Contents
- ABSTRACT
- INTRODUCTION
- STRUCTURE OF PP2A
- REGULATIONS OF PP2A IN CONTROLLING ALZHEIMER’S DISEASE
- DRUGS USED FOR THE ACTIVATION OF PP2A IN CONTROLLING ALZHEIMER’S
- CERAMIDES
- SPHINGOSIDES
- FOLATE AND XYLULOSE 5 PHOSPHATE
- SODIUM SELENATE
- ADAMANTANE DERIVATIVES
- PHENOLIC ANTIOXIDANTS
- PP2A MODULATORS ISOQUINOLONES AND TETRALONES
- FUTURE PROSPECTS
- CONCLUSION
- ACKNOWLEDGEMENT
- ABBREVIATIONS
- References
ABSTRACT
ABSTRACT
Alzheimer’s disease is a progressive brain condition. It is characterized by alterations in the brain that result in the deposition of certain proteins. The brain shrinks and finally dies as a result of Alzheimer’s disease. Over the years there has been very concerned efforts to control Alzheimer’s disease with strategies like vaccinations but all off them failed in phase three trial. This leads to exploration of new strategy like activation of Protein Phosphatase 2A (PP2A) to control the tau phosphorylation in Alzheimer’s. In this review, a comprehensive review regarding activation of PP2A using drugs like metformin, sphingosides, ceramides, folate and xylulose 5 phosphate, Sodium selenate, Adamantane derivatives, phenolic derivatives, Isoquinolones and Tetralones. Therefore, this approach can offer a fresh glimmer of hope for the treatment of Alzheimer’s disease.
INTRODUCTION
Alzheimer’s Disease (AD) is the highly prevalent type of dementia, according to medical literature. The condition, which gets worse over time and eventually results in death, has no known total cure. Alois Alzheimer, a German psychiatric and neuropathologist, first described it in 1906, and he is the inspiration for its name. There are a number of theories as to what causes this condition, one of the oldest of which is the cholinergic hypothesis,1 according to which the development of AD is sped up by a deficiency in cholinergic signalling. The isoprenoid hypothesis demonstrated isoprenoid changes in AD,2 which are distinct from the symptoms seen in normal ageing, which is why it is referred to as premature ageing. The amyloid hypothesis3,4 describes the formation of aggregated amyloid fibrils, which are one of the toxic forms of protein. The tau hypothesis is one of the most recent hypotheses in which amyloid plaques are formed that have no relation to neuron loss,5 and tau is the main causative factor of this hypothesis. The loss of microtubule-stabilizing tau protein, which leads to cytoskeleton degradation, has been proposed as a mechanism for neurotoxicity. Current treatments only address the disease’s symptoms. There are no treatments available that can stop or reverse the progression of the disease. The number of people who will have the condition by 2050 will be astonishing-100 million. As of 2022, more than 1,000 clinical studies testing various drugs for Alzheimer’s disease have been completed or are ongoing. In both mild and moderate Alzheimer’s disease, acetyl cholinesterase inhibitors like donepezil, rivastigmine, and galantamine offer momentary relief, while memantine, a low-affinity N-methyl D-aspartate receptor antagonist, is permitted for use in moderate-to-severe Alzheimer’s disease.6 Huperzine A has also attracted the attention of US medical researchers. It can also be used to treat diseases characterised by neurodegeneration. As a result, Huperzine A has been discovered to be an inhibitor of the enzyme acetylcholinesterase.7 Though some of them are currently undergoing clinical trials for Alzheimer’s disease, one strategy has been used to develop vaccination therapies, and the field of Alzheimer’s research has recently been shaken by three phase failures. Phase 3 clinical trials for vaccine development and grounds for their rejection included: a) Semagecestat (LY450139), a gamma secretase inhibitor that prevents the generation of the A Beta peptide, failed to meet its key end goals in 2011. Bapineuzumab (Pfizer/ Janssen Alzheimer Immunotherapy) and solanezumab, two anti-Abeta monoclonal antibodies that were being investigated in phase 3 studies, failed to achieve their key end goals, which were an improvement in cognition and daily living activities. c) The failures of Tarenflurbil Phase 3 in 2008 and Tramiprosate Phase 3 in 2007.8 The development of above amyloidcentric vaccines based on the amyloid cascade hypothesis failed in phase three trials. This failure of vaccination therapy suggests that we might be able to treat Alzheimer’s disease by activating PP2A. (Protein Phosphatase 2A enzyme). Because a very low level of this enzyme was sufficient to cause Alzheimer’s disease, researchers focused on activating this enzyme or inhibiting the generation of PP2A inhibitors by new chemical entities, which became the new trends in Alzheimer’s drug discovery.
