Contents
ABSTRACT
Background
Pharmaceutical residues are biologically active in trace concentrations. This has increased worries about their potential hazards to human health and living organisms and has highlighted the need to treat them once they are discharged into aquatic ecosystems. In this study, the potential of removal of Ciprofloxacin antibiotic (CIP) using novel magnetic Activated carbon nanocomposite (Fe3O4/AC) was studied.
Materials and Methods
For conducting equilibrium analysis, a batch system was considered and operated under conditions including CIP initial concentration, solution pH, Fe3O4/AC dosage, and mixing time. Analyzing the experimental data was done using different isotherm and kinetic models.
Results
The analysis of the physical and chemical properties of Fe3O4/AC was indicative of the successful preparation of Fe3O4/AC; the surface area, microspores volume, and average pore diameter were observed to be 129.2 m2/g, 0.512 cm3/g, and 4.6 nm, respectively. A removal percentage of 100% was obtained for CIP using Fe3O4/AC under optimal values of the studied parameters; the optimum values of these parameters were as follows: solution pH of 5, adsorbent dosage of 0.8 g/L, and an initial CIP amount of 25 mg/L. The Langmuir model was found to be best for labeling the equilibrium data (maximum CIP adsorption capacity of 156.2 mg/g was obtained based on the Langmuir model). The fit of experimental data kinetic with the pseudo-second-order equation was also detected.
Conclusion
The obtained results let us suggest Fe3O4/AC nanocomposite as a decidedly competent adsorbent for removing CIP.
INTRODUCTION
An extensive range of cytostatic drugs are detected in aquatic ecosystems, chiefly in groundwater and surface water, the sources of which are normally diverse wastewater effluents.1 These pharmaceutical residues are linked with hospitals, animals farming, and industrial agricultural activities.2,3 These compounds are biologically active in trace concentrations. This has increased worries about their potential hazards to the human health and living organisms and has highlighted the need to treat them once they are discharged into aquatic ecosystems.4,5
Ciprofloxacin (CIP) is recorded as a hazardous material included in the “class 1 classification of drugs and is not biodegradable and is seldom adsorbed to sediment owing to its high hydrophilicity, low log Kow value (Kow= 0.63) and high mobility in aqueous solutions.6–8
Effluent and drinking water treatment operations have not been designed specifically for the removal of pharmaceuticals.9 Hence, plans for the elimination of pharmaceuticals from water samples have been predominantly investigated.10 Advanced wastewater treatment operations propose great removal rates for drug compounds.11,12 However, there are concerns in regard with the unpleasant by-products and cost-effectiveness of these treatment processes.13 Among above-mentioned methods, adsorption has been reported as a more preferable technique than the others owing to its high versatility and efficiency.14,15
Unfortunately, activated carbon is environmentally unfriendly and a costly adsorbent because it is derived from non-renewable materials, making its use limited on industrial scales.16,17Therefore, it is necessary to prepare low-cost activated carbon from materials such as waste bamboo, waste tires, waste tea, sugar cane bagasse, and rice husks. Rice is a rich source of carbohydrates in many countries of the world, chiefly in Asia. Each kilogram of harvested grain generates 1- 1.5 kg of straw, giving an approximately global rice straw production of 750-1120 million tons.18 Most of these rice wastes are left or burned on agricultural lands and cause possible damage to the environment due to methane emissions and airborne carbon particulate matter, which can have harmful effects on human health and climate change.19 Carbonization and activation of rice straw in inert atmosphere produces highly porous carbon with a large specific surface area and high porosity.20 Although, newly porous carbons synthesized from rice straw have attracted attentions in the removal of pollutants from water, however, more research is needed on rice straw modifications such as acid treatment and the introduction of different functional groups on its surface for a better adsorption performance of contaminants.21
In this study, Rice was studied as feedstock for the preparation of Activated Carbon (AC) functionalized via magnetite (Fe3O4) and utilized for the elimination of CIP from water samples. The effect of pH, contact time, Fe3O4/AC dose, and initial CIP concentration were also checked. Moreover, isotherm and kinetics of CIP adsorption were considered.
MATERIALS AND METHODS
Chemicals
In this study, CIP antibiotic with the chemical formula (C17H18N3O3F), the molecular weight of 331.3 g/mol and it was produced by Sigma-Aldrich Company and was used in the preparation of the stock solution. Also, H2SO4 and NaOH were prepared from Merck Company, Germany (laboratory purity) to adjust the pH of the solution. Double distilled water was used in all stages of the experiments.
