ABSTRACT
Background
Tofacitinib Citrate (TCB) is a selective Janus kinase inhibitor approved by the USFDA for the treatment of Rheumatoid arthritis. However, TCB faces extensive first pass metabolism and causes GI trouble on oral administration. To overcome these adverse effects of orally administered TCB, topical delivery can be a suitable alternative.
Objectives
The present research study aims to determine the process variables that affect the incorporation of TCB into niosomes by employing Box-Behnken Design (BBD) to finally produce an optimized TCB-Niosomes (TN), that could improve drug delivery to arthritic tissues and incorporating it in to hydrogel system to prepare TCB niogel.
Materials and Methods
Niosomes were prepared using Ethanol injection method. The critical material attributes and process parameters and their effect on product quality variables were assessed and optimized using BBD and a hydrogel was prepared with optimized TN and thus, has effectively trapped TCB. In vitro release properties of the TCB-loaded niosomes were studied and the anti-inflammatory effectiveness of these nanostructures was evaluated using an adjuvant-induced arthritis rat model.
Results
Optimized niosomes have particle size of 167±8.7 nm, PDI 0.2±0.04 and %EE 80.2±3.1. ZP of optimized TN was -32±2.1 indicating a stable preparation. In vitro drug release study for TCB niosomes, performed using dialysis bag method has shown an improvement compared to saturated drug solution and it followed korsmeyer peppas model (R2=0.9511) and Quasi-Fickian diffusion (n<0.4). In vivo studies revealed the improved efficiency of TCB niogel over conventional TCB gel with significant reduction in paw volume and arthritic score.
Conclusion
Our findings demonstrated that TCB niosomes can target inflamed tissues when administered topically in a niogel system and thereby, a promising and effective delivery of TCB that could offer safer and more efficient treatment to RA.
INTRODUCTION
The American College of Rheumatology (ACR) advises Disease-Modifying Antirheumatic Drugs (DMARDs) as therapy for the management of chronic inflammatory Rheumatoid Arthritis (RA), individually or in combination with other classes of anti-inflammatory drugs, for moderate to high disease responses (Lundquist et al., 2014). Tofacitinib (TCB) is a DMARD, an inhibitor of the Janus Kinase 3 (JAK3) is a medication prescribed for individuals with moderate to severe active rheumatoid arthritis, who are intolerant to Methotrexate (MTX) therapy or did not respond well, either alone or along with non-biological DMARDs to manage disease activity (Zerbini and Lomonte, 2012). The novel DMARD, TCB apart from providing pain relief also influences disease progression and joint damage (Songet al., 2014; Kawalecet al., 2018). TCB belongs to the BCS class-III with water solubility <1 mg/mL and poor permeation efficiency (National Center for Biotechnology Information, 2024). Though patients adhere well to the treatment, the oral administration of tofacitinib citrate does not allow specific targeting to the affected area and has relative issues of poor therapeutic efficacy as a reason of low drug concentration at the effective sites and adverse systemic effects (Fleischmannet al., 2016). It was noted that the therapeutic outcomes of the drugs could be enhanced by incorporating them into nanocarrier delivery systems, enabling TCB to effectively target the sites affected by rheumatoid arthritis (Fanget al., 2019).
Drug delivery systems are vital in developing dermatological products for effective treatment, thereby improving therapeutic efficacy by establishing patient compliance and an increased selectivity and reduced systemic toxicity of the drug. Nanomedicine is recognized as a potent tool for enhancing the pharmacokinetics of therapeutic agents (Prasadet al., 2015; Shietal., 2017) and a variety of them such as liposomes, nanoparticles, micelles, etc., was being employed in the RA condition to deliver anti-inflammatory drugs to inflamed joints (Shenet al., 2020). They work by using a passive targeting strategy thereby improving the drug delivery efficiency to inflamed tissues for an effective treatment (Ulmanskyet al., 2012; Wanget al., 2016; Feng and Chen, 2018). The EPR effect, analogous to ELVIS, the extravasation and inflammatory cell-mediated sequestration, as observed in solid tumors, was the one that modulates the drug delivery to arthritic joints as RA is also an angiogenesis-dependent disease (Wanget al., 2016; Alamet al., 2017; Heoet al., 2017).
Niosomes are drug-loaded vesicular systems, of size 10-1000 nm in diameter, uni or multi-lamellate bi-layered non-ionic surfactant membrane enclosing an aqueous core with or without cholesterol impregnated in the surfactant layer. The inclusion of cholesterol improves the stability and brings about an order in the niosomal bilayer. Niosome’s unique structure allows them to encapsulate the lipophilic as well as hydrophilic drugs in the bilayer matrix and the core inside. Bilayer’s fluidity is influenced by the cholesterol content in the layer and surfactant’s profile of hydrophilic head group and alkyl chain length (Peeyush et al., 2020; Gannu et al., 2011).
Nanotechnology is often seen as more effective than traditional topical forms in delivering drugs through the skin due to its high capacity for trapping drugs, solubility, permeability and penetration (Cláudia Paiva-Santoset al., 2022). Delivery of drugs across the skin’s surface is frequently acknowledged as more beneficial than administering them systemically when the target sites are near the dermal layers or it itself is the target, or when oral delivery is not admissible, for avoiding side effects from the uptake of drug by untargeted sites (Ahmad,et al., 2019; Khanet al, 2018; Bashiret al., 2020). Niosomes have received significant attention for their role in delivering drugs through the skin. They possess qualities like biodegradability, non-toxicity, ability to alter drug behavior in the body. Niosomes facilitate drug penetration into the skin and prolong their presence, thereby reducing systemic absorption. This can be advantageous in regulating drug effects within the body (Muzzalupo,et al., 2011; Rungphanichkulet al., 2011; Shah and Patel, 2023; Junyaprasertet al., 2012) When niosomes are applied to the skin, they enhance the properties of the outermost skin layer by decreasing its water loss. Additionally, the attachment and merging of niosomes with the skin elements will enhance the drug’s thermodynamic activity gradient, affecting drug permeation (El-Menshawe and Hussein, 2013; Manconiet al., 2006).