PP2A as Emerging Target
While the pharmaceutical industry has invested a lot of time and energy in the search for and development of anti-amyloid agents, little has been done to pinpoint candidates that could be turned into drugs to inhibit tau protein phosphorylation. Due to the drawbacks of AChEI (Acetyl Choline Esterase Inhibitor) and vaccine therapy in the course of illness, a novel and appealing strategy was developed by boosting PP2A activity. By boosting and inhibiting the enzymes that controlled the phosphorylation and dephosphorylation of the tau protein, phosphorylation of the tau protein could be decreased. As a result, a new strategy for developing PP2A agonists to control tau phosphorylation in Alzheimer’s disease has been discovered.9
STRUCTURE OF PP2A
Protein Phosphatase 2 (PP2), sometimes referred to as PP2A, is an enzyme that is produced in humans by the PPP2CA gene.10 A commonly present and conserved serine/threonine phosphatase with a wide range of biological uses is the PP2A heterotrimeric protein phosphatase (Figure 1). The structural A subunit, the regulatory B subunit, and the catalytic C subunit are the three subunits that make up the dimeric core enzyme PP2A. There are multiple different kinds of holoenzymes with unique roles and characteristics that are created when the PP2A catalytic C subunit connects with the A and B subunits. The A subunit (Huntington-elongation-A subunit-TOR), a founding member of the HEAT repeat protein family, serves as the scaffold necessary for the assembly of the heterotrimeric complex (Table 1).11 Even if the B subunit is absent, the enzymatic activity of the catalytic subunit is completely altered when the A subunit binds. Even though the C and A subunits are in order, there is a remarkable amount of variation between eukaryotes. The more varied regulatory B subunits have been found to be essential in regulating the location and particular activity of different holoenzymes.12
Units | Gene | Description | Functions |
---|---|---|---|
Structural subunit A | PPP2R1A | Regulatory subunit A alpha isoform with atomic mass of 65kDa. | Serves as a scaffolding molecule to coordinate the assembly of the catalytic subunit and negative control of cell growth and division. |
PPP2R1B | Regulatory subunit A beta isoform with atomic mass of 65kDa. | This gene encodes a beta isoform of the constant regulatory subunit A. Defects in this gene could be the cause of some lung and colon cancers. | |
PPP2R2B | regulatory subunit B beta isoform with atomic mass of 55kDa. | It modulates substrate selectivity and catalytic activity. Defects in the 5’ UTR of this gene may cause a rare form of autosomal dominant spinocerebellar ataxia. | |
Regulatory subunit B | PPP2R2C | regulatory subunit B gamma isoform with atomic mass of 55kDa. | It may modulate the catalytic activity and substrate selectivity. It is also implicated in the negative control of cell growth. |
PPP2R2D | Regulatory subunit B delta isoform with atomic mass of 55kDa. | The activity of PP2A complexes containing PPP2R2D (PR55-delta) fluctuate during the cell cycle: the activity is high in interphase and low in mitosis. | |
Catatytic subunit C | PPP2R3A | Regulatory subunit B” subunit alpha with 70/130 kDa. | The regulatory subunit is encoded by a diverse set of genes that have been grouped into the B/PR55, B’/PR61, and B’’/ PR72 families. Alternative splicing results in multiple transcript variants encoding different isoforms. |
PPP2R3B | Regulatory subunit B’’ subunit beta with atomic mass 48 kDa. | The product of this gene belongs to the beta subfamily of regulatory subunit B’’. Alternative splicing results in multiple transcript variants encoding different isoforms. | |
PPP2R3C | Regulatory subunit B’’ subunit gamma. | that catalyze the removal of phosphate groups from serine and/ or threonine residues by the hydrolysis of phosphoric acid monoesters. They directly oppose the actions of kinases and phosphorylases and therefore play an integral role in many signal transduction pathways. | |
PPP2R4 | PP2A regulatory subunit B | The regulatory subunit is encoded by set of genes that have been grouped into the B/PR55, B’/PR61, and B/PR72 families. it shows distinct enzymatic specificities and intracellular localizations to the holozenzyme. | |
PPP2R5A | Regulatory subunit alpha isoform with atomic mass 56kDa. | The regulatory subunit might modulate substrate selectivity and catalytic activity. This gene encodes an alpha isoform of the regulatory subunit B56 subfamily. | |
PPP2R5B | Regulatory subunit beta isoform with atomic mass 56 kDa. | The B regulatory subunit improve substrate selectivity and catalytic activity. This gene encodes a beta isoform of the regulatory subunit B56 subfamily. | |
PPP2R5C | Regulatory subunit gama isoform with atomic mass 56 kDa. | This gene encodes a gamma isoform of the regulatory subunit B56 subfamily. The B regulatory subunit modulate substrate selectivity and catalytic activity. | |
PPP2R5D | Regulatory subunit delta isoform with atomic mass 56 kDa. | It is involved in the negative control of cell growth and division and improve substrate selectivity. | |
PPP2R5E | Regulatory subunit epsilon isoform with atomic mass 56kDa. | This gene encodes an epsilon isoform of the regulatory subunit B56 subfamily and it modulates catalytic activity. | |
PPP2CA | Serine/threonine-protein phosphatase 2A catalytic subunit alpha isoform. | ||
PPP2CB | Catalytic subunit beta isoform. |
REGULATIONS OF PP2A IN CONTROLLING ALZHEIMER’S DISEASE
