ABSTRACT
Surgical intestinal wound healing is a significant cellular process managed by several cellular and molecular factors. The present review aims to focus on the evolution of medical device bandages that can help improve the healing of intestinal injuries in patients who undergo gastrointestinal surgery or suffer from IBD. Restitution, proliferation and differentiation of IECs are critical for wound healing in the gut and restoration of barrier function. For example, patients with diabetes develop poor wound healing and associated consequences such as anastomotic leak or fistula formation. These challenges are discussed in this review to show how smart and bioelectronic bandages can help to overcome these by offering the necessary healing conditions through moisture, monitoring and drug release. For instance, smart bandages covered with sensors and bioelectric bandages open unique therapeutic perspectives due to the stimulation of cell migration and anti-inflammatory action. These innovations are much better than the conventional bandages, for they hold the potential in improving clinical outcome of wounds. Further research should be directed at working on the practicality, affordability and sustained clinical effectiveness of such functional bandages so that they can be incorporated in daily practice.
INTRODUCTION
Intestinal wound healing is a healthy physiologic response to restore the injured intestinal mucosa and enteral nutrition (Iizuka, 2011). The damage is not only caused by surgical procedures but also by other diseases such as inflammatory bowel diseases and trauma (Liuet al., 2021). Healing takes place mainly in three stages: restitution, proliferation and differentiation of IECs (Intestinal Epithelial Cells) coming into contact with the wound area (Sommeret al., 2021). The epithelial cells cover the wound rapidly through restitution within hour from the injury. Then follows proliferation, which synthesizes the extra cells needed to replace the epithelial layer and finally differentiation, which ensures barrier function and homeostasis in the gut (Iismaaet al., 2018).
Effective wound healing after gastrointestinal surgery and disease is highly critical because impaired wound healing may compromise intestinal surgery with significant complications, such as potential anastomotic leaks and fistula formation, thus increasing morbidity (Girardet al., 2014). Impaired mucosal healing in these patients with IBD (Inflammatory Bowel Diseases) worsens disease symptoms and complicates surgical outcomes (Wanget al., 2023). Therefore, it is essential to understand the mechanisms of intestinal wound healing to develop targeted therapeutic strategies optimizing recovery and enhancing patient outcomes.
The latest and most innovative tools are the medical device bandages used in improving the process of healing the intestinal wound. Such high-tech dressings protect the wound with an ideal microenvironment favoring healing by retaining moisture and delivering bioactive agents directly to the wound site (Derakhshandehet al., 2018). The latest innovation in bandage development is a sensorized smart bandage, updating continuously in real-time, on the status of the wound, thereby enabling personally tailored adjustment of treatment procedures according to specific patient needs (Hossainet al., 2024). Besides, bioelectric bandages apply electrical stimulation for cell stimulation, which accelerates healing rates. It also holds true when discussing the matter of medical device bandages due to the reason that these innovations open channels for a remarkable improvement in clinical outcomes when patients undergo surgery for gastrointestinal areas or if they are affected by chronic intestinal diseases (Kloth, 2013).
In this review, the processes of healing the injuries in the intestine will be described and, in addition, several existing types of bandages for medical devices will be considered concerning their applicability and effectiveness on the clinic.
Wound Healing in the Intestine
Cell behaviour in wound healing
Proliferation and differentiation of Intestinal Epithelial Cells (IECs), restitution
Intestinal wound healing is an active, organized response that begins instantly on injury (Dignasset al., 2004). The first critical step is restitution, in which surviving IECs move to cover the denuded mucosal surface, flatten and extend over the wound to provide a provisional barrier preventing further insult from luminal contents (IMEMP, 1992). After restitution, proliferation can occur with stem cells located at the crypt base proliferating to replace the lost cells (Axel and Podolsky, 1993; Dignasset al., 1994). These proliferating cells eventually differentiate to regain the functional activities of mucosa, including the absorption and secretion of mucus (Dignasset al., 1994). In case any of these processes are delayed or defective, healing is compromised, hence demanding a delicate interplay between these processes (Dignasset al., 1994).
Role of growth factors and cytokines in the healing process
Some of these factors include Epidermal Growth Factor (EGF), Transforming Growth Factor-Beta (TGF-β) and Fibroblast Growth Factor (FGF) (Ohnedaet al., 1997; Parket al., 1992). These factors play crucial roles in the induction and even guiding of the repair process in the intestines, such as Interleukin-10 (IL-10) and Tumor Necrosis Factor-alpha (TNF-α). These cytokines are involved in a range of cellular processes, including migration and proliferation and also possess immunomodulatory functions. For instance, EGF has been established to cause IEC migration and proliferation as well as support wound healing. Conversely, TGF-β limits over-inflammation and encourages matrix deposition in the wound, which acts as scaffolding for repair (Ciacciet al., 1993; Dignass and Podolsky, 1996; Pacliket al., 2008; Bénédicteet al., 2007; Bhattacharyaet al., 2004).
Factors Influencing Healing
Neuropeptides and neurohumors
Healing of the intestine and mucosa is a vital and a more complicated process as compared to cell wound healing and also does involve richment of regulatory peptides and intracellular signaling. With these peptides are the Trefoil Factor Family Peptides (TFFs) peptides (Dignasset al., 1994), that promote epithelial integrity; and Vascular Endothelial Growth Factor (VEGF) to induce angiogenesis. The Wnt/β-catenin, Notch and PI3K/Akt signaling pathways coordinate this healing process (Iizukaet al., 2007). For instance, the Wnt/β-catenin pathway causes the proliferation and migration of epithelial cells, while Notch signaling is involved in the differentiation of stem cells into functional epithelial cells.
