ABSTRACT
Major molecular, physical as well as cellular changes inside their host tissues are enthused by the tumor cells. The evolving TME is an active and multifaceted system. The structure of the TME differs amongst the categories of tumor, although stromal cells, ECM cells of immune and blood vessels be included in the hallmark characteristics. It is supposed that the -TME is not only a quiet bystander, though slightly a vigorous cancer progression promoterij. Understanding the TME’s obscurity and how it influences the way dissimilar anticancer medicines, such as immunotherapies, react to patients has come an elongated way in the last 20 years. The cancer immunotherapy and cancer cells are recognised as well as slaughtered by the immune structure of the body. It has established promising handling outcomes in an array of solid tumors as well as hematological malignancies. Lately, the growth of Antigen-Chimeric T cells (CAR-T) plus tumor vaccines, as well as the blockage of PD-1 (Programmed Death-1), PD-L1 (Programmed Death-1 Ligand-1), along with PD-L2, (Programmed Death-2) have achieved recognition as immunotherapies. Tumor microenvironment is intimately associated with progression, metastasis as well as Tumorigenesis. Therefore in this review article we have discussed about tumor microenvironment, different cells present in it, such as immune cells, cancer cells, mesenchymal cells, endothelial cells as well as fibroblasts. The importance of ECM in the tumor microenvironment. The TME function, in the metastasis and progression of the cancer. The crucial part of TME in regulating PD-1 plus PD-L1 expression, challenges and future directions of targeting the TME for cancer treatment.
INTRODUCTION
The varied populace of compartments collected of tumor cells and close by endogenous cells of stroma which are conscripted by the tumor is termed as a tumor microenvironment.1 The fact that the tumor cell “seed” and the nearby microenvironment “soil” co-evolve during tumor advancement and that there is significant cell-type-to-cell cross-talk that fosters tumor growth and development are becoming well-established.1 The two aspects that influence progression and development procedure of tumor are genetic/epigenetic alterations into the cells of tumor as well as via dynamic and mutual interactions that rearranges the constituents of the Tumor Microenvironment (TME).2 TME is collected of different cellular and non-cellular constituents, named tumor cells, tumor stromal cells, which include stromal fibroblasts, immune cells plus endothelial cells such as macrophages, lymphocytes and microglia as well as the non-cellular constituents of extracellular matrix like fibronectin, collagen, laminin, hyaluronan amongst others.3,4 The non-malignant cells are used for their advantage via the networks of complex signaling so that the roles of cellular as well as non-cellular constituents are prohibited by the tumor cells which is the central component of TME. Such crosstalks have detrimental effects on therapy response, Multi-Drug Resistance (MDR), tumor development and maintenance. The nonmalignant cells TME have a crucial part in promoting tumorigenesis in every stage of cancer metastasis plus growth.5
Many different intriguing interaction mechanisms are up-coming, whereas the resource of intercellular communication is a multifaceted system of chemokines, cytokines, inflammatory mediators, growth features plus matrix remodeling enzymes. Apoptotic bodies, exosomes, Circulating Tumor Cells (CTCs), as well as cell-free DNA (cfDNA) are examples of these new Horizontal Gene Transfer (HGT) moderators made commencing cells of tumor that transfer information to target cells that are far, such as cancerous or healthy cells.6 Current developments in the field of tumor biology have demonstrated the importance of thoroughly examining the various connections that take place among tumor cells as well as their immediate surroundings in order to comprehend an assortment of fundamental mechanisms of tumor growth as well as metastasis.7 Tumor cells’ reciprocal interactionswiththe cellularaswellasnon-cellular(ECM)elements of the TME result in the loss of tissue integrity, carcinogenesis and further advancement.8,9 Thus, most phases in the development of tumors, such as invasion, migration, heterogeneity in cancer evolution of clones, apoptosis, Epithelial-Mesenchymal Transition (EMT), chemotherapeutic drug resistance and development of metastasis, neovascularization, are effectively controlled by relationship between genetically modified tumor cells, reactive non- neoplastic cells as well as Extracellular Matrix (ECM).10–12 Various efforts on this part are focused as TME has a persuasive function in malignancy.13 In other words, it is anticipated that a deeper comprehension of the mechanisms by which TME influences the advancement of cancer would open up new avenues for the isolation and therapy of cancer cells. Disrupting the complex interactions that arise between host cells, cancer cells, as well as their surroundings Extracellular Matrix (ECM) can accomplish this.9 One significant obstacle in creating experimental cancer models is recapitulating TME. Preserving important features of the original tumor is crucial for the development of a trustworthy instrument for tailored cancer treatment as well as medication development. With the utilization of lab-on-a-chip as well as microfluidic instruments, recent advancements in three-Dimensional (3D) platforms have created a great potential to bridge the translational gap between preclinical as well as clinical research, plus to more effectively promote the biology plus role of TME situations.14,15 In the present article we have focused on the functions of different cellular and non-cellular constituents of TME, the importance of ECM in the tumor microenvironment, function of TME in the metastasis as well as development of cancer and the crucial part of TME in regulating PD-1 as well as PD-L1 expression, therapeutic strategies and future directions of targeting the TME for cancer treatment.
Immune Cells
TME immunological cells are an essential component. The interaction amid immune cells as well as TMEs is dichotomous: immune cells can both of stimulate or inhibit tumor augmentation, depending on the situation. TME immunological cells are an essential component. The interaction connecting immune cells as well as TMEs is dichotomous: immune cells can either stimulate or inhibit tumor augmentation, depending on the situation. Hepatocellular, cervical and colorectal cancers are among the many cancer types whose tumor augmentation is commonly caused by a chronic infection-related, ongoing inflammation. In general, there are divided into two categories of immune cells: adaptive as well as innate. Upon exposure to particular antigens, adaptive immunity is triggered, utilizing an immune memory to assess the threat and strengthen the body’s defenses. T-cells, B-cells, as well as NK cells are components of the adaptable defense. A general defense system called innate immunity kicks in a few hours after the body is exposed to a foreign antigen.
Dendritic cells, neutrophils and macrophages are examples of innate immune response cells.16
T-Cells
Every T-cell produces a T Cell Receptor (TCR) that is unique to it and can identify a particular antigen. Within the TME, there are several distinct T-cell populations that influence tumorigenesis. Tumor cells are the target of Cytotoxic T-cells (CD8+), which identify aberrant tumor antigens that are expressed in cells of the cancer as well as destroy them. A favorable prognosis for cancer patients is frequently linked to the cytotoxic T-cells being present in the TME. Cytotoxic T-cells not only eradicate tumor cells but also stop angiogenesis by releasing Interferon gamma (IFN-γ). CD4+ T-cells can differentiate into numerous subtypes inside the TME, which allows them to coordinate a wide range of immune reponses. Interleukin-2 (IL-2) and IFN-γ are secreted by proinflammatory CD4+ T-cells known as T helper 1 (Th-1) cells, which aid CD8+ cells. Positive results in numerous cancer types are linked to elevated Th-1 cell counts inside the tumor microenvironment. It is typically necessary for regulatory T cells (Tregs) to inhibit provocative reactions as well as manage autoimmunity. Tregs are widely distributed inside the TME as well as promote the development plus dissemination of tumors by attenuating the immune responses against the tumor. Tregs, for instance, release IL-2, which affects the homeostasis and functionality of Natural Killer (NK) cells. Furthermore, Treg cells interact with stromal cells including fibroblasts plus endothelial cells to indirectly promote the ability of cancer cells to survive by the release of development features. Three primary groups characterize the immunological landscape inside the TME: immune silent, immune excluded and immune permeated. Immune cells-like T-cells that are cytotoxic are uniformly dispersed all the way through a tumor that has been penetrated by the immune system, signifying an active immune response. On the other hand, some cancers are categorized as immune barred when T-cells be present near the tumor’s perimeter but have not penetrated the TME. Lastly, some cancers are classified as “immune silent” because they have no immune cell infiltrate at all, suggesting that the tumor has not elicited an immune response.16
B-Cells
Specialized immune cells called B-cells are in charge of producing antibodies, presenting antigens and secreting cytokines. B-cells typically gather in the periphery of tumors and are frequently detected in lymph nodes near the Tumor Microenvironment (TME). Although there aren’t as many B-cells entering the TME as there are T-cells, new research has shown that B-cell presence and function are crucial during tumorigenesis. Ectopic lymphoid structures that arise in the tumor microenvironment are referred to as “tertiary lymphoid structures” and are created in part by tumor infiltrating B-cells. In melanoma, ovarian and breast cancer, tertiary lymphoid structures are a favorable predictive factor that facilitates tight interaction between T and B cells. B-cells play several anti-tumorigenic functions, such as presenting antigen to T-cells, producing anti-tumor antibodies and secreting cytokines that encourage cytotoxic immune responses (such IFN-γ). On the other hand, B-cells have the ability to cause tumors and their existence in the Tumor Microenvironment (TME) may indicate a bad prognosis for prostate, bladder and renal cell carcinomas. By producing cytokines (like Transforming Growth Factor-beta (TGF-β) as well as IL-10), which promote immune-suppressive phenotypes in macrophages, cytotoxic T cells, as well as neutrophils, regulatory B-cells encourage tumor aggression.16
Nk-Cells
Naturally, NK cells search for tumor or host cells that have been infected by viruses as they circulate through the blood. There are two categories for NK cells functional varieties according to whether they actively take part in the destruction of tumor cells through cell-mediated means or whether they release inflammatory cytokines. NK cells are excellent at removing tumor cells from the circulation and can help prevent metastasis, but they are less successful in removing tumor cells from the tumor microenvironment (Table 1 and Figure 1).16
Table 1:
Innate immune cells in the tumor microenvironment.