Preparation of Activated Carbon (AC)
The raw material used in this study for preparing the activated carbon was rice straw obtained from Rasht, Iran. After washing them, they dried in an oven, powdered using a steel blender, and sieved using a mesh 40 sieve. 15 g of the sample was then placed in Teflon-lined hydrothermal autoclave reactors. After that, it was placed in a furnace at 500°C for one hr. The sample was then rinsed with deionized water several times, and rinsing was continued until reaching the pH of the wastewater to a neutral state. It finally underwent in drying process at 110°C for 24 hr.
Preparation of magnetite-activated carbon (AC/Fe3O4)
This section started with the dispersion of the obtained AC in 70 mL EtOH and the homogenization of the prepared mixture via ultrasonication. After 3 hr and the addition of 1.5 g FeCl3.6H2O and 3.2 g C2H3NaO2 to the mixture at 25°C, it was stirred for 30 min. Finally, after transferring the mixture into a 100 mL Teflon-lined stainless-steel autoclave for 10 hr at 200°C and washing several times with ethanol and deionized water, it was exposed to a drying process in a vacuum for 6 hr at 60°C.
Adsorption tests
The static adsorption batch experiments were conducted in a 200 mL conical flask with 0.08 g of the AC/Fe3O4 nanocomposite and 100 mL of various primary concentrations of CIP solution, and after that the samples were kept in a water bath shaker at 180 rpm for 24 hr at 25°C. Moreover, the impacts of pH factor on the CIP adsorption were altered from 3.0–10.0, utilizing hydrochloric acid and/or sodium hydroxide solutions (0.10 mol/L). The kinetic investigations were carried out in the same way by taking 100 mL of CIP (10-100 mg/L) and 0.08 g of AC/Fe3O4. Finally, the AC/ Fe3O4 were isolated at the designed adsorption time through magnetic decantation. Meanwhile, the residual concentration of CIP molecules in the bulk (Ce) was measured utilizing UV-visible spectrophotometry at 280 nm and removal efficiency and the adsorption capacity of CIP were computed in accordance with Equations (1 and 2): 22–24
RESULTS
According to the results of Figure 1, the time was effective on removal efficiency, and the optimum contact time to obtain the best removal efficiency was 90 min. After the mentioned time, unchanged removal efficiency is detected, which is representative of the saturation of active sites on the AC/Fe3O4 surface. In Figure 1, the CIP adsorptions of AC/Fe3O4 as a function of CIP concentrations were represented. Based on Figure 1, for the concentration of CIP higher than 25 mg/L, a decrease in the ability of AC/Fe3O4 to bind to it is detected. Hence, the optimum concentration of CIP in the present study was 25 mg/L.
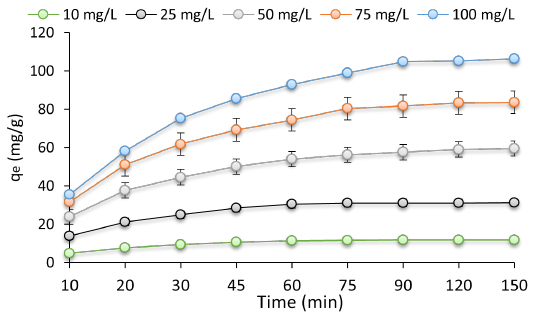
Figure 1:
Effect of contact time and concentration on the removal of CIP (dosage: 0.8 g/L, pH: 5 and tem; 25°C).
According to the results, there is a decline in the adsorption efficiency of the studied pollutant by reducing pH (Figure 2). Moreover, results evidently introduce a pH of 5 as the optimum pH for CIP removal.
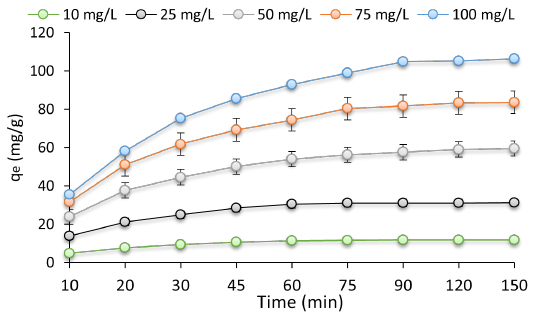
Figure 2:
Effect of different pH on the removal of CIP (CIP concentration: 10 mg/L, dosage: 0.8 g/L, contact time: 90 min and tem; 25°C).