Quality by Design (QbD) approach involves creating a product for which manufacturing processes meet the predetermined product standards (Nayak et al., 2024). It can be utilized to analyze the impact of various factors on responses by simultaneously adjusting them in a limited number of experiments (Grangeiaet al., 2020). This is vital, for gaining a thorough understanding of the product and process behavior, process parameters and critical material attributes and their influence on product quality and for optimizing the parameters to final specifications by determining the optimal formulation composition (Mishraet al., 2018; Katonaet al., 2020).
Design of Experiments (DoE), a method within the QbD approach is used to study the effect of different factors on product quality. It helps to plan, carry out, analyze and interpret experiments to find connections between factors that affect a process and the outcomes. DoE uses statistical techniques to optimize processes, reduce variability and improve product performance (Pratik et al., 2019; Rajanaet al., 2024). The Box-Behnken Design (BBD) is an experimental designing method in the Response Surface Methodology (RSM) used to optimize processes involving multiple factors, requiring fewer experimental runs than other methods (Ahadet al., 2012; Sailaja and Bhupalam, 2023). The Design-Expert 13 software was used in this study, to develop an experimental design for understanding the impact of variables on predetermined and intended responses.
MATERIALS AND METHODS
Chemicals and reagents
TCB, a generous gift from Hetero Labs Ltd, Hyderabad. Span 60, pluronic F127 and cholesterol were procured from SRL, India and dialysis membrane, Freund’s complete and incomplete and Carbopol 934 from Merck, Sigma-Aldrich. High-quality analytical grade solvents were procured and used.
Male Wistar rats for antiarthritic activity evaluation were purchased from authenticated breeders from Hyderabad, Telangana. IAEC has approved the protocol of animal experiments in protocol number: IAEC/SVCP/2022/10.
Preparation and optimization of TCB Niosomes
Ethanol injection method was used to fabricate TCB niosomes. Preparation procedure in brief, span 60 and cholesterol were dissolved in 2 mL of ethanol over a bath sonicator (Ikon Industries Ultrasonic Bath sonicator) to obtain a clear solution. Drug-TCB and pluronic-F127 were added to phosphate buffer (pH 7.4)-the hydrating solution, in a separate beaker. The organic solution was added drop-wise into the aqueous solution maintained at 60-65ºC with a syringe plunger at a 1 mL/min rate while continuously stirring at 850 rpm, using REMI 5mLH Q-20A model magnetic stirrer with hot plate. Vesicle formation occurs at the temperature above the transition temperature and the evaporation of the organic phase at the interphase between the 2 phase’s results in niosomes. Stirring was continued at the same temperature maintained over a hot mantle, for 45 min and later at room temperature for 2 hr, for pruning and stabilization of niosomes to take place (Pratik et al., 2019).
After a keen understanding of the influential factors in niosomes preparation from the preliminary trials, the variable parameters that crucially affect the quality of niosomes were enlisted. Optimization studies were conducted by applying these factors in BBD design model developed using Design-Expert 13 software, which is an appropriate model in statistical validation techniques of quadratic Response surface methodology when more complexity of multiple factors and responses exit. The independent variables were the span 60 concentration (A), cholesterol concentration (B), hydration volume (C) and the dependent variables being particle size (nm): (Y1), polydispersity index: (Y2) and the entrapment efficiency: (Y3) as responses, studied in a total of 17 formulation batches suggested by the Design expert. An average of triplicate values of responses for each formulation batch were taken and proceeded for optimization data analysis (Sailaja and Bhupalam, 2023).
Characterization of formulation batches
The formulation batches thus prepared were assessed for the responses comprehended as dependent variable constraints.
Particle Size (PS), PDI and Zeta potential
PS and PDI, could be analyzed in one setting in Zeta sizer and Zeta Potential (ZP) in the other and these parameters were assessed for the formulation batches using ZS 90 in a dynamic light scattering approach (Malvern instruments, UK). Separate cuvettes were used for estimation of particle size and zeta potential. The samples were diluted appropriately using distilled water and transferred to cuvettes for, to be placed in the Dynamic Light Scattering (DLS) analyzer to measure the parameters.
Preparation of TCB calibration curve
Quantification of TCB at various instances during the evaluation was availed with the RP-HPLC (Shimadzu, column oven CTO-10AS VP, pump LC-20AD, UV detector-SPD-20A) by plotting the TCB calibration curve prepared using the method developed by Atmakuri et al. with modifications. An isocratic mobile phase used was Methanol: Water in 70:30 ratios and using C18 column, with a flow rate of 1 mL/min. injection volume was maintained constant at 10 μL, a detection wavelength at 289 nm, peak retention time was 4.7 min (Atmakuriet al., 2023).
Entrapment efficiency
An indirect method of estimation was used for finding the entrapment efficiency of formulated batches of niosomes wherein the trapped drug quantity of TCB in the niosomal sediment was obtained by subtracting the quantity of untrapped TCB in supernatant solution, after centrifugation (Centrifuge 5430 G), from the amount of total drug added to the formulation and indicates the efficiency of delivery system to uptake and hold the drug into its constitution.
Drug Loading
Drug Loading Capacity (LC%) of a nano-formulation is the amount of drug that could be trapped in the system to its weight. Its effectiveness lies in its ability to hold the maximum quantity of drug and still being stable. LC% of the optimized formulation batch was estimated.
ATR-FTIR and Surface morphology analysis
It was performed to qualitatively validate the TCB to have been loaded into niosomes without inflicting any molecular changes in other components of the formulation or to the drug itself. Perkin Elmer-FTIR spectrum 2 spectrometer aided scanning of samples at a wavenumber range of 4000-400 cm-1. Reports were analyzed by comparing the peaks in the fingerprint region, peaks for characteristic shifts representing the molecular changes(Songet al., 2014).
