ABSTRACT
Background
Rheumatoid arthritis causes chronic joint pain and inflammation, significantly impacting quality of life. Current treatments are limited, with oral Leflunomide commonly used. However, prolonged oral administration can lead to severe liver toxicity, posing substantial health risks. To mitigate these adverse effects, a novel targeted drug delivery system is proposed.
Materials and Methods
This research focuses on developing a Microsponge-based gel formulation for leflunomide, aiming to enhance localized drug delivery directly to the affected joints. A 3² full factorial design was employed to optimize and characterize the Microsponge for various parameters, including particle size, entrapment efficiency, and surface morphology. Following optimization, the microsponge was incorporated into a gel formulation. Developed gel was subsequently evaluated based on several parameters.
Results and Discussion
The pH and viscosity were adjusted to ensure skin compatibility and ease of application. Drug content uniformity was verified to ensure consistent dosing. The optimized gel formulation demonstrated a diffusion-controlled drug release profile up to 6 hr, thereby ensuring prolonged therapeutic effect.
Conclusion
This innovative approach seeks to alleviate pain and inflammation while minimizing systemic toxicity, potentially offering a safer and more effective treatment option for rheumatoid arthritis patients.
INTRODUCTION
Leflunomide, an immunomodulatory medication, is mainly prescribed for treating Rheumatoid Arthritis (RA). It works by inhibiting dihydroorotate dehydrogenase, a crucial enzyme in the synthesis of pyrimidine, which is necessary for lymphocyte proliferation. By reducing lymphocyte activity, leflunomide helps to decrease inflammation and slow the progression of arthritis. Administered orally, it is metabolized into its active form, teriflunomide (Junqueira and Bruschi, 2018).
A microsponge is a porous, polymer-based delivery system designed to encapsulate and release active ingredients in a controlled manner. Its unique structure allows for sustained, targeted release, enhancing stability and efficacy, making it ideal for topical applications in pharmaceuticals and cosmetics (Tiwariet al., 2022).
Topical delivery of leflunomide offers a targeted approach for treating rheumatoid arthritis, focusing on local inflammation with reduced systemic side effects. By incorporating leflunomide into a microsponge-based gel, controlled and sustained drug release is achieved, enhancing its therapeutic efficacy at the site of application (Shaikhet al., 2017). This method minimizes gastrointestinal disturbances and hepatotoxicity associated with oral administration. The microsponge system also improves drug stability and penetration through the skin, ensuring effective local drug concentration. This innovative delivery strategy holds promise for improving patient compliance and outcomes in managing rheumatoid arthritis through a localized treatment regimen (Bae and Park, 2016).
Microsponge were prepared by Emulsification-solvent evaporation method. Formulation and process variables were examined through a preliminary study, followed by further optimization using a 32 full factorial design with Design Expert software (Version 11.0.1). The optimized batch of microsponge was then assessed based on various evaluation parameters (Galatage, 2019; Meng and Huang, 2018). Microsponge was incorporated in Carbopol gel and further characterized to evaluate the suitability for topical application.
MATERIALS AND METHODS
Materials
Leflunomide was generously provided by Torrent Pharma Ltd., Ahmedabad, India. Polyvinyl Alcohol, Ethyl Cellulose, and Calcium chloride were sourced from Sulab Chemicals. All other chemicals used in the study were of analytical grade.
Preparation of Microsponge
The microsponges were formulated with leflunomide as the active drug, ethyl cellulose as the polymer, polyvinyl alcohol serving as both stabilizer and emulsifying agent, Calcium chloride as the pore-forming agent, acetone as the solvent, and water as the vehicle.
Emulsification-solvent evaporation method was adopted to prepare Microsponges (Shaikh, 2017). Briefly, the organic phase and aqueous phase were prepared by dissolving weighed amounts of the drug and polymer in acetone; and PVA in water respectively. This organic phase was gradually incorporated to an aqueous phase, with constant stirring. Aqueous phase was previously added with small amount of Calcium chloride as a porogen. The mixture was stirred at 900 rpm for 180 minutes. After the complete evaporation of organic solvent, microsponges were separated by centrifugation and dried (Jain and Singh, 2010; El-Setouhy,et al., 2015; Saad and Sabri, 2023).