Type of cell | Functions | Effect | References |
---|---|---|---|
Macrophages | Stimulate Th1 responses, phagocytosis, type 4 hypersensitivity. | Antitumor | 17–19 |
M1 | |||
M2a | Activate Th2 responses lesion curing, allergy. | Protumor | 17–19 |
M2b | Th2 commencement, immunoregulation. | Protumor | 18,19 |
M2c | Tissue refurbishes, matrix modification, immunoregulation. | Protumor | 18,19 |
M2d | Angiogenesis, apoptotic tissues clearance. | Protumor | 18,19 |
DCs Immature DCs | Acknowledge antigens, phagocytosis, stimulates T-cell energy, nominal APC, move to resultant lymphoid organs and endorse Th2 plus T-reg responses. | Reliants on tumor variety | 20 |
cDC1 | APC to CD8 T-cells, traverse arrangement, emission of IL12. | Reliants on tumor variety | 21–23 |
cDC2 | APC to CD8 T-cells. | Reliants on tumor variety | 21–23 |
pDCs | Copious secretory commotion (IFN kind 1), retort to viral illness. | Reliants on tumor variety | 20–23 |
MoDCs | Fabricate increased intensities of the pro-inflammatory cytokines TNF, IL6 as well as IL12. | Antitumor | 21–24 |
Tolerogenic DCs | Moderate APC, kindle Th2 reactions plus Tregs to stimulate acceptance. | Protumor | 24,25 |
Neutrophils N1 | Phagocytosis; liberates NETs, inflammatory cytokines, endorsement of tumor cell apoptosis, contaminant plus ROS, respiratory rupture. | Antitumor | 26 |
N2 | Migration plus invasion of cancer cells, sustain angiogenesis, metastasis as well as secrete chemokines, cytokines plus ROS/RNS and immune surveillance. | Protumor | 26 |
MDSCs M-MDSCs | Restrain adaptive plus innate reactions. | Protumor | 27,28 |
PMN-MDSCs | Restrain adaptive plus innate reactions. | Protumor | 27,28 |
eMDSCs | Restrain adaptive plus innate reactions. | Protumor | 27 |
NK Cells CD56hi CD16± Nks | Inflammatory cytokines are produced. | Reliants on tumor variety | 29 |
CD56lo CD16hi NKs | Antibody reliant cellular cytotoxicity is stimulated, augmented homicide, increased manufacturing of perforin. | Reliants on tumor variety | 29 |
ILCs ILC1 NK cells | Macrophage, commencement, CD8 T-cell commencement, continual inflammation, cytotoxicity. | Antitumor | 30,31 |
ILC1 Non-NK | Continual inflammation, macrophage commencement. | Antitumor | 30,31 |
ILC2 | Motivates T-cell reactions via Th2- related cytokines, supports skin inflammation. | Protumor plus antitumor | 30,31 |
ILC3 | Severe inflammation, developed lymphoid bacterial immunity, intestinal homeostasis. | Protumor | 30,31 |
Macrophages
An integral part of the immune system that is innate, macrophages delivers antigens and phagocytoses pathogens to alter immune responses. Macrophages are essential for tissue healing as well as wound healing. Both immune-suppressive supports inflammatory M1 macrophages so as to phagocytose as well as promote wound healing plus destroy cells are subtypes of monocyte-derived macrophages. Both classes of macrophages are present within a tumor, but in order to support the growth and propagation of the tumor, the TME induces hypoxia as well as the release of cytokines, like IL-4, to enhance the M2 phenotype. Macrophages can make up to 50% of the mass of some tumor types when they are severely invaded. In several cancer types, including breast, lung and stomach cancers, a patient’s poor prognosis is usually associated with a high amount of macrophage infiltration. (VEGF)-A (Vascular Endothelial Growth Factor) is secreted by macrophages in the TME, which encircles blood vessels and encourages the formation of new blood vessels (Table 1).16
Neutrophils
Neutrophils constitute the first line of protection against numerous infections, accounting for as much as 70% of circulating leukocytes. Neutrophils in the context of cancer can, based on the kind of stage of development of the tumor, either avoid or accelerate tumor development. Tumor cell death and inflammation are caused by the release of cytokines and reactive oxygen species by neutrophils that are attracted to the Tumor Microenvironment (TME) as the tumor grows. As a tumor develops, neutrophils modify the extracellular matrix to encourage angiogenesis, which in turn leads to tumor progression and local invasion. They also release VEGF and produce Matrix Metalloprotease (MMP)-9 (Table 1 and Figure 1).16
Dendritic Cells
Dendritic cells, also known as antigen-presenting cells, or DCs, are vital to the immune system because they offer antigens to T-cells, also referred to as antigen-presenting cells, or APCs at derived lymphoid organs like lymph nodes. There also recognise, seize as well as present antigens to T-cells. Eventually, DCs start pathogen-specific T-cell reactions by establishing a connection between innate plus adaptive immunity. By supplying environmental cues can either trigger an immune reaction directed at tumor cells or encourage their acceptance, the tumor microenvironment influences the destiny of DCs. Cytokines produced from the TME not only help DCs to tolerate the existence of tumor cells but also suppress the immunological response (Table 1 and Figure 1).16
Stromal Cells
Assisting the cells from surrounding endogenous tissue stroma are enlisted by cancer cells to expedite crucial stages of tumor development. The makeup of stromal cells, which can differ greatly throughout tumor types, is composed of fibroblasts, adipocytes, stellate cells and vascular endothelial cells. Stromal cells release a range of chemicals that impact invasion, growth, angiogenesis as well as metastases after they are recruited to the TME.16
Endothelial Cells
A thin monolayer of Endothelial Cells (ECs) known as the vascular endothelium controls the formation of blood vessels. Vascular endothelium not only provides water plus nutrients, maintains metabolic balance, transports immunological cells as well as facilitates the development of novel blood vessels, but it also separates circulating blood from tissues. The passive diffusion that cancer cells rely on for nutrition and gas transfer throughout the early phases of tumor growth. When tumors reach a volume of 1-2 mm3, oxygen is depleted and metabolic waste builds up, causing the TME to become hypoxic and acidic. Tumors must establish their own blood supply to counteract hypoxia and acidity. The transcription factors known as Hypoxia Inducible Factors (HIFs), which are crucial for coordinating cellular reactions to stumpy oxygen levels, is triggered by a hypoxic TME. Tumors frequently utilize vascular sprouting as a technique to take control of preexisting blood vessels and encourage the formation of new ones. HIFs specifically tell ECs to emit proangiogenic substances like VEGF, EGF, plus Platelet-Derived Growth Factor (PDGF) in order to start vascular sprouting. The paracrine and autocrine effects of VEGF encourage EC migration, which results in the formation of new blood vessel lumens. In order to create new basement membranes, ECs then produce proteins. Leaky vasculature is the result of blood vessels in the TME frequently failing to reach the last stages of maturity.
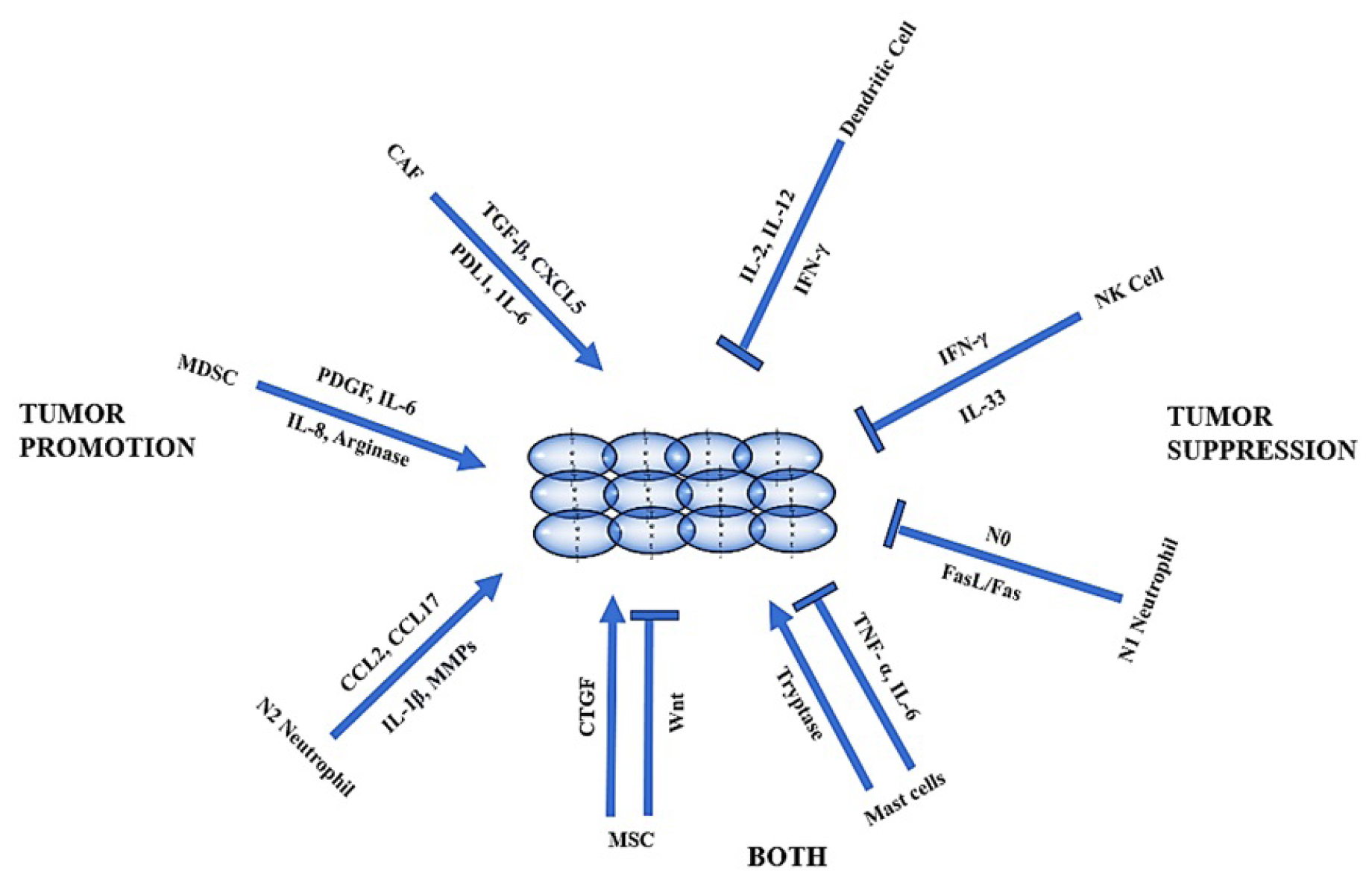
Figure 1:
Cancer progression is modulated by tumor-stroma interactions within the tumor microenvironment. The extracellular matrix, different stromal cells, as well as cancerous cells make up the tumor microenvironment. Fibroblasts, innate plus adaptive resistant cells and endothelial cells are examples of these stromal cells. During the emission of development features, cytokines, as well as other chemicals, these cells can each endorse otherwise hinder the progression of cancer. Most cells, including Mesenchymal Stem Cells (MSCs), can promote and prevent the spread of cancer.
