atrophied dendrites.34 Weakens the preformed fibrillar Aβ structure.35 Diminishes Aβ via lowering the amounts of the GSK3 protein and mRNA, which lowers apoptosis and malfunction in the mitochondria.36 Reduces synaptic toxicity and mitochondrial dysfunction in AD while preserving cell viability, mitochondrial dynamics, and biogenesis.37 |
Isoxazoles and Pyrazoles. (Curcumin derivatives) | Blocks APP metabolism. Rises macrophage cellular absorption of Aβ.38 | |
Ginkgo biloba | Extract | Enhances the soluble form of APP (sAPPα) by shifting the manner of APP metabolism more in the direction of the Aβ-secretase pathway.39 Reduces circulating free cholesterol levels, which improves APP processing and amyloidogenesis in turn, lowering the stages of Aβ.40 |
Panax ginseng | Ginsenoside Rg1 | Prevents the production of Aβ through the GSK3β beta/tau signaling pathway.41 |
Ginsenoside Rb1, Rb2, CK, F1, Rh1, Rh2 and Re. | The β-Secretase activity must be suppressed.41 | |
Ginsenoside Rh2 | Inhibits APP endocytosis by lowering the levels of lipid rafts and cholesterol.42 | |
Gintonin | Decreases Aβ production and lessens the neurotoxicity caused by Aβ.35 Changes the signaling pathways of the lysophosphatidic acid (LPA) receptor, which activates the non-amyloidogenic Aβ pathways.41 | |
Bacopa monniera | Bacoside A | The developed aggregates of Aβ are effectively dissolved.43 |
Extract | The extract’s impact on oligomeric Aβ led to a considerable reduction in the levels of Aβ40 and Aβ42.44 | |
Centella siatica | Asiaticoside | Inhibits (PLA2) enzymes, particularly cPLA2, and sPLA2, which are responsible for the neurotoxicity caused by Aβ.45 |
Aqueous extract | enhances cognitive performance. Decreases the Aβ-associated aberrant behavior in mouse models. decreases the MC65 cells’ production of Aβ aggregates.46 | |
Rosmarinus officinalis | Carnosic acid | Aβ-42 secretion is decreased.47 Inhibits the non-amyloidogenic pathway’s processing of APP.47 Decreases AB-40 and sAPPβ, while sAPPα is increased (a product of the non-amyloidogenic pathway).48 increases the activity of α-site APP-cleavage, which in turn causes an inhibition of the formation of Aβ-42 and Aβ-43, by enhancing the mRNA expression of the α-secretase TACE.48 |
Withania somnifera | Withanamides A and C. | Inhibits fibril formation by specifically binding to the Aβ active motif (25-35).49 LRP levels in plasma are increased.50 increases the liver’s expression of the Aβ-degrading enzymes neprilysin (NEP) and LRP, which raises plasma levels of Aβ-42/40 and lowers brain levels of Aβ-monomers. (LRP is a key receptor on the cell surface that plays a role in removing Aβ from the fluid surrounding brain cells. Furthermore, it facilitates the endocytosis of APP, influencing the synthesis of Aβ).50 |
Extract | decreases the amounts of the cluster in chaperones and RAGE receptors on receptors linked with Aβ aggregation neuropathologies.51 | |
Withanolides and withanoside | Removes Aβ peptides out from the brain and encourages its accumulation in plasma to degrade it.52 | |
Magnolia officinalis | Extract | Cleaves Aβ from APP, reducing the activity of β-secretase. Reduces the expression of APP, its product, C99, and the enzyme that breaks down APP, β site APP cleaving enzyme 1 (BACE1). Decreases β-secretase activity to prevent memory loss.53 |
List of bioactive constituents used against Aβ plaques.
Plant | Component/ extract | Activity |
---|---|---|
Curcuma longa | Curcumin | Interacting with the 4R0N tau inhibits the aggregation of pure tau by liquifying the polymers, and speeds up the disaggregation of tau.54 |
Panax ginseng | Ginsenoside | By manipulation of GSK-3β, Tau K18 fragment dissociation, and aggregation were considerably reduced.55 |
Ginsenoside Rg1 and Rd | Modulates the GSK-3β/Tau signaling pathway to reduce the toxicity of tau.55 | |
Ginsenoside Rd | Enhance the activity of PKB/Akt, a crucial kinase in charge of inhibiting GSK-3β activity and raising PP-2 A.55 | |
Bacopa monniera | Extract | It Lessens tau’s hyperphosphorylation and the toxicity that tau causes.43 |
Rosmarinus officinalis | Carnosic Acid, Carnosol, Epiisorosmanol, Rosmanol, and Rosmarinic acid. | Action that prevents tau from accumulating.56 Reduces tau aggregation by interacting via salt bridges with 306 VQIVYK 311 fibers.56 |
Uncaria rhynchophylla | Rhynchophylline and isorhynchophylline. | Minimizes tau hyperphosphorylation and high calcium ions.57 |
Vitis vinifera | Meganatural-Az | Reduces the production and synaptic plasticity consistency that isn’t folded correctly.58 |
Grapeseed (Vitis) | Proanthocyanidins-enriched grape seed Extract. | decreases aggregation of tau proteins by interacting non-covalently with their polyphenols.59 |
Salvia miltiorrhiza | Tanshinone IIA | via altering the calcium and p35/cdk5 pathways, protects against tau hyperphosphorylation and numerous other pathologies caused by Aβ. Reduces Cdk5 activation, which reduces tau hyperphosphorylation in primary cortical neurons.60 |
Salvianolic acid B | Suppresses tau hyperphosphorylation and inhibits GSK-3β activity in vitro.61 | |
Ganoderma lucidum | Triterpenoids (GLTs) | Lowers the quantity of NFT in the cytoplasm clearly (of AD mice).62 |
Allium sativum | Aged Garlic extract | Decreases the activity of GSK-3β to lower tau phosphorylation.63 |
Camellia sinensis | (-)-Epigallocatechin-3-gallate | Phosphorylated tau epitopes are removed by controlling the amplification of Huntingtin, Aβ, and α-synuclein in primary neurons.64 |
Azadirachta indica | Limonoids | Prevents tau aggregation that is overwhelming, producing pieces of tau that are thin, short, and fragile.65 |
Nimbin and Salannin | Decreases the cytotoxicity caused by tau.65 | |
Rhodiola rosea | Salidroside. | Reduces the phosphorylation of tau by modifying the GSK-3β pathway.66 |
Cornus officinalis | Cornel iridoid glycoside (CIG) | Lowers the risk of tau hyperphosphorylation. Increases PI3K/AKT pathway activity and reduces GSK-3β-mediated tau hyp erphosphorylation. Increases PP2A by preventing PP2Ac’s demethylation at Leu309, which is thought to be a contributing reason to the preeminent equal of tau hyperphosphorylation. This increases PP2A, which in chance decreases tau hyperphosphorylation by maintaining the balance between GSK-3β and PP2A.67 |
Myrica cerifera | Myricanol | Encourages tau autophagic clearance.68 |
Cinnamomum zeylanicum | Cinnamaldehyde | Avoids tau filament production and the process of nucleation.69 Stop the oxidation caused by H2O2 from forming high-molecular-weight tau species.69 |
List of plant ingredients that prevent tau aggregation.