Surface morphology of optimized TCB-loaded niosomes was analyzed using TEM (Thermofisher, USA). A drop of the preparation placed on the carbon-coated grid, dried and stained was viewed under TEM’s suitable magnification operating at an accelerated voltage.
In vitro release study-TCB niosomes
In vitro release studies were conducted using a dialysis sac technique. A 2 mL of TCB niosomal suspension, equivalent to 0.6 mg of TCB was placed in the dialysis bag of nitrocellulose membrane, priorly tied on one side properly so as not to allow any leakage. The other end of the tubing was also tied and was kept submerged in the beaker containing 60 mL release medium-Phosphate Buffer Saline (PBS buffer) (pH 7.4), maintained at 37±1ºC and a stirring speed of 70 rpm. Samples were drawn at regular time intervals (0.5, 1, 2, 3, 4, 5, 6, 7, 8, 24 hr), for HPLC analysis and replaced an equivalent quantity of fresh buffer for balancing the sink condition. The release data was interpreted in different mathematical models to understand the release profile of TCB from TCB-loaded niosomes to compare with that of the free-TCB drug solution examined in similar test conditions (National Center for Biotechnology Information, 2024).
Stability studies
The optimized formulation batch of TCB niosomes was assessed for physical and chemical stability according to ICH guidelines, at varied conditions of storage (2-8±2ºC, 25±2ºC, 45±2ºC at 65%±5% RH), in a stability chamber, for three months and a regular monitoring for changes in size of particles and EE% was carried out to assess the stability of the formulation.
Preparation and characterization of TCB Niosomal gel (TCB Niogel)
TCB niosomes concentrate was obtained by centrifuging the suspension of niosomal formulation for 20 min at 20,000 rpm in the centrifuge and discarding the supernatant portion with unentrapped TCB and the niosomal concentrate thus obtained was resuspended into water. Carbopol 934 was the gelling agent used. A 1% hydrogel was prepared by dispersing the niosomal concentrate along with the gelling agent into a relevant quantity of water, soaking and incubating for 6 hr. while continuously mixing and finally adjusting the pH using 0.5% triethanolamine. A visual inspection of the gel’s color and texture were conducted.
A digital pH meter (model CPH 102) was used to measure the pH of TCB Niogel after a predetermined amount of gel was appropriately diluted in distilled water. Using a Brookfield Viscometer, spindle 64 (DV-III, USA), the viscosity of the produced gel was measured. 0.5 gm samples from different portions of the formulations were obtained and individually dissolved in PBS of pH 7.4 before being evaluated by HPLC to ascertain the percentage drug content and homogeneity (El-Menshawe & Hussein, 2013).
In vivo anti-arthritic activity
The induction of arthritis in male Wistar rats was accomplished using Freund’s complete and incomplete adjuvant factor. For the study, four groups, each containing 6 animals were used, among which one group served as the Normal Control (group-1) (NC) and one was the Disease Control (group-2) (DC). Two were Treatment Groups (TG). Arthritis was induced in three groups, except NC, with 0.1 mL of FCA injected in the sub-plantar region of the right hind paw and incomplete Freund’s factor into the same paw as a booster dose on the 7th day. The NC group remained unimmunized. Development of arthritis was confirmed through visual observations, measurement of inflammation, joint and paw swelling and estimation of arthritic score, indicating an established RA condition. The study continued with bis-in-die topical treatment of two animal groups using TCB gel-the control gel (group-3) and TCB niogel (group-4) for 14 days starting from the 14th day of disease inoculation with FCA. Throughout the study period, arthritic scores, paw volumes and joint stiffness were regularly assessed.21
Statistical data analysis
Experiments were conducted in triplicates and data interpretation was through a two-tailed student t-test with mean±SD values using the GraphPad prism version 5.0 software, to derive into the probability value. p-value<0.05 is considered statistical significance.
RESULTS
TCB belongs to BCS class III, a drug with low permeability. To overcome the systemic side effects and issues related to oral administration, it is better to formulate into a topical delivery system and thus hypothesized that formulating a nano-vesicular system can enhance the TCB penetrability and hydrogel-based systems will act as a drug depot for a slow and sustained release. From the preliminary investigations, the formulation and process variables considered as independent variables that were majorly influencing the responses i.e., the dependent variables, were identified and with this set of factors, Box Behnken experimental design was developed. The responses evaluated were then interpreted in different process orders to rationalize between the models to suggest the best-fit model and the aliased models, by the highest order of F-value and lack of fit, from summary statistics. For individual response variables analyzed, ANOVA (Analysis of variance) of the thereby suggested model was pursued and the final equation in terms of coded factors was taken. Table 1 shows the responses of formulation batches (Figure 1).
Response1: Particle size (Y1)
ANOVA for quadratic model suggested significance with F-value of 22.06. Terms A, C, A2, B2, C2 were significant model terms in this case. The difference between predicted R2 and adjusted R2 and the adequate precision were within the leverage and the lack of fit F-value was non-significant. The final equation in terms of coded factors for response, particle size:
Response 2: PDI (Y2)
Terms B, C, AB, AC, A2, B2 and C2 were significant model terms. The difference between predicted and adjusted R2 values was <0.2, adequate precision was as high as 16.13 and a non-significant lack of fit. The final equation with the coded factors for response PDI:
Response 3: %EE (Y3)
The significant model terms for the model with F-value 9.11 were A and C. The final equation in terms of coded factors for this response %EE:
TN1-17 formulation batches were fabricated by a general Ethanol-injection method. PS, PDI and ZP were analysed using Malvern’s zeta sizer. PS was in the range of 152.4±5.61 to 260.7±8.58 nm. PDI was a minimum of 0.19±0.03 and a maximum, 0.36±0.04. %EE was operating between 71±6.83 and 88±4.17. The ZP of the optimized batch was -32.29 mV.