The treatment of Alzheimer’s disease and related studies clearly linked PP2A to various levels of Alzheimer’s disease (Figure 2). We can control Alzheimer’s disease by lowering mRNA expression and encoding the PP2A catalytic subunit. A protein called Inhibitors 2 (I2) that binds to and inhibits PP2A is highly expressed in Alzheimer’s brain.13–15 According to a number of biochemical studies, the abilities of various PP2As preparations immunoprecipitated from human brain, which were used to dephosphorylate the hyperphosphorylated tau, and thus phosphoprotein tau phosphatase appears to play a significant role.16 As a result, decreased PP2A activity is a major contributor to increased tau phosphorylation and NFT (Neurofibrillary Tangles) pathology.17
When the activity of the PP2A enzyme is reduced, the levels of protein phosphorylation rise. Folate deficiency worsens Alzheimer’s symptoms and increases tau phosphorylation. The hyperphosphorylated tau in NFTs is the most visible evidence of this. Along with tau phosphatase activity, PP2A also dephosphorylates and inactivates kinases18 that phosphorylate tau.19 PP2A controls the formation of amyloid plaques, the main cause of Alzheimer’s disease. The production of Amyloid (A) from Amyloid Precursor Protein (APP) via secretases is modulated by phosphorylation at APP-Thr-668,20 at least in part via JNK (c-Jun N-terminal Kinases).21 Through decreased PP2A activity towards phospho-APP or indirectly through decreased phosphatase activity towards phospho-JNK, increased APP phosphorylation can lead to increase Amylose (A) production and, ultimately, the formation of amyloid plaques. Alzheimer’s disease’s last stages are marked by neuronal cell loss. In neuroblastoma cell lines, decreased PP2A methylation has been found to cause death, and PP2A deficiency results in kinase activation, which in turn causes tau hyperphosphorylation, which promotes NFT production and microtubule disruption. Alzheimer’s disease is brought on by NFTs, amyloid plaques, phosphotylated tau, phosphorylated phosphoprotein, disordered microtubules, and other factors.22,23
DRUGS USED FOR THE ACTIVATION OF PP2A IN CONTROLLING ALZHEIMER’S
Metformin as MID1-α4 (Midline-1 Alpha Four) protein complex inhibitor
Metformin inhibits tau phosphorylation by inhibiting the protein phosphatase 2A enzyme. Metformin increases PP2A activity and decreases tau phosphorylation.24 Okadic acid and fostreicin, two PP2A inhibitors, totally limit this tau dephosphorylating ability, demonstrating that PP2A is a key mediator of the observed effects. According to Kickstein et al., the effects of metformin on PP2A activity and tau phosphorylation appear to be unrelated to AMPK (5’ AMP-activated protein kinase) activation. Tau phosphorylation is unaffected by AICAR (5-aminoimidazole-4 -carboxyamide ribo nucleotide), the AMPK activator, while metformin decreases AMPK activity while increasing PP2A activity. Finally, research using affinity chromatography and immunoprecipitation demonstrates that metformin prevents the catalytic subunit of PP2A (PP2Ac) from joining the so-called MID1-4 protein complex. The complex that develops controls PP2Ac degradation and affects PP2A activity.25
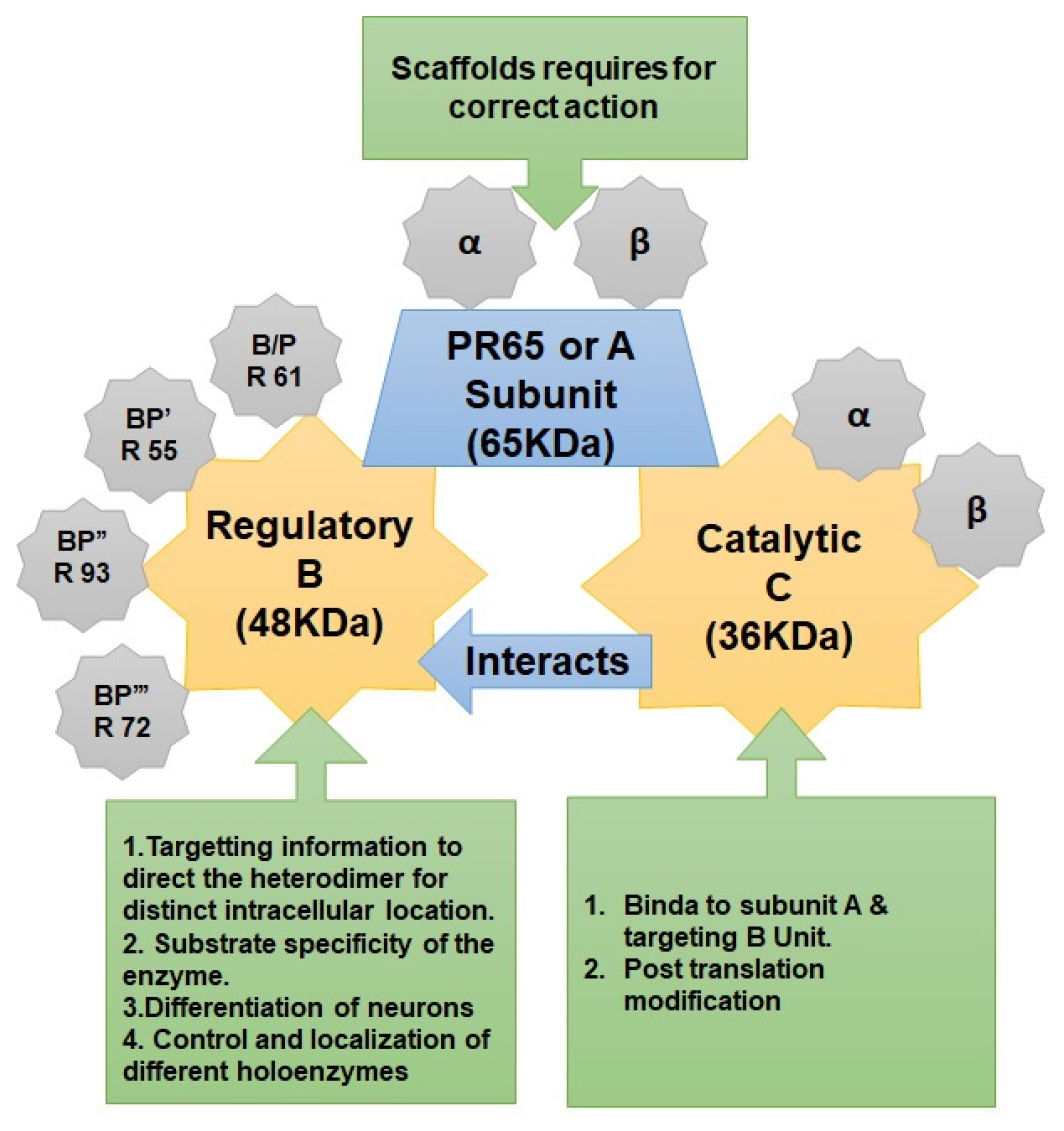
Figure 1:
Structural features of PP2A.
CERAMIDES
This is the waxy lipid molecule family. Ceramides are made up of sphingosine and a fatty acid. These can only be found in high concentrations within the cell membranes.26 Dobronsky et al. demonstrate that PP2A and CAPP (Ceramide Actived Protein Phosphate) have similar properties in T9 glioma cells and rat brain, leading to the hypothesis that ceramide has direct catalytic activity on PP2A. C-2 ceramides can increase PP2A activity by up to 3.5 times. Actually, PP2A requires a free B subunit for ceramide activation, and ceramides containing hexanoyl, decanoyl, and myrestoyl improve PP2A activity.27,28
SPHINGOSIDES
These lipids have sphingoid bases as their backbone, a class of aliphatic amino alcohols that also contains sphingosine. They were named after the mythical sphinx due to their riddle after being found in brain samples in the 1870s. Unexpectedly, medications such FTY720 (Fingolimod), 1, 9-dideoxy-forskolin, and forskolin activate PP2A. The Indian Coleus (Coleus forskohlii) plant produces the labdane diterpene forskolin, often known as coleonol.29,30 Forskolin displays activation and overexpression of Protein Phosphatase-2A (PP-2A) when administered at 200mM, according to Qing Tian et al.’s study with the supplement, and it also shows promise in reducing the okadaic acid-induced PP-2A inhibition.31 Fingolimod was created chemically from the Immunosuppressive natural substance myriocin (ISP-I).32,33 Oaks J.J. et al. reported that FTY720 (2-amino-2-[2-(4-octylphenyl) ethyl]-1,3-propanediol hydrochloride, fingolimod, Gilenya) activated PP2A in PV model cell lines.
FOLATE AND XYLULOSE 5 PHOSPHATE
In numerous studies, E. Sontag and J.M. Sontag et al. have demonstrated that methylation of the PP2AC catalytic subunit directly affects the recruitments of specific B regulatory subunits to the PP2A heterodimeric complex, and thus would be expected to promote the dephosphorylation of its substrate.34
The methylation of the c-terminal leucine 309 promotes the assembly of the B, PP2ABC complex, which in turn promotes tau dephosphorylation. The rate-limiting step in this process is the regulation of the PP2AC catalytic subunit by S- Adenosine -L Methionine (SAM) dependent methyl transferase.