Plant | Component/ extract | Activity |
---|---|---|
Ginkgo biloba | Extract | The brains of aged animals have an activity that normalizes acetylcholine receptors.70 |
Bacopa monniera | Extract | Lowers AChE action.35 |
Centella asiatica | Asiaticoside and Madecassoside | AChE blockers.71 |
Melissa officinalis | Phenolic compounds | Muscarinic and nicotinic acetylcholine receptors are bound, and their activity is inhibited.72 |
Rosmarinic acid | Increases choline availability and binds to and inhibits AchE.73 | |
Lavandula angustifolia | Lavender | Increases choline availability and binds to and inhibits AchE.73 |
Phytoconstituents with anti-AChE action.
AChE targeted phytoconstituents
To increase acetylcholine levels in AD brains, the majority of phytotherapy strategies focus on lowering increased AChE activity. The phytoconstituents that work to lessen AChE activity include those that are listed in Table 3.
Phyto-constituents in the clinical trials
A new strategy for treating AD involves using phytoconstituents with anti-AD effects. To assess their efficacy as a treatment and latent negative effects, human trials consume stood conducted in recent years.74 The first natural phytoproduct to be examined in a clinical trial for this was nicotine, which debuted in 1992.75 Galantamine, an alkaloid derived then plants in the Amaryllis family, was recently created.76 Galantamine is still being tested in clinical trials today. Nonetheless, it has FDA approval and is currently used to treat moderate to serious complaints of AD.77 Similar to Physostigmine, the Calabar bean is a naturally occurring source of para-sympathomimetic alkaloids. The FDA developed and approved its semi-synthetic derivative, rivastigmine, which is specified for the behavior of AD dementia.78 Figure 1 depicts numerous phytochemicals that are being evaluated versus AD and are currently in phase two clinical studies for the establishment of AD therapies.
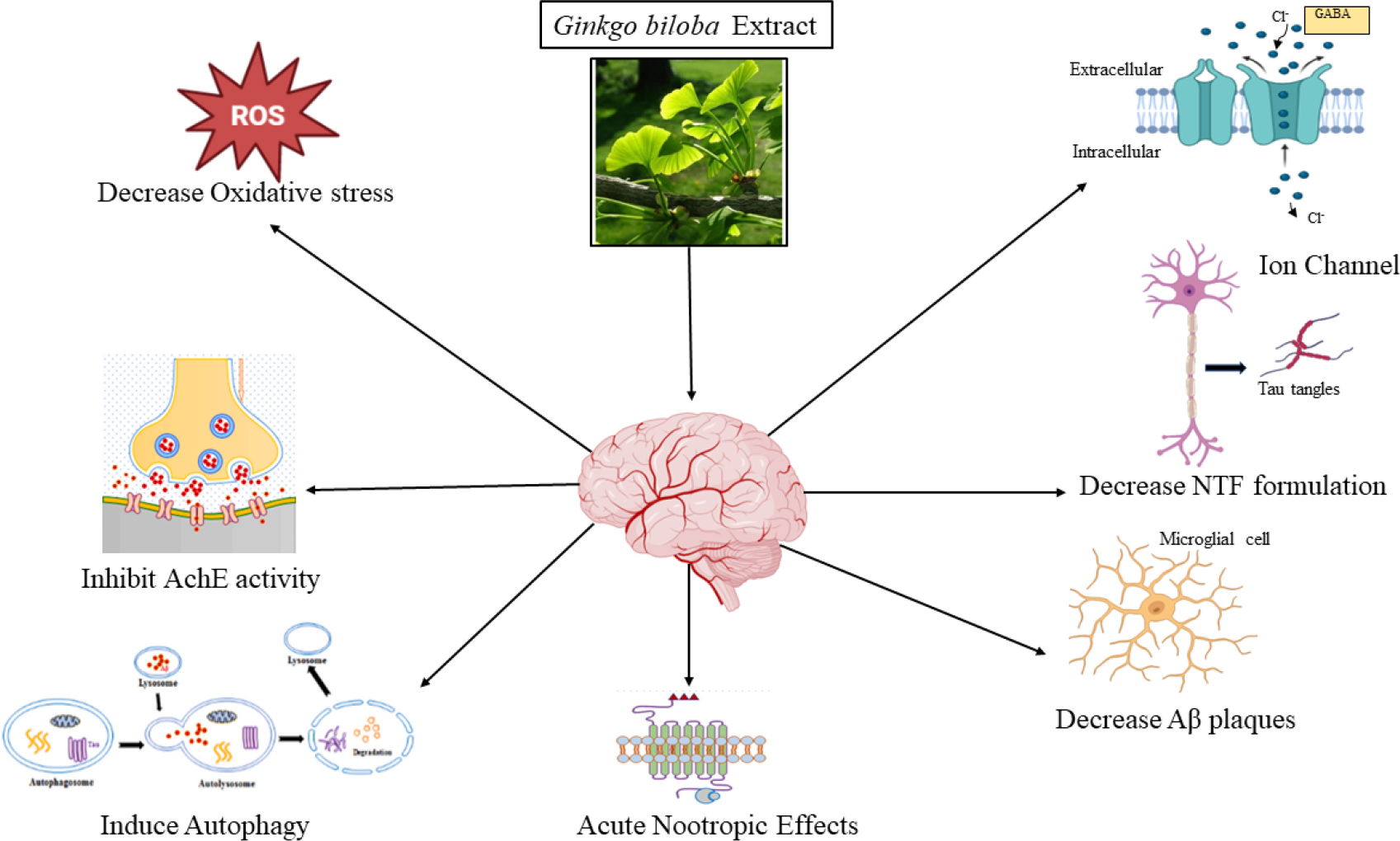
Figure 1:
Effect of Ginkgo biloba phytoconstituents on neuroprotective characteristics.