Impact of dietary factors, such as glutamine, on mucosal healing
Dietary factors are considered the fundamental principles for mucosal healing (Yamamotoet al., 2007). Among the most studied nutrients is the amino acid glutamine, important for the effective functioning of enterocytes (Smith, 2008; YAMAMOTOet al., 2006). Glutamine influences improved gut barrier function, modulates immune responses and accelerates the proliferation of IECs (Larsonet al., 2007; https://pubmed.ncbi.nlm.nih.gov/4605420/, 1974). It has been observed when patients are suffering from inflammatory bowel disease or had undergone intestinal surgery taking glutamine can support intestinal mucosal healing and general well-being (Sonet al., 2005).
The role of microbiota and toll-like receptors for the regulation of gut health
The gut microbiota is both involved in the regulation of gut physiological processes including homeostasis and wound healing (Carioet al., 2007).
Gut bacteria have their interaction with the host through the Toll-Like Receptors (TLRs), understanding microbial components and eventually initiating an immune response. Among the published works, it was reported that TLR2 and TLR4 mediate inflammatory responses and promote repair following injury (Eyet al., 2009; Masayuki et al., 2005). An equilibrated microbial environment tends to create an anti-inflammatory setting allowing repair to proceed; conversely, dysbiosis can impair healing processes as it leads to chronic inflammation and prevents proper recovery (Podolskyet al., 2009; Rakoff-Nahoumet al., 2006). The mechanism of wound healing was described in Figure 1.
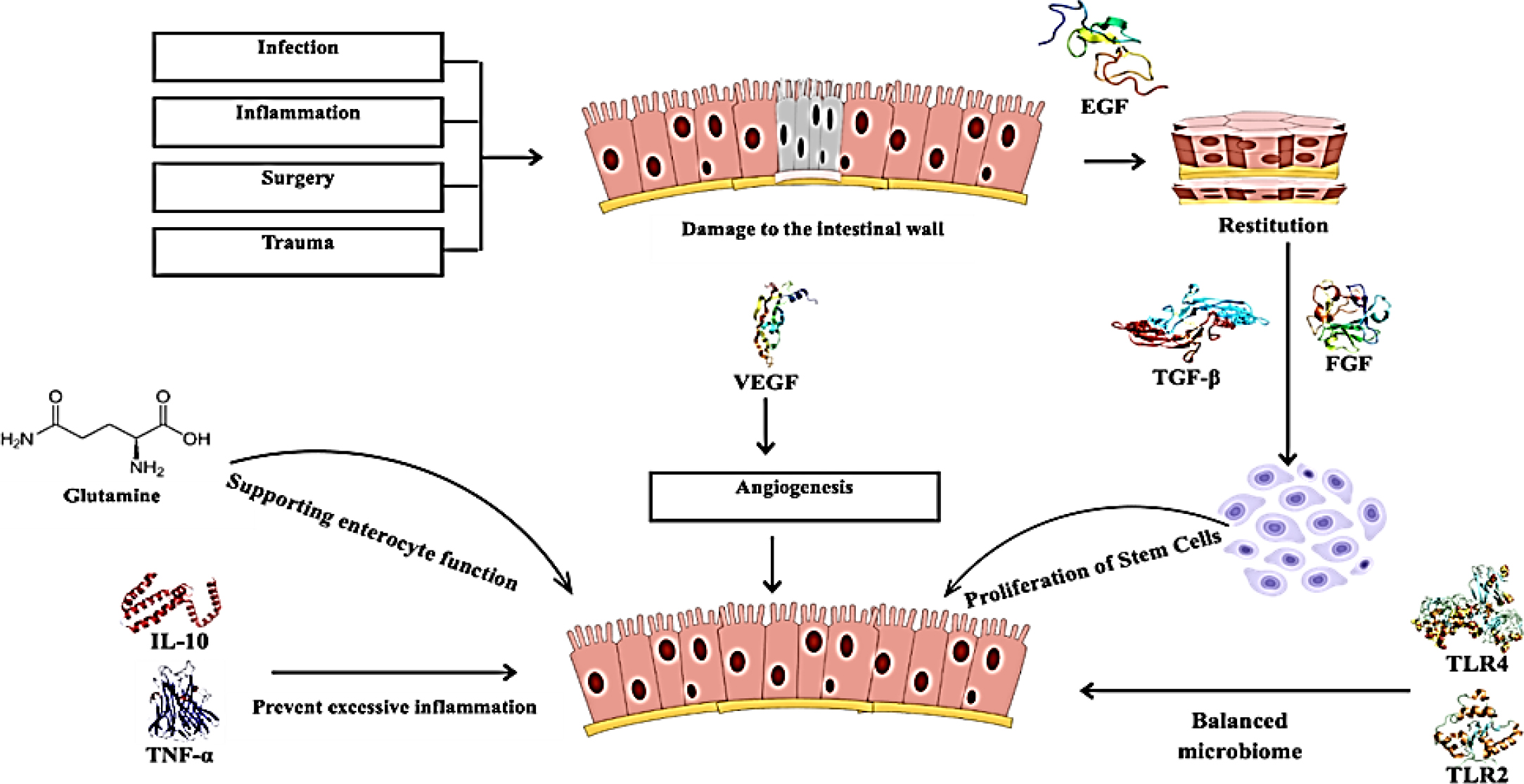
Figure 1:
Mechanisms of Intestinal Wound Healing.