Increasing the levels of SAM precursors, such as Folate and especially methyl folate, promotes PP2AC methylation and the formation of a B-containing PP2A complex, which leads to increased tau dephosphorylation.35,36 M.A Davare et al. described xylulose 5 phosphate as another potential agent that enriches PP2A activation by increasing methylation of the PP2AC catalytic subunit. It is a glucose metabolic compound that recruits and activates PP2A via the glycolytic and lipolytic pathways.37
SODIUM SELENATE
Sodium Selenate regulates the function of the PP2A phosphatase protein, which is important in angiogenesis and has been linked to Alzheimer’s disease progression. Niall M. Corcoran et al. demonstrated that sodium selanate specifically activates PP2A phosphatase and dephosphorlates tau by demonstrating that sodium selate acts as a specific agonist for PP2A by improved phosphatase activity in transgenic TAU441 old mice. According to research, sodium selenate is an ideal PP2A stimulator with significant efficacy in inhibiting angiogenetic signalling.38
Niall M. Corcoran et al. discovered that sodium selenate reduces tau phosphorylation both in vitro and in vivo in their study. It has also been shown in clinical studies to improve contextual memory and motor performance while preventing neurodegeneration. Sodium selenate, in particular, reduces tau pathology in the PP2A phosphatase protein.27 The aforementioned information provides strong support for the use of sodium selenate in early clinical trials with patients who have Mild Cognitive Impairment (MCI) or mild Alzheimer’s Disease (AD).
ADAMANTANE DERIVATIVES
Memantine is the first drug in a new class of Alzheimer’s disease treatments. Eli Lilly and Company was the first to synthesize it in 1968. Memantine is marketed under the brand name. Memantine (Figure 3A) has been shown to have a minor effect in moderate-to-severe Alzheimer’s disease48 and Lewy body dementia.46,50 Despite years of study, there is little evidence of a benefit in mild Alzheimer’s disease.51
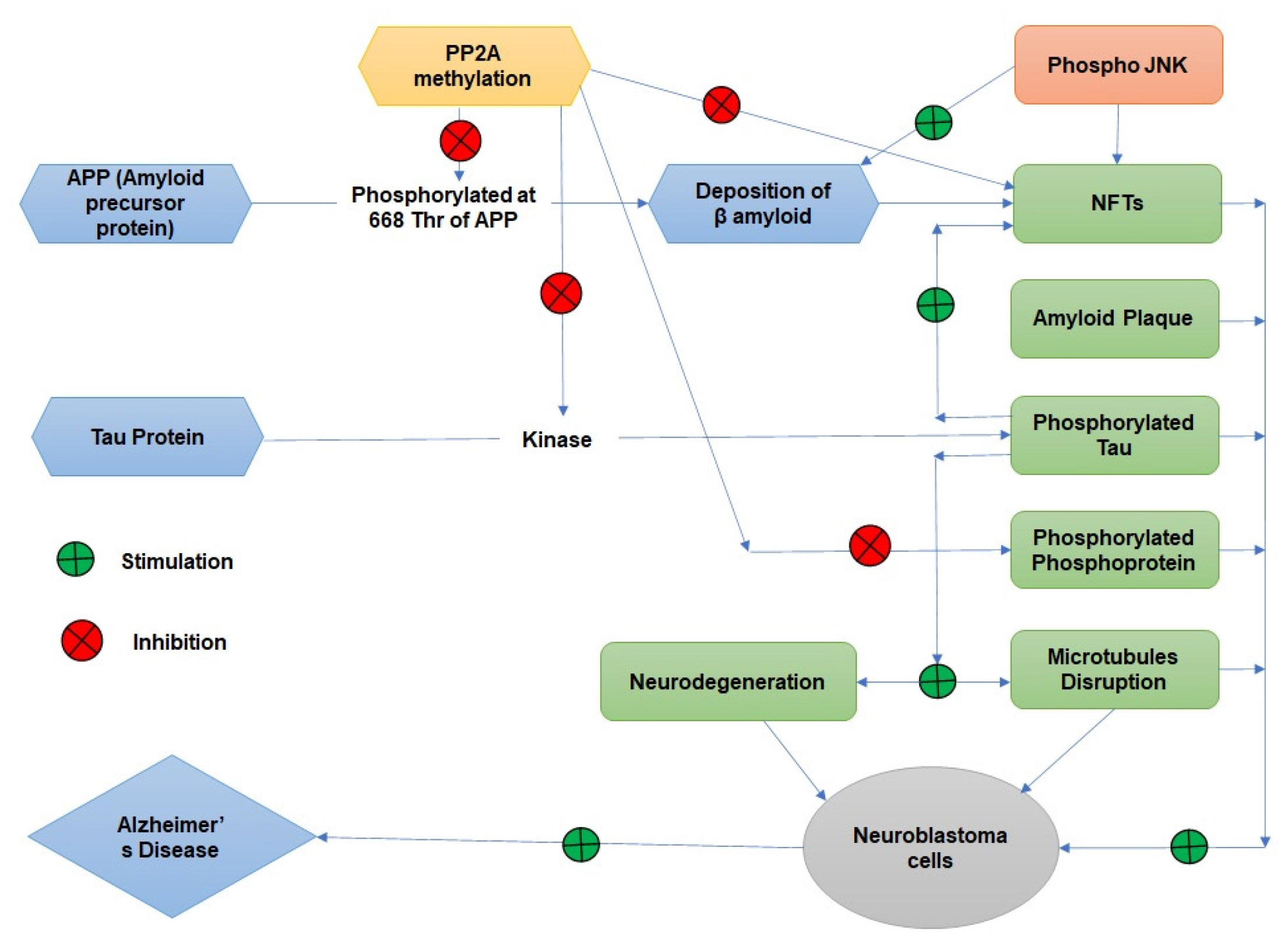
Figure 2:
Schematic representation of PP2A’s control over Alzheimer’s disease.
According to the study by Chan, S.F. et al., memantine, a low to moderate potency, noncompetitive inhibitor of glutamate gated NMDA receptor channels, is effective in improving functional outcomes in AD patients.