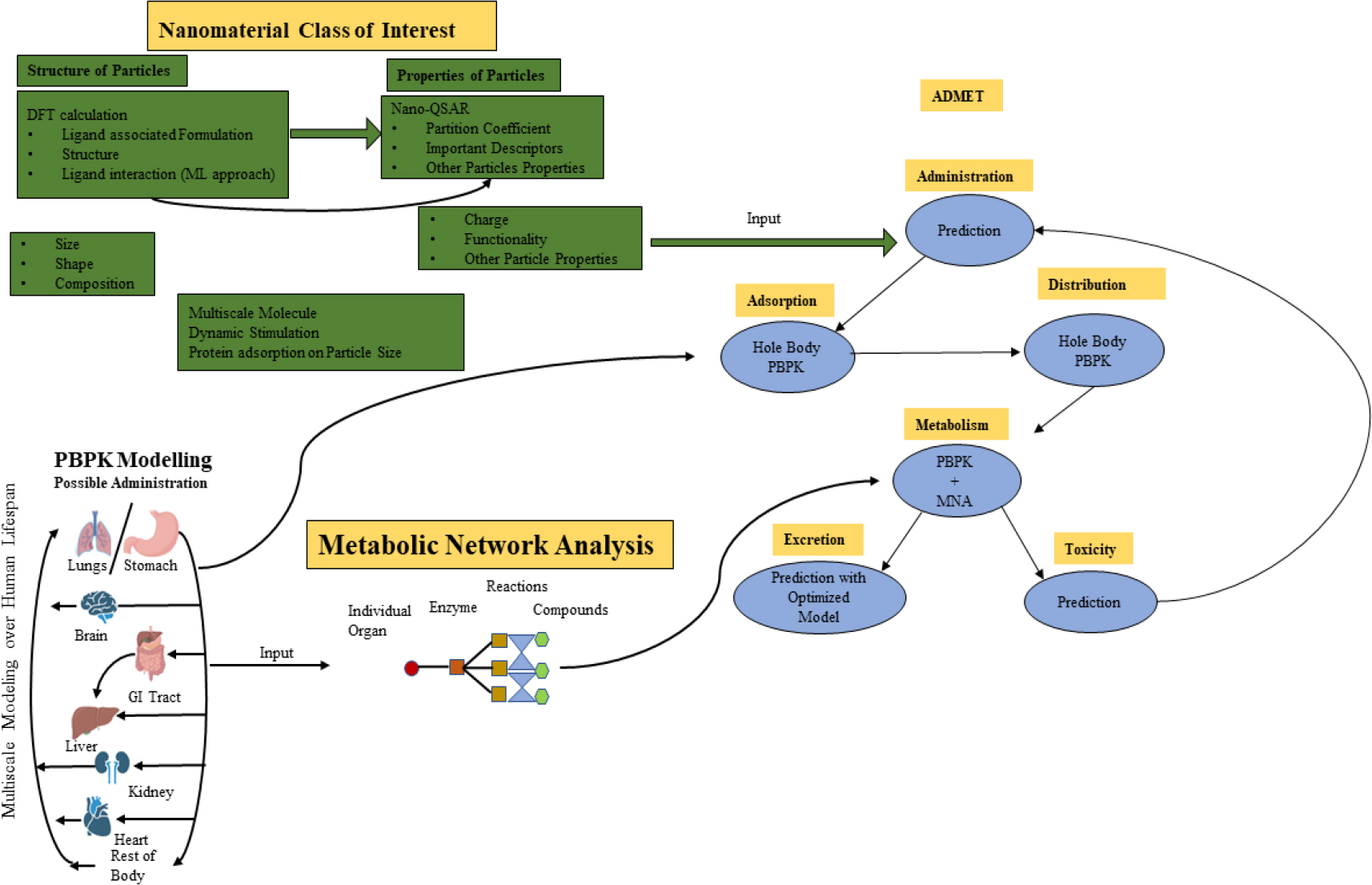
Figure 2:
A futuristic hybrid multimodal system for relating in vitro models.52
Challenges in phytoconstituents-Based Pharmacotherapy
Currently, since it proves various facts, such as the inefficiency of traditional medications and the offensive routine of synthetic pharmaceuticals, certifying its negative belongings, alternative therapies using plant-derived natural chemicals are becoming popular nowadays.79 Nonetheless, because of their extremely concentrated doses or mode of use, numerous plants are widely used to treat a variety of anomalies and illnesses without suffering from their poisonous effects, which can cause serious toxicity.80 Several enigmatic issues about the effectiveness and protection of different usual items persist unresolved, along with unexplained hazardous properties.81 Without screening, these uncharacterized compounds may cover up the action of latent compounds that produce inaccurate results.82 Despite being aware of their potential, the majority of chemicals produced from natural products that cause neurodegeneration have only been used conventionally. Their lack of clinical effectiveness that is statistically significant is the main issue.83 Nearly all published studies on Natural substances can safeguard the brain. Compounds are based on in vitro cell-based research, which should be supported by well-conventional in vivo substantiations confirming their anti-AD effects bioavailable and should penetrate BBB. The mechanism of action of phytochemicals that target a wider variety of AD pathophysiology is complex. Although AChE inhibitors can improve cognitive abilities, they are unable to stop or slow the progression of AD. Hence, developing a single treatment that may be effective in contradiction of all important AD targets, such as Aβ formation, fibrillation, Aβ-mediated oxidative stress, and soreness, may be the most fruitful method of classifying AD therapies.82
Future perspective for drug development for AD
Monoclonal antibodies as anti-AD agents
Several ideas have proposed the use of monoclonal antibodies (mAbs) in immunotherapy-related anti-Aβ treatment strategies to precisely eliminate amyloid plaques formed in AD.84 To perform this effect, the appropriate antibody must initially traverse the BBB and enter the brain.85 Later then, it must exhibit a significantly lower affinity for monomers while exhibiting a high affinity for aggregated amyloid, demonstrating its Phagocytosis action mediated by Fc receptors within invading microglia or macrophage/monocytes.86
Due to their poor efficacy, bapineuzumab and further medications that particularly target amyloid plaques and fibrils were not successful.87 Phase 3 trials for medicines that target amyloid monomers, such as solanezumab, have failed to show improvement in cognitive function.88 The anti-amyloid antibodies described in Table 4 exhibited significant outcomes in their phase 2 and 3 studies.