In the preliminary study, both formulation and process variables were investigated. The variables selected, as detailed in Table 1; were chosen because they led to the formation of spherical microsponges.
Process variables | Variables | Selected Parameters | ||
---|---|---|---|---|
Stirring speed (rpm) | 800 | 900 | 1000 | 900 |
Stirring Time (min.) | 120 | 180 | 240 | 180 |
Addition rate | 0.5 | 1 | 1.5 | 0.5 |
Formulation variables | ||||
Type of Porogen | Tween 80 | Liq. Paraffin | Calcium Chloride | Calcium Chloride |
Organic solvent | Ethanol | Acetone | Dichloromethane | Acetone |
Screening of Process and formulation variables.
Further optimization was conducted by choosing appropriate Experimental design (Galatage, 2019), with the amount of polymer and the concentration of surfactant as independent variables, and the percentage of drug release and entrapment efficiency as dependent variables.
Thirteen batches were prepared, including four center points to ensure precision (Deviet al., 2020). Different graphs were prepared by using a simplified polynomial equation. An overlay plot was generated to verify the influence of experimental parameters on the results, which is crucial for validating the model’s accuracy.
One percent drug loaded dried microsponge was incorporated in Carbopol Gel. Carbopol gel (2% w/w) was prepared by dispersing accurately weighed amount of Carbopol 934 in required volume of water using magnetic stirrer (Gaoet al., 2019; Nimithaet al., 2017; Gunasheelaet al., 2022). Drug loaded microsponge was added to Carbopol dispersion. Finally, pH was adjusted by dropwise addition of triethanolamine up to 6-6.5.
Characterization-Microsponge
Particle size
Particle size was measured using optical microscopy. The measurements were taken with an eyepiece micrometer that had been previously calibrated with a stage micrometer (Nidhiet al., 2021). Slide was prepared by dispersing few mg of dried microsponge in water and observed under 10X magnification. Randomly 50 particles were observed for particle size.
Surface morphology
SEM reveals detailed images of microsponge shape and surface morphology, giving further insights of structural and textural characteristics (Ammaret al., 2015; Chardeet al., 2013). By coating the microsponges with a conductive material like gold, SEM allows for high-resolution visualization, revealing surface structures and particle characteristics crucial for assessing the formulation’s quality and performance.
% Entrapment Efficiency
Product Yield (PY)
The product yield of the microsponge is calculated by dividing the final weight of the microsponge obtained by the initial weight of the raw materials used, multiplied by 100 to express it as a percentage (Aldawsari and Badr-Eldin, 2013). This measurement provides an indication of the efficiency of the method, reflecting how much of the intended product was successfully produced relative to the starting materials.
In vitro drug release
Previously soaked desired sized dialysis membrane was tied to diffusion cell. In donor compartment, 1 g of microsponge dispersed in 5 mL of methanolic PBS pH 5.5 was placed (Gaikwad et al., 2024). 100 mL of methanolic PBS pH 5.5 was placed in receptor compartment, positioned on a magnetic stirrer at a temperature 37°C ± 0.5°C. The amount of released drug was quantified spectrophotometrically at 259 nm.
Gel- Organoleptic Property
pH
Washability
Spreadability
Spreadability of gel shows effortlessly covering the skin surface with minimal effort. Gel with smooth texture shows even distribution without clumping or streaking, ensuring a consistent application (Nurmanet al., 2019). The spreadability of a leflunomide-loaded microsponge gel was determined by placing 5 g of the gel on the lower wooden block. The time taken for the upper movable glass slide to move 5 cm away from lower block was recorded. The Spreadability coefficient (S) was determined using the formula,
Where S – Spreadability coefficient, g-cm/s; M- weight placed on the Pan, L- distance moved by the slide, and T – time taken by the slide to move.
Viscosity
The viscosity was measured using the Brookfield Viscometer (DV-III Ultra, AMETEK Brookfield) at a temperature of 25±5°C. 100 g sample of accurately weighed leflunomide-loaded microsponge gel was used, employing a helipath T-F 96 spindle (Sancheset al., 2023). Furthermore, the rheological behaviour of gel was evaluated at different rotational speeds.