The results show that when the dosage of AC/Fe3O4 increased, the CIP removal increased and, no decreasing trend was observed in their adsorption process (Figure 3).
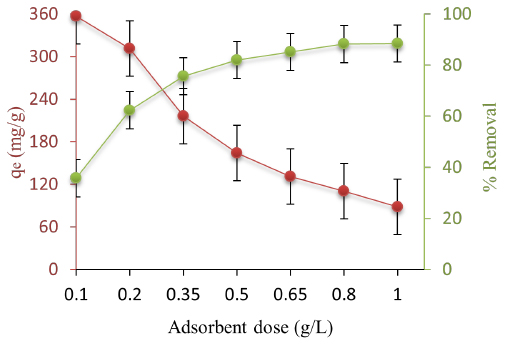
Figure 3:
Effect of AC/Fe3O4 dose on the removal of CIP (CIP concentration: 100 mg/L, time: 90 min, pH: 5 and tem; 25°C).
The adsorption isotherm models which have been utilized for assessing the results of CIP adsorption onto AC/Fe3O4 were different models (Table 1). Accordingly, the Langmuir model was found to be the most useful model for analysis of the adsorption data; based on this, the occurrence of monolayer adsorption on the particular homogenous sites of the AC/Fe3O4 surface is confirmed.
Models | Langmuir = × + | Models | Temkin qe = A + B Ln Ce | |||||||||
---|---|---|---|---|---|---|---|---|---|---|---|---|
Tem | 288 K | 298 K | 308 K | 318 K | Tem | 288 K | 298 K | 308 K | 318 K | |||
qm | 117.9 | 132.4 | 141.2 | 156.2 | A | 6.11 | 7.23 | 9.16 | 11.2 | |||
R2 | 0.995 | 0.996 | 0.999 | 0.997 | B | 0.72 | 0.83 | 0.93 | 1.05 | |||
RL | 0.421 | 0.654 | 0.711 | 0.652 | R2 | 0.652 | 0.784 | 0.789 | 0.821 | |||
Models | Freundlich Ln qe = Ln Ce + Ln KF | Models | D-R Ln qe = Ln qm– Kε2 | |||||||||
KF | 2.45 | 3.12 | 3.68 | 4.58 | qm | 44.5 | 56.2 | 71.3 | 92.1 | |||
1/n | 0.28 | 0.45 | 0.61 | 0.73 | E | 1.47 | 3.24 | 2.19 | 5.75 | |||
R2 | 0.897 | 0.842 | 0.854 | 0.931 | R2 | 0.765 | 0.873 | 0.832 | 0.798 |
In Table 2, equations related to kinetic models and results obtained from their application are brought. PSO was introduced as a superior model compared to other employed models, including pseudo-first-order and IPD, when their correlation coefficients were compared with each other. Besides, the closeness could be detected between the calculated equilibrium capacity value (qe, cal=156.2 mg/g) using the PSO and the corresponding experimental one (qe, exp), which approves the more applicability of this model compared to other models.