The selective anti-oligomer drug ALZ-801/tramiprosate is regarded as the next generation because it does not bind amyloid plaque and is not connected to ARIA-E events.91 As a result, oral ALZ-801 provides senior patients with the right at-home dosage and may also be used to potentially treat individuals who are presymptomatic and have a high possibility of getting AD.91 A human monoclonal antibody called aducanumab (BIIB037) is being tested as an Alzheimer’s disease treatment. Since 2017, Biogen and Eisai Co., Ltd. have worked together on the international development and commercialization of aducanumab. To estimate the efficiency and attention of aducanumab, two multicenter, double-blind, randomized, placebo-controlled, parallel-group Phase 3 trial studies were steered: EMERGE and ENGAGE. Aducanumab might be the first medication to implicitly alter the course of AD if it is authorized. Acunumab was submitted by Biogen to the FDA in July 2020.92
Artificial Intelligence in AD Management
The understanding of organelle interactions from a pathophysiological and prognosis perspective has advanced thanks to AI and ML technologies in the design and simulation of healthcare for AD.93 deploying ML approaches and ab initio simulated to enhance physiological stability for robust qualitative analyses and to aid in silico simulations and contextual contributes significantly to relatively secure advancements in neurotoxicology/ neuro medicine.94 Also, investigators consume shown the probable, actuality, difficulties, and upcoming advancements that AI and ML bring in enhanced AD treatment strategy and noxiousness forecasts exploiting phytochemical and enhancements in plant nano bionics.95 The NEM-based nano-sensors used in this project are also important instruments for monitoring plant signaling pathways and physiology, permitting us to execute non-invasive, intrusive, and actual time studies of both biological and chemical issues for enhanced crop viability.96 As a result, we believe there is a chance that incorporating information from NEM-based nano-sensors into present precision medicine, urban farming, and seedlings nano bionics is evolving innovations that may serve as an agroecological strategy Figure 2.97
Name of drug | Selectivity for soluble amyloid oligomers | % Clearance of CSF p-tau (% versus placebo) | Clearance of Amyloid plaque | ARIA-E | Clinical trial | Reference |
---|---|---|---|---|---|---|
Aducanumab | L | 15 | H | >30% | Phase 3 | 89 |
gantenerumab | P | 31 | H | >30% | Phase 3 | 85 |
BAN2401 | H | 13 | H | 10% | Phase 2 | 89 |
ALZ-801/ tramiprosate | High (Blocks oligomers creation) | Not assessed | No interface | There were no happenings (due to the lack of contact with the amyloid plaque and vascular amyloid). | Phase 3 | 90 |
Anti-amyloid activity research using humanized monoclonal anti-bodies.
Increasing evidence indicates that AD neuropathology is complex and therefore includes a variety of biological processes. The same diverse strategies are required for its treatment because AD is a complicated and multifaceted illness. The successful eradication of AD pathology is likely facilitated by early disease detection, combination therapy, and lifestyle choices.98 Unhealthy eating can increase the likelihood of developing AD disease, according to several studies.99 In addition, antioxidant-supplemented meals have been demonstrated to enhance the antioxidant status, reinstate rehabilitated AChE and BChE activity, and highlight AD pathophysiology.100 Several research has focused primarily on the suppression and eradication of Aβ also senile plaques, as stated previously in this appraisal, as the amyloid cascade concept has been marginalized for the past two decades. The major goal of current investigation techniques is to measure the amounts of Aβ (1-42), total Tau and phosphorylated Tau in the brain and CSF fluid utilizing MRI imaging processes.101
Dendritic spine deficits clearly show the cognitive deterioration seen in AD, and regrettably, amyloid-centric therapies have failed to improve patients’ cognizance.102 Such flaws should be thought about for potential future innovative drug development as they could be seen as an early sign of memory circuit destabilization.103 The amyloid cascade theory should not be the only criterion for novel pharmacological therapy; rather, synaptic events should be the primary focus to understand the etiology of this disease.104 The upcoming treatment for AD would therefore be created on a chemotherapy-like multidrug and multi-target strategy. Even however, it is unclear how these potential medication therapies will be communicated with the appropriate subgroups or even in what order they would be chosen. Such trends would effectively change the therapeutic landscape, such as non-amyloid methods.82
CONCLUSION
The phytoconstituents will undoubtedly contribute to the development of novel, safer AD treatments that are superior to the currently existing pharmaceutical options, whether taken alone or in combination.
Cite this article
Sharma H, Chandra P Challenges and Future Prospects: A Benefaction of Phytoconstituents on Molecular Targets Pertaining to Alzheimer’s Disease. Int. J. Pharm. Investigation. 2024;14(1):117-26.
ACKNOWLEDGEMENT
The authors are thankful to the Principal, Department of Pharmacy, Teerthanker Mahaveer University, Moradabad for providing the necessary facilities.
ABBREVIATIONS
ABB | Amyloid β-protein |
---|---|
AchE | Acetylcholinesterase |
AD | Alzheimer’s Disease |
AI | Artificial Intelligence |
APP | Amyloid precursor protein |
Aβ | Amyloid-beta |
BACE1 | Beta-site APP-cleaving enzyme 1 |
BBB | Blood-brain barrier |
BChE | Butyrylcholinesterase |
FDA | Food and Drug Administration |
GSK3 | Glycogen synthase kinase 3 |
IL-6 | Interleukin 6 |
LPA | Lysophosphatidic acid |
LRP-1 | Low-density lipoprotein receptor-related protein-1 |
MABS | Monoclonal antibodies |