The promotion of cancer cell motility, invasion and metastasis is facilitated by ECs, in addition to angiogenesis. Being highly malleable, ECs have the ability to alter the course of a cell. ECs change from endothelial to mesenchymal phase during tumor growth to form Cancer-Associated Fibroblasts (CAFs). TGF-β and Bone Morphogenetic Protein (BMP) facilitate the conversion of an EC into a CAF, which results in lost connections amid cells, elongation plus separation, increased motility, as well as hammering of endothelial characteristics. Tumor cell migration and invasion are significantly aided by CAF. Cancerous cells migrate from the primary TME to distant sites during the multi-step process of metastasis. In a process known as intravasation, prior to entering the circular system, cells of the tumor must exit the primary tumor location. During intravasation, tumor cells adhere to Endothelial Cells (ECs); this interaction alters the endothelium barrier and allows tumor cells to migrate between two ECs. Because blood vessels created in the TME are typically immature and do not have appropriate cell-to- cell contacts, cancer cells are able to spread.16
Cancer Associated Fibroblasts
In order to facilitate interaction among cancerous cells as well as TME, a substantial fraction of the tumor stroma, known as cancer-associated fibroblasts, or CAFs, are required. Though their sources vary, tissue-resident fibroblasts are frequently the source of CAFs. Adipocytes, ECs, bone marrow-derived mesenchymal stem cells, stellate cells, as well as pericytes are other sources of CAFS. Myofibroblasts, which actively aid in wound healing, can be reversibly produced from fibroblasts that ordinarily exist within tissues in response to injury. TGF-β signaling causes myofibroblasts to proliferate, acquire contractile qualities, develop secretory phenotypes and create extracellular matrix-all of which are crucial for wound healing. It is fitting that tumors are known as “wounds that never heal.” To turn fibroblasts into CAFs, cancer cells and other stroma cells release transforming growth factors, including as FGF2, PGDF and TGF-β, in the TME. In many cancer types, an accumulation of CAFs inside the tumor microenvironment is habitually connected to a poor prediction. For instance, there is a substantial correlation between the recurrence of colorectal cancer and the existence of CAFs. It has been established that CAFs both encourage and prevent tumorigenesis, despite being associated with a bad prognosis. Conversely, when a cancer type is extremely desmoplastic, its prognosis and overall survival increases. Examples of these cancer types include lung and breast cancer. Most extracellular elements, including cytokines, growth features as well as extracellular matrix, are produced by cancer-associated fibroblasts within the TME. Four primary mechanisms by which CAFs influence the TME include immunosuppression, neoangiogenesis, ECM remodeling and tumor growth and metastasis. A pivotal phase in the spread of malignancies with epithelial origins is the epithelial-mesenchymal transition, or EMT, during which the epithelial cells acquire invasive and migrating phenotypes while losing cell polarity and cell-to-cell adhesions. CAFs regulate metastasis in part by secreting TGF-β, a growth factor necessary for angiogenesis and EMT. MMP-3, which breaks down E-cadherin to encourage cancer cell incursion, is secreted by CAFs in order to facilitate cancer cells migration via the TME. Another key foundation of VEGF, which MMP-13 might liberate to encourage angiogenesis, is the Extracellular Matrix (ECM). CAFs generally produce immune modulatory chemokines and cytokines that support an immunosuppressive phenotype (Figure 2).16
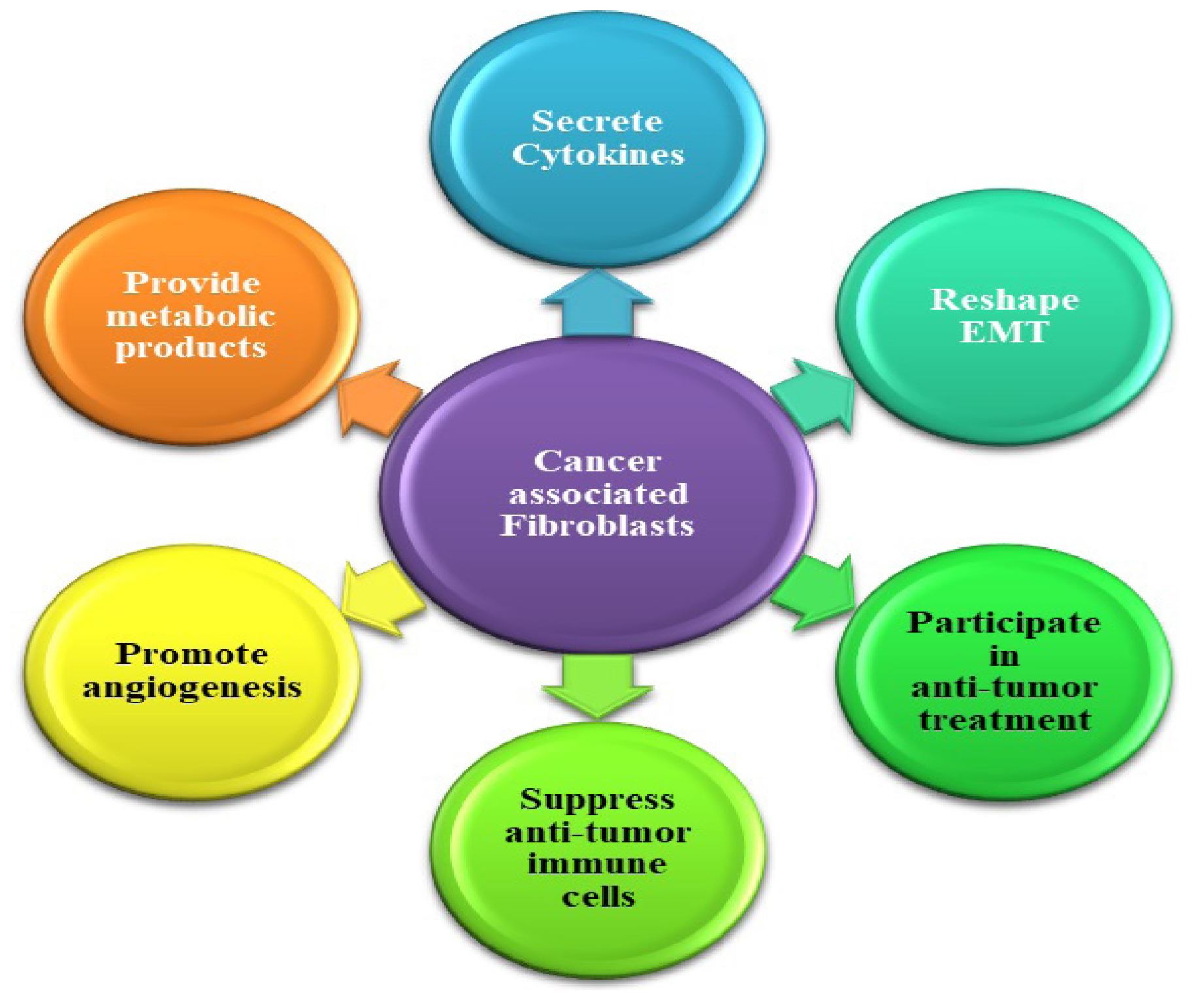
Figure 2:
By secreting a range of cytokines, altering the Extracellular Matrix (ECM), encouraging angiogenesis, suppressing anti-tumor immune cells, giving tumor cells metabolites (such as lactic acid, amino acids and fatty acids) and contributing to treatment resistance, among other mechanisms, CAFs can encourage the growth of tumors.