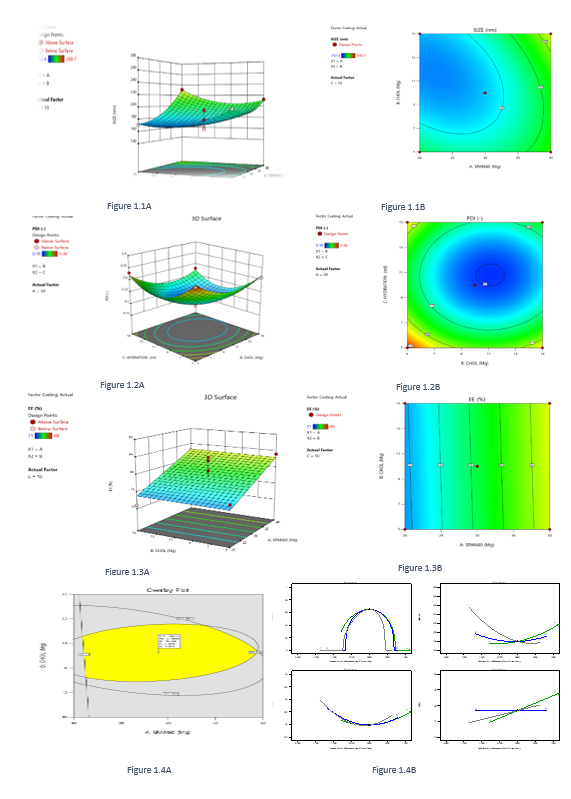
Figure 1:
3D surface and contour plots showing effect of significant factors on dependent variables. 1A, 1B shows the effect of A and B factors on particle size; 2A, 2B show the effect of B and C on PDI; 3A, 3B the effect of A and B factors on %EE and 4A was an overlay plot between AandB factors and 4B, Perturbation plots showing deviation from coded units.
Factor A | Factor B | Factor C | Response Y1 | Response Y2 | Response Y3 | |
---|---|---|---|---|---|---|
Formulation code | Span60 | Chol | Hydration | PS | PDI | EE |
Mg | Mg | mL | nm | – | % | |
TN1 | 20 | 15 | 10 | 170.5 | 0.27 | 71 |
TN2 | 20 | 5 | 10 | 196.8 | 0.28 | 75 |
TN3 | 40 | 5 | 10 | 212.3 | 0.33 | 84 |
TN4 | 30 | 10 | 10 | 162.3 | 0.21 | 78 |
TN5 | 40 | 15 | 10 | 230.9 | 0.26 | 83 |
TN6 | 20 | 10 | 15 | 152.4 | 0.23 | 76 |
TN7 | 30 | 15 | 5 | 250.2 | 0.31 | 75 |
TN8 | 30 | 5 | 5 | 258.9 | 0.35 | 73 |
TN9 | 30 | 10 | 10 | 193.4 | 0.19 | 80 |
TN10 | 40 | 10 | 5 | 260.7 | 0.31 | 77 |
TN11 | 30 | 10 | 10 | 177.1 | 0.21 | 84 |
TN12 | 30 | 15 | 15 | 176.6 | 0.28 | 80 |
TN13 | 30 | 10 | 10 | 161.2 | 0.19 | 78 |
TN14 | 20 | 10 | 5 | 240.9 | 0.36 | 72 |
TN15 | 30 | 10 | 10 | 175.1 | 0.21 | 83 |
TN16 | 40 | 10 | 15 | 201.8 | 0.32 | 88 |
TN17 | 30 | 5 | 15 | 171.8 | 0.33 | 76 |
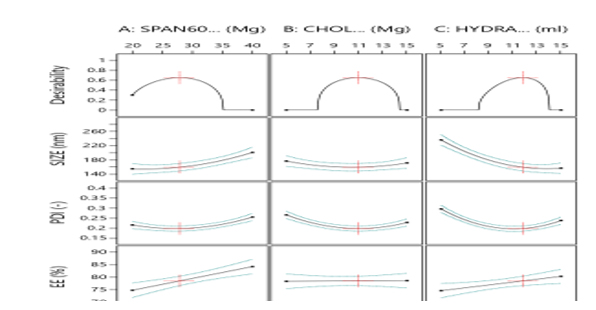
Figure 2:
All factors effect on individual responses and on the desirability factor.
The formulation factors for the solution suggested by the BBD design showing a desirability of 0.649 was repeated to obtain the confirmation report that was Two-sided at confidence 95% and it has given a data mean value of responses closer to the predicted mean (Figure 2).
Preparation of TCB calibration curve
Drug content estimations were performed using HPLC assay method and RP-HPLC calibration curve for TCB was plotted thereof with concentration Vs peak area detected at a wavelength of 289 nm for different concentrations of TCB to derive at the R2 value near to 1 indicating good linearity. Slope (m) is calculated in the linear regression model equation (y=mx+c).
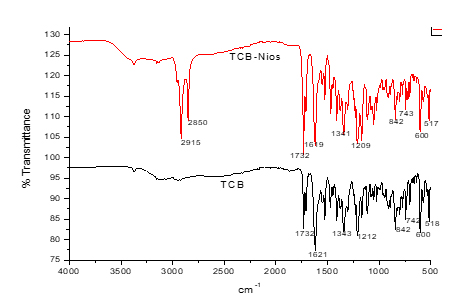
Figure 3:
FTIR spectrum of TCB and niosome formulation physical mixture.