Drug Content
The drug content was determined by dissolving the leflunomide-loaded microsponge topical gel in phosphate buffer (pH 5.5). The concentration of leflunomide was then measured spectrophotometrically at 259 nm.
The drug content of the gel was evaluated by dissolving the gel in a phosphate buffer pH 5.5. This method ensures the accurate determination of the leflunomide concentration within the gel, confirming the consistency and effectiveness of the formulation for therapeutic use.
Drug release
The drug release of the gel was measured by using a diffusion cell apparatus where the dispersion of gel in 5 mL of methanolic Phosphate buffer was placed in donor compartment and 100 mL of dispersion medium was placed in receptor compartment and assembly was set on magnetic stirrer. Samples were collected at specified intervals, and the concentration of leflunomide was measured using an appropriate analytical method (Borseet al., 2020; Tiwariet al., 2021).
Stability study
Leflunomide loaded Microsponge gel formulation was subjected to stability study. The optimized gel was placed in sealed wide mouth container and stored at ICH storage condition (30 ± 2ºC/60% ± 5% RH, 40 ± 2ºC/75% ± 5% RH) for 30 days. The samples were analyzed for appearance, clarity, pH, viscosity and drug release (Aiyaluet al., 2016).
RESULTS AND DISCUSSION
Microsponge based gel was optimized by 32 full factorial design. Thirteen batches were prepared according to the Design Expert software (Table 2), and the resulting data were analyzed to derive the following polynomial equation.
Batch no. | A: Amount of EC | B: Concentration of Surfactant | Drug release (%) | Entrapment Efficiency (%) |
---|---|---|---|---|
1 | 150 | 0.75 | 78.66 | 73.73 |
2 | 175 | 0.75 | 89.9 | 79.97 |
3 | 200 | 0.75 | 85.23 | 78.76 |
4 | 150 | 1 | 83.39 | 77.16 |
5 | 175 | 1 | 94.56 | 83.2 |
6 | 200 | 1 | 90.47 | 81.3 |
7 | 150 | 1.25 | 81.25 | 79.65 |
8 | 175 | 1.25 | 92.11 | 85 |
9 | 200 | 1.25 | 88.09 | 83.15 |
10 | 175 | 1 | 95.1 | 83.92 |
11 | 175 | 1 | 94.15 | 83.69 |
12 | 175 | 1 | 94.59 | 83.2 |
13 | 175 | 1 | 93.92 | 83.11 |
Influence of formulation variables on responses.
Polynomial equation for % Drug release,
Polynomial equation for % Entrapment Efficiency,
The magnitude and sign of the coefficients indicate the effect of chosen independent variables on responses. The software suggested an optimized batch based on selected constraints. To validate the chosen model, a checkpoint analysis was performed.
Effect of Predictor variables on % Drug release
The independent variable, the amount of Ethyl Cellulose, directly impacts the dependent variable, the % drug release of Microspheres. When the Polymer amount increases from 150 mg to 175 mg, the % drug release also increases. However, beyond 175 mg, the % drug release of Microspheres decreases (Figure 1). From the experimental values, it can be concluded that increasing the amount of polymer leads to increase in drug release up to some extent, but beyond that drug release was observed decreased, may be due to matrix formation hinder the release of the drug. The 3D response surface plot indicates that increasing the surfactant concentration promotes the % drug release by sufficiently lowering the interfacial tension.