Adipocytes
Specialized cells called adipocytes are found throughout the body. They control vigor stability plus hoard additional vigor as plump. Adipocytes secrete metabolites, enzymes, hormones, development features, plus cytokines, which have an impact on the TME. Adipose cells and tumor cells interact dynamically and reciprocally inside the TME to promote tumor growth. Adipocytes are an essential component of the TME for breast cancer since White Adipose Tissue (WAT) makes up the majority of breast tissue. Adipocytes can be stimulated by breast cancer cells to undergo lipidlysis, a process that breaks down lipid reserves and makes accessible free fatty acids for cancerous cells to absorb. Free fatty acids are used by breast cancer cells to produce exosomes, lipid bioactive compounds, cell membranes and energy. Leptin is a crucial hormone that is made by adipocytes. It influences the proliferation of breast cancer cells, which directly advances tumor growth and it also indirectly advances tumor growth by stimulating macrophages. Adipocytes notably contribute to the extracellular matrix’s alteration through the secretion of metalloproteases, including MMP-1, MMP-7, MMP-10, MMP-11 plus MMP-14. Obesity is a substantial risk factor for several cancer types, including breast, pancreatic and ovarian cancer, as over 40% of cancer patients are overweight. Being an endocrine organ, white adipose tissue can encourage cancerous breast cells to spread to the liver plus lungs by paracrine indication (Figure 3).16
Stellate Cells
Stellate cells are mesenchymal stromal cells that are quiescent and found in the pancreas and liver. Stellate cells undergo activation upon tissue damage, cell cycle entry and induction into myofibroblasts. Stellate cells are characterized by the accumulation of vitamin A in lipid globules. HSC (Hepatic Stellate Cells) can make up to 15% of the total mass of the liver and are typically found in the portal and perisinusoidal regions. The most common sort of liver cancer is Hepatocellular Carcinoma (HCC) and HSCs help to encourage communication among TME cells. TGF-β is an essential signaling molecule that HCC produces and that activates HSCs. HSCs that have been triggered alter the extracellular matrix and release proangiogenic factors like MMP-2 and VEGF-A. Important components of HSCs that generate fresh ECM and remodel it by generating MMPs are lipid droplets. With thick fibrotic tissue or desmoplasia, pancreatic ductal adenocarcinoma is the mainly frequent kind of pancreatic cancer, accounting for 95% of cases. During their quiescent state, Pancreatic Stellate Cells (PSCs) produce degradation enzymes and ECM proteins including vimentin and desmin, which aid in the alteration of the extracellular matrix. PSC activation brought on by vitamin A deficiency results in the release of cytokines and chemokines as well as increased migratory and proliferation capacity. The promotion of the desmoplastic character of PDAC tumors and their hypoxic microenvironments is largely dependent on activated PSCs.16
TME-Non Cellular Components ECM
Within the TME, the ECM or extracellular matrix is essential for supporting the spread of tumor cells in addition to giving them structural scaffolding. ECM, which is made up of elastin, laminin, collagen, plus fibronectin, is a crucial molecular constituent of the TME. Large ECM deposits can make up as much as 60% of the bulk of solid tumors. Desmoplasia is characterized by huge collagen dumps and an elevated proportion of fibroblast permeation; this condition is significantly associated with a negative prognosis for the patient. Though CAFs are the main source of ECM, several cells within the TME secrete its constituent parts. The ECM is remodeled by MMPs, which are the breaking down proteases in ECM proteins plus aid in the spread plus metastasis of tumors.
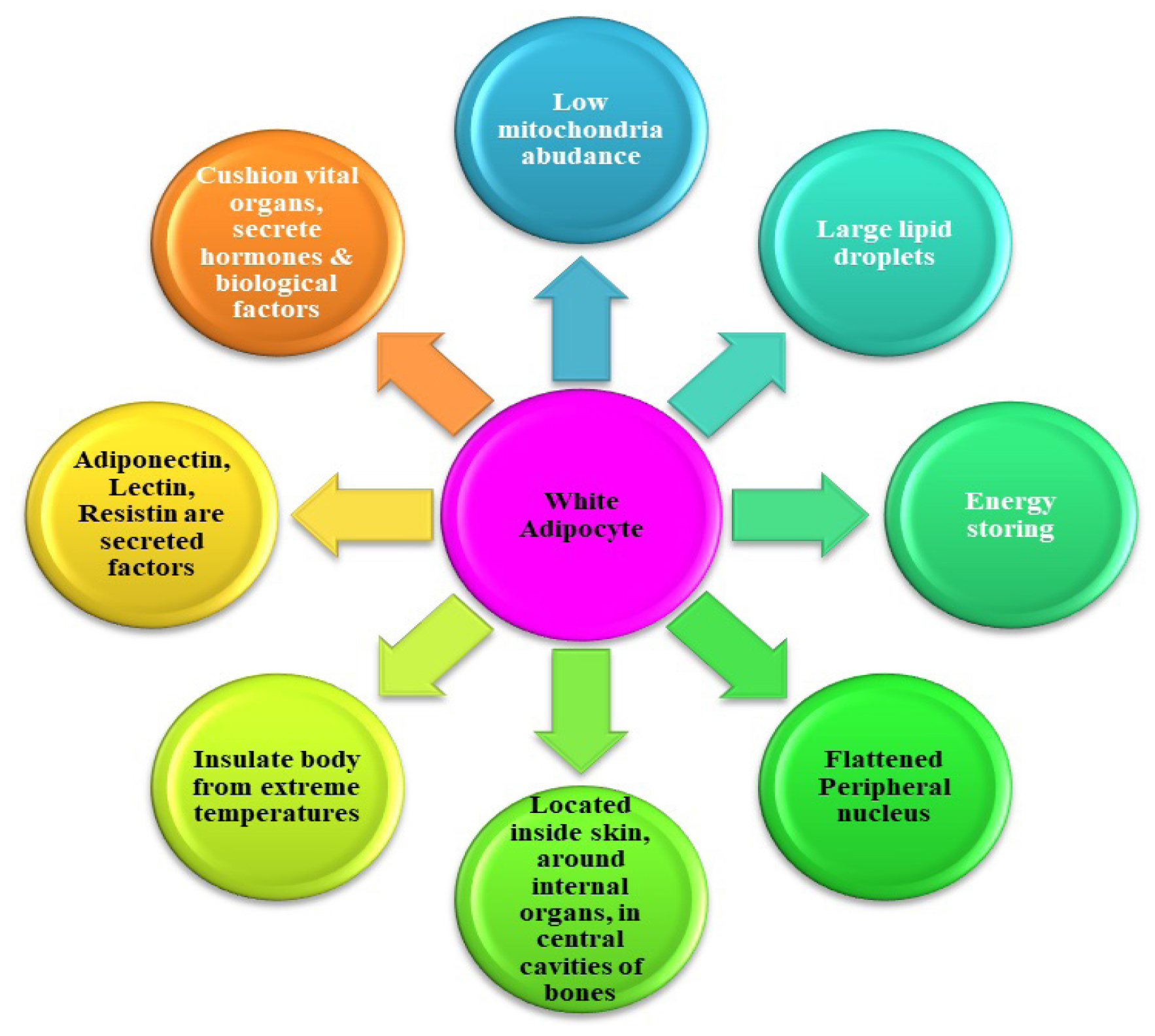
Figure 3:
Overview of the white adipocyte showing its morphology and functions in different organs.
Growth factors and cytokines are released by proteases, such as MMPs and are stored in the Extracellular Matrix (ECM). Examples of proangiogenic factors that may be deposited in the ECM include VEGF, FGF, PDGFB and TGFB.16
Exosomes
Microvesicles with a diameter ranging from 30 to 200 nm are called exosomes. They contain lipids, protein, RNA and DNA, all of which are present in the original cell. Exosomes within the TME are essential for promoting communication between stromal and malignant cells. Exosomes have been demonstrated to functionally stimulate angiogenesis, tumor development, inflammation, plus metastases inside the TME. Hypoxic conditions seem to aggravate cancer cells’ production of exosomes and encourage stromal cells to differentiate into CAFs.
ECM in TME
Numerous ECM molecules, including, elastin, laminins, fibronectin as well as fibrillar collagens, are articulated at elevated points in a large number of solid tumors. Furthermore, Pancreatic Ductal Adenocarcinomas (PDACs), one type of malignancy, have unusually high hyaluronan content. Up to 60% of the tumor mass is compromised by the ECM in many cancers. Although these ECM constituents originate from the tumor cells themselves, cancer-associated fibroblasts are the primary source.32 In fact, many solid tumors exhibit the invasion of fibroblasts/myofibroblasts as well as the consequent formation of sizable volumes of collagenous extracellular matrix. The desmoplasia process is closely associated with a dismal prognosis as well as systemic treatment resistance. Through the provision of Transforming Growth Factor beta (TGFβ) plus paracrine Stromal cell-Derived Factor-1 (SDF1) indications to support tumor cells, CAFs additionally contribute to the development of a more malignant tumor phenotype by inducing the synthesis of collagen plus additional extracellular matrix molecules, as well as by driving the Epithelial-to-Mesenchymal Transition (EMT).33 The TME’s constituent parts are all very different from one another in non-malignant tissues. As a result, the Extracellular Matrix (ECM) of the tumor fluctuates significantly commencing the surrounding standard tissue in terms of post-translational alteration, composition plus association in addition to the amount of deposition. When compared to benign mammary abrasions, the fabrication of collagen is pushed toward Col I and Col III in persistent ductal carcinomas. The tumor’s stromal region is where the enhanced expression is mostly seen. Given that the benign lesions are primarily composed of fibroblastic cells, this indicated the propensity plus ability of breast tumor cells to reabsorb the stroma’s produced matrisome.32 Col V, an uncommon collagen isoform in fibrocystic (<0.1%) and normal breast tissue, makes up to 15% of the collagenous matrix in the desmoplastic stroma of breast carcinomas. Collagen fiber length and organization are shown to decrease with an increase in the Col V/Col I proportion, according to experiments such as the second harmonics observation of fiber development. A gel-like Extracellular Matrix (ECM) can be produced when fiber production is totally blocked at higher ratios. In contrast, Col IV expression in ovarian carcinomas is downregulated as compared to benign tissue and it has an inverse relationship with both stage and malignancy markers. Human lung cancers exhibit the same trend of reduced Col IV appearance plus amplified Col I/Col III appearance.33–34 Increased Col I expression has been seen in melanoma and is linked to angiogenesis, invasiveness and decreased survival.35 Signs of rapid collagen turnover in malignancies include elevated expression of matrix proteases, abnormal fibrous collagen spindle shape and elevated collagen messenger RNA (mRNA) appearance.