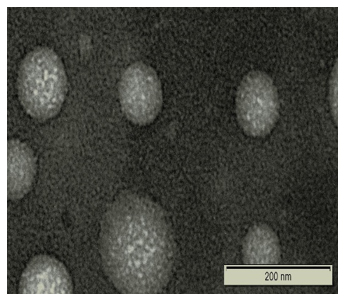
Figure 4:
TEM images of optimized formulation of TCB-loaded niosomes.
ATR-FTIR and Surface morphology analysis
FTIR spectral analysis of TCB and physical mixture of ingredients along with TCB used in the preparation of TCB niosomes were read and compared for identifying the new spectra formations that are not found in the individual ingredient’s spectral analysis indicating an occurrence of chemical reaction and a new chemical compound formation or retention of Drug peaks indicating compatibility, significant shifting, disappearance, or broadening of these peaks. Major peaks of TCB at 1490 cm-1 corresponding C=C stretching, Amide functional group- NH stretching at 3384 cm-1, 1664 cm-1 corresponding C=O in aromatic ring and for citrate component, C-O stretching between 1000-1300 cm-1 were retained in the spectra of physical mixture and stretching was within the limits showing compatibility of TCB with other formulation components and thereby no chemical interactions among the ingredients. FTIR spectra are shown in Figure 3.
Surface morphology analysis was conducted. TEM images in Figure 4 have revealed the spherical vesicular shape of niosomes and lamellarity could be observed. Figure 5 depicts the images of reports of PS and ZP and they were in correspondence with the TEM size analysis reports.
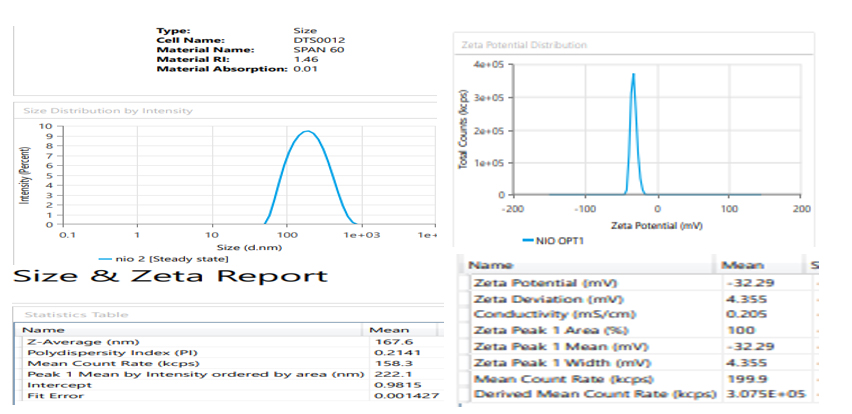
Figure 5:
Images of particle size and PDI report; Zeta potential report of optimized batch.
Stability studies
Stability studies were conducted following a standard protocol, for a definite period. The results of assessed parameters did not show any significant changes at low temperatures. Sedimentation and rise in particle size were observed, which could be because of particle aggregation, discharge of drug from vesicles and a decrease in EE%, sooner after one month of storage at 45±2ºC and later, at 25±2ºC. Physical instability and microbial mould growth were observed when stored at temperatures other than cool temperature, most apparently because cholesterol is an accommodable media for microbial growth at favorable temperatures, appealing a requirement of the addition of stabilizer and preservative.
In vitro release study
Dialysis sac method was used to perform the drug release study. Release samples collected at fixed intervals of time, for 24 hr, were assayed for drug content using HPLC and %Cumulative Drug Release (%CDR) data was interpreted in kinetic models. Drug release from TCB niosomes was compared with the release pattern of TCB-saturated solution. The %CDR after 24 hr. for the TCB solution was 83.2±3.4% and TCB niosomes have shown a release of 99.9±0.11%. A significant increase in the %CDR of TCB niosomes over TCB solution has thereby illustrated the statement that niosomes can enhance the permeation capacity of the drugs. A release kinetic study of TCB niosomes conducted in four models, the zero-order, first-order, Higuchi and Korsmeyer peppas model indicated, the model Korsmeyer peppas, to be the best fit, as this has the highest R2 value-0.9511. The kinetic model graphs are depicted in Figure 6.
Preparation and characterization of TCB niogel
TCB niogel was prepared by incorporating the optimized formulation into 1% Carbopol 934 and the prepared hydrogel was analyzed for physical parameters. Prepared TCB niogel was white homogenous with uniform consistency and pH was found to be 6.4±0.02. The viscosity of the TCB niogel was recorded with a Brookfield viscometer and the value was 20120±8.68 cp.
Drug content in the gel was quantitatively estimated using HPLC. The content was estimated by letting the drug get released into the aqueous PBS solution added and measuring the freed drug in the centrifuge supernatant and it was 0.61±0.02 mg of TCB in 0.5gms of TCB niogel and the content uniformity parameter was found within the limits.
In vivo antiarthritic activity
Arthritis was induced using FCA, as adjuvant-induced arthritis is the best-known simulating model of human RA. The day of arthritis induction with FCA complete was termed day “0” and a booster dose, incomplete Freund’s factor was given on the 7th day. Treatment started from day 14 after established arthritis was confirmed and up to day 28, with the topical application of TCB gel and TCB niogel. TCB gel is 0.15% TCB free drug in 1% Carbopol gel taken as the control gel and TCB niogel is Carbopol gel incorporated with niosomes equivalent to 0.12% of TCB. The arthritic score was assessed, with various parameters like paw volume, joint inflammation, joint stiffness and immobility, at regular intervals during the study. The Disease Control (DC) group and the treatment groups have shown an increase in paw volume after the FCA inoculation. In treatment groups, the paw inflammation gradually reduced upon treatment with TCB gel and TCB niogel, separately to the groups and in the DC group, it was sustained throughout the study period. Changes in the paw volume are depicted in the chart in Figure 6.