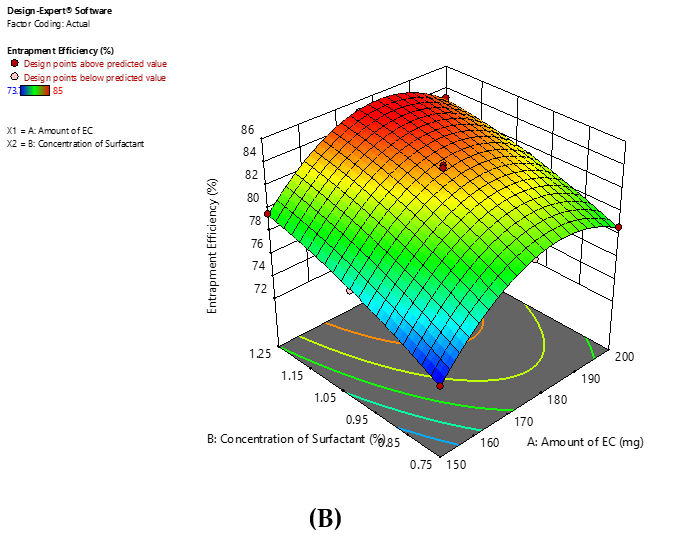
Figure 1:
Response surface plot for (A) %Drug release, (B) %Entrapment Efficiency.
Effect of Predictor variables on % Entrapment Efficiency
From the 3D surface plots, it can be concluded that Amount of Polymer is directly proportional to % Entrapment efficiency. Further increase in amount of polymer does not improve % EE as maximum amount of drug has been entrapped. It has observed that higher amount of polymer lead to decrease in % EE may be due to greater viscosity of internal phase.
Increasing the concentration of surfactant enhances the % EE due to sufficient reduction of interfacial tension.
Based on the response surface plots, it is evident that the amount of polymer is directly proportional to the percentage of Entrapment Efficiency (% EE) (Figure 1). However, after reaching a certain point, increasing the polymer amount further does not enhance % EE, as the maximum drug entrapment has been achieved. It is noted that a higher polymer amount can actually reduce % EE, likely because of the increased viscosity of the internal phase. Additionally, increasing the concentration of surfactant boosts % EE by effectively reducing the interfacial tension.
Optimized batch of Microsponge was selected based on constraints for desirable responses. Yellow region of Overlay plot (Figure 2) indicates the range of predictable batches which can be further validated by selecting few points for check point analysis (Table 3). Batches were selected based on desirability close to 1.
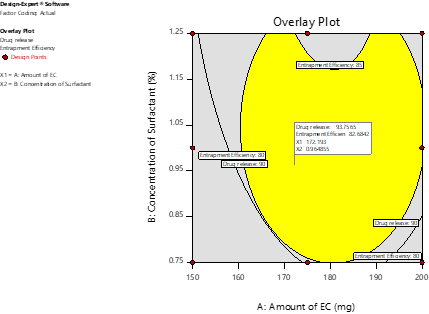
Figure 2:
Overlay Plot.
X1 | X2 | Observed | Predicted | %Error | |||
---|---|---|---|---|---|---|---|
%Drug release (6 hrs) | %Entrapment Efficiency | %Drug release (6 hrs) | %Entrapment Efficiency | %Drug release (6 hrs) | %Entrapment Efficiency | ||
200 | 1 | 90.29 | 81.52 | 91.18 | 80.24 | -0.99 | 1.57 |
175 | 1 | 94.48 | 83.35 | 96.21 | 81.65 | -1.83 | 2.04 |
170 | 1.15 | 92.99 | 84.10 | 90.45 | 85.69 | 2.73 | -1.89 |
172.2 | 0.96 | 93.75 | 82.68 | 91.65 | 80.98 | 2.24 | 2.06 |
164.2 | 0.981 | 91.49 | 81.50 | 92.34 | 83.14 | -0.93 | -2.01 |
Check point analysis of Leflunomide Microsponge.
Characterization-Microsponge
Particle Size
Using optical microscopy, particle size of prepared microsponge was measured under 10X magnification. Mean particle size of microsponge was found to be 26.98 μm (Table 4).
No. of divisions covered on Eyepiece micrometer | No. of particles of microsponge | Diameter of microsponge (no. of divisions * calibration factor) (µm) | Particle size (µm) |
---|---|---|---|
1 | 19 | 19*14.2 | 269.8 |
2 | 17 | 17*28.4 | 482.8 |
3 | 14 | 14*42.6 | 596.4 |
Average Particle size (µm) | 1349/50=26.98 |
Particle size measurement.