Model | Parameters | 10 mg/L | 25 mg/L | 50 mg/L | 75 mg/L | 100 mg/L |
---|---|---|---|---|---|---|
Qe exp | 11.2 | 31.5 | 64.2 | 95.5 | 117.4 | |
PFO Log (qe – qt) = log qe – t | qe cal | 6.14 | 17.4 | 31.5 | 53.2 | 71.4 |
K1 | 0.051 | 0.061 | 0.065 | 0.069 | 0.071 | |
R2 | 0.893 | 0.886 | 0.854 | 0.814 | 0.902 | |
PSO = + | qe cal | 11.6 | 29.4 | 66.8 | 98.2 | 120.4 |
K2 | 0.009 | 0.007 | 0.004 | 0.002 | 0.001 | |
R2 | 0.997 | 0.994 | 0.996 | 0.994 | 0.996 | |
IPD qt = K t1/2 + C | K | 4.02 | 5.32 | 7.45 | 115 | 13.4 |
C | 1.19 | 1.34 | 3.02 | 3.11 | 4.23 | |
R2 | 0.902 | 0.913 | 0.918 | 0.924 | 0.934 |
DISCUSSION
CIP adsorption might be negatively and/or positively affected by changes in contact time. As can be detected in the related Figure 1, the contact of CIP and adsorbent in the upward part of the plot has resulted in operative adsorption since there are enough unsaturated active sites yet.25 After establishing equilibrium between the sorption sites and CIP molecules, there is no significant effect on adsorption due to additional reaction time; this is ascribed to saturation of the sorption sites during contact time and lack of more unoccupied space for additional CIP adsorption.26,27
The pH of the solution is a factor that effectively governs adsorption effectiveness because it has a role in the determination of the surface charge of the adsorbent and adsorbate. It represents the importance of taking into consideration of the deprotonation (or protonation) of CIP.28 Due to effects associated with pH, the ionization degree, the adsorbent surface charge, and the molecular structures of the adsorbates can be changed; thus, it is effective in determination of the kind of interaction between the adsorbent and CIP, which occurs due to the ionization of species in the solution.29,30
The initial concentration of pollutant, i.e., CIP in the present study, is another effective factor in adsorption, and its effects should be evaluated. Previous reports have been indicative of discernible effects of this parameter up to a certain limit. Furthermore, the willingness of the adsorbent to adsorb CIP molecules has been dependent on the accessible sorption sites on the adsorbent’s surface.31 For values higher than the optimum value, a saturation of active sites on the adsorbent surface results in a decline in adsorption efficiency. Moreover, there is limited vacant space between the layers of AC/Fe3O4, and the adsorption process is carried out based on replacing interlayer anions of the adsorbent with contaminants. Therefore, this space has the capacity for accepting the optimum AC/Fe3O4 values and participating in efficient adsorption. Accordingly, a saturation of active sites of the interlayer of AC/Fe3O4 led to stopping further diffusion of contaminants onto the adsorbent.32
KL is indicative of the Langmuir constant. The homogeneous adsorption and single-layer coverage of the AC/Fe3O4 surface by CIP without any interaction between molecules is the assumption represented by the Langmuir model. Freundlich isotherm, as an experimental model, offers surface heterogeneity and an exponential distribution of the sorption sites of adsorbent and energy corresponding to them; this is a reversible adsorption model and is not restricted to monolayer formation.33
The mean separation factors (RL) obtained by the equation RL = 1 / 1+(kL C0) are between zero and one for all temperatures, which indicates that the adsorption processes are favorable. The n values obtained from the Freundlich model for all temperatures are between 1 and 10, indicating that CIP molecules were favorably adsorbed on AC/Fe3O4 adsorbent. The values of b (Temkin adsorption heat) in Table 1 indicates the amount of heat that the adsorbate needs to be adsorbed on the adsorbent surface.34–36Therefore, the adsorption of CIP on AC/Fe3O4 requires more heat, which will be discussed in the section of the thermodynamics of adsorption. The values of the mean adsorption energy parameter (E) obtained from the D-R isotherm model, are less than 8 kJ/ mol in all temperatures, which indicate that all of the adsorption processes are physical.37–38
Generally, electrostatic attraction, π–π stacking, hydrogen bonding, base-acid interactions, Van der Waals forces, and hydrophobic contact are involved in the adsorption of water contaminants on adsorbents. The main driving force of adsorption is the replacement of pollutants with the interlayer anion of AC/Fe3O4. Based on this, the adsorption phenomenon is assisted by various interactions. During the CIP removal process, the interaction between π-conjugated electrons and positively charged AC/Fe3O4 as π-cation interaction is possible to occur.