Collagens
Collagen synthesis and maturation are intricate processes. In addition to enhanced-collagen programming gene transcription plus translation collagen, the TME’s enhanced production of collagen also necessitates the increased expression of some enzymes required for appropriate post-translational processing plus collagen molecule release. In fact, the TME frequently expresses high levels of collagen-processing enzymes such as prolyl hydrolases and lysyl hydroxylases.32 In many malignancies, particularly desmoplastic cancers, lysyl oxidases are also markedly elevated.32 Increased collagen and elastin cross-linking results from this, making the tumor more rigid and adding to its palpability along with the previously noted rise in extracellular matrix deposition. The strongly cross-linked collagenous matrix decreases total oxygen supply and in the TME while also increasing mechanical strain plus Focal Adhesion Kinase (FAK)-mediated indication.36 Intracellular modifications of collagen are mediated by ascorbic acid-dependent enzymes called Prolyl-4-Hydroxylases (P4HAs). This mechanism produces hydroxyproline, which is required to improve helical collagen stability in physiological settings. Intratumoral collagen deposition is increased by high P4HA expression.32 Additionally; it has a substantial correlation with chemotherapy resistance and a lower chance of survival for patients with triple-negative breast cancer.37
Proteoglycans
Proteoglycans (PGs), another significant component of the Extracellular Matrix (ECM), like collagens, depend on enzymes for proper synthesis and assembly. A core protein that has undergone extensive glycosylation is combined with shorter, longer chains of Glycosaminoglycans (GAGs) to form PGs. The core protein is translocated in the Golgi apparatus, which is also where the GAGs attach. A tetra saccharide is initially attached by glycosyltransferases to one of the main proteins serine, which serves as a connector. Subsequently, other glycosyltransferases link monosaccharides to the expanding GAG chain. For appropriate chain modification, sulfotransferases and epimerases are required in addition to other glycosyltransferases.32 Repetitive disaccharide patterns make up the GAGs. GAGs are classified as heparan sulfate, keratan sulfate, chondroitin sulfate, plus dermatan sulfate based on the makeup of these disaccharides.
PGs’ molecular weights can vary greatly. PGs are the building blocks of cartilage, together with Collagens (mostly Col II), highlighting their ability to support tissue rigidity and durability. Increased PG levels are seen in many tumors, but the narrative appears more complicated since variations between different PGs, particularly among small- along with increased-molecular load PGs, account for the majority of the PG content differences between malignant and normal tissue.32 While gastric tumors displayed versican plus decorin appearance, as well as a doubling of the total GAG content, Suhovskih et al. compared prostate cancer compared to healthy prostate tissue and observed decreased levels of decorin but increased appearance of CSPG4 plus aggrecan. Similar to this, versican and decorin are more prevalent in squamous cell laryngeal carcinoma as compared to standard tissue, whereas aggrecan is entirely downregulated. Brown et al. discovered that versican plus decorin appearance, in addition to Col I as well as fibronectin, were significantly enriched in the breast cancer stroma. Despite using mRNA appearance data for the important proteins, versican, decorin and lumican were highly overexpressed in prostate cancer; hence, conclusions on the actual PG content are challenging. Versican is more abundant in melanoma peritumoral stroma than in benign nevi. GAG sulfation patterns appear to alter in cancer as well. It’s interesting to note that there appears to be a change from 4- to 6-sulfation in Colorectal Cancer (CRC) plus gastric cancer, but the shift is reversed in laryngeal carcinomas, with greater 4-sulfation compared to 6-sulfation in normal tissue.32
Hyaluronic Acid
Unlike the other three GAGs, Hyaluronic Acid (HA) is not produced in the Golgi apparatus and is not conjugated to peptides. Three transmembrane glycosyltransferases of the Hyaluronan Synthase 1-3 (HAS1) family catalyze synthesis. In turn they conjugate N-acetylglucosamine plus gluconic acid.38 HASs are proteins that are attached to plasma membranes and are likely in charge of both the export of the extracellular synthesised HA macromolecule by enzymatic poremediated direct extrusion as well as its synthesis. Regarding whence ABC transporters export HA such MRP5 or MDR1, there is some debate.32 While human fibroblasts treated with ABC transporter inhibitors such as trequinsin or S-decylglutathione produced less HA, the same strategy with other inhibitors was unable to prevent HA release in breast cell outlines of cancer that articulate the desired ABC transporters. Although the exact role of ABC haulers in the manufacture of HA is yet unknown, 4-Methylumbelliferone (4-MU) effectively and selectively restrains HAS1-3, preventing the synthesis of HA.39,40 Many malignancies have elevated HA production, including prostate, breast, CRC and even brain tumors,41 in addition to pancreatic carcinomas.32,33 Histologically speaking, stromal cells, or fibroblasts, are frequently shown to be the primary source of HA in tumors and tumor cells have the ability to boost HA synthesis in fibroblasts that are cocultured.
Low HA levels and HAS1-3 expression are associated with a worse prognosis.42 Because HA binds to CD44, it may contribute to EMT, which increases metastasis and invasiveness.32 Following the suppression of HA formation, there is also a decrease in the expression of proteins (CD90, CD133 and EpCAM) that are believed to be indicators of cancer stem cells.43
Laminins
Additionally, malignant tissue frequently expresses laminins more strongly. Laminins are components of normal tissue that are not found in the basement membrane; this leads to a continuous, clearly defined linear staining as determined by immunohistology. Tumors frequently exhibit this aspect distorted, or seems that laminin is widely dispersed within the stromal portions of the tumors.44 Of fact, one of the hallmarks of invasive behavior is the separation of the foundation membrane plus the lack of adhesion to its distinct structure. In summary, there is a confirmed correlation between poor prognosis and invasiveness and elevated laminin expression and aberrant distribution, which has been present since the early 1980s. Laminin over expression is typically seen in breast cancer.32 Naba et al. examined the serious mutual immunodeficiency mice, the human melanoma xenografts matrisomes formed using an intricate and sophisticated proteomics technique. The method enables them to differentiate between the involvement of the orthologs of most proteins in humans plus mice varies enough in terms of amino acid sequence, which accounts for the contribution of both human tumor cells plus mouse stromal cells. The studies outcomes demonstrated that specific proteins (like Col7a1, Lama4, Lamb1) are only expressed by tumor cells, whereas other proteins (like Col5a3, Lama2, Eln) are only expressed by stromal cells. Furthermore, during development and metastasis, the ECM composition alters dramatically.32
Role of TME in the Metatasis Plus Progression of Cancer Complexity of TME
Alterations in Homeostasis And an aberrant immune response control the development of tumors.45 Cancer cells interactions with immune cells within the TME influence cancer growth and metastasis.46 The TME controls the fundamental survival of tumor cells and enhances their activity. Cancer cells are encouraged to become more aggressive plus to spread to distant locations by the interaction among the cellular plus structural elements of TME. There is mounting evidence that, when present in the Tumor Microenvironment (TME), innate immune cells (such as macrophages, neutrophils, dendritic cells, innate lymphocytes, myeloid inhibitory cells, plus Natural killer cells) as well as adaptive immunity cells (such as T cells plus B cells) aid in the growth of tumors.47 Cytotoxic CD8+ T Lymphocytes (CTLs) in particular participate in a critical part in the prevention and removal of tumors. On the other hand, because effector CTL evolution toward depletion CTLs has a significant part in the eventual failure of CTL-mediated tumor killing in many patients to eliminate cancer cells, which results in the progression of the disease.48 Furthermore, interactions between different cells and elements within the TME are also necessary for tumor spread.