Surface morphology
The surface morphology of the microsponge, examined via scanning electron microscope (Figure 3), revealed a spherical structure with numerous tiny pores. This porous surface is characteristic of microsponge formulations, aiding in effective drug delivery by providing a larger surface area for interaction and sustained release of the active ingredient.
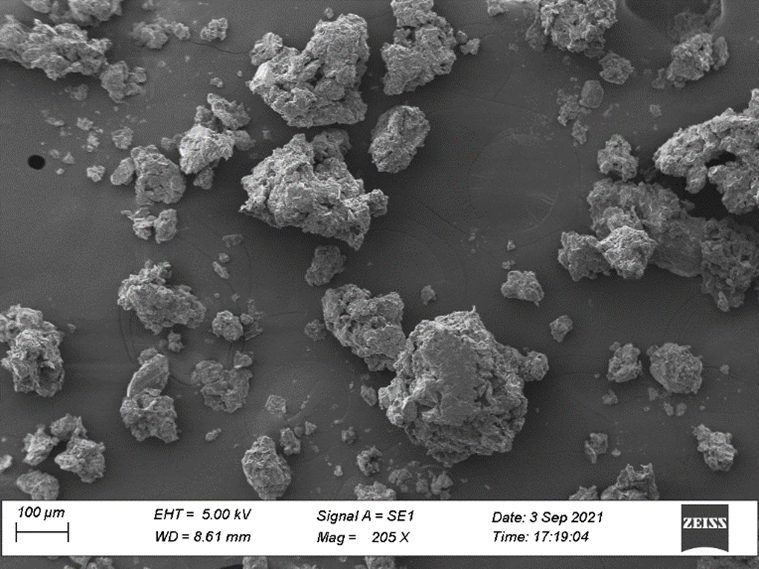
Figure 3:
SEM of Microsponge.
%Production yield
%Production yield was found to be 80%. %Yield was found to be increased with increase an amount of polymer and also increased with increase in amount of surfactant due to sufficient lowering of interfacial tension.
% Entrapment Efficiency
Entrapment efficiency was found to be in the range of 73.73-83.92%. % EE as found to be increased with increase in amount of polymer as shown in Figure 1.
In vitro drug release
The percentage of drug release ranged from 81.25% to 95.1%, as illustrated in the figure. This range indicates the extent of drug release from the formulation over a specified period, crucial for determining its release kinetics and potential therapeutic effectiveness. Drug release kinetic showed to follow Higuchi model.
Gel
Organoleptic characteristics
The organoleptic properties of the gel were assessed, showing an off-white colour, odourless and smooth texture. These attributes are important indicators of aesthetic quality and user acceptability of gel, ensuring it meets sensory expectations for pharmaceutical or cosmetic applications.
pH
The pH of aqueous solution was found within a range of 5.54 ± 0.85, which closely matches the natural pH of the skin. This acidic pH level is crucial as it indicates compatibility with the protective barrier, minimizing the risk of irritation. Maintaining this pH range ensures that the gel formulation is well-suited for topical application, enhancing its effectiveness and safety for use in dermatological application.
Washability
The gel applied to the dorsal side of the hand was easily washed out, even after drying, by exposing the hand to running water. This demonstrates the effective washability of gel, ensuring it can be readily removed from the skin.
Spreadability
Spreadability of the gel was evaluated using a specially designed apparatus, showing a measured range of 20.83% ± 3.89%. This parameter indicates the ease and extent to which the gel can spread on the surface of skin. Optimal Spreadability is desirable for ensuring uniform application and coverage, enhancing user experience and efficacy in dermatological or cosmetic applications.
Viscosity
The formulation was subjected to shear at speeds ranging from 10 to 100 rpm using spindle no. 96 at 25℃. The average viscosity measured was 9580 cps (centipoise).
Drug content
UV spectroscopy was employed to analyse the Leflunomide content per gram of gel. The drug content ranged from 99.12% to 96.61% across three replicates.
Drug release
Figure 4 depicted an initial burst release, which was attributed to the presence of unentrapped drug within the three-dimensional structure of the gel. Drug release data were analyzed using various kinetic models, confirming that the release followed the Higuchi model. This model suggests that the drug release from the formulation is primarily controlled by diffusion processes within the gel matrix.