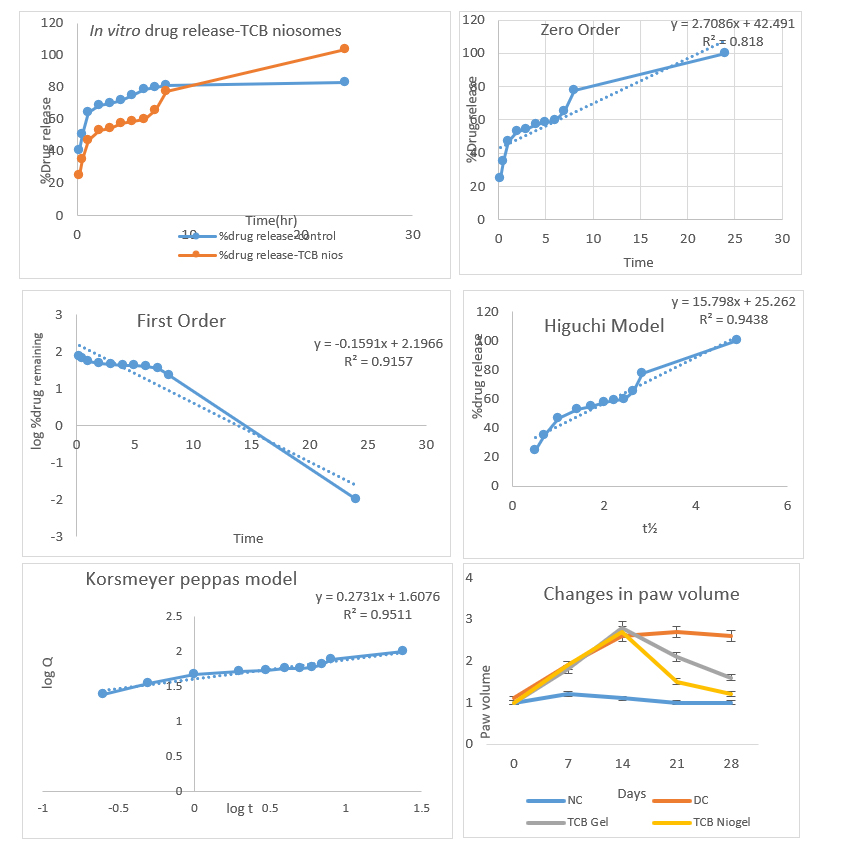
Figure 6:
Drug release studies showing %CDR of TCB saturated solution and TCB niosomes suspension and kinetic model plots with drug release data of TCB niosomes suspensions; the chart showing the changes in paw volume in the groups of in vivo antiarthritic activity.
DISCUSSION
TCB is an antiarthritic drug with relative issues like GI-related adverse effects and systemic toxicity that need to be addressed to tap its full potential as an antirheumatic agent. Encapsulating the hydrophilic drug into a deformable vesicular system and improving the penetrability of, thus a lesser lipophilic drug through the dermal layers is a novel strategy which was effectively worked out in the present study for TCB using BBD. Concerns about the limits on residual solvents in the nano-formulations have found the ethanol-injection method of preparation, a method to destine, in fabrication of niosomes. The preliminary investigations have evaluated a set of non-ionic surfactants to conclude at span-60 as the surfactant of choice which has HLB of 4.7, can form stable niosomes and has shown to enhance the vesicle properties when admixed with cholesterol that will impart rigidity and prevents leakage of vesicles at a certain concentration, but less or high concentrations of cholesterol will lead to the formation of fragile or more rigid vesicles limiting their %EE. All factor response graph acts as evidence for revealing the behavior of independent factors and their effects on the desirability and the dependent variables. There is an increase in the particle size and PDI, with an increase in span concentration which can be because of an increase in the viscosity of the medium with the increased surfactant concentration. This factor has a greater influence on the responses PDI and EE. The increase in the PDI of preparation explains the formation of micelles or aggregation, upon an increase in viscosity. More span will create more sites for entangling and for getting entrapped in the larger vesicles, so a higher %EE with higher concentration surfactant. Cholesterol, the other factor, increase in concentration has initially decreased the size as it decreases the fluidity and allows the formation of rigid and compact vesicles, but after a certain concentration, the increase in rigidity has limited the curvature of vesicles and so the vesicles could not take an acute curvature resulting in bigger vesicles. PDI is similarly affected but has an insignificant effect on %EE. Another factor assessed was the hydration volume which has a significant impact on all the responses considered. An increase in hydration volume has an inverse effect on particle size as vesicles are more spread with fewer interactive faces and less aggregation, thereby impacting the PDI factor. EE has significantly changed as it allows a steep gradience in drug concentration to diffuse into vesicles passively and get encapsulated in the hydrophilic core. More intermolecular surfaces can aid vesicle stabilization. The desirability of solutions varied relatively, and BBD design will interpret and evaluate the responses to frame the optimized solutions based on design goals. In vitro drug release data from vesicles has followed a quasi-fickian rule where release is concentration gradient-dependent, and the release mechanism is diffusion and bead swelling and relaxation of swellable and erodible hydrophilic matrix. Release % from niosomes has considerably increased over the TCB solution. In in vivo studies, an increase in paw volume indicates the disease activity, which has persistently increased in the DC group over the NC. The 2 groups of TG have shown a significant decrease in swelling which is a direct function of the formulation’s therapeutic efficiency and the effect of TCB niogel was more pronounced than the TCB gel.
CONCLUSION
The present study has allowed the development and optimization of TCB niosomes and the fabrication of niogel for the topical delivery of TCB. The niosomes thus prepared have shown an improved in vitro permeability and a sustained release pattern from the topical application, thereby it could be inferred that TCB niosomes in a topical delivery is a potential method of avoiding the adverse effects associated with oral administration and still can be an efficient antiarthritic drug in the topical delivery system. The in vitro and in vivo studies conducted have supported and the results are in agreement with the hypothesis proposed and thus conclusion could be drawn from the study that TCB-loaded niosomes in topical hydrogel system could effectively manage rheumatoid arthritis and find prospects in the commercialization of the formulation.