ML | Machine learning |
MRI | Magnetic resonance imaging |
NEM | Nano-engineered material |
NEP | Neprilysin |
NETs | Neurofibrillary tangles |
PLA | Phospholipase A2 |
PS1 | Presenilin-1 |
PS2 | Presenilin-2 |
RAGE | Receptor for advanced glycation end products |
ROS | Reactive oxidative species |
TLR2 | Toll-like receptor 2 |
TNF-α | Tumor necrosis factor α |
References
- Alzheimer A. Über eigenartige Erkrankung der Hirnrinde. All Z psychiatr. 1907;64:146-8. [Google Scholar]
- Korolev IO. Alzheimer’s disease: a clinical and basic science review. Med Student Res J. 2014;4(1):24-33. [Google Scholar]
- Serrano-Pozo A, Frosch MP, Masliah E, Hyman BT. Neuropathological Alterations in Alzheimer Disease. Cold Spring Harb Perspect Med. 2011;1(1):a006189 [CrossRef] | [Google Scholar]
- Kang J, Lemaire HG, Unterbeck A, Salbaum JM, Masters CL, Grzeschik KH, et al. The precursor of Alzheimer’s disease amyloid A4 protein resembles a cell-surface receptor. Nature. 1987;325(6106):733-6. [PubMed] | [CrossRef] | [Google Scholar]
- Gupta S, Banerjee P, Laferla FM, Selkoe DJ. Alzheimer’s disease: genes, proteins, and therapy. Physiol Rev. 2010;81:741-66. [PubMed] | [CrossRef] | [Google Scholar]
- Chow VW, Mattson MP, Wong PC, Gleichmann M. An overview of APP processing enzymes and products. Neuro Molecular Med. 2010;12(1):1-12. [PubMed] | [CrossRef] | [Google Scholar]
- Halliwell B, Clement MV, Long LH. Hydrogen peroxide in the human body. FEBS Lett. 2000;486(1):10-3. [PubMed] | [CrossRef] | [Google Scholar]
- Kolarova M, García-Sierra F, Bartos A, Ricny J, Ripova D. Structure and pathology of Tau protein in Alzheimer disease. Int J Alzheimers Dis. 2012;2012:731526 [PubMed] | [CrossRef] | [Google Scholar]
- Pchitskaya EI, Zhemkov VA, Bezprozvanny IB. Dynamic microtubules in Alzheimer’s disease: association with dendritic spine pathology. Biochemistry (Mosc). 2018;83(9):1068-74. [PubMed] | [CrossRef] | [Google Scholar]
- Baas PW, Rao AN, Matamoros AJ, Leo L. Stability properties of neuronal microtubules. Cytoskeleton (Hoboken). 2016;73(9):442-60. [PubMed] | [CrossRef] | [Google Scholar]
- Mietelska-Porowska A, Wasik U, Goras M, Filipek A, Niewiadomska G. Tau protein modifications and interactions: their role in function and dysfunction. Int J Mol Sci. 2014;15(3):4671-713. [PubMed] | [CrossRef] | [Google Scholar]
- Tiwari S, Atluri V, Kaushik A, Yndart A, Nair M. Alzheimer’s disease: pathogenesis, diagnostics, and therapeutics. Int J Nanomedicine. 2019;14:5541-54. [PubMed] | [CrossRef] | [Google Scholar]
- Persson T, Popescu BO, Cedazo-Minguez A. Oxidative stress in Alzheimer’s disease: why did antioxidant therapy fail?. Oxid Med Cell Longev. 2014;2014:427318 [PubMed] | [CrossRef] | [Google Scholar]
- Singh AV, Jungnickel H, Leibrock L, Tentschert J, Reichardt P, Katz A, et al. ToF-SIMS 3D imaging unveils important insights on the cellular microenvironment during biomineralization of gold nanostructures. Sci Rep. 2020;10(1):261 [PubMed] | [CrossRef] | [Google Scholar]
- Henstridge CM, Hyman BT, Spires-Jones TL. Beyond the neuron-cellular interactions early in Alzheimer disease pathogenesis. Nat Rev Neurosci. 2019;20(2):94-108. [PubMed] | [CrossRef] | [Google Scholar]
- Karch CM, Goate AM. Alzheimer’s disease risk genes and mechanisms of disease pathogenesis. Biol Psychiatry. 2015;77(1):43-51. [PubMed] | [CrossRef] | [Google Scholar]
- Cummings JL, Back C. The cholinergic hypothesis of neuropsychiatric symptoms in Alzheimer’s disease. Am J Geriatr Psychiatry. 1998;6((2);Suppl 1):S64-78. [PubMed] | [CrossRef] | [Google Scholar]
- Braak H, Del Tredici K. Alzheimer’s disease: pathogenesis and prevention. Alzheimers Dement. 2012;8(3):227-33. [PubMed] | [CrossRef] | [Google Scholar]
- Denk J, Boelmans K, Siegismund C, Lassner D, Arlt S, Jahn H, et al. MicroRNA profiling of CSF reveals potential biomarkers to detect Alzheimers disease. PLOS ONE. 2015;10(5):e0126423 [PubMed] | [CrossRef] | [Google Scholar]
- Perl DP. Neuropathology of Alzheimer’s disease Transl Pers Med A J Transl Pers Med. Mt Sinai J Med. 2010;77(1):32-42. [PubMed] | [CrossRef] | [Google Scholar]
- Prasad ASV. Essentials of Anatomy as Related to Alzheimer’s disease: a review. J Alzheimers Dis, (Park). 2020;10(486):2 [PubMed] | [CrossRef] | [Google Scholar]
- Boeve BF, Lang AE, Litvan I. Corticobasal degeneration and its relationship to progressive supranuclear palsy and frontotemporal dementia. Ann Neurol. 2003;54(Suppl 5):S15-9. [PubMed] | [CrossRef] | [Google Scholar]
- Braak H, Braak E. Diagnostic criteria for neuropathologic assessment of Alzheimer’s disease. Neurobiol Aging. 1997;18((4);Suppl):S85-8. [PubMed] | [CrossRef] | [Google Scholar]
- Thal DR, Rüb U, Orantes M, Braak H. Phases of Aβ-deposition in the human brain and its relevance for the development of AD. Neurology. 2002;58(12):1791-800. [PubMed] | [CrossRef] | [Google Scholar]
- Shayan M, Barangi S, Hosseinzadeh H, Mehri S. The protective effect of natural or chemical compounds against arsenic-induced neurotoxicity: cellular and molecular mechanisms. Food Chem Toxicol. 2023;175:113691 [PubMed] | [CrossRef] | [Google Scholar]
- Cummings J, Jones R, Wilkinson D, Lopez O, Gauthier S, Waldemar G, et al. Effect of donepezil on cognition in severe Alzheimer’s disease: a pooled data analysis. J Alzheimers Dis. 2010;21(3):843-51. [PubMed] | [CrossRef] | [Google Scholar]
- Ballard CG, Gauthier S, Cummings JL, Brodaty H, Grossberg GT, Robert P, et al. Management of agitation and aggression associated with Alzheimer disease. Nat Rev Neurol. 2009;5(5):245-55. [PubMed] | [CrossRef] | [Google Scholar]
- Del Prado-Audelo ML, Caballero-Florán IH, Meza-Toledo JA, Mendoza-Muñoz N, González-Torres M, Florán B, et al. Formulations of curcumin nanoparticles for brain diseases. Biomolecules. 2019;9(2):56 [PubMed] | [CrossRef] | [Google Scholar]