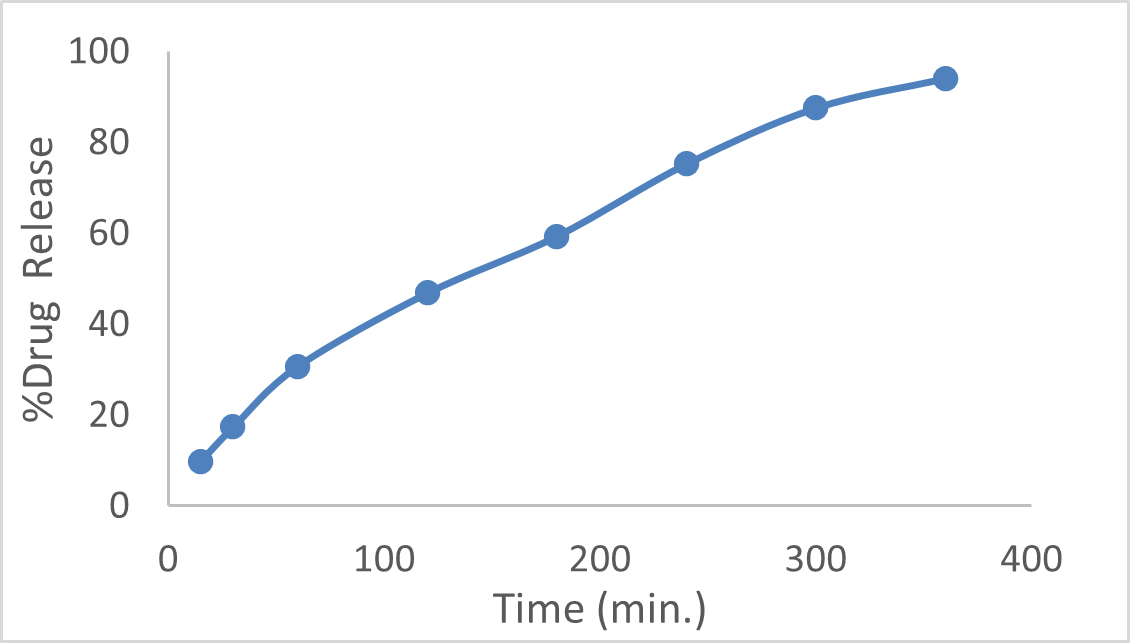
Figure 4:
In vitro Drug Release.
Stability study
A stability study was conducted following ICH guidelines, revealing no significant changes in the physical appearance, rheological behaviour, or drug release of the formulation (Table 5). Therefore, the formulation is deemed stable under the specified storage conditions.
Sl. No. | Parameter | Before storage | After 1 month storage |
---|---|---|---|
1 | pH | 5.54 ± 0.01 | 5.54 ± 0.01 |
2 | Appearance | White, Homogenous, Smooth | White, Homogenous, Smooth |
3 | In vitro drug release | 94.6 % | 93.2 % |
Stability Study.
DISCUSSION
An optimized batch of microsponge was successfully developed using a 32 full factorial design, resulting in a uniform particle size and a spherical morphology. The preparation method employed demonstrated a high production yield of the microsponge. The formulated microsponge showed satisfactory drug entrapment efficiency and controlled drug release profiles. The microsponge-loaded gel exhibited desirable rheological properties, including appropriate viscosity and spreadability, making it suitable for topical application. Additionally, the gel formulation facilitated a diffusion-controlled release of the encapsulated drug, supporting its potential for effective skin delivery.
CONCLUSION
Leflunomide, an anti-rheumatoid agent used in the treatment of Rheumatoid arthritis, was successfully formulated as microsponge loaded topical gel. Microsponge were prepared by solvent evaporation method. Few process and formulation variables were kept constant for further optimization of microsponge using 32 full factorial design. Microsponge were characterized and further optimized batch of microsponge was incorporated in Carbopol gel. Viscosity and drug content of developed Gel was found to be satisfactory. Developed formulation exhibited drug release for 6 hr, at the site of application, avoiding side effects caused by oral administration.