Types of Medical Device Bandages
Traditional Bandages
Composition and applications
Traditional bandages are based on the lowest consumable material like cotton, gauze and adhesive materials and have been part and parcel of wound care for centuries. These bandages have been used to constitute a mere physical barrier against superficial contaminants while offering a moist environment that is conducive to healing, most of all in relation to intestinal wound healing. They achieve this by being placed after surgery about incision sites from outside in protection against infections, leading to lower rates of complications. Traditionally, bandages have a very poor biological or technological functionality, apart from creating protective coverage of the wound and absorption of exudates (Joneset al., 2006). The various types of bandages were described in Table 1.
Aspect | Traditional Bandages | Smart Bandages | Bioelectronic Bandages |
---|---|---|---|
Composition | Cotton, gauze, adhesive materials. | Sensors, electronic components, advanced materials. | Bioresorbable materials (e.g., magnesium, silk). |
Primary Function | Physical barrier, moisture retention. | Monitor wound parameters, drug delivery. | Electro-stimulation to enhance healing. |
Mechanism of Action | Passive protection against contaminants. | Real-time monitoring and adaptive treatment. | Controlled electrical currents to stimulate cellular activities. |
Monitoring Capabilities | Manual observation required. | Wireless data transfer to monitor wound status. | Continuous monitoring with biofeedback. |
Clinical Efficacy | Limited; primarily passive. | Improved healing rates, reduced inflammation and infection. | Faster healing rates, reduced scar formation. |
Limitations | Inadequate for complex internal wounds. | Higher costs, requires technological infrastructure. | Expensive, potential adverse reactions in sensitive populations. |
Examples of Use | Standard post-surgical applications. | Hydrogel-based smart bandages. | Bioresorbable electronic devices for intestinal wounds. |
Types of Medical Device Bandages.
Limitations in intestinal wound healing
The main challenges with traditional bandages when applied to internal or intestinal wound healing include the failure to support tissue repair by promoting the necessary conditions for healing or monitoring the status of the wound. They are inappropriate for the specialized complex conditions within the gastrointestinal tract. Besides, their inability to alter moisture levels, pH and bacterial load of the wound can lead to problems concerning infection or delayed healing. These bandages rely significantly on repeated observation and manual readjustments, which may be invasive and lead to contamination (Soodet al., 2014).
Smart Bandages
An outline definition and the presentation of the related technologies
Smart bandages are the modern wound care products which have been developed to eliminate the variety of limitations that are associated with regular bandages. They are integrated with sensing and electronic elements that can measure for a variety of factors in a wound, for instance, the temperature, pH level, humidity or any biomarker at all. This action is more efficient and controlled; they can also change the nature of the wound and deliver drugs or therapeutic agents in accordance with the changing conditions (Jianget al., 2022).
Mounting of sensors for assessment of conditions of the wound
It is possible to attach different kinds of sensors to smart bandages helping a wound status assessment in real-time. For example, pH sensors will allow the measurement of acidity levels in the wound, sometimes considered an indicator of bacterial infection or not healing appropriately. Temperature sensors can facilitate the early identification of signs of inflammation or infection. These sensors wirelessly transfer data to an external monitoring system and providers can monitor healing without interfering with the wound, which significantly reduces dressing changes and in-office follow-up (Derakhshandehet al., 2018; Hossainet al., 2024).
Examples of smart bandages and their mechanisms of action
One of the most known examples is a hydrogel-based smart bandage embedded with microelectronic sensors. These bandages can detect the fluctuations of the temperature at the wound site and respond by releasing therapeutic agents. Other examples are bandage that is made of a layer that can release antibiotics once a growth of bacteria is realized through a sensor analysis. They are used to somewhat cut out the external action and make the healing process more self-governing (Shirzaeiet al., 2023).
Bioelectronic Bandages
Explanations of the bioresorbable electronic bandages
Bio electronic bandages constitute a novel class of wound healing devices that is rapidly emerging in the current technological frame work. The electronics involved are biodegradable meaning they will melt in the body once they have served their purpose hence do not need to be removed.
Composed of materials such as magnesium and silk, these will interact with the wound electrically and biologically to optimize the repair process (Nature Electronics, 2024). Its bio resorptive nature is great for sensitive areas like the intestines due to the fact that existing devices have to be extracted through a surgical process, causing damage during the retrieval process (Wuet al., 2024).
The principles of electrostimulation treatments and their influence on recovery
The way that the bio electronic bandage’s function can be described as a type of bio monitoring of the status of the wound and electro stimulation of cells and it has been figured out that the application of small and controllable electric currents at the location of the injured tissue is capable of stimulating the critical series of cell activities that are necessary for the wound healing process. In experiments, it has been proven that electro stimulation helps not only fibroblasts and keratinocyte displacement but also accelerates the rate of wound healing and their closure (Chenet al., 2023). In addition; this technology may effectively reduce inflammation and the activation of immune cells at an injured site.
Recent advancements and studies demonstrating efficacy
Bio electronic bandages have proved as a promising method to heal wounds faster. For instance, if compared with traditional treatments, 30% more rate of healing is obtained if bio electronic bandages are given. Furthermore, the area of materials science has advanced with the development of stretchable and flexible bioelectronics that can shape precisely onto the wound site for offering more accurate stimulation and minimizing discomfort to the patient (College of Medicine, 2023).
Clinical Applications and Efficacy
Case Studies and Clinical Trials
Several clinical trials and cases have cited the evidence of success of medical device bandages in terms of healing intestinal wounds. The findings and effectiveness of clinical trials were described in Table 2.