It has been demonstrated that the NMDA receptor and PP2A are in a complex, and activation of this receptor results in PP2A dissociation and a subsequent drop in PP2A activity.52
In another study, Muhammad Omar Chohan et al. discovered a novel mechanism in which intracellular PP2A activity is regulated by I2 PP2A, which also regulates tau phosphorylation and memantine modulates PP2A expression and inhibits neurodegeneration.53
According to Parsons CG et al., charged amino containing substitutions at R6 in aminoalkyl cyclohexane (Figure 3B) with methyl substitutions at R1, R2, and R5, at least one methyl or ethyl substitution at R3 and R4, and substitutions at R3 and R4 could be helpful therapeutics in a variety of CNS disorders linked to disruptions of glutamatergic neurotransmission.54
Adamantane group
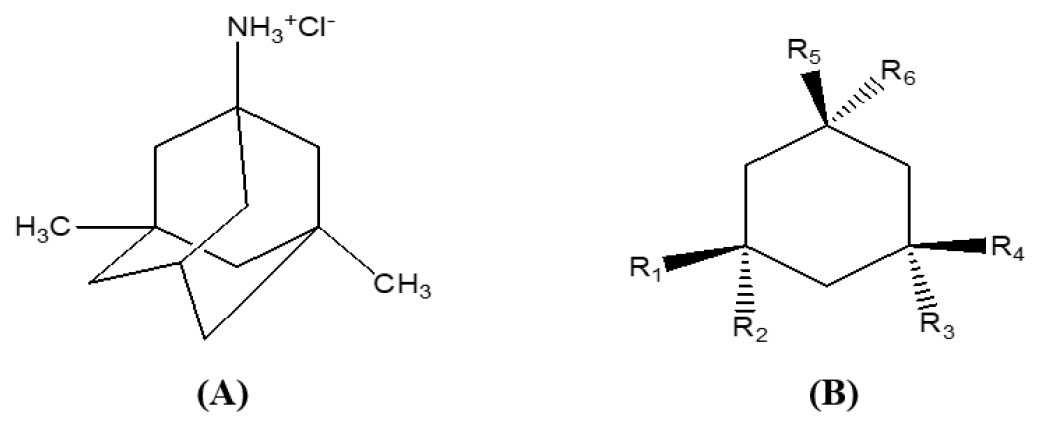
Figure 3:
A. Structure of Memantine Hydrochloride B. Basic structure of aminoalkyl cyclohexane.

Figure 4:
A. Isoquinolones and B. Tetralones.
PHENOLIC ANTIOXIDANTS
According to Roberta Ricciarelli et al., alpha tocopherol, a phenolic antioxidant, activates the enzyme PP2A via autophosphorylation of Protein Kinase C (PKC) inhibition. Because the inhibition of PKC by -tocopherol has been linked to its dephosphorylation, the in vitro activation of protein kinase 2A by -tocopherol suggests that this enzyme may be responsible for PKC dephosphorylation and subsequent deactivation.55
According to Guichard C et al., dihydroxyphenyl ethanol, a phenolic antioxidant derived from olive oil, has a promising role in activating Ser/Thr Phosphatase 2A. (PP2A). The structural features required for selective PP2A activation are the fewest in diydroxyphenyl ethanol.56
PP2A MODULATORS ISOQUINOLONES AND TETRALONES
Lorenzo M et al. demonstrate PP2A modulation activity by some isoquinoline and tetralone derivatives, which exhibit variable activity in the presence of 20 M C-2 ceramides. They also show that SAR studies on isoquinolones (Figure 4A) and tetralones (Figure 4B) show that when the bicyclic hydroxyl groups are methylated, the compounds appear to increase PP2A activity in the presence of C-2 ceramides.57 Based on the substituents of R1 and R2 positions, some compounds neither enhance nor inhibit appear to be neutral, and some compounds act as inhibitors of the enzyme under study.
In detail, in this class of compounds, when R1 and R2 are both substituted with ‘-H’ in both isoquinolones and tertalones, there is an inhibitor of PP2A in the presence of ceramides. However, substituting a methyl group for R1 and R2 increases PP2A activity. In the case of isoquinolones, the quinazoline ring with R1 and R2 as methyl substitutions and 3,5 di methoxy benzyl as R3 substitution had the highest increasing PP2A activity. The same inhibitor activity was discovered in the tetralone class with the substitution of ‘hydrogen’ at R1 and R2 positions. As we increase the substitution with methyl in both R1 and R2 position, and 2,6 2,5 3,5 di methoxy at R3 PP2A increasing activity was discovered, and the highest increasing activity of this class was discovered with 4- Pyridine N-oxide at R3 and methyl group at R1 and R2 position57 the different substitution and their PP2A modulation activity as shown by Lorenzo M et al. in Tables 2, 3 and 4.
Class | Drugs | Structure | MOA |
---|---|---|---|
Biguanide Antidibetic | Metformin | Interferes with the association of the catalytic subunit of PP2A (PP2Ac) to the so-called MID1-α4 protein complex.39 | |
Ceramides | D erythro C-18 ceramide, B C6 ceramide, C6 Pyridinium ceramide, C16-C18 endogeneous ceramide. | R= Alkyl portion of fatty acids. | Interact with I2PP2A to inhibit its overexpression.40 |
Cationic activators and methyl folates | Xylulose-5 phosphates. (1) Levomefolic acid (2). | Boosting the level of SAM precursors and enhancing the methylation of PP2AC catalytic subunit.41 | |
Selenium salt | Sodium Selenate. | Stimulation of PP2A that shows significant effectiveness is inhibiting angiogenetic signaling.42 | |
Sphingoids | FTY 720 (Fingolimod) (1) Forskolin (2) | Activate PP2A phosphate activity towards phosphorylase A, with selectivity over PP1 and inhibit PP2A demethylation.43 | |
Adamentane | Memantine | Improving PP2A activity acts through the inhibition of glutamate gated NMDA receptor channels.44 | |
Isoquinolones | 1,3 –bis (3,5 dimethoxy benzyl)-6,7 dimethoxy dihydroquinazolin -4 one. | The ability of the compounds to stabilize the interaction of ceramide with the multimeric PP2A enzyme.45 | |
Tetralones | 2-(3,5 dimethoxybenzylidine)-6,7-dimethoxy 3,4 dihydronaphthalene-1-one. | Ability of the compounds to stabilize the interaction of ceramide with the multimeric PP2A enzyme.46 | |
Phenolic antioxidents | Dihydroxy phenyl ethanol (1) Alpha Tocopherol (2). | Inhibition of PKCα that involved in phosphorylation of Tau.47 |
R1 = R2 | R3 | PP2A activity (μm/mL) |
---|---|---|
-H | 6.8 | |
-CH3 | – (CH2) COOH | 9.5 |
-H | 12.1 | |
-CH3 | -PO[N (CH3)] | 40.4 |
37.7 |
R1 = R2 | R3 | PP2A activity (μm/ mL) |
---|---|---|
-H | 3,5 di -OH | 1.2 |
-CH3 | 3,5 di -OH | 4.5 |
-H | 2,6 di- OH | 6.5 |
-H | 3,4,5 triol | 11.2 |
-CH3 | 2,4 di-OCH3 | 12.4 |
-CH3 | 3-COOH, 4-OH | 16.2 |
-CH3 | Cinnamoyl | 21.0 |
-CH3 | 4-OCH3 | 27.7 |
-CH3 | 2,5 di-OCH3 | 30.9 |
-CH3 | 3,5 di-OCH3 | 38.7 |
-CH3 | 4-pypiridine N Oxide | 44.5 |
FUTURE PROSPECTS