- Chopade P, Chopade N, Zhao Z, Mitragotri S, Liao R, Chandran Suja V, et al. Alzheimer’s and Parkinson’s disease therapies in the clinic. Bioeng Transl Med. 2023;8(1):e10367 [PubMed] | [CrossRef] | [Google Scholar]
- Bandura A. Health promotion from the perspective of social cognitive theory. Psychol Heal. 1998;13(4):623-49. [CrossRef] | [Google Scholar]
- Bravo-Vázquez LA, Mora-Hernández EO, Rodríguez AL, Sahare P, Bandyopadhyay A, Duttaroy AK, et al. Current advances of plant-based vaccines for neurodegenerative diseases. Pharmaceutics. 2023;15(2):711 [PubMed] | [CrossRef] | [Google Scholar]
- Acero N, Ortega T, Vi l lag rasa V, Leon G, Muñoz-Mingarro D, Castillo E, et al. Phytotherapeutic alternatives for neurodegenerative dementias: scientific review, discussion and therapeutic proposal [scientific review]. Phytother Res. 2023;37(3):1176-211. [PubMed] | [CrossRef] | [Google Scholar]
- Yang F, Lim GP, Begum AN, Ubeda OJ, Simmons MR, Ambegaokar SS, et al. Curcumin inhibits formation of amyloid β oligomers and fibrils, binds plaques, and reduces amyloid in vivo. J Biol Chem. 2005;280(7):5892-901. [PubMed] | [CrossRef] | [Google Scholar]
- Mishra S, Palanivelu K. The effect of curcumin (turmeric) on Alzheimer’s disease: an overview. Ann Indian Acad Neurol. 2008;11(1):13-9. [PubMed] | [CrossRef] | [Google Scholar]
- Vickers NJ. Animal communication: when I’m calling you, will you answer too?. Curr Biol. 2017;27(14):R713-5. [PubMed] | [CrossRef] | [Google Scholar]
- Huang HC, Xu K, Jiang ZF. Curcumin-mediated neuroprotection against amyloid-β-induced mitochondrial dysfunction involves the inhibition of GSK-3β. J Alzheimers Dis. 2012;32(4):981-96. [PubMed] | [CrossRef] | [Google Scholar]
- Reddy PH, Manczak M, Yin X, Grady MC, Mitchell A, Tonk S, et al. Protective effects of Indian spice curcumin against amyloid-β in Alzheimer’s disease. J Alzheimers Dis. 2018;61(3):843-66. [PubMed] | [CrossRef] | [Google Scholar]
- Zhang L, Fiala M, Cashman J, Sayre J, Espinosa A, Mahanian M, et al. Curcuminoids enhance amyloid-β uptake by macrophages of Alzheimer’s disease patients. J Alzheimers Dis. 2006;10(1):1-7. [PubMed] | [CrossRef] | [Google Scholar]
- Colciaghi F, Borroni B, Zimmermann M, Bellone C, Longhi A, Padovani A, et al. Amyloid precursor protein metabolism is regulated toward alpha-secretase pathway by Ginkgo biloba extracts. Neurobiol Dis. 2004;16(2):454-60. [PubMed] | [CrossRef] | [Google Scholar]
- Bodovitz S, Klein WL. Cholesterol modulates α-secretase cleavage of amyloid precursor protein. J Biol Chem. 1996;271(8):4436-40. [PubMed] | [CrossRef] | [Google Scholar]
- Song XY, Hu JF, Chu SF, Zhang Z, Xu S, Yuan YH, et al. Ginsenoside Rg1 attenuates okadaic acid induced spatial memory impairment by the GSK3β/tau signaling pathway and the Aβ formation prevention in rats. Eur J Pharmacol. 2013;710(1-3):29-38. [PubMed] | [CrossRef] | [Google Scholar]
- Qiu J, Li W, Feng SH, Wang M, He ZY. Ginsenoside Rh2 promotes nonamyloidgenic cleavage of amyloid precursor protein via a cholesterol-dependent pathway. Genet Mol Res. 2014;13(2):3586-98. [PubMed] | [CrossRef] | [Google Scholar]
- Dubey T, Chinnathambi S. Brahmi (Bacopa monnieri): An ayurvedic herb against the Alzheimer’s disease. Arch Biochem Biophys. 2019;676:108153 [PubMed] | [CrossRef] | [Google Scholar]
- Holcomb LA, Dhanasekaran M, Hitt AR, Young KA, Riggs M, Manyam BV, et al. Bacopa monniera extract reduces amyloid levels in PSAPP mice. J Alzheimers Dis. 2006;9(3):243-51. [PubMed] | [CrossRef] | [Google Scholar]
- Defillipo PP, Raposo AH, Fedoce AG, Ferreira AS, Polonini HC, Gattaz WF, et al. Inhibition of cPLA2 and sPLA2 activities in primary cultures of rat cortical neurons by Centella asiatica water extract. Nat Prod Commun. 2012;7((7): 1934578X1200700709):841-3. [PubMed] | [Google Scholar]
- Soumyanath A, Zhong YP, Henson E, Wadsworth T, Bishop J, Gold BG, et al. Centella asiatica extract improves behavioral deficits in a mouse model of Alzheimer’s disease: investigation of a possible mechanism of action. Int J Alzheimers Dis. 2012;2012:381974 [PubMed] | [CrossRef] | [Google Scholar]
- Meng P, Yoshida H, Matsumiya T, Imaizumi T, Tanji K, Xing F, et al. Carnosic acid suppresses the production of amyloid-β 1-42 by inducing the metalloprotease gene TACE/ADAM17 in SH-SY5Y human neuroblastoma cells. Neurosci Res. 2013;75(2):94-102. [PubMed] | [CrossRef] | [Google Scholar]
- Meng P, Yoshida H, Tanji K, Matsumiya T, Xing F, Hayakari R, et al. Carnosic acid attenuates apoptosis induced by amyloid-β 1-42 or 1-43 in SH-SY5Y human neuroblastoma cells. Neurosci Res. 2015;94:1-9. [PubMed] | [CrossRef] | [Google Scholar]
- Jayaprakasam B, Padmanabhan K, Nair MG. Withanamides in Withania somnifera fruit protect PC-12 cells from β-amyloid responsible for Alzheimer’s disease. Phytother Res. 2010;24(6):859-63. [PubMed] | [CrossRef] | [Google Scholar]
- Sehgal N, Gupta A, Valli RK, Joshi SD, Mills JT, Hamel E, et al. Withania somnifera reverses Alzheimer’s disease pathology by enhancing low-density lipoprotein receptor-related protein in liver. Proc Natl Acad Sci U S A. 2012;109(9):3510-5. [PubMed] | [CrossRef] | [Google Scholar]
- Nuutinen T, Suuronen T, Kauppinen A, Salminen A. Clusterin: a forgotten player in Alzheimer’s disease. Brain Res Rev. 2009;61(2):89-104. [PubMed] | [CrossRef] | [Google Scholar]
- Singh AV. Commentary on “peptide-conjugated nanoparticles as targeted anti-angiogenesis therapeutic and diagnostic in cancer” by Shaker A. Mousa, pharmaceutical research institute. Curr Med Chem 12144, United States-. 2020;27(17):2927-8. [PubMed] | [CrossRef] | [Google Scholar]
- Lee YJ, Choi DY, Han SB, Kim YH, Kim KH, Hwang BY, et al. Inhibitory effect of ethanol extract of Magnolia officinalis on memory impairment and amyloidogenesis in a transgenic mouse model of Alzheimer’s disease via regulating β-secretase activity. Phytother Res. 2012;26(12):1884-92. [PubMed] | [CrossRef] | [Google Scholar]