The occurrence and metastasis of tumors are tightly correlated with the internal and exterior surroundings of tumor cells. This process includes the inner surroundings (cytoplasmic and nuclear) of the tumor cells plus the metabolism, function and structure of the tissue present in the tumor. The tumor microenvironment is created when tumor cells invade healthy tissues and alter the microenvironment around them by recruiting Cancer-Associated Fibroblasts (CAFs), controlling immune cells plus the factors they secrete and causing vascular endothelial cells to become neovascularized.49 Together with immune cell constituents, the TME contains a variety of non-immune stromal cell constituents that are important in hallmark tumor events such as, ECM Extracellular Matrix (ECM) invasion, metastasis plus angiogenesis. These constituents include endothelial cells, fibroblasts plus tissue-specific cells. An increasing body of research indicates that these stromal cells are crucial for immune escape plus Immune Checkpoint Blockade (ICB) resistance mechansims.50–52 The primary players in the TME are CAFs, which influence not only cancer cells other than also the Extracellular Matrix (ECM) plus immune infiltration. According to histopathological examination, CAFs can control the therapeutic impact and are correlated with the prognosis of many malignancies.53 The circulatory system irregularities that follow the TME’s fast pace of angiogenesis, the high interstitial pressure within the tumor, plus these factors all work against immune cell infiltration as well as checkpoint inhibitor penetration.50 Furthermore, tumor resistance to immune checkpoint blockers may potentially be influenced by tissue-specific stromal cells. The migration of cancer cells, the control of therapy response and the advancement of cancer are all significantly impacted by the tumor extracellular matrix.54 Another element that influences tumor immunogenicity through several processes is the metabolic status of the tumor microenvironment. Less frequently, dendritic cells plus tumor cells in hypoxic malignancies exhibit type I Major Histocompatibility Complex (MHC).55 Dysregulation of mitochondrial biosynthesis was also observed in Natural Killer (NK) cells infiltrated with tumors and in T lymphocytes that were depleted. Because of this, scientists are now interested in developing methods to increase tumor immunogenicity by enhancing mitochondrial production.56 Aside from the significant effect of hypoxia on tumor immunogenicity, researchers are actively exploring novel mechanisms of immune escape plus confrontation to ICBs. These mechanisms may include other elements of the TME regulated by metabolic conditions, such as variations in nutrient supply.55 For instance; hypoxia has an impact on exosome content and release.57 Exosomes are defined as biologically active lipid bilayer nanoparticles secreted by a number of cells that facilitate cell-to-cell signaling.58 The advancement of cancer depends on these TME interactions. Evidence points to hypoxia as the cause of more aggressive cancer phenotypes. It has been demonstrated that non encoding RNAs, passing via exosomes commencing hypoxic tumors are vital molecules that regulate cancer biology plus change the TME. Furthermore, as possible diagnostic and prognostic indicators, exosomal ncRNAs from hypoxic malignancies have been found in bodily fluids.59 Furthermore, in patients with melanoma undergoing immunotherapy, analyses of human samples revealed differences amid responders plus non-responders in the composition of the tumor microbiome.60 Tumor-associated bacteria have been related to immunosuppressive microenvironment remodeling and decreased immune cell infiltration. Another element of the TME, intratumoral microorganisms, was previously underappreciated, but studies have demonstrated that they have a major impact on ICB responses as well as tumor immune responses.61 The three main types of TME features include immunosuppression, hypoxia and chronic inflammation. Together, they form a complicated molecular network that is essential to several stages of tumor development, including distant metastasis, immune escape and local treatment resistance.
Metabolic Crosstalk is The Primary Driver of Cancer Progression in the Tumor Microenvironment
Studies show to facilitate modifications to tumor metabolism providesanadequateenergysource,providethemetabolichalf-way needed for tumor development, plus suppress the antitumor reaction. Tumor and immune cells have reciprocal metabolic needs, which often characterize TME immunosuppression. Tumor cells have a higher need for amino acids and glucose when NK as well as cytotoxic T-cell commencement occurs.62 The competition for metabolites resulting from this metabolic interdependency limits the growth and effector function of immune cells specific to tumors. Furthermore, tumor-driven alterations in metabolite abundances plus the accumulation of metabolic squander goods (such as lactic acid) lead to local immunosuppression, which promotes tumor spread and progression.63 The way that immune cells and cancer cells interact is greatly influenced by metabolism, which also affects how the immune system responds to tumors. Glycolysis, lactic acid production and TME acidification are the hallmarks of the majority of cancer cells. These processes are linked to a number of aggressive characteristics, such as immune evasion.64 The initial stage in the glycolysis process, from glucose to glucose-6-phosphate, is catalyzed by Hexokinases (HK). It has been shown that HK2, among the four kinds found in tissues of mammals, is notably overexpressed in cancerous tissues. For a mouse model of breast cancer driven by ErB2/Her2, it has been demonstrated that HK2 is necessary for carcinogenesis, plus HK2 ablation inhibits breast cancer cells aggressive behaviour in both vitro as well as in vivo. In addition, Phosphoenolpyruvate (PEP) is converted to pyruvate during the third stage of glycolysis, which is catalyzed by Pyruvate Kinase (PK). Many cancer types have highly proliferating cells that exhibit high expression of the PKM2 isoform. PKM2 is essential for glycolysis, but it also functions as a protein kinase and coactivator in carcinogenesis.65 A meta-analysis published recently highlighted the importance in terms of clinical enhanced PKM2 in breast cancer, finding that high expression of PKM2 was linked to lymph node metastases and expected a low rate of endurance in patients with breast cancer.66 Reprogramming takes place in tumors and their constituent cells when they construct pro-tumorigenic cocoons. This allows the tumors to take advantage of interactions with surrounding cells to guarantee a steady contribute of anaplerotic chemicals plus nutrients to support tumor cell development, still in hypoxic environments.67 Furthermore, immunological attack and treatment resistance are suppressed by tumor cell metabolic reprogramming. The IGF1R/mTOR pathway is the most frequently erroneously activated growth factor signalling cascade. IGF-1 controls osteogenesis plus equilibrium, both of which are crucial to the pathophysiology of sarcoma. The IGF-1 signaling pathway, which regulates a number of cellular processes such as protein synthesis, metabolism, cell survival, plusas well as proliferation via PI3K/Akt/Mtor, is one of the mechanisms, however, by which many solid tumors show dysregulation of the pathway mediated by PI3K/Akt downstream of development feature receptor tyrosine kinase.68 Osteosarcoma (OS) as well as Ewing Sarcoma (ES) is thought to be attracted to the IGF-1R/ mTOR pathway because it is often triggered in sarcomas plus additional concrete tumors and reflects the combination of numerous chemical plus mechanical signaling pathways. Tumor cell proliferation, metastasis and treatment resistance are supported not only by intrinsic heritable plus epigenetic alterations in the cells, however in addition by metabolic rivalry plus collaboration among different components of the TME.69 The metabolic complexity and adaptability mentioned above pose a significant risk to our capacity to create medications that specifically target TME metabolic markers.
The immune cell population in the tumor microenvironment is substantial. Tumor microenvironment and cell surface immunological checkpoints control the interaction of immune cells with tumor cells plus resistant cells with additional immune cells. Tumor stem cells are responsible for the formation of malignancies and have an impact on their inherent heterogeneity.70 During carcinogenesis, Mast Cells (MC) are drawn to areas close to tumors and emit a range of cytokines, chemokines, etc. Tumor necrosis factor and perforin are secreted by Natural Killer cells (NK), which are cytotoxic plus kill tumor cells.71 Tumor-associated macrophages are macrophages that settle around Tumor cells (TAM). These macrophages fall into two primary categories: M1 type, which is traditionally activated and M2 type, which is alternatively activated. M2 type macrophages have the ability to aid in the immune system’s escape from malignancies. As antigen-presenting cells, Dendritic Cells (DC) can trigger immunological responses, but they frequently collapse and undergo apoptosis in tumor microenvironment (TME).72 Myeloid-derived suppressor cells (MDSC) can thwart immunotherapy, depress the immune system and contribute to the development and maintenance of tumors.73 The immune system can be suppressed by a subpopulation of T cells called regulatory T-cells (Tregs). Tregs can also inhibit T cell initiation plus propagation and a high concentration of Tregs in Tumor Microenvironment (TME) is frequently associated with a poor prognosis. In TME, normal fibroblasts have the potential to become Cancer-Associated Fibroblasts (CAFs), which can create an environment that is conducive to tumor cell proliferation and metastasis.74
OTHER FACTORS
Three-dimensional in vitro cultures were used to represent the dynamic, prolonged interactions among matrix elements plus cancerous epithelial breast cells (fibroblasts, endothelial cells and lymph cells).75 The process of protein hydrolysis that occurs in the TME following tumor cell invasion could now be seen thanks to this. The tumor microenvironment plays crucial roles in the preservation of tumor stem cell properties and the reprogramming of mesenchymal stem cells. Matrix factors facilitate angiogenesis and aid in the development plus increase of tumors. Reactive Oxygen Species (ROS) generation as well as the inflammatory response in the microenvironment plays key parts in the development of cancer. NO produced by the matrix stimulates the signalling pathways that support malignancy in breast cancer cells that lack Estrogen Receptors (ER). It also causes white blood cells to produce IL-10 and TGF-β, which suppress the immune system. iNOS is a potent predictor of a poor prognosis.