Since there is no π-conjugation plane in AC/Fe3O4, the π-π stacking interaction between them is ruled out.39 The adsorption process is progressed at alkaline pH, and electrostatic interaction with CIP AC/Fe3O4 can be occurred due to partial deprotonation of the AC/Fe3O4, which is led to an acceleration of the studied process.36 In the acidic pH, the CIP adsorption is decreased due to partial protonation of CIP; this diminishes its electrostatic attraction with the adsorbent. In this case, the formation of π-cation interaction is detected between CIP and AC/Fe3O4 due to the presence of π electrons density. Furthermore, bonding hydrogen with hydroxides of AC/Fe3O4 can be carried out in the case of the presence of OH groups.40
CONCLUSION
To sum up, effective AC/Fe3O4 for elimination of CIP molecules from water were prepared via chemical activation. The optimum conditions for removal efficiency of CIP onto AC/ Fe3O4 nanocomposite were obtained at CIP concentration of 25 mg/L, AC/Fe3O4 nanocomposite dose of 0.08 g, solution pH of 5, equilibrium time of 90 min, and temperature of 25°C, giving the highest percentage of CIP removal of 100%. The isotherm and kinetic of CIP molecules adsorption onto nanocomposite follow the Langmuir and PSO models which suggests that the complexation chemisorption system is anticipated in the adsorption processes and AC/Fe3O4 nanocomposite can be swiftly and effortlessly isolated utilizing a magnet. High CIP adsorption by the AC/Fe3O4 suggest that AC/Fe3O4 have excellent utility for practical applications.
Cite this article
Zisti F, Chandrika K, Balarak D. Effective Adsorption of Ciprofloxacin Antibiotic Using of Novel Magnetic F?3O4/Activated Carbon Nanocomposite from Aqueous Solution. Int. J. Pharm. Investigation. 2023;13(4):778-83.
ACKNOWLEDGEMENT
The authors are grateful to Zahedan University of Medical Sciences, because of supporting this research (IR.ZAUMS.REC.1401.147).
ABBREVIATIONS
AC/Fe3O4 | Magnetic Activated carbon nanocomposite |
---|---|
CIP | Ciprofloxacin |
D-R | Dubinin-Radushkevich |
PSO | Pseudo-second-order kinetic |
IPD | Intra particle diffusion |
References
- Moein H, Balarak D, Meshkinain A, Chandrika K, Yazdani N. Effects of operational parameters on the removal of tetracycline from aqueous solutions by electrocoagulation. Int J Pharm Investig. 2021;11(1):23-6. [CrossRef] | [Google Scholar]
- Mahvi AH, Mostafapour FK. Biosorption of tetracycline from aqueous solution by : equilibrium kinetic and thermodynamics studies. Fresenius Environ Bull. 2018;27(8):5759-67. [CrossRef] | [Google Scholar]
- Lv JM, Ma YL, Chang X, Fan SB. Removal and removing mechanism of tetracycline residue from aqueous solution by using Cu-13X. Chem Eng J. 2015;273:247-53. [CrossRef] | [Google Scholar]
- Rostamian R, Behnejad H. A comparative adsorption study of sulfamethoxazole onto graphene and graphene oxide nanosheets through equilibrium, kinetic and thermodynamic modeling. Process Saf Environ Prot. 2016;102:20-9. [CrossRef] | [Google Scholar]
- Chen H, Gao B, Li H. Removal of sulfamethoxazole and ciprofloxacin from aqueous solutions by graphene oxide. J Hazard Mater. 2015;282:201-7. [PubMed] | [CrossRef] | [Google Scholar]
- Peng X, Hu F, Dai H, Xiong Q, Xu C. Study of the adsorption mechanisms of ciprofloxacin antibiotics onto graphitic ordered mesoporous carbons. J Taiwan Inst Chem Eng. 2016;65:472-81. [CrossRef] | [Google Scholar]
- Ahmadi S, Banach A, Mostafapour FK, Balarak D. Study survey of cupric oxide nanoparticles in removal efficiency of ciprofloxacin antibiotic from aqueous solution: adsorption isotherm study. Desalin Water Treat. 2017;89:297-303. [CrossRef] | [Google Scholar]