Authors | Study | Findings | Effectiveness |
---|---|---|---|
(Wu, H.et al., 2024) | Flexible biodegradable electronic bandage for intestinal wound healing. | Developed a self-powered bandage that delivers dual-mode electrical stimulation to accelerate local intestinal wound healing. Shows promise for reducing postoperative complications. | Synergistically accelerates healing; potential application in other tissues and organs. |
(Ameer, G.A. et al., 2023) | Stretchable electrotherapy bandage for accelerated healing. | Created a small, flexible bandage that delivers electrotherapy directly to the wound site. In an animal study, it healed diabetic ulcers 30% faster than controls. The bandage actively monitors healing and dissolves harmlessly after use. | Accelerates healing by 30%; enhances monitoring of the healing process; biodegradable post-use. |
Case Studies and Clinical Trials.
Comparative analysis of outcomes between traditional and advanced bandages
Traditional dressings are widely applied, but they work more like passive barriers, providing a shielding effect to the wound dressing (Chen and Peng, 2023; Rezvaniet al., 2019). However, their frequency of monitoring and replacement is very high (Brittoet al., 2024). On the contrary, smart and bio electronic dressings are active promoters of healing wounds and optimize the cellular regenerative environment as depicted in clinical trials (Panget al., 2023). The comparative analysis were described in Table 3.
Study | Description |
---|---|
(Heyeret al., 2013) | This systematic review and meta-analysis evaluated the effectiveness of advanced versus conventional wound dressings on chronic wound healing. The study found that advanced dressings had a mean odds ratio of complete healing at 1.52, indicating a significant advantage over conventional dressings. |
(Ehtesabiet al., 2022) | This mini-review discusses the role of smart phone-based wound dressings, highlighting their advantages over traditional methods, including real-time monitoring and targeted treatment, which can enhance healing outcomes. |
(Soodet al., 2014) | This article discusses various advanced wound dressings and their comparative effectiveness. It emphasizes the need for more high-quality randomized controlled trials to clearly demonstrate the superiority of advanced materials over traditional options. |
Comparative analysis of outcomes between traditional and advanced bandages
Challenges and Limitations
Challenges implicated by diverse factors in clinical advance of made-up advanced bandages
While showing great potential, there are a wide range of challenges that have to be overcome in order to increase the clinical accessibility of advanced bandages (Levinet al., 2023). Important barriers include cost. Smart and bio electronic bandages, as advanced medical devices, are considerably more expensive to manufacture and access than regular bandages, so use is very limited to only the most resource-poor settings (Wanget al., 2021). There are few fully comprehensive clinical guidelines or evidence from longer-term studies against them (Shirzaeiet al., 2023). This means that healthcare service providers may be reluctant to embrace these technologies, simply because they do not have information regarding the long-term efficacy of such technologies, regulatory approvals and even side effects.
Considerations for patient populations, including those with comorbidities
Some patient populations present unique difficulties for advanced bandage use, such as diabetes, cardiovascular diseases and immuno-suppressed patients. Patients with comorbidities are generally more prone to a compromised healing process in wounds because of the concurrent disease; they are more susceptible to complications, which may cause delay or other worse conditions- infection or poor tissue regeneration (Wanget al., 2021). These populations may be particularly well suited to being treated with smart and bio electronic bandages, which have a superior ability to monitor and intervene. However, lack of tailor-made studies in these grouping is the limitation of the clinical use of such bandages. Moreover, the potential adverse reaction of advanced materials with autoimmune disorders or allergic patients could deter the potential benefit bestowed by the bandages (Levinet al., 2023).
Technology Trends for Further Research and Development
New Directions in Bandage and Dressing Materials
Opportunity in new material development and design
The future prospect of new medical bandages utilizes new materials and designs to integrate new steps within the approach used for wound management. Nanomaterials such as silver nanoparticles, graphene and bioresorbable polymers are under research because of their remarkable antimicrobial, anti-inflammatory and wound-healing properties (Tsegayet al., 2022). This material can be used in the formation of customized bandages that can adapt to the respective environments of a wound while bettering moisture levels, drug delivery and tissue repair (Schadteet al., 2023). Since advancement in the 3D printing technology is fast and has been booming, it becomes possible to design the patient-specific bandages that can actually be designed to fit into shapes of various geometries of wound tissues located in very hard-to-reach areas in the intestines (Dhandet al., 2024).
Another promising direction is self-healing hydrogels that mimic the great elasticity of skin and its ability to retain moisture (Tsegayet al., 2022). Such hydrogels might be drug-loaded and would deliver drugs based on the needs of the wound bed, thereby reducing dressing changes (Wanget al., 2021). As a design element, stretchable electronic bandages offer improved flexibility and conformability for use at dynamic sites, like the abdominal wall or gastrointestinal tract.
Integration of artificial intelligence for personalized wound care
AI is emerging as the non-invasive cutting-edge device for personalized wound care. Smart bandages, which would contain AI algorithms, could input and analyze data collected from implanted sensors, providing an edge for identifying early the course of a wound’s healing as well as for providing treatment recommendations (Jianget al., 2022). For instance, AI empowered smart bandages could automatically tweak the dosages of any drugs or the values of electro stimulations to match the healing characteristics of the patient with limited human interventions. For instance, through mobile phones or medical portals, healthcare providers would receive weekly updates about the state of the wound for proper and proactive wound care (Heet al., 2020).
Incorporation of the machine learning algorithms in the wound care devices would also help in improving the earlier detection of complications arising from infection or chronic non-healing wounds. AI analysis of vast datasets from earlier cases provides avenues for subtle changes in wound conditions that might not have been noted during the manual inspections-earlier interventions and reduced hospital stays (Mostafaluet al., 2018).