Hence, it can be concluded that Leflunomide loaded microsponge gel can be a better drug delivery system as compared to currently available conventional dosage forms.
Cite this article:
Gohil D, Patel U, Patel P, Shah N, Kardani S. Optimizing Leflunomide Embedded Microsponge Gel for Effective Topical Application. Int. J. Pharm. Investigation. 2025;15(2):10-8.
ABBREVIATIONS
RA | Rheumatoid Arthritis |
---|---|
PVA | Polyvinyl alcohol |
SEM | Scanning Electron Microscope |
PY | Production Yield |
PBS | Phosphate Buffer Saline |
3D | Three dimensional |
EE | Entrapment Efficiency |
ICH | International Conference on Harmonization |
RH | Relative Humidity |
References
- Aiyalu R., Govindarjan A., Ramasamy A.. (2016) Formulation and evaluation of topical herbal gel for the treatment of arthritis in animal model. Brazilian Journal of Pharmaceutical Sciences 52: 493-507 Google Scholar
- Aldawsari H., Badr-Eldin S.. (2013) Microsponges as promising vehicle for drug delivery and targeting: Preparation, characterization and applications. African Journal of Pharmacy and Pharmacology 7: 873-881 Google Scholar
- Alhamhoom Y., Honmane S. M., Hani U., Osmani R. A. M., Kandasamy G., Vasudevan R., Paramshetti S., R Dudhal R., K Kengar N., Charde M. S., et al. (2023) Study of formulation and process variables for optimization of piroxicam nanosuspension using 32 factorial design to improve solubility and in vitro bioavailability. Polymers 15: 483 Google Scholar
- Ammar A. A., Eladawy S. A., Elosaily G. H., Amin O. M.. (2015) Formulation and in vitro evaluation of leflunomide oral tablet with enhanced dissolution. Journal of American Science 11: 140-153 Google Scholar
- Bae J., Park J. W.. (2016) Topical delivery of leflunomide for rheumatoid arthritis treatment: Evaluation of local tissue deposition of teriflunomide and its anti-inflammatory effects in an arthritis rat model. Drug Development and Industrial Pharmacy 42: 254-262 Google Scholar
- Borse V. A., Gangude A. B., Deore A. B.. (2020) Formulation and evaluation of antibacterial topical gel of doxycycline hyclate, neem oil and tea tree oil. Indian Journal of Pharmaceutical Education and Research 54: 206-212 Google Scholar
- Charde M. S., Ghanawat P. B., Welankiwar A. S., Kumar J., Chakole R. D.. (2013) Microsponge A novel new drug delivery system: A review. International Journal of Advances in Pharmaceutics 2: 63-70 Google Scholar
- Devi N., Kumar S., Prasad M., Rao R.. (2020) Eudragit RS100 based microsponges for dermal delivery of clobetasol propionate in psoriasis management. Journal of Drug Delivery Science and Technology 55: 101347 Google Scholar
- El-Setouhy D. A., Abdelmalak N. S., Anis S. E., Louis D.. (2015) Leflunomide biodegradable microspheres intended for intra-articular administration: Development, anti-inflammatory activity and histopathological studies. International Journal of Pharmaceutics 495: 664-670 Google Scholar
- Galatage S. T.. (2019) Development and characterization of microsponge of amphotericin B for topical drug delivery. Research Journal of Pharmaceutical, Biological and Chemical Sciences 10: 1288-1300 Google Scholar
- Gao S., Tian B., Han J., Zhang J., Shi Y., Lv Q., Li K., et al. (2019) Enhanced transdermal delivery of lornoxicam by nanostructured lipid carrier gels modified with polyarginine peptide for treatment of carrageenan-induced rat paw edema. International Journal of Nanomedicine 14: 6135-6150 Google Scholar
- Gunasheela S., Chandrakala V., Srinivasan S.. (2022) Microsponge: An adaptable topical drug delivery system. World Journal of Advanced Research and Reviews 15: 396-411 Google Scholar