- Cao J, Xiong Z, Lai B. Effect of initial pH on the tetracycline (TC) removal by zero-valent iron: adsorption, oxidation and reduction. Chem Eng J. 2018;343:492-9. [CrossRef] | [Google Scholar]
- Acosta R, Fierro V, Martinez de Yuso AM, Nabarlatz D, Celzard A. Tetracycline adsorption onto activated carbons produced by KOH activation of tyre pyrolysis char. Chemosphere. 2016;149:168-76. [PubMed] | [CrossRef] | [Google Scholar]
- Peterson JW, Petrasky LJ, Seymour MD, Burkhart RS, Schuiling AB. Adsorption and breakdown of penicillin antibiotic in the presence of titanium oxide nanoparticles in water. Chemosphere. 2012;87(8):911-17. [PubMed] | [CrossRef] | [Google Scholar]
- Darweesh TM, Ahmed MJ. Adsorption of ciprofloxacin and norfloxacin from aqueous solution onto granular activated carbon in fixed bed column. Ecotoxicol Environ Saf. 2017;138:139-45. [PubMed] | [CrossRef] | [Google Scholar]
- Balarak D, Khatibi AD, Chandrika K. Antibiotics removal from aqueous solution and pharmaceutical wastewater by adsorption process: a review. Int J Pharm Investig. 2020;10(2):106-11. [CrossRef] | [Google Scholar]
- Kerkez-Kuyumcu Ö, Bayazit ŞS, Salam MA. Antibiotic amoxicillin removal from aqueous solution using magnetically modified graphene nanoplatelets. J Ind Eng Chem. 2016;36:198-205. [CrossRef] | [Google Scholar]
- Mostafapour FK, Haseeb S, Balarak D, Moein H, Sajadi AA, Jalalzaei Z, et al. Thermodynamic study of amoxicillin and naphthalene adsorption on activated carbon derived from Salvadora persica. Int J Pharm Investig. 2021;11(1):41-5. [CrossRef] | [Google Scholar]
- Li M, Liu Y, Liu S, Shu D, Zeng G, Hu X, et al. Cu(II)-influenced adsorption of ciprofloxacin from aqueous solutions by magnetic graphene oxide/nitrilotriacetic acid nanocomposite: competition and enhancement mechanisms. Chem Eng J. 2017;319:219-28. [CrossRef] | [Google Scholar]
- Peng H, Pan B, Wu M, Liu Y, Zhang D, Xing B, et al. Adsorption of ofloxacin and norfloxacin on carbon nanotubes: hydrophobicity- and structure-controlled process. J Hazard Mater. 2012:233-234. 89-96 [PubMed] | [CrossRef] | [Google Scholar]
- Han H, Cui Y, Gao R, Huang Y, Luo Y, Shen S, et al. Study on nitrogen removal from rice paddy field drainage by interaction of plant species and hydraulic conditions in eco-ditches. Environ Sci Pollut Res Int. 2019;26(7):6492-502. [PubMed] | [CrossRef] | [Google Scholar]
- Sarker N, Fakhruddin ANM. Removal of phenol from aqueous solution using rice straw as adsorbent. Appl Water Sci. 2017;7(3):1459-65. [CrossRef] | [Google Scholar]
- Asif Z, Chen Z. Removal of arsenic from drinking water using rice husk. Appl Water Sci. 2017;7(3):1449-58. [CrossRef] | [Google Scholar]
- Filipinas JQ, Rivera KKP, Ong DC, Pingul-Ong SMB, Abarca RRM, de Luna MDG, et al. Removal of sodium diclofenac from aqueous solutions by rice hull biochar. Biochar. 2021;3(2):189-200. [CrossRef] | [Google Scholar]
- Li G, Zhang D, Wang M, Huang J, Huang L. Preparation of activated carbons from Iris tectorum employing ferric nitrate as dopant for removal of tetracycline from aqueous solutions. Ecotoxicol Environ Saf. 2013;98:273-82. [PubMed] | [CrossRef] | [Google Scholar]
- Balarak D, Ganji F, Chandrika K, Haseeb S. Montmorillonite nanoparticles effectiveness in removal of amoxicillin from water solutions. Int J Pharm Investig. 2020;10(2):122-6. [CrossRef] | [Google Scholar]
- Ji L, Chen W, Duan L, Zhu D. Mechanisms for strong adsorption of tetracycline to carbon nanotubes: a comparative study using activated carbon and graphite as adsorbents. Environ Sci Technol. 2009;43(7):2322-7. [PubMed] | [CrossRef] | [Google Scholar]
- Marzbali MH, Esmaieli M, Abolghasemi H, Marzbali MH. Tetracycline adsorption by H3PO4-activated carbon produced from apricot nut shells: A batch study. Process Saf Environ Prot. 2016;102:700-9. [CrossRef] | [Google Scholar]