Potential carcinogens may affect epithelial cells-the most prevalent cells from which cancer originates-immune cells, extracellular matrix constituents and stromal cells in the TME to stimulate various phases of tumor growth. Thus, additional research into the impacts of substances plus mixtures on the TME, in relation to dose may disclose significant broad processes of tumorigenesis prevention plus etiology.76 Individual feature of tumor microenvironment is hypoxia, which as well causes PD-L1 overexpression. Under hypoxic conditions, Nuclear Factor kappa B (NF-κB) plus Hypoxia-Induced Factor 1 alpha (HIF-1α) are activated.77 By acting on the Hypoxia Response Element-4 (HRE-4) of the PD-L1 proximal supporter, HIF-1α can enhance the production of PD-L1 mRNA. Furthermore, to causing immune cell activation, chronic inflammation and cell damage, hypoxia can also produce cytokines like TNF-α plus IFN-γ, this could lead to PD-L1 expression in cells.77 PD1 expression is induced by cytokines, specifically IL-2, IL-6, IL-15, IFN-α, plus IFN-β, through their respective receptors as well as signaling conduits. For instance, IL-6 modifies the PD1 chromatin structure and enhances PD1 transcription via the STAT3-STAT4 pathway as well as throughout TCR commencement. (B) PDL1’s epigenetic alteration and expression regulation: Hypoxia factors in the TME boost PDL1 expression is mediated via passing through overactive HIF-1α,
NF-κB and common cytokines. This includes HIF-1α binding to its proximal promoter. (C) PDL1 translation and regulation of the PD-L1 protein: During translation, small molecules like as miR-200 and miR-34a can bind to one end of the PDL1 mRNA and impede transcription; EGF controls the deprivation of the PDL1 protein; VEGF plus FGF also influence the translation via equivalent signal conduits. Exosomes are a means of secreting the produced PD-L1, which is also articulated on the membrane. Alterations in homeostasis and an aberrant immune response control the development of tumors.78 The progression plus spread of cancer in the TME are influenced by interactions among immune cells plus cancerous cells.79,50
Tumor Microenvironment Plays a Crucial Part in the Parameter of Pd-1 Plus Pd-L1 Expression
Tumor immune escape is a crucial approach used by tumors to boost their chances of survival. It involves a number of processes, one of which is immunosuppression, which has been the focus of much recent research.80 There are two basic mechanisms of action for tumor-induced immunosuppression. In order to lower the immune acceptance of tumor cells, immunosuppressive cells such as regulatory T cells (Treg cells), Myeloid Suppressor Cells (MDSCs), Dendritic Cells (DCs), plus M2-type macrophages are first stimulated to aggregate about the tumor and release immunosuppressive features that inactivate CTLs.81
Second, the expression of molecules or receptors that suppress the immune system is stimulated. These include immune benchmarks such as Indoleamine 2,3-Doxygenase 1 (IDO1), PD-L1/PD-1, Lymphocyte Activating 3 (LAG3), galectin-9-T cell immunoglobulin mucin 3 (TIM3), as well as Cytotoxic T-Lymphocyte associated protein 4 (CTLA4). These molecules prevent effector T lymphocyte activation, which allows tumors to evade the immune system. Thus, to lessen immunosuppression and reestablish immune system function, immunotherapy research has been focusing on blocking these immunological checkpoints recently.81
The cell surface expresses the Ig receptors PD-L1 plus PD-L2, which work in conjunction with PD-1 to facilitate tumor immune evasion.82 PD-L1 is present in both hematological and nonhematopoietic cells and its expression is regulated by interferon-gamma (IFN-γ) plus cytokines such as IL-7 as well as IL-15.51,83,84 The TME’s T cell activation, proliferation, plus consequent cytotoxicity can all be inhibited by the PD-1/ PD-L1 conduits.80 The effect of anti-PD-1/PD-L1 therapy is also highly linked with PD-L1 expression in non-small cell lung cancer, metastatic urethral carcinoma, melanoma, plus different cancers.85 While PD-L1 as well as PD-L2 expression in tumor cells can considerably inhibit the anti-tumor immune reaction.85,86 PD-L2 appears on the facade of dendritic cells, macrophages, mast cells, as well as certain B cell organizations. PD-L2 is highly expressed in the Tumor Microenvironment (TME) of Lung Squamous Cell carcinoma (LUSC) and Renal Cell Carcinoma (RCC).86 In the near opportunity, anti-PD-L2 monoclonal antibody drugs may be able to circumvent the challenges faced by anti-PD-L1 monoclonal antibody drugs. The TME has intricate molecular signaling networks where cytokines plus development features influence associated signal conduits plus control the production of PD-1 as well as PD-L1.51,87 The TME has the ability to upregulate PD-L1 expression via increasing IFN-γ, TNFα, as well as IL-6. For example, IL-6 can strengthen the connection with the catalytic subunit A (STT3A) of the n-glycosyltransferase STT3 oligosaccharyltransferase complex and raise the production of PD-L1 via the IL-6-Janus kinase 1 (Jak1) conduits.88 PDL1 mRNA translation in the TME can be blocked by raising the level of expression of miR-155, or the translation inhibition associated with miR-155 can be lessened by downregulating the expression of miR-155, which will raise the levels of PD-L1 protein.89
Furthermore, different cells within the TME secrete exosomes, which are bioactive lipid bilayer nanovesicles with a variety of bioactive compounds including proteins, RNA, DNA and miRNAs.90 PD-L1 can generate drug resistance in receptor cells, spread to other tumor cells via exosomes and encourage the growth and metastasis of tumors.91 For instance, PD-L1- containing exosomes released by HER2 cells might cause treatment resistance in cells that are susceptible to HER2-targeted medications (Table 2).92
Table 2:
Checkpoint molecules of Immune along with its biological functions, receptor and cell type.
Receptor | Cell nature | Biological functions | References |
---|---|---|---|
CTLA-4 | T-cell | CD28 is a sovereign T-cell c-stimulator that enhances TCR conduits: its inhibition of costimulation inhibits T-cell reactions. Inside the T-cells the interior commencement of TCR conduits is inhibited by activating SHP2 phosphatase. The censoring role of regulatory T-cells is operated. | 93 |
PD-1 | T-cells, B-cells, Natural killer cells. | Inhibitory phosphatase SHP-2 as well as PP2A is recruited to straightly hinder TCR signalling. | 93 |
Myeloid-derived suppressor cells | NK cells and B-cells are hindered. CTLs is inhibited by enhancing Treg plus MDSC function. | ||
PD-L1 | T-cells, B-cells, Natural killer cells, Macrophages, Epithelial cells Endothelial cells. | T-cell reactions are inhibited. | 93 |
Therapeutic Implications
For cancer cells to initiate tumorigenesis, a vast array of genetic alterations is necessary. Despite significant advancements, the clinical treatment of numerous human cancer types has primarily targeted the malignant cancer cell itself. Despite this, cancer therapy continues to pose significant challenges. The most widely utilized radiation and chemotherapy techniques at the moment have major adverse effects, like the patient’s immune system being destroyed and patients quickly become resistant to treatment. As previously mentioned, the tumor microenvironment frequently engage in recreation in both the expansion plus progression of tumors in a variety of tumor types, offering us hope that therapeutically focusing on these mechanisms may be successful in treating cancer. Well-built proof for the part of tumor stromal cells in the formation of the TME in chemoresistance be able to be found in recent articles. For instance, when given doxorubicin, CCR2 deficient congregation mice fared enhanced than a power group. This resulted from the conscription of myeloid cells to tumors treated with doxorubicin via the stromal CCL2/ CCR2 chemokine/chemokine receptor alliance, which causes chemoresistance. In a similar vein, endogenous Mesenchymal Stem Cells (MSCs) stimulated by platinum analog therapy emission of polyunsaturated fatty acids that protects cancer cells from a variety of chemotherapy drugs.94 These remarkable results demonstrate the environment surrounding a tumor is a powerful regulator of confrontation to conventional cytotoxic treatments, primarily radiotherapy plus chemotherapy, as well as they identify putative goals to improve patients’ response to chemotherapy.
In recent years, multitargeted methods have been developed that involve simultaneous inhibition of the tumor microenvironment and tumor cells. Comparing these multitargeted strategies to conventional medicines reveals numerous benefits. Although it is well established that tumor cells are genetically unstable, stromal cells in the surrounding tissue of the tumor are most likely genetically steady. As a result, people with cancer are fewer expected to have adaptive mutations or quickly develop resistance to radiation and chemotherapy. However, there are some similarities between the tumor microenvironment of different cancer kinds, which means that a single therapeutic aim within the TME may be connected to multiple cancer types. Many tools, such fluid biopsy as well as in silico molecular ecology, have been created for the study of research-based cancer into the tumor microenvironment.94 Regarding patients with bladder cancer that is not muscle-invasive, the liquid biopsy offers a non-invasive method of detecting bladder cancer by analyzing tumor DNA in the urine.94 For the purpose of cancer therapy, GLUT4-specific inhibitors are found using in silico biomarker profiling technology.95 These findings have significant implications for customized/precision treatment and personalized detection.
Focusing on the tumor microenvironment has considerable promise for cancer therapy because of these benefits. The TME contains an assortment of components that endorse tumor development, which suggests that blocking these factors or severing these pathways of signaling can stop cancer from developing. For instance, tumors treated with a TGF-β inhibitor in a stroma xenograft form showed a decrease in blood vessels.94 Since stromal cells may provide cysteine, which I essential for leukemia cell viability plus medication resistance, which is enhanced by GSH synthesis in CLL cells, blocking the transporter to influence stromal cell uptake of cysteine plus remove the stromal protection of CLL cells may be a useful treatment for CLL, especially when used in conjunction with conventional medications. Moreover, the remodeling of extracellular matrix can be regulated by a variety of families of matrix-degrading enzymes, such as chymases, tryptases, MMPs plus heparanase.94 Inhibitors of these enzymes, like the heparanase inhibitor PI-88, may be used to treat cancer by halting the multistep process in the TME. That being said, some MMP-produced fragments of basement membrane collagen serve as endogenous integrin-mediated suppressors of tumor development plus pathologic angiogenesis.94 This observation suggests that the careful balancing act between stromal cells’ tumor-inhibitory and tumor-promoting roles needs to be taken into account. Furthermore, therapy shouldn’t interfere with stromal cells’ ability to operate normally. To create cancer prevention strategies that are more effective, further study is required.
Future Perspective
Originally believed to be a problem of gene plus cell appearance, cancer is now recognized as a tumor microenvironment illness. TME individuality have the potential to uncover novel biomarkers for prognosis plus prediction, which could lead to the discovery of new therapeutic goals plus the tactics that go along with them. These discoveries could possibly inform algorithms used to determine first-line therapy. While each patient’s primary lesion site has different TME drivers, most individuals share several characteristics. Finding an anti-tumor milieu, which is defined by a high concentration of Th1 cells, CD8+ T cells, as well as the cytokines they produce, typically indicates that the immune system has partially or completely eradicated the tumor. Considerating the intricacy of the tumor microenvironment plus how it affects reactions to different anti-cancer medicines, particularly immunotherapy, has advanced significantly over the last 20 years.63 Due to the significant clinical success of immunotherapies that target CTLA-4 and PD-1, more research is being done to better understand tumor immunity. Excellent activity is shown by other receptors, the Nectin family (it comprises the CD226 activating receptor plus its primary negative regulatory counterpart, the T cell immune receptor), the activated receptors on T cells include TNFRSF4, CD27 (also known as TNFRSF7), GITR (also known as TNFRSF18), CD137 agonist antibodies, plus LAG3, a negative regulator of CD4+ T cell activation.