The literature indicates that PP2A activation has a significant role in Alzheimer’s disease, and it is currently being used as a treatment approach in the condition. Recently, there has been a resurgence of interest in PP2A activation methods due to the recent failure of vaccination therapy in a phase 3 clinical trial and the low activity of other acetylcholine esterase inhibitors. In this review article, we gathered information on the active controlling role of PP2A in Alzheimer’s disease, the failure of vaccination therapy and its likely cause, the available limited activity compounds, and PP2A activating compounds in particular. We are concentrating on the available chemical compounds with basic pharmacophores of PP2A activating compounds, their mechanism of action to induce enzyme activity, and their structure activity relationship, which is how some of the synthesised low molecular weight chemical compounds can show the greatest PP2A activating effect in the presence of C-2 ceramide. PP2A activation is currently a hot topic in Alzheimer’s therapy research. It has been demonstrated in the neuroscience research sector that several chemical compounds listed in the Table 2 of this article are useful in the treatment of Alzheimer’s disease by activating the enzyme PP2A in various ways. This collective information may entice researchers to learn more about the pharmacophore that is fundamentally involved in PP2A activation and may pique their interest in synthesizing more similar derivatives or modifications of existing compounds that activate PP2A. Although the outcomes are difficult to predict, researchers hope to shed new light on Alzheimer’s therapy.58,59
CONCLUSION
Multiple drugs have been reported that show promise in activating PP2A for the treatment of Alzheimer’s disease, according to the review. PP2A remains a challenging therapeutic target despite mounting evidence that it is possible and has clear therapeutic benefits in the treatment of Alzheimer’s disease. This is in part because of its complex structure and lack of knowledge regarding the physiological roles and regulation of particular PP2A holoenzymes in cells with normal vs. cells affected by Alzheimer’s disease. In the near future, this knowledge should enable us to modulate the phosphatase activity of a specific subset of PP2A holoenzymes, particular PP2A-regulator interactions, or PP2A-substrate interactions, enabling us to enhance the already promising Alzheimer’s disease treatment approaches described in this review.
Cite this article
Mondal P, Samajdar S, Acharyya S, Mondal S. Protein Phosphatase 2A Activation for the Treatment of Alzheimer Disease-Promises and Challenges. Int. J. Pharm. Investigation. 2023;13(4):711-20.
ACKNOWLEDGEMENT
We would like to acknowledge Brainware University for providing us with the inspiration to work on this Research.
ABBREVIATIONS
PP2A | Protein Phosphatase 2A |
---|---|
AD | Alzheimer’s disease |
SAM | S- adenosine -L methionine |
AChEI | Acetyl choline esterase inhibitor |
AICAR | 5-aminoimidazole-4-carboxyamide ribo nucleotide |
MCI | Mild cognitive impairment |
MID1-α4 | Midline-1 alpha four |
PKC | Protein kinase C |
References
- Shen ZX. Brain cholinesterases: II. The molecular and cellular basis of Alzheimer’s disease. Med Hypotheses. 2004;63(2):308-21. [PubMed] | [CrossRef] | [Google Scholar]
- Edlund C, Söderberg M, Kristensson K. Isoprenoids in aging and neurodegeneration. Neurochem Int. 1994;25(1):35-8. [PubMed] | [CrossRef] | [Google Scholar]
- Nistor M, Don M, Parekh M, Sarsoza F, Goodus M, Lopez GE, et al. Alpha- and beta-secretase activity as a function of age and beta-amyloid in Down syndrome and normal brain. Neurobiol Aging. 2007;28(10):1493-506. [PubMed] | [CrossRef] | [Google Scholar]
- Lott IT, Head E. Alzheimer disease and Down syndrome: factors in pathogenesis. Neurobiol Aging. 2005;26(3):383-9. [PubMed] | [CrossRef] | [Google Scholar]
- Schmitz C, Rutten BP, Pielen A, Schäfer S, Wirths O, Tremp G, et al. Hippocampal neuron loss exceeds amyloid plaque load in a transgenic mouse model of Alzheimer’s disease. Am J Pathol. 2004;164(4):1495-502. [PubMed] | [CrossRef] | [Google Scholar]
- Wimo A, Prince M. World Alzheimer report 2010: the global economic impact of dementia. Alzheimers Dis Int. 2011:1-52. [PubMed] | [CrossRef] | [Google Scholar]
- Houghton PJ, Howes MJ. Natural products and derivatives affecting neurotransmission relevant to Alzheimer’s and Parkinson’s disease. Neurosignals. 2005;14(1-2):6-22. [PubMed] | [CrossRef] | [Google Scholar]
- Aisen PS, Gauthier S, Ferris SH, Saumier D, Haine D, Garceau D, et al. Tramiprosate in mildto-moderate Alzheimer’s disease – a randomized, double-blind, placebo-controlled, multi-centre study (the Alphase Study). Arch Med Sci. 2011;7(1):102-11. [PubMed] | [CrossRef] | [Google Scholar]
- Yi K. Passive immunotherapy-a viable treatment for Alzheimer’s disease. Psychiatr Danub. 2014;26(Suppl 1):256-65. [PubMed] | [Google Scholar]
- Jones TA, Barker HM, Silva ED, Mayer-Jaekel RE, Hemmings BA, Spurr NK, et al. Localization of the genes encoding the catalytic subunits of protein phosphatase 2A to human chromosome bands 5q23→ q31 and 8p12→ p11. 2, respectively. Cytogenet Cell Genet. 1993;63(1):35-41. [PubMed] | [Google Scholar]
- Pandey P, Seshacharyulu P, Das S, Rachagani S, Ponnusamy MP, Yan Y, et al. Impaired expression of protein phosphatase 2A subunits enhances metastatic potential of human prostate cancer cells through activation of AKT pathway. Br J Cancer. 2013;108(12):2590-600. [PubMed] | [Google Scholar]