Regulatory and Ethical Considerations
Overview of regulatory pathways for new medical devices as bandage technology evolves, so too must the regulatory frameworks be governing their approval and use. Such advanced devices as smart or bio electronic bandages would require such a background as their use into the market entails very stringent assessments and validation by bodies, like in the U.S. Food and Drug Administration (FDA) or European Medicines Agency (EMA) (FDA, 2020). For such devices, clinical trials are offered to test their safety, the efficiency and biocompatibility prior to being approved for market use (Lievevrouw et al., 2021). The challenge is that these products have biological as well as electronic elements in their composition and therefore require interdisciplinary regulatory standards. For instance, the channels entering the regulatory procedure, such as U.S. 510(k) clearance, allow a certain innovative medical equipment to bypass the traditional long procedure if it could demonstrate substantial equivalence with devices that are already cleared (FDA, 2020).
Regulatory agencies are designing new legislations to ensure that such bioelectronic bandages and injective systems by AI-based technology are transparent and not liable for any risks that make no sense. For example, the FDA’s Digital Health Innovation Action Plan outlined a pathway for speeding up the process of developing health technologies while keeping up with the safety records.
Ethical implications of using advanced technologies in pati)ent care
The other concern is that advanced bandages promise better patient outcomes, but they raise several ethical dilemmas. One of the principal concerns is access and affordability-particularly in low-income or rural areas where advanced medical devices may be unaffordable (Quilliam et al., 2022). Ethical dilemmas arise, too, in terms of data privacy. Such smart bandages that collect and transmit data relating to the patient’s wound condition raise many questions about storing such information, which might have access to it and what could be done with such information. Patient consent and data security will be the major challenges as these technologies are increasingly adopted in healthcare (Wanget al., 2023).
Another ethical issue is over-reliance on technology. For example, even as they have the potential to enhance care, the risks are that healthcare providers will start relying on such automated systems to do things more efficiently and fast, thus diluting the quality of interaction between patients and clinicians. As long as these technologies supplement instead of replacing human judgment, patient trust and the quality of care will be diluted (MacIntyreet al., 2023).
CONCLUSION
In conclusion, the advancements in medical devices on bandages for intestinal wounds healing, especially the smart and bio electronic ones, signify an enormous jump in the management of wound healing. The advancements herein not only protect through physical barrier action but actively perform in relation to the healing process by maintaining favorable wound conditions, delivering therapeutic agents and ensuring cellular regeneration through such technologies as electro stimulation. These technologies may increase the chances of healing and simplify post-surgical patients and offer real-time monitoring capabilities that far exceed traditional bandage systems. However, costs, access and clinical acceptance still are the dominant challenges and perhaps more so in underserved populations. Continued research and development, along with targeted clinical trials, are essential to establish these technologies as standard practice in gastrointestinal surgery and wound care, promising improved patient outcomes and more personalized treatment strategies.
Cite this article:
Murthannagari VR, Nagarajan JR, Krishnan GGN, Ponmurugesan K, Devaraj HT, Jani K. Regulatory and Quality Aspects of Bio Absorbable Bandages for Intestinal Wound Healing. Int. J. Pharm. Investigation. 2025;15(3):313-24.
REFERENCES
- New smart bandage blends flexible electronics and wound healing science [Internet]. (2023). College of Medicine. https://medicine.arizona.edu/news/2023/new-smart-bandage-blends-flexible-electronics-and-wound-healing-science
- Bhattacharya, S., Ray, R. M., & Johnson, L. R. (2004). Prevention of TNF-α-induced apoptosis in polyamine-depleted IEC-6 cells is mediated through the activation of ERK1/2. American Journal of Physiology. Gastrointestinal and Liver Physiology, 286(3), G479–G490. https://doi.org/10.1152/ajpgi.00342.2003
- Britto, E. J., Nezwek, T. A., & Robins, M. (published 2024). Wound dressings. PubMed. https://www.ncbi.nlm.nih.gov/books/NBK470199/
- Buffin-Meyer, B., Crassous, P.-A., Delage, C., Denis, C., Schaak, S., & Paris, H. (2007). EGF receptor transactivation and PI3-kinase mediate stimulation of ERK by α2A-adrenoreceptor in intestinal epithelial cells: A role in wound healing. European Journal of Pharmacology, 574(2–3), 85–93. https://doi.org/10.1016/j.ejphar.2007.07.014