Cite this article:
Lalitha K, Kusuma MP. Formulation Development of Tofacitinib Niosomes Using Design of Experiments. Int. J. Pharm. Investigation. 2025;15(2):10-8.
ACKNOWLEDGEMENT
The authors like to express their gratitude to Hetero Labs Pvt. Ltd., for the gift sample of Tofacitinib citrate and NIPER-Hyderabad and SVCP-Hyderabad for facilitating the experimental work.
ABBREVIATIONS
TCB | Tofacitinib citrate |
---|---|
TN | Tofacitinib niosomes |
PS | Particle size |
PDI | Polydispersity index |
%EE | % Entrapment efficiency |
LC% | Loading capacity |
ATR-FTIR | Attenuated total reflectance-Fourier transform infrared spectroscopy |
ZP | Zeta potential |
BBD | Box-Behnken design |
%CPR | Cumulative percentage release |
ANOVA | Analysis of Variance |
TEM | Transmission Electron microscopy |
FCA | Freund’s complete Adjuvant |
HPLC | High-performance liquid chromatography |
References
- Ahad A., Aqil M., Kohli K., Sultana Y., Mujeeb M., Ali A., et al. (2012) Formulation and optimization of nanotransfersomes using experimental design technique for accentuated transdermal delivery of valsartan. Nanomedicine: Nanotechnology, Biology, and Medicine 8: 237-249 Google Scholar
- Ahmad U., Sohail M., Ahmad M., Minhas M. U., Khan S., Hussain Z., Kousar M., Mohsin S., Abbasi M., Shah S. A., Rashid H., et al. (2019) Chitosan based thermosensitive injectable hydrogels for controlled delivery of loxoprofen: Development, characterization and evaluation. International Journal of Biological Macromolecules 129: 233-245 Google Scholar
- Alam M. M., Han H. S., Sung S., Kang J. H., Sa K. H., Al Faruque H., Hong J., Nam E. J., Kim I. S., Park J. H., Kang Y. M., et al. (2017) Endogenous inspired biomineral-installed hyaluronan nanoparticles as pH-responsive carrier of methotrexate for rheumatoid arthritis. Journal of Controlled Release 252: 62-72 Google Scholar
- Atmakuri S., Nene S., Jain H., Joga R., Devabattula G., Godugu C., Srivastava S., et al. (2023) Topical delivery of tofacitinib citrate loaded novel nanoemulgel for the management of 2, 4-dichlorodinitrobenzene induced atopic dermatitis in mice model. Journal of Drug Delivery Science and Technology 80: 104145 Google Scholar
- Bashir S., Aamir M., Sarfaraz R. M., Hussain Z., Sarwer M. U., Mahmood A., Sarwer M. U., Akram M. R., Qaisar M. N., et al. (2020) Fabrication, characterization and release kinetics of tofacitinib-encapsulated polymeric nanoparticles: A promising implication in the treatment of rheumatoid arthritis. International Journal of Polymeric Materials and Polymeric Biomaterials 70: 449-458 Google Scholar
- Bhardwaj P., Tripathi P., Gupta R., Pandey S.. (2020) Niosomes: A review on niosomal research in the last decade. Journal of Drug Delivery Science and Technology 56: 101581 Google Scholar
- Cláudia Paiva-Santos A. C., Gama M., Peixoto D., Sousa-Oliveira I., Ferreira-Faria I., Zeinali M., Abbaspour-Ravasjani S., Mascarenhas-Melo F., Hamishehkar H., Veiga F., et al. (2022) Nanocarrier-based dermopharmaceutical formulations for the topical management of atopic dermatitis. International Journal of Pharmaceutics 618: 121656 Google Scholar
- El-Menshawe S. F., Hussein A. K.. (2013) Formulation and evaluation of meloxicam niosomes as vesicular carriers for enhanced skin delivery. Pharmaceutical Development and Technology 18: 779-786 Google Scholar
- Fang G., Zhang Q., Pang Y., Thu H. E., Hussain Z.. (2019) Nanomedicines for improved targetability to inflamed synovium for treatment of rheumatoid arthritis: Multi-functionalization as an emerging strategy to optimize therapeutic efficacy. Journal of Controlled Release 303: 181-208 Google Scholar
- Feng X., Chen Y.. (2018) Drug delivery targets and systems for targeted treatment of rheumatoid arthritis. Journal of Drug Targeting 26: 845-857 Google Scholar
- Fleischmann R., Kremer J., Tanaka Y., Gruben D., Kanik K., Koncz T., Krishnaswami S., Wallenstein G., Wilkinson B., Zwillich S. H., Keystone E., et al. (2016) Efficacy and safety of tofacitinib in patients with active rheumatoid arthritis: Review of key Phase 2 studies. International Journal of Rheumatic Diseases 19: 1216-1225 Google Scholar
- Grangeia H. B., Silva C., Simões S. P., Reis M. S.. (2020) Quality by design in pharmaceutical manufacturing: A systematic review of current status, challenges and future perspectives. European Journal of Pharmaceutics and Biopharmaceutics 147: 19-37 Google Scholar
- Heo R., You D. G., Um W., Choi K. Y., Jeon S., Park J.-S., Choi Y., Kwon S., Kim K., Kwon I. C., Jo D.-G., Kang Y. M., Park J. H., et al. (2017) Dextran sulfate nanoparticles as a theranostic nanomedicine for rheumatoid arthritis. Biomaterials 131: 15-26 Google Scholar
- Junyaprasert V. B., Singhsa P., Suksiriworapong J., Chantasart D.. (2012) Physicochemical properties and skin permeation of Span 60/Tween 60 niosomes of ellagic acid. International Journal of Pharmaceutics 423: 303-311 Google Scholar