- Balarak D, Chandrika K. Batch Studies on biosorption of ciprofloxacin on Freshwater Macro alga Lemna minor. Int J Pharm Investig. 2019;9(3):117-21. [CrossRef] | [Google Scholar]
- Ahmed MB, Zhou JL, Ngo HH, Guo W. Adsorptive removal of antibiotics from water and wastewater: progress and challenges. Sci Total Environ. 2015;532:112-26. [PubMed] | [CrossRef] | [Google Scholar]
- Zhang Y, Jiao Z, Hu Y, Lv S, Fan H, Zeng Y, et al. Removal of tetracycline and oxytetracycline from water by magnetic Fe3O4@graphene. Environ Sci Pollut Res Int. 2017;24(3):2987-95. [PubMed] | [CrossRef] | [Google Scholar]
- Ji L, Shao Y, Xu Z, Zheng S, Zhu D. Adsorption of monoaromatic compounds and pharmaceutical antibiotics on carbon nanotubes activated by KOH etching. Environ Sci Technol. 2010;44(16):6429-36. [PubMed] | [CrossRef] | [Google Scholar]
- Li S, Zhang X, Huang Y. Zeolitic imidazolate framework-8 derived nanoporous carbon as an effective and recyclable adsorbent for removal of ciprofloxacin antibiotics from water. J Hazard Mater. 2017;321:711-19. [PubMed] | [CrossRef] | [Google Scholar]
- Wang F, Yang B, Wang H, Song Q, Tan F, Cao Y, et al. Removal of ciprofloxacin from aqueous solution by a magnetic chitosan grafted graphene oxide composite. J Mol Liq. 2016;222:188-94. [CrossRef] | [Google Scholar]
- Zha Y, Zhou Y, Jin X, Chen Z. The removal of amoxicillin from wastewater using organobentonite. J Environ Manage. 2013;129:569-76. [PubMed] | [CrossRef] | [Google Scholar]
- Guler UA, Sarioglu M. Removal of tetracycline from wastewater using pumice stone: equilibrium, kinetic and thermodynamic studies. J Environ Health Sci Eng. 2014;12:79 [PubMed] | [CrossRef] | [Google Scholar]
- Azarpira H, Mahdavi Y, Khaleghi O. Thermodynamic studies on the removal of metronidazole antibiotic by multi-walled carbon nanotubes. Pharm Lett. 2016;8(11):107-13. [PubMed] | [CrossRef] | [Google Scholar]
- Yu F, Li Y, Han S, Ma J. Adsorptive removal of antibiotics from aqueous solution using carbon Materials. Chemosphere. 2016;153:365-85. [PubMed] | [CrossRef] | [Google Scholar]
- Mostafapour FK, Bazi M, Siddiqui SH, Bagheri H, Balarak D. Highly efficient adsorption and removal of amoxicillin from aqueous solution by magnetic graphene oxide nanocomposite. Int J Pharm Investig. 2021;11(4):384-8. [CrossRef] | [Google Scholar]
- Bazi M, Balarak D, Khatibi AD, Siddiqui SH, Mostafapour FK. Investigation of isotherm, kinetics and thermodynamics of ciprofloxacin adsorption by molecularly imprinted polymer from aqueous solutions. Int J Pharm Investig. 2021;11(3):269-73. [CrossRef] | [Google Scholar]
- Balarak D, Baniasadi M, Bazzi M. Adsorption equilibrium and thermodynamic studies of ciprofloxacin from aqueous solutions by magnetic bentonite nanocomposites. Int J Pharm Investig. 2020;10(3):339-43. [CrossRef] | [Google Scholar]
- Zisti F, Alizadeh R, Azhdari Tehrani AZ, Morsali A, Eichhorn SH, Rawson JM, et al. Synthesis, characterization and single crystal X-ray analysis of Zn(II) phenanthridine complexes. J Mol Struct. 2019;1181:579-86. [CrossRef] | [Google Scholar]
- Zisti F, Eichhorn SH, Alizadeh R, Tehrani AA, Morsali A, Rawson JM, et al. Single crystals and nanoparticles of Zn(II) supramolecular compounds via sonochemical method: synthesis, characterization and structural studies. Inorg Chim Acta. 2019;496:118995 [CrossRef] | [Google Scholar]
- Zisti F, Tehrani AA, Alizadeh R, Abbasi H, Morsali A, Eichhorn SH, et al. Synthesis and structural characterization of three nano-structured Ag(I) coordination polymers; Syntheses, characterization and X-ray crystal structural analysis. J Solid State Chem. 2019;271:29-39. [CrossRef] | [Google Scholar]