- Rane JS, Bhaumik P, Panda D. Curcumin inhibits tau aggregation and disintegrates preformed tau filaments in vitro. J Alzheimers Dis. 2017;60(3):999-1014. [PubMed] | [CrossRef] | [Google Scholar]
- Zhang X, Shi M, Ye R, Wang W, Liu X, Zhang G, et al. Ginsenoside Rd attenuates Tau protein phosphorylation via the PI3K/AKT/GSK-3β pathway after transient forebrain ischemia. Neurochem Res. 2014;39(7):1363-73. [PubMed] | [CrossRef] | [Google Scholar]
- Cornejo A, Aguilar Sandoval F, Caballero L, Machuca L, Muñoz P, Caballero J, et al. Rosmarinic acid prevents fibrillization and diminishes vibrational modes associated to β sheet in Tau protein linked to Alzheimer’s disease. J Enzyme Inhib Med Chem. 2017;32(1):945-53. [PubMed] | [CrossRef] | [Google Scholar]
- Xian YF, Lin ZX, Mao QQ, Hu Z, Zhao M, Che CT, et al. Bioassay-guided isolation of neuroprotective compounds from Uncaria rhynchophylla against beta-amyloid-induced neurotoxicity. Evid Based Complement Alternat Med. 2012;2012:802625 [PubMed] | [CrossRef] | [Google Scholar]
- Ho L, Yemul S, Wang J, Pasinetti GM. Grape seed polyphenolic extract as a potential novel therapeutic agent in tauopathies. J Alzheimers Dis. 2009;16(2):433-9. [PubMed] | [CrossRef] | [Google Scholar]
- Sun Q, Jia N, Li X, Yang J, Chen G. Grape seed proanthocyanidins ameliorate neuronal oxidative damage by inhibiting GSK-3β-dependent mitochondrial permeability transition pore opening in an experimental model of sporadic Alzheimer’s disease. Aging (Albany, NY). 2019;11(12):4107-24. [PubMed] | [CrossRef] | [Google Scholar]
- Shi LL, Yang WN, Chen XL, Zhang JS, Yang PB, Hu XD, et al. The protective effects of tanshinone IIA on neurotoxicity induced by β-amyloid protein through calpain and the p35/Cdk5 pathway in primary cortical neurons. Neurochem Int. 2012;61(2):227-35. [PubMed] | [CrossRef] | [Google Scholar]
- Durairajan SSK, Yuan Q, Xie L, Chan WS, Kum WF, Koo I, et al. Salvianolic acid B inhibits Aβ fibril formation and disaggregates preformed fibrils and protects against Aβ-induced cytotoxicty. Neurochem Int. 2008;52(4-5):741-50. [PubMed] | [CrossRef] | [Google Scholar]
- Yu N, Huang Y, Jiang Y, Zou L, Liu X, Liu S, et al. Ganoderma lucidum triterpenoids (GLTs) reduce neuronal apoptosis via inhibition of ROCK signal pathway in APP/PS1 transgenic Alzheimer’s disease mice. Oxid Med Cell Longev. 2020;2020:9894037 [PubMed] | [CrossRef] | [Google Scholar]
- Ray B, Chauhan B. N, K Lahiri D. The “aged garlic extract” (AGE) and one of its active ingredients S-allyl-L-cysteine (SAC) as potential preventive and therapeutic agents for Alzheimer’s disease (AD). Curr Med Chem. 2011;18(22):3306-13. [PubMed] | [CrossRef] | [Google Scholar]
- Sonawane SK, Chidambaram H, Boral D, Gorantla NV, Balmik AA, Dangi A, et al. EGCG impedes human Tau aggregation and interacts with Tau. Sci Rep. 2020;10(1):12579 [PubMed] | [CrossRef] | [Google Scholar]
- Gorantla NV, Das R, Mulani FA, Thulasiram HV, Chinnathambi S. Neem derivatives inhibits tau aggregation. J Alzheimers Dis Rep. 2019;3(1):169-78. [PubMed] | [CrossRef] | [Google Scholar]
- English LD. STEM education K-12: perspectives on integration. Int J STEM Educ. 2016;3(1):1-8. [CrossRef] | [Google Scholar]
- Yang C, Li X, Gao W, Wang Q, Zhang L, Li Y, et al. Cornel iridoid glycoside inhibits tau hyperphosphorylation via regulating cross-talk between GSK-3β and PP2A signaling. Front Pharmacol. 2018;9:682 [PubMed] | [CrossRef] | [Google Scholar]
- Martin MD, Calcul L, Smith C, Jinwal UK, Fontaine SN, Darling A, et al. Synthesis, stereochemical analysis, and derivatization of myricanol provide new probes that promote autophagic tau clearance. ACS Chem Biol. 2015;10(4):1099-109. [PubMed] | [CrossRef] | [Google Scholar]
- George RC, Lew J, Graves DJ. Interaction of cinnamaldehyde and epicatechin with tau: implications of beneficial effects in modulating Alzheimer’s disease pathogenesis. J Alzheimers Dis. 2013;36(1):21-40. [PubMed] | [CrossRef] | [Google Scholar]
- Jahanshahi M, Nikmahzar E, Yadollahi N, Ramazani K. Protective effects of Ginkgo biloba extract (EGB 761) on astrocytes of rat hippocampus after exposure with scopolamine. Anat Cell Biol. 2012;45(2):92-6. [PubMed] | [CrossRef] | [Google Scholar]
- Hafiz ZZ, Amin M, Afif M, Johari James RM, Teh LK, Salleh MZ, Adenan MI, et al. Inhibitory effects of raw-extract Centella asiatica (RECA) on acetylcholinesterase, inflammations, and oxidative stress activities via in vitro and in vivo. Molecules. 2020;25(4):892 [PubMed] | [CrossRef] | [Google Scholar]
- Dastmalchi K, Ollilainen V, Lackman P, Boije af Gennäs GB, Dorman HJD, Järvinen PP, et al. Acetylcholinesterase inhibitory guided fractionation of Melissa officinalis L. Bioorg Med Chem. 2009;17(2):867-71. [PubMed] | [CrossRef] | [Google Scholar]
- Mahboubi M. Melissa officinalis and rosmarinic acid in management of memory functions and Alzheimer disease. Asian Pac J Trop Biomed. 2019;9(2):47 [CrossRef] | [Google Scholar]
- Sharma R, Kuca K, Nepovimova E, Kabra A, Rao MM, Prajapati PK, et al. Traditional Ayurvedic and herbal remedies for Alzheimer’s disease: from bench to bedside. Expert Rev Neurother. 2019;19(5):359-74. [PubMed] | [CrossRef] | [Google Scholar]
- Haiming G, Danping Y, Shulin S, Xinmin L. Least-squares mixed finite element method for a class of stokes equation. Appl Math Mech. 2000;21(5):557-66. [CrossRef] | [Google Scholar]
- Dall’Acqua S. Plant-derived acetylcholinesterase inhibitory alkaloids for the treatment of Alzheimer’s disease. Bot Targets Ther. 2013;3:19-28. [CrossRef] | [Google Scholar]
- Marco-Contelles J, do Carmo Carreiras M, Rodríguez C, Villarroya M, García AG. Synthesis and pharmacology of galantamine. Chem Rev. 2006;106(1):116-33. [PubMed] | [CrossRef] | [Google Scholar]
- Rates SMK. Plants as source of drugs. Toxicon. 2001;39(5):603-13. [PubMed] | [CrossRef] | [Google Scholar]
- Cummings J, Lee G, Ritter A, Sabbagh M, Zhong K. Alzheimer’s disease drug development pipeline: 2020. Alzheimers Dement (N Y). 2020;6(1):e12050 [PubMed] | [CrossRef] | [Google Scholar]