- Jain N., Sharma P., Banik A.. (2011) Recent advances on microsponge delivery system. International Journal of Pharmaceutical Sciences Review and Research 8: 13-23 Google Scholar
- Jain V., Singh R.. (2010) Development and characterization of eudragit RS 100 loaded microsponges and its colonic delivery using natural polysaccharides. Acta Poloniae Pharmaceutica 67: 407-415 Google Scholar
- Junqueira M. V., Bruschi M. L.. (2018) A review about the drug delivery from microsponges. AAPS PharmSciTech 19: 1501-1511 Google Scholar
- Kadhim Z. M., Mahmood H. S., Alaayedi M., Ghareeb M. M.. (2020) Formulation of flurbiprofen as microsponge drug delivery system. International Journal of Pharmaceutical Research 12: 748-753 Google Scholar
- Meng Z., Huang R.. (2018) Topical treatment of degenerative knee osteoarthritis. The American Journal of the Medical Sciences 355: 6-12 Google Scholar
- Nidhi K., Verma S., Kumar S.. (2021) Microsponge: An advanced drug delivery system. Journal of Clinical and Scientific Research 10: 108-111 Google Scholar
- Nimitha V. V., Parvathy S., Nair S. C., Viswanad V.. (2017) Leflunomide loaded solid lipid nanoparticle in rheumatoid arthritis. International Journal of Pharmacy and Technology 9: 29681-29706 Google Scholar
- Nurman S., Yulia R., Irmayanti , Noor E., Candra Sunarti T.. (2019) The optimization of gel preparations using the active compounds of arabica coffee ground nanoparticles. Scientia Pharmaceutica 87: 32 Google Scholar
- Raviraj G., Kale A., Rajashree C., Ashok B.. (2024) Formulation and evaluation of flurbiprofen microsponges tablet for effective treatment of rheumatoid arthritis. Journal of Chemical Health Risks 14: 654-665 Google Scholar
- Saad A., Sabri L.. (2023) Study the variables affecting formulation of ethylcellulose-based microsponges loaded with clobetasol. Iraqi Journal of Pharmaceutical Sciences (P-ISSN 1683 – 3597 E-ISSN 2521 – 3512) 32: 225-234 Google Scholar
- Sanches S. C. D. C., Ré M. I., Silva-Júnior J. O. C., Ribeiro-Costa R. M.. (2023) Organogel of Acai Oil in Cosmetics: Microstructure, Stability, Rheology and Mechanical Properties. Gels 9: 150 Google Scholar
- Shaikh A. A., Chaudhari P. D., Holkar S. S.. (2017) A design of experiment approach for optimisation and characterization of etodolac ternary system using spray drying. International Journal of Pharmacy and Pharmaceutical Sciences 9: 233-240 Google Scholar
- Shaikh A. A., Chaudhari P. D., Lavate P. K.. (2017) Central composite design for enhancement of etodolac solubility via inclusion complexation with PVP k-30 and hydroxypropyl β–Cyclodextrin. European Journal of Pharmaceutical and Medical Research 4: 657-663 Google Scholar
- Singh J. A., Saag K. G., Bridges S. L., Akl E. A., Bannuru R. R., Sullivan M. C., Vaysbrot E., McNaughton C., Osani M., Shmerling R. H., Curtis J. R., Furst D. E., Parks D., Kavanaugh A., O’Dell J., King C., Leong A., Matteson E. L., Schousboe J. T., McAlindon T., et al. (2016) 2015 American College of Rheumatology guideline for the treatment of rheumatoid arthritis. Arthritis and Rheumatology 68: 1-26 Google Scholar
- Tiwari A., Bag P., Sarkar M., Chawla V., Chawla P. A.. (2021) Formulation, validation and evaluation studies on metaxalone and diclofenac potassium topical gel. Environmental Analysis, Health and Toxicology 36: e2021001 Google Scholar
- Tiwari A., Tiwari V., Palaria B., Kumar M., Kaushik D.. (2022) Microsponges: A breakthrough tool in pharmaceutical research. Future Journal of Pharmaceutical Sciences 8: 31 Google Scholar