- [Cited: 2022 Mar 10];Protein Phosphate 2. en.wikipedia.org/wiki/Protein_phosphatase_2? [PubMed] | [Google Scholar]
- Braithwaite SP, Stock JB, Lombroso PJ, Nairn AC. Protein phosphatases and Alzheimer’s disease. Prog Mol Biol Transl Sci. 2012;106:343-79. [PubMed] | [CrossRef] | [Google Scholar]
- Liu WB, Li Y, Zhang L, Chen HG, Sun S, Liu JP, et al. Differential expression of the catalytic subunits for PP-1 and PP-2A and the regulatory subunits for PP-2A in mouse eye. Mol Vis. 2008;14:762-73. [PubMed] | [Google Scholar]
- Tanimukai H, Grundke-Iqbal I, Iqbal K. Up-regulation of inhibitors of protein phosphatase-2A in Alzheimer’s disease. Am J Pathol. 2005;166(6):1761-71. [PubMed] | [Google Scholar]
- Qian W, Shi J, Yin X, Iqbal K, Grundke-Iqbal I, Gong CX, et al. PP2A regulates tau phosphorylation directly and also indirectly via activating GSK-3beta. J Alzheimers Dis. 2010;19(4):1221-9. [PubMed] | [CrossRef] | [Google Scholar]
- Iqbal K, Alonso AC, Chen S, Chohan MO, El-Akkad E, Gong CX, et al. Tau pathology in Alzheimer disease and other tauopathies. Biochim Biophys Acta. 2005;1739(2-3):198-210. [PubMed] | [CrossRef] | [Google Scholar]
- Liu F, Grundke-Iqbal I, Iqbal K, Gong CX. Contributions of protein phosphatases PP1, PP2A, PP2B and PP5 to the regulation of tau phosphorylation. Eur J Neurosci. 2005;22(8):1942-50. [PubMed] | [CrossRef] | [Google Scholar]
- Millward TA, Zolnierowicz S, Hemmings BA. Regulation of protein kinase cascades by protein phosphatase 2A. Trends Biochem Sci. 1999;24(5):186-91. [PubMed] | [CrossRef] | [Google Scholar]
- Lee MS, Kao SC, Lemere CA, Xia W, Tseng HC, Zhou Y, et al. APP processing is regulated by cytoplasmic phosphorylation. J Cell Biol. 2003;163(1):83-95. [PubMed] | [CrossRef] | [Google Scholar]
- Colombo A, Bastone A, Ploia C, Sclip A, Salmona M, Forloni G, et al. JNK regulates APP cleavage and degradation in a model of Alzheimer’s disease. Neurobiol Dis. 2009;33(3):518-25. [PubMed] | [CrossRef] | [Google Scholar]
- Sontag E, Nunbhakdi-Craig V, Lee G, Brandt R, Kamibayashi C, Kuret J, et al. Molecular interactions among protein phosphatase 2A, tau, and microtubules: implications for the regulation of tau phosphorylation and the development of tauopathies. J Biol Chem. 1999;274(36):25490-8. [PubMed] | [CrossRef] | [Google Scholar]
- Gong CX, Singh TJ, Grundke‐Iqbal I, Iqbal K. Phosphoprotein phosphatase activities in Alzheimer disease brain. J Neurochem. 1993;61(3):921-7. [PubMed] | [CrossRef] | [Google Scholar]
- Kickstein E, Krauss S, Thornhill P, Rutschow D, Zeller R, Sharkey J, et al. Biguanide metformin acts on tau phosphorylation via mTOR/Protein Phosphatase 2A (PP2A) signaling. Proc Natl Acad Sci U S A. 2010;14;107(50):21830-5. [PubMed] | [CrossRef] | [Google Scholar]
- Bharatam PV, Patel DS, Iqbal P. Pharmacophoric features of biguanide derivatives: an electronic and structural analysis. J Med Chem. 2005;48(24):7615-22. [PubMed] | [CrossRef] | [Google Scholar]
- Ceramide. Wikipedia. [cited Jul 19 2022]. Available fromhttp://en.wikipedia.org/wiki/Ceramide
- Dobrowsky RT, Kamibayashi C, Mumby MC, Hannun YA. Ceramide activates heterotrimeric protein phosphatase 2A. J Biol Chem. 1993;268(21):15523-30. [PubMed] | [CrossRef] | [Google Scholar]
- Chalfant CE, Kishikawa K, Mumby MC, Kamibayashi C, Bielawska A, Hannun YA, et al. Long chain ceramides activate protein phosphatase-1 and protein phosphatase-2A. Activation is stereospecific and regulated by phosphatidic acid. J Biol Chem. 1999;274(29):20313-7. [PubMed] | [CrossRef] | [Google Scholar]
- Chun J, Hartung HP. Mechanism of action of oral Fingolimod (FTY720) in multiple sclerosis. Clin Neuropharmacol. 2010;33(2):91-101. [PubMed] | [CrossRef] | [Google Scholar]
- Forskolin. Wikipedia. [cited Jun 05 2022]. Available fromhttp://en.wikipedia.org/wiki/Forskolin
- Tian Q, Zhang JX, Zhang Y, Wu F, Tang Q, Wang C, et al. Biphasic effects of forskolin on tau phosphorylation and spatial memory in rats. J Alzheimers Dis. 2009;17(3):631-42. [PubMed] | [CrossRef] | [Google Scholar]
- Khandelwal Y, Moreas G, Lal B, Aroskar VA, Dhodwalla AN, Rupp RH, et al. Patent 5. 1993;252:598 [PubMed] | [CrossRef] | [Google Scholar]
- Guertin KR. The synthesis of highly oxygenated decalin compounds. Med Chem. 1983;26:436-9. [PubMed] | [CrossRef] | [Google Scholar]
- Sontag E, Nunbhakdi-Craig V, Lee G, Bloom GS, Mumby MC. Regulation of the phosphorylation state and microtubule-binding activity of Tau by protein phosphatase 2A. Neuron. 1996;17(6):1201-7. [PubMed] | [CrossRef] | [Google Scholar]
- Ettenger R, Schmouder R, Kovarik JM, Bastien MC, Hoyer PF. Pharmacokinetics, pharmacodynamics, safety, and tolerability of single-dose Fingolimod (FTY720) in adolescents with stable renal transplants. Pediatr Transplant. 2011;15(4):406-13. [PubMed] | [CrossRef] | [Google Scholar]
- Sample records for pharmacokinetics biodistribution safety. [cited Nov 11 2022]. Available fromhttp://worldwidescience.org/topicpages/p/pharmacokinetics+biod istribution+safety.html
- Sontag JM, Nunbhakdi-Craig V, Montgomery L, Arning E, Bottiglieri T, Sontag E, et al. Folate deficiency induces in vitro and mouse brain region-specific downregulation of leucine carboxyl methyltransferase-1 and protein phosphatase 2A Bα subunit expression that correlate with enhanced tau phosphorylation. J Neurosci. 2008;28(45):11477-87. [PubMed] | [CrossRef] | [Google Scholar]