- Katona G., Balogh G. T., Dargó G., Gáspár R., Márki Á., Ducza E., Sztojkov-Ivanov A., Tömösi F., Kecskeméti G., Janáky T., Kiss T., Ambrus R., Pallagi E., Szabó-Révész P., Csóka I., et al. (2020) Development of meloxicam-human serum albumin nanoparticles for nose-to-brain delivery via application of a Quality by design approach. Pharmaceutics 12: 97 Google Scholar
- Kawalec P., Śladowska K., Malinowska-Lipień I., Brzostek T., Kózka M.. (2018) European perspective on the management of rheumatoid arthritis: Clinical utility of tofacitinib. Therapeutics and Clinical Risk Management 14: 15-29 Google Scholar
- Khan S., Imran M., Butt T. T., Ali Shah S. W., Sohail M., Malik A., Das S., Thu H. E., Adam A., Hussain Z., et al. (2018) Curcumin based nanomedicines as efficient nanoplatform for treatment of cancer: New developments in reversing cancer drug resistance, rapid internalization, and improved anticancer efficacy. Trends in Food Science and Technology 80: 8-22 Google Scholar
- Kulkarni P., Rawtani D., Barot T.. (2019) Formulation and optimization of long acting dual niosomes using Box-Behnken experimental design method for combinative delivery of ethionamide and D-cycloserine in tuberculosis treatment. Colloids and Surfaces A 565: 131-142 Google Scholar
- Kumar G. P., Rajeshwarrao P.. (2011) Nonionic surfactant vesicular systems for effective drug delivery-An overview. Acta Pharmaceutica Sinica B 1: 208-219 Google Scholar
- Lundquist L. M., Cole S. W., Sikes M. L.. (2014) Efficacy and safety of tofacitinib for treatment of rheumatoid arthritis. World Journal of Orthopedics 5: 504-511 Google Scholar
- Manconi M., Sinico C., Valenti D., Lai F., Fadda A. M.. (2006) Niosomes as carriers for tretinoin. III. A study into the cutaneous delivery of vesicle-incorporated tretinoin. International Journal of Pharmaceutics 311: 11-19 Google Scholar
- Mishra V., Thakur S., Patil A., Shukla A.. (2018) Quality by design (QbD) approaches in current pharmaceutical set-up. Expert Opinion on Drug Delivery 15: 737-758 Google Scholar
- Muzzalupo R., Tavano L., Cassano R., Trombino S., Ferrarelli T., Picci N., et al. (2011) A new approach for the evaluation of niosomes as effective transdermal drug delivery systems. European Journal of Pharmaceutics and Biopharmaceutics 79: 28-35 Google Scholar
- PubChem compound summary for CID 9926791, tofacitinib Google Scholar
- Nayak A. K., Laha B., Sen K. K.. (2011) Development of hydroxyapatite-ciprofloxacin bone-implants using “Quality by design”. Acta Pharmaceutica 61: 25-36 Google Scholar
- Prasad L. K., O’Mary H., Cui Z.. (2015) Nanomedicine delivers promising treatments for rheumatoid arthritis. Nanomedicine 10: 2063-2074 Google Scholar
- Rajana N., Chary P. S., Pooja Y. S., Bhavana V., Singh H., Guru S. K., Singh S. B., Mehra N. K., et al. (2024) Quality by design approach-based fabrication and evaluation of self-nanoemulsifying drug delivery system for improved delivery of venetoclax. Drug Delivery and Translational Research 14: 1277-1300 Google Scholar
- Rungphanichkul N., Nimmannit U., Muangsiri W., Rojsitthisak P.. (2011) Preparation of curcuminoid niosomes for enhancement of skin permeation. Die Pharmazie 66: 570-575 Google Scholar
- Sailaja C., Bhupalam P. K.. (2023) A box Behnken design optimized Nano vesicular transdermal patch for allergies. International Journal of Pharmaceutical Investigation 14: 68-75 Google Scholar
- Shah N., Patel K.. (2023) Formulation, development and characterization of liposome-based gel of eberconazole nitrate for topical delivery. International Journal of Pharmaceutical Investigation 13: 485-495 Google Scholar
- Shen Q., Shu H., Xu X., Shu G., Du Y., Ying X., et al. (2020) Tofacitinib citrate-based liposomes for effective treatment of rheumatoid arthritis. Die Pharmazie 75: 131-135 Google Scholar
- Shi J., Kantoff P. W., Wooster R., Farokhzad O. C.. (2017) Cancer nanomedicine: Progress, challenges and opportunities. Nature Reviews. Cancer 17: 20-37 Google Scholar
- Song G. G., Bae S.-C., Lee Y. H.. (2014) Efficacy and safety of tofacitinib for active rheumatoid arthritis with an inadequate response to methotrexate or disease-modifying antirheumatic drugs: A meta-analysis of randomized controlled trials. The Korean Journal of Internal Medicine 29: 656-663 Google Scholar
- Ulmansky R., Turjeman K., Baru M., Katzavian G., Harel M., Sigal A., Naparstek Y., Barenholz Y., et al. (2012) Glucocorticoids in nano-liposomes administered intravenously and subcutaneously to adjuvant arthritis rats are superior to the free drugs in suppressing arthritis and inflammatory cytokines. Journal of Controlled Release 160: 299-305 Google Scholar
- Wang Q., Jiang J., Chen W., Jiang H., Zhang Z., Sun X., et al. (2016) Targeted delivery of low-dose dexamethasone using PCL-PEG micelles for effective treatment of rheumatoid arthritis. Journal of Controlled Release 230: 64-72 Google Scholar
- Zerbini C.A.F., Lomonte A.B.V.. (2012) Tofacitinib for the treatment of rheumatoid arthritis. Expert Review of Clinical Immunology 8: 319-331 Google Scholar