- Cario, E., Gerken, G., & Podolsky, D. K. (2007). Toll-like receptor 2 controls mucosal inflammation by regulating epithelial barrier function. Gastroenterology, 132(4), 1359–1374. https://doi.org/10.1053/j.gastro.2007.02.056
- Chen, X., & Peng, Y. (2023). Wearable bioelectronic system for wound healing and management. Biomaterials Translational. PubMed, 4(2), 65–66. https://doi.org/10.12336/biomatertransl.2023.02.002
- Ciacci, C., Lind, S. E., & Podolsky, D. K. (1993). Transforming growth factor β regulation of migration in wounded rat intestinal epithelial monolayers. Gastroenterology, 105(1), 93–101. https://doi.org/10.1016/0016-5085(93)90014-4
- Derakhshandeh, H., Kashaf, S. S., Aghabaglou, F., Ghanavati, I. O., & Tamayol, A. (2018). Smart bandages: The future of wound care. Trends in Biotechnology, 36(12), 1259–1274. https://doi.org/10.1016/j.tibtech.2018.07.007
- Dhand, A. P., Davidson, M. D., Zlotnick, H. M., Kolibaba, T. J., Killgore, J. P., & Burdick, J. A. (2024). Additive manufacturing of highly entangled polymer networks. Science, 385(6708), 566–572. https://doi.org/10.1126/science.adn6925
- Dignass, A., Lynch-Devaney, K., Kindon, H., Thim, L., & Podolsky, D. K. (1994). Trefoil peptides promote epithelial migration through a transforming growth factor beta-independent pathway. The Journal of Clinical Investigation, 94(1), 376–383. https://doi.org/10.1172/JCI117332
- Dignass, A. U., Baumgart, D. C., & Sturm, A. (2004). The aetiopathogenesis of inflammatory bowel disease – Immunology and repair mechanisms. Alimentary Pharmacology and Therapeutics, 20 (Suppl. 4), 9–17. https://doi.org/10.1111/j.1365-2036.2004.02047.x
- Dignass, A. U., Lynch-Devaney, K., & Podolsky, D. K. (1994). Hepatocyte growth factor/scatter factor modulates intestinal epithelial cell proliferation and migration. Biochemical and Biophysical Research Communications, 202(2), 701–709. https://doi.org/10.1006/bbrc.1994.1987
- Dignass, A. U., & Podolsky, D. K. (1993). Cytokine modulation of intestinal epithelial cell restitution: Central role of transforming growth factor β. Gastroenterology, 105(5), 1323–1332. https://doi.org/10.1016/0016-5085(93)90136-z
- Dignass, A. U., & Podolsky, D. K. (1996). Interleukin 2 modulates intestinal epithelial cell function in vitro. Experimental Cell Research, 225(2), 422–429. https://doi.org/10.1006/excr.1996.0193
- Dignass, A. U., Tsunekawa, S., & Podolsky, D. K. (1994). Fibroblast growth factors modulate intestinal epithelial cell growth and migration. Gastroenterology, 106(5), 1254–1262. https://doi.org/10.1016/0016-5085(94)90017-5
- Ehtesabi, H., Kalji, S.-O., & Movsesian, L. (2022). Smartphone-based wound dressings: A mini-review. Heliyon, 8(7), Article e09876. https://doi.org/10.1016/j.heliyon.2022.e09876
- Ey, B., Eyking, A., Gerken, G., Podolsky, D. K., & Cario, E. (2009). TLR2 mediates gap junctional intercellular communication through connexin-43 in intestinal epithelial barrier injury. Journal of Biological Chemistry, 284(33), 22332–22343. https://doi.org/10.1074/jbc.M901619200
- Fukata, M., Michelsen, K. S., Eri, R., Thomas, L. S., Hu, B., Lukasek, K., Nast, C. C., Lechago, J., Xu, R., Naiki, Y., Soliman, A., Arditi, M., & Abreu, M. T. (2005). Toll-like receptor-4 is required for intestinal response to epithelial injury and limiting bacterial translocation in a murine model of acute colitis. American Journal of Physiology. Gastrointestinal and Liver Physiology, 288(5), G1055–G1065. https://doi.org/10.1152/ajpgi.00328.2004
- Girard, E., Messager, M., Sauvanet, A., Benoist, S., Piessen, G., Mabrut, J. Y., & Mariette, C. (2014). Anastomotic leakage after gastrointestinal surgery: Diagnosis and management. Journal of Visceral Surgery, 151(6), 441–450. https://doi.org/10.1016/j.jviscsurg.2014.10.004
- He, M., Ou, F., Wu, Y., Sun, X., Chen, X., Li, H., Sun, D., & Zhang, L. (2020). Smart multi-layer PVA foam/CMC mesh dressing with integrated multi-functions for wound management and infection monitoring. Materials and Design, 194, Article 108913. https://doi.org/10.1016/j.matdes.2020.108913
- Heyer, K., Augustin, M., Protz, K., Herberger, K., Spehr, C., & Rustenbach, S. J. (2013). Effectiveness of Advanced versus Conventional Wound Dressings on Healing of Chronic Wounds: Systematic Review and Meta-Analysis. Dermatology, 226(2), 172–184. https://doi.org/10.1159/000348331
- Hossain, Md. I., Zahid, Md. S., Chowdhury, M. A., Hossain, M. M. M., Hossain, N., Islam, M. A., & Mobarak, M. H. (2024). Smart bandage: A device for wound monitoring and targeted treatment. Results in Chemistry, 7, Article 101292. https://doi.org/10.1016/j.rechem.2023.101292
- Housley, R. M., Morris, C. F., Boyle, W., Ring, B., Biltz, R., Tarpley, J. E., Aukerman, S. L., Devine, P. L., Whitehead, R. H., & Pierce, G. F. (1994). Keratinocyte growth factor induces proliferation of hepatocytes and epithelial cells throughout the rat gastrointestinal tract. The Journal of Clinical Investigation, 94(5), 1764–1777. https://doi.org/10.1172/JCI117524