Agonists haven’t, on the other hand, demonstrated any appreciable clinical activity in humans. Apoptosis of T cells may result from over-commencement, down-regulation of goal receptors, inadequate investigation of the dosage and procedure necessary for activation and insufficient agonist activity.96 Cancer patients’ responsiveness to treatment and chances of survival are influenced by their tumor immune environment. Finding therapies which can lessen immunosuppression, ease T cell breakdown, as well as improve tumor microenvironment effector purpose is crucial. Specifically, it is imperative to create arrangement therapy for the tumor microenvironment can lower the quantity of immunosuppressive immune cells (like TAMs plus FOXP3+ Tregs) while increasing the activity of CD4+ as well as CD8+ effector T cells.97 These therapies may be able to modify the TME and stimulate the immune system against malignancy.
It is now urgently necessary to figure out how to increase the aim reaction pace in cancer patients who do not respond to immune checkpoint blockade therapy. LIGHT, TNF Superfamily Member 14 (TNSF14), an affiliate of the tumor necrosis factor superfamily, targets tumors by triggering signals from lymphocytic toxin receptors. This results in the generation of chemokines plus the conscription of a significant number of T lymphocytes. Additionally, antibody-guided LIGHT overcomes tumor resistance to checkpoint inhibition by focusing on non-T-cell-inflamed tumor tissue and establishing a T-cell inflammatory milieu.
According to data, checkpoint inhibition and other immunotherapies may be more effective against non-T- cell inflammatory cancers when targeting LIGHT.98 The characterization of the organizers immune response against the tumor by multiplex as well as multimodal biomarkers may one day aid in the prediction of which patients might get advantages from immune-based therapies. Immunomodulatory Compounds (ICIs) that aim the PD-1/PD-L1 alliance enclose showed significant promise as possible therapeutic targets in immunological microenvironments have been found by research. The FDA has approved anti PD-1/PD-L1 treatments for a range of tumor types, some of which can be diagnosed with PD-L1 immunohistochemistry. There are still questions about how specific carcinogenic drivers in tumor cells figure plus promote the TME and how this adds to the diversity of stromal cells, often in the same tissues.79 Other difficulties include determining whom immunotherapy would be beneficial for, figuring out the best anticancer and TME-targeted medication combinations and figuring out how to mitigate or get around intrinsic or acquired resistance in the TME. The TME’s capacity to have both positive and negative effects on carcinogenesis exacerbates these difficulties. Furthermore, the TME has the ability to regularize cells of the tumor, suggesting that retraining of stromal cells could be a more effective cancer treatment strategy than targeted tumor ablation.99 Future research on malfunctioning TME re-education may prove very beneficial in controlling and mitigating disease, as seen by the noteworthy cancer immunotherapies current level of success.
Finding important drug-acting components as well as conduits in the tumor microenvironment to increase the effectiveness of existing cancer conducts is a significant problem because the TME is one of the primary characteristics of cancer. An FDA approval goes to just 13.8% of pharmaceuticals that are in clinical trials; Pharma companies recently conducted a study and discovered that the standard study and about $648 million (USD) was needed for the development of an effective therapy. The success rate of cancer medications was found to be lower, at about 3.4%. Due in part to the lack of translatability in the present in vitro models, the failure rate of early drug trials was high. Since single-level replicas have shown to be insufficient, scientists have begun modeling tumors in three Dimensions (3D).100–102 Information on the cellular characteristics, mechanisms of disease progression, plus reactions to therapy in sarcoma, with a focus on chemical physical cues in TME, have been made available by means of such 3D-engineered tumor models. TME biological signals, including the interstitial and tumor vascular system, have been evaluated; little research has been done on immune cells invading tumor engineering models.67 One of the biggest obstacles to identifying metabolic markers in the TME is the complexity and adaptability of metabolism.103–105 To identify the process of metabolic reprogramming linked to cancer, the metabolic flux should be measured in the future. Kinetic or constraint modeling can be used to evaluate this type of metabolic flow at the genome level. The steady-state metabolic flux is stimulated by constraint-based models using a set of linear constraints.106 Cell fluxes and concentrations can be evaluated using dynamic (kinetic) models to determine their transient behavior as well as their steady-state values.107 Context-specific replicas built by incorporating cell or tissue-specific information can reflect metabolic properties specific to a given cell type or tissue. Since the metabolic interaction among resistant cells plus tumors in the tumor microenvironment cannot be fully modeled by current modeling frameworks, new mixed kinetic/ stoichiometry formulations may be necessary in the future.
CONCLUSION
The field of Tumor Microenvironment (TME) is a relatively new one that presents both opportunities and challenges. Gaining a thorough understanding of TME as well as its function in tumor immune growth and progression will transform our understanding of the relationship between tumor, immune system and precision medicine. TME affects cancer patients’ response to treatment and chances of survival. Among the challenges faced by TME is determining the best therapeutic modalities to reduce immunosuppression, lessen T cell failure, as well as improve effector purpose. Reeducating TME immune cells is especially important to limit the buildup of TME. It is becoming increasingly obvious that the TME is a heterogeneous mixture of tumor cells plus endogenous host stroma that co-evolves as the illness worsens. Importantly, the cells of the stromal compartment of tumors, which include MSCs, fibroblasts, endothelial cells, pericytes, adipocytes and immune cells, are all necessary for the development of the tumor microenvironment, metastasis, immune infiltration plus inflammation, as well as therapeutic approaches. Recognizing the TME’S intricacy as well as how it affects reactions to different anticancer medicines, particularly immunotherapies, has advanced significantly over the past 20 years. Cancer immunotherapy works by controlling the immune system of the deceased to identify plus eliminate cancer cells. It has shown promising results in delighting a variety of concrete tumors and hematological malignancies. Recently, the development of Antigen-Chimeric T cells (CAR-T), tumor vaccines and the inhibition of Programmed Death-1 (PD-1), Programmed Death-1 Ligand-1 (PD-L1), plus Programmed Death Ligand-2 (PD-L2) have all gained popularity as immunotherapies. TME is closely linked to tumorigenesis, progression and metastasis. As a result, we go over the traits of different TME cells and chemicals, how PD-1 and TME interact and potential cancer immunotherapy treatments.
Cite this article:
Althobiti MM. Tumor Microenvironment-Different Cells and Functions in the Metastasis and Progression of Cancer. Int. J. Pharm. Investigation. 2025;15(1):86-102.
ACKNOWLEDGEMENT
The author appreciates the Shaqra University for their support during this work
CONFLICT OF INTEREST
The authors declare that there is no conflict of interest.
ABBREVIATIONS
CAR-T | Antigen-chimeric T cells |
---|---|
PD-1 | Programmed death-1 |
PD-L1 | Programmed Death-1 Ligand-1 |
PD-L2 | Programmed Death-2 |
TME | Tumor microenvironment |
MDR | Multi-drug resistance |
CTCs | Circulating tumor cells |
cfDNA | Cell-free DNA |
HGT | Horizontal gene transfer |
EMT | Epithelial-mesenchymal transition |
ECM | Extracellular matrix |
TCR | T cell receptor |
VEGF | Vascular endothelial growth factor |
MMP | Matrix metalloprotease |
HIFs | Hypoxia inducible factors |
PDGF | Platelet-derived growth factor |
BMP | Bone morphogenetic protein |
HCC | Hepatocellular carcinoma |
PSCs | Pancreatic stellate cells |
MSCs | Mesenchymal stem cells |
FAK | Focal adhesion kinase |
P4Has | Prolyl-4-hydroxylases |
PGs | Proteoglycans |
GAGs | Glycosaminoglycans |
CRC | Colorectal cancer |
4-MU | 4methylumbelliferone |
ICB | Immune checkpoint blockade |
ROS | Reactive oxygen species |
CTLA4 | Cytotoxic T-lymphocyte associated protein 4 |
LAG3 | Lymphocyte activating 3 |
References
- Bussard KM, Mutkus L, Stumpf K, Gomez-Manzano C, Marini FC. Tumor-associated stromal cells as key contributors to the tumor microenvironment. Breast Cancer Res. 2016;18(1):84. [CrossRef] | [PubMed]
- Jahanban-Esfahlan R, Seidi K, Monhemi H, Adli AD, Minofar B, Zare P, et al. RGD delivery of truncated coagulase to tumor vasculature affords local thrombotic activity to induce infarction of tumors in mice. Sci Rep. 2017;7(1):8126. [CrossRef] | [PubMed]
- Jahanban-Esfahlan R, Seidi K, Banimohamad-Shotorbani B, Jahanban-Esfahlan A, Yousefi B. Combination of nanotechnology with vascular targeting agents for effective cancer therapy. J Cell Physiol. 2018;233(4):2982-92. [CrossRef] | [PubMed]
- Jahanban-Esfahlan R, Seidi K, Zarghami N. Tumor vascular infarction: prospects and challenges. Int J Hematol. 2017;105(3):244-56. [CrossRef] | [PubMed]
- Frisch J, Angenendt A, Hoth M, Prates Roma L, Lis A. STIM-Orai channels and reactive oxygen species in the tumor microenvironment. Cancers. 2019;11(4):457. [CrossRef] | [PubMed]
- Denisenko TV, Budkevich IN, Zhivotovsky B. Cell death-based treatment of lung adenocarcinoma. Cell Death Dis. 2018;9(2):117. [CrossRef] | [PubMed]
- Jahanban-