- Sen S, Chakraborty R. Toward the integration and advancement of herbal medicine: a focus on traditional Indian medicine. Bot Targets Ther. 2015;5:33-44. [CrossRef] | [Google Scholar]
- Park SY. Potential therapeutic agents against Alzheimer’s disease from natural sources. Arch Pharm Res. 2010;33(10):1589-609. [PubMed] | [CrossRef] | [Google Scholar]
- Dhage PA, Sharbidre AA, Dakua SP, Balakrishnan S. Leveraging hallmark Alzheimer’s molecular targets using phytoconstituents: current perspective and emerging trends. Biomed Pharmacother. 2021;139:111634 [PubMed] | [CrossRef] | [Google Scholar]
- Brewer GJ. Copper excess, zinc deficiency, and cognition loss in Alzheimer’s disease. BioFactors. 2012;38(2):107-13. [PubMed] | [CrossRef] | [Google Scholar]
- Sevigny J, Chiao P, Bussière T, Weinreb PH, Williams L, Maier M, et al. The antibody aducanumab reduces Aβ plaques in Alzheimer’s disease. Nature. 2016;537(7618):50-6. [PubMed] | [CrossRef] | [Google Scholar]
- Bohrmann B, Baumann K, Benz J, Gerber F, Huber W, Knoflach F, et al. Gantenerumab: a novel human anti-Aβ antibody demonstrates sustained cerebral amyloid-β binding and elicits cell-mediated removal of human amyloid-β. J Alzheimers Dis. 2012;28(1):49-69. [PubMed] | [CrossRef] | [Google Scholar]
- Logovinsky V, Satlin A, Lai R, Swanson C, Kaplow J, Osswald G, et al. Safety and tolerability of BAN2401-a clinical study in Alzheimer’s disease with a protofibril selective Aβ antibody. Alzheimers Res Ther. 2016;8(1):14 [PubMed] | [CrossRef] | [Google Scholar]
- Salloway S, Sperling R, Fox NC, Blennow K, Klunk W, Raskind M, et al. Two phase 3 trials of Bapineuzumab in mild-to-moderate Alzheimer’s disease. N Engl J Med. 2014;370(4):322-33. [PubMed] | [CrossRef] | [Google Scholar]
- Honig LS, Vellas B, Woodward M, Boada M, Bullock R, Borrie M, et al. Trial of solanezumab for mild dementia due to Alzheimer’s disease. N Engl J Med. 2018;378(4):321-30. [PubMed] | [CrossRef] | [Google Scholar]
- Schneider L. A resurrection of aducanumab for Alzheimer’s disease. Lancet Neurol. 2020;19(2):111-2. [PubMed] | [CrossRef] | [Google Scholar]
- Kocis P, Tolar M, Yu J, Sinko W, Ray S, Blennow K, et al. Elucidating the Aβ42 anti-aggregation mechanism of action of tramiprosate in Alzheimer’s disease: integrating molecular analytical methods, pharmacokinetic and clinical data. CNS Drugs. 2017;31(6):495-509. [PubMed] | [CrossRef] | [Google Scholar]
- Tolar M, Abushakra S, Hey JA, Porsteinsson A, Sabbagh M. Aducanumab, gantenerumab, BAN2401, and ALZ-801—the first wave of amyloid-targeting drugs for Alzheimer’s disease with potential for near term approval. Alzheimers Res Ther. 2020;12(1):95 [PubMed] | [CrossRef] | [Google Scholar]
- Mukhopadhyay S, Banerjee D. A primer on the evolution of aducanumab: the first antibody approved for treatment of Alzheimer’s disease. J Alzheimers Dis. 2021;83(4):1537-52. [PubMed] | [CrossRef] | [Google Scholar]
- Singh AV, Ansari MHD, Rosenkranz D, Maharjan RS, Kriegel FL, Gandhi K, et al. Artificial intelligence and machine learning in computational nanotoxicology: unlocking and empowering nanomedicine. Adv Healthc Mater. 2020;9(17):e1901862 [PubMed] | [CrossRef] | [Google Scholar]
- Singh AV, Maharjan RS, Kanase A, Siewert K, Rosenkranz D, Singh R, et al. Machine-learning-based approach to decode the influence of nanomaterial properties on their interaction with cells. ACS Appl Mater Interfaces. 2021;13(1):1943-55. [PubMed] | [CrossRef] | [Google Scholar]
- Borderud SP, Li Y, Burkhalter JE, Sheffer CE, Ostroff JS. Electronic cigarette uses among patients with cancer: characteristics of electronic cigarette users and their smoking cessation outcomes. Cancer. 2014;120(22):3527-35. [PubMed] | [CrossRef] | [Google Scholar]
- Ansari MHD, Lavhale S, Kalunke RM, Srivastava PL, Pandit V, Gade S, et al. Recent advances in plant nanobionics and nanobiosensors for toxicology applications. Curr Nanosci. 2020;16(1):27-41. [CrossRef] | [Google Scholar]
- Tiwari Pandey A, Pandey I, Zamboni P, Gemmati D, Kanase A, Singh AV, et al. Traditional herbal remedies with a multifunctional therapeutic approach as an implication in COVID-19 associated co-infections. Coatings. 2020;10(8):761 [CrossRef] | [Google Scholar]
- Vikram Singh A, Sigloch H, Laux P, Luch A, Wagener S, Tentschert J, et al. Micro/nanoplastics: an emerging environmental concern for the future decade. Front Nanosci Nanotech. 2021;7(1):1-2. [CrossRef] | [Google Scholar]
- Kamphuis PJGH, Scheltens P. Can nutrients prevent or delay onset of Alzheimer’s disease?. J Alzheimers Dis. 2010;20(3):765-75. [PubMed] | [CrossRef] | [Google Scholar]
- Agunloye OM, Oboh G, Falade AO. Pleurotus ostreatus and Lentinus subnudus supplemented diets restore altered acetylcholinesterase and butyrylcholinesterase activities and improve antioxidant status in transgenic Drosophila melanogaster model. J Diet Suppl. 2021;18(4):372-86. [PubMed] | [CrossRef] | [Google Scholar]
- Falcon C, Tucholka A, Monté-Rubio GC, Cacciaglia R, Operto G, Rami L, et al. Longitudinal structural cerebral changes related to core CSF biomarkers in preclinical Alzheimer’s disease: A study of two independent datasets. NeuroImage Clin. 2018;19:190-201. [PubMed] | [CrossRef] | [Google Scholar]
- Counts SE, Ikonomovic MD, Mercado N, Vega IE, Mufson EJ. Biomarkers for the early detection and progression of Alzheimer’s disease. Neurotherapeutics. 2017;14(1):35-53. [PubMed] | [CrossRef] | [Google Scholar]
- Love MN, Clark DG, Cochran JN, Den Beste KA, Geldmacher DS, Benzinger TL, et al. Clinical, imaging, pathological, and biochemical characterization of a novel presenilin 1 mutation. Neurobiol Aging. 2017 49;N135Y causing Alzheimer’s disease:216-e7 [PubMed] | [CrossRef] | [Google Scholar]
- Knopman DS, Amieva H, Petersen RC, Chételat G, Holtzman DM, Hyman BT, et al. Alzheimer disease. Nat Rev Dis Primers. 2021;7(1):33 [PubMed] | [CrossRef] | [Google Scholar]