- Iismaa, S. E., Kaidonis, X., Nicks, A. M., Bogush, N., Kikuchi, K., Naqvi, N., Harvey, R. P., Husain, A., & Graham, R. M. (2018). Comparative regenerative mechanisms across different mammalian tissues. npj Regenerative Medicine, 3(1), 6. https://doi.org/10.1038/s41536-018-0044-5
- Iizuka, M., & Konno, S. (2011). Wound healing of intestinal epithelial cells. World Journal of Gastroenterology, 17(17), 2161–2171. https://doi.org/10.3748/wjg.v17.i17.2161
- Iizuka, M., Sasaki, K., Hirai, Y., Shindo, K., Konno, S., Itou, H., Ohshima, S., Horie, Y., & Watanabe, S. (2007). Morphogenic protein epimorphin protects intestinal epithelial cells from oxidative stress by the activation of EGF receptor and MEK/ERK, PI3 kinase/Akt signals. American Journal of Physiology. Gastrointestinal and Liver Physiology, 292(1), G39–G52. https://doi.org/10.1152/ajpgi.00181.2006
- Song, J. W., Ryu, H., Bai, W., Xie, Z., Vázquez-Guardado, A., Nandoliya, K., Avila, R., Lee, G., Song, Z., Kim, J., Lee, M.-K., Liu, Y., Kim, M., Wang, H., Wu, Y., Yoon, H.-J., Kwak, S. S., Shin, J., Kwon, K., . . . Rogers, J. A. (2023). Bioresorbable, wireless and battery-free system for electrotherapy and impedance sensing at wound sites. Science Advances, 9(8), Article eade4687. https://doi.org/10.1126/sciadv.ade4687
- Jiang, Y., Trotsyuk, A. A., Niu, S., et al. (2022). Wireless, closed-loop, smart bandage with integrated sensors and stimulators for advanced wound care and accelerated healing. Nature Biotechnology, 41, 1–11. https://doi.org/10.1038/s41587-022-01528-3
- Jones, A. M., & San Miguel, L. (2006). Are modern wound dressings a clinical and cost-effective alternative to the use of gauze? Journal of Wound Care, 15(2), 65–69. https://doi.org/10.12968/jowc.2006.15.2.26886
- Kloth, L. C. (2014). Electrical stimulation technologies for wound healing. Advances in Wound Care, 3(2), 81–90. https://doi.org/10.1089/wound.2013.0459
- Larson, S. D., Li, J., Chung, D. H., & Evers, B. M. (2007). Molecular mechanisms contributing to glutamine-mediated intestinal cell survival. American Journal of Physiology. Gastrointestinal and Liver Physiology, 293(6), G1262–G1271. https://doi.org/10.1152/ajpgi.00254.2007
- Levin, A., Gong, S., & Cheng, W. (2023). Wearable smart bandage-based bio-sensors. Biosensors, 13(4), 462–462. https://doi.org/10.3390/bios13040462
- Lievevrouw, E., Marelli, L., & Van Hoyweghen, I. (2022). The FDA’s standard-making process for medical digital health technologies: co-producing technological and organizational innovation. BioSocieties, 17(3), 549–576. https://doi.org/10.1057/s41292-021-00232-w
- Liu, C. Y., Cham, C. M., & Chang, E. B. (2021). Epithelial wound healing in inflammatory bowel diseases: The next therapeutic frontier. Translational Research: The Journal of Laboratory and Clinical Medicine, 236, 35–51. https://doi.org/10.1016/j.trsl.2021.06.001
- MacIntyre, M. R., Cockerill, R. G., Mirza, O. F., & Appel, J. M. (2023). Ethical considerations for the use of artificial intelligence in medical decision-making capacity assessments. Psychiatry Research, 328, Article 115466. https://doi.org/10.1016/j.psychres.2023.115466
- Mostafalu, P., Tamayol, A., Rahimi, R., Ochoa, M., Khalilpour, A., Kiaee, G., Yazdi, I. K., Bagherifard, S., Dokmeci, M. R., Ziaie, B., Sonkusale, S. R., & Khademhosseini, A. (2018). Smart bandage for monitoring and treatment of chronic wounds. Small, 14(33), Article e1703509. https://doi.org/10.1002/smll.201703509
- A flexible bioelectronic bandage for accelerated repair of intestinal wounds. (2024). Nature Electronics, 7(4), 262–263. https://doi.org/10.1038/s41928-024-01139-7
- Independent modulation of enterocyte migration and proliferation by growth factors, matrix proteins and pharmacologic agents in an in vitro model of mucosal healing [Internet]. (1992). PubMed. https://pubmed.ncbi.nlm.nih.gov/1353641/
- Uptake and metabolism of plasma glutamine by the small intestine [Internet]. (1974). PubMed. https://pubmed.ncbi.nlm.nih.gov/4605420/
- Ohneda, K., Ulshen, M. H., Fuller, C. R., D’Ercole, A. J., & Lund, P. K. (1997). Enhanced growth of small bowel in transgenic mice expressing human insulin-like growth factor I. Gastroenterology, 112(2), 444–454. https://doi.org/10.1053/gast.1997.v112.pm9024298
- Paclik, D., Lohse, K., Wiedenmann, B., Dignass, A. U., & Sturm, A. (2008). Galectin-2 and −4, but not galectin-1, promote intestinal epithelial wound healing in vitro through a TGF-beta-independent mechanism. Inflammatory Bowel Diseases, 14(10), 1366–1372. https://doi.org/10.1002/ibd.20499
- Pang, Q., Yang, F., Jiang, Z., Wu, K., Hou, R., & Zhu, Y. (2023). Smart wound dressing for advanced wound management: Real-time monitoring and on-demand treatment. Materials and Design, 229, Article 111917. https://doi.org/10.1016/j.matdes.2023.111917