- Davare MA, Avdonin V, Hall DD, Peden EM, Burette A, Weinberg RJ, et al. A β2 adrenergic receptor signaling complex assembled with the Ca2+ channel Cav1. 2. Science. 2001;293(5527):98-101. [PubMed] | [CrossRef] | [Google Scholar]
- Jackson RA, Hawa MI, Jaspan JB, Sim BM, DiSilvio L, Featherbe D, et al. Mechanism of metformin action in non-insulin-dependent diabetes. Diabetes. 1987;36(5):632-40. [PubMed] | [CrossRef] | [Google Scholar]
- Rodríguez-González A, Ramirez de Molina A, Fernández F, Lacal JC. Choline kinase inhibition induces the increase in ceramides resulting in a highly specific and selective cytotoxic antitumoral strategy as a potential mechanism of action. Oncogene. 2004;23(50):8247-59. [PubMed] | [CrossRef] | [Google Scholar]
- Surguchov A. Invertebrate models untangle the mechanism of neurodegeneration in Parkinson’s disease. Cells. 2021;10(2):407 [PubMed] | [CrossRef] | [Google Scholar]
- Iqbal J, Zhang K, Jin N, Zhao Y, Liu Q, Ni J, et al. Effect of sodium selenate on hippocampal proteome of 3× Tg-AD mice—exploring the antioxidant dogma of selenium against Alzheimer’s disease. ACS Chem Neurosci. 2018;9(7):1637-51. [PubMed] | [CrossRef] | [Google Scholar]
- Galli C, Meucci O, Scorziello A, Werge TM, Calissano P, Schettini G, et al. Apoptosis in cerebellar granule cells is blocked by high KCl, forskolin, and IGF-1 through distinct mechanisms of action: the involvement of intracellular calcium and RNA synthesis. J Neurosci. 1995;15(2):1172-9. [PubMed] | [CrossRef] | [Google Scholar]
- Zemek F, Drtinova L, Nepovimova E, Sepsova V, Korabecny J, Klimes J, et al. Outcomes of Alzheimer’s disease therapy with acetylcholinesterase inhibitors and memantine. Expert Opin Drug Saf. 2014;13(6):759-74. [PubMed] | [CrossRef] | [Google Scholar]
- Cahlíková L, Vrabec R, Pidaný F, Peřinová R, Maafi N, Mamun AA, et al. Recent progress on biological activity of Amaryllidaceae and further isoquinoline alkaloids in connection with Alzheimer’s disease. Molecules. 2021;26((17):26(17)):5240 [PubMed] | [CrossRef] | [Google Scholar]
- Mehta M, Adem A, Sabbagh M. New acetylcholinesterase inhibitors for Alzheimer’s disease. Int J Alzheimers Dis. 2012;2012:728983 [PubMed] | [CrossRef] | [Google Scholar]
- Schroeter H, Williams RJ, Matin R, Iversen L, Rice-Evans CA. Phenolic antioxidants attenuate neuronal cell death following uptake of oxidized low-density lipoprotein. Free Radic Biol Med. 2000;29(12):1222-33. [PubMed] | [CrossRef] | [Google Scholar]
- Corcoran NM, Martin D, Hutter-Paier B, Windisch M, Nguyen T, Nheu L, et al. Sodium selenate specifically activates PP2A phosphatase, dephosphorylates tau and reverses memory deficits in an Alzheimer’s disease model. J Clin Neurosci. 2010;17(8):1025-33. [PubMed] | [CrossRef] | [Google Scholar]
- Guo JA, Wang XY, Dai LM. Pharmacokinetics of sodium selenite in human body at different levels of selenium. Zhongguo Yao Li Xue Bao. 1991;12(3):226-8. [PubMed] | [Google Scholar]
- Reisberg B, Doody R, Stöffler A, Schmitt F, Ferris S, Möbius HJ, et al. Memantine in moderater-to-severe Alzheimer’s disease. N Engl J Med. 2003;348(14):1333-41. [PubMed] | [CrossRef] | [Google Scholar]
- Aarsland D, Ballard C, Walker Z, Bostrom F, Alves G, Kossakowski K, et al. Memantine in patients with Parkinson’s disease dementia or dementia with Lewy bodies: a double-blind, placebo-controlled, multicentre trial. Lancet Neurol. 2009;8(7):613-8. [PubMed] | [CrossRef] | [Google Scholar]
- Johansson C, Ballard C, Hansson O, Palmqvist S, Minthon L, Aarsland D, et al. Efficacy of memantine in PDD and DLB: an extension study including washout and open-label treatment. Int J Geriatr Psychiatry. 2011;26(2):206-13. [PubMed] | [CrossRef] | [Google Scholar]
- Schneider LS, Dagerman KS, Higgins JP, McShane R. Lack of evidence for the efficacy of memantine in mild Alzheimer disease. Arch Neurol. 2011;68(8):991-8. [PubMed] | [CrossRef] | [Google Scholar]
- Parsons CG, Danysz W, Bartmann A, Spielmanns P, Frankiewicz T, Hesselink M, et al. Aminoalkyl-cyclohexanes are novel uncompetitive NMDA receptor antagonists with strong voltage-dependencyand fast blocking kinetics: in vitro and in vivo characterization. Neuropharmacology. 1999;38(1):85-108. [PubMed] | [CrossRef] | [Google Scholar]
- Periclou AP, Ventura D, Sherman T, Rao N, Abramowitz WT. Lack of pharmacokinetic or pharmacodynamic interaction between memantine and donepezil. Ann Pharmacother. 2004;38(9):1389-94. [PubMed] | [CrossRef] | [Google Scholar]
- Beconi MG, Howland D, Park L, Lyons K, Giuliano J, Dominguez C, et al. Pharmacokinetics of memantine in rats and mice. Version 2 PLOS Curr. 2011;3 [CrossRef] | [Google Scholar]
- Leoni LM, Shih HC, Deng L, Tuey C, Walter G, Carson DA, et al. Modulation of ceramide-activated protein phosphatase 2A activity by low molecular weight aromatic compounds. Biochem Pharmacol. 1998;55(7):1105-11. [PubMed] | [CrossRef] | [Google Scholar]
- Martin L, Latypova X, Wilson CM, Magnaudeix A, Perrin ML, Terro F, et al. Tau protein phosphatases in Alzheimer’s disease: the leading role of PP2A. Ageing Res Rev. 2013;12(1):39-49. [PubMed] | [CrossRef] | [Google Scholar]
- Gratuze M, Julien J, Petry FR, Morin F, Planel E. Insulin deprivation induces PP2A inhibition and tau hyperphosphorylation in hTau mice, a model of Alzheimer’s disease-like tau pathology. Sci Rep. 2017;7(1):46359 [PubMed] | [CrossRef] | [Google Scholar]