- Park, J. H., McCusker, R. H., Vanderhoof, J. A., Mohammadpour, H., Harty, R. F., & MacDonald, R. G. (1992). Secretion of insulin-like growth factor II (IGF-II) and IGF-binding protein-2 by intestinal epithelial (IEC-6) cells: Implications for autocrine growth regulation. Endocrinology, 131(3), 1359–1368. https://doi.org/10.1210/endo.131.3.1380441
- Podolsky, D. K., Gerken, G., Eyking, A., & Cario, E. (2009). Colitis-associated variant of TLR2 causes impaired mucosal repair because of TFF3 deficiency. Gastroenterology, 137(1), 209–220. https://doi.org/10.1053/j.gastro.2009.03.007
- Quilliam, C., Glenister, K., Ervin, K., & Weller-Newton, J. (2023). Revisiting rural healthcare access through Held’s ethics of care. Social Theory and Health, 21(2), 178–193. https://doi.org/10.1057/s41285-022-00181-9
- Rakoff-Nahoum, S., Hao, L., & Medzhitov, R. (2006). Role of toll-like receptors in spontaneous commensal-dependent colitis. Immunity, 25(2), 319–329. https://doi.org/10.1016/j.immuni.2006.06.010
- Rezvani Ghomi, E., Khalili, S., Nouri Khorasani, S., Esmaeely Neisiany, R., & Ramakrishna, S. (2019). Wound dressings: Current advances and future directions. Journal of Applied Polymer Science, 136(27), Article 47738. https://doi.org/10.1002/app.47738
- Schadte, P., Rademacher, F., Andresen, G., Hellfritzsch, M., Qiu, H., Maschkowitz, G., Gläser, R., Heinemann, N., Drücke, D., Fickenscher, H., Scherließ, R., Harder, J., Adelung, R., & Siebert, L. (2023). 3D-printed wound dressing platform for protein administration based on alginate and zinc oxide tetrapods. Nano Convergence, 10(1), 53. https://doi.org/10.1186/s40580-023-00401-6
- Shirzaei Sani, E., Xu, C., Wang, C., Song, Y., Min, J., Tu, J., Solomon, S. A., Li, J., Banks, J. L., Armstrong, D. G., & Gao, W. (2023). A stretchable wireless wearable bioelectronic system for multiplexed monitoring and combination treatment of infected chronic wounds. Science Advances, 9(12), Article eadf7388. https://doi.org/10.1126/sciadv.adf7388
- Smith, P.-A. (2008). Nutritional therapy for active Crohn’s disease. World Journal of Gastroenterology, 14(27), 4420–4423. https://doi.org/10.3748/wjg.14.4420
- Sommer, K., Wiendl, M., Müller, T. M., Heidbreder, K., Voskens, C., Neurath, M. F., & Zundler, S. (2021). Intestinal mucosal wound healing and barrier integrity in IBD–crosstalk and trafficking of cellular players. Frontiers in Medicine, 8, Article 643973. https://doi.org/10.3389/fmed.2021.643973
- Son, D. O., Satsu, H., & Shimizu, M. (2005). Histidine inhibits oxidative stress- and TNF-α-induced interleukin-8 secretion in intestinal epithelial cells. FEBS Letters, 579(21), 4671–4677. https://doi.org/10.1016/j.febslet.2005.07.038
- Sood, A., Granick, M. S., & Tomaselli, N. L. (2014). Wound dressings and comparative effectiveness data. Advances in Wound Care, 3(8), 511–529. https://doi.org/10.1089/wound.2012.0401
- Tsegay, F., Elsherif, M., Butt, H., & Singapore-MIT Alliance for Research and Technology Center. (2022). Smart 3D printed hydrogel skin wound bandages: A review. Polymers, 14(5), 1012. https://doi.org/10.3390/polym14051012
- United States Food and Drug Administration. (2020). Digital health innovation action plan. https://www.fda.gov/media/106331/
- Wang, C., Liu, S., Yang, H., Guo, J., Wu, Y., & Liu, J. (2023). Ethical considerations of using ChatGPT in health care. Journal of Medical Internet Research, 25(1), Article e48009. https://doi.org/10.2196/48009
- Wang, C., Shirzaei Sani, E., & Gao, W. (2021). Wearable bioelectronics for chronic wound management. Advanced Functional Materials, 32(17), Article 2111022. https://doi.org/10.1002/adfm.202111022
- Wang, M., Shi, J., Yu, C., Zhang, X., Xu, G., Xu, Z., & Ma, Y. (2023). Emerging strategy towards mucosal healing in inflammatory bowel disease: What the future holds? Frontiers in Immunology, 14, Article 1298186. https://doi.org/10.3389/fimmu.2023.1298186
- Wu, H., Wang, Y., Li, H., Hu, Y., Liu, Y., Jiang, X., Sun, H., Liu, F., Xiao, A., Chang, T., Lin, L., Yang, K., Wang, Z., Dong, Z., Li, Y., Dong, S., Wang, S., Chen, J., Liu, Y., . . . Chang, L. (2024). Accelerated intestinal wound healing via dual electrostimulation from a soft and biodegradable electronic bandage. Nature Electronics, 7(4), 299–312. https://doi.org/10.1038/s41928-024-01138-8
- Yamamoto, T., Nakahigashi, M., Saniabadi, A. R., Iwata, T., Maruyama, Y., Umegae, S., & Matsumoto, K. (2007). Impacts of long-term enteral nutrition on clinical and endoscopic disease activities and mucosal cytokines during remission in patients with Crohn’s disease: A prospective study with Crohnʼs disease: A prospective study. Inflammatory Bowel Diseases, 13(12), 1493–1501. https://doi.org/10.1002/ibd.20238
- Yamamoto, T., Nakahigashi, M., Umegae, S., Kitagawa, T., & Matsumoto, K. (2007). Impact of long-term enteral nutrition on clinical and endoscopic recurrence after resection for Crohn’s disease: A prospective, non-randomized, parallel, controlled study. Alimentary Pharmacology and Therapeutics, 25(1), 67–72. https://doi.org/10.1111/j.1365-2036.2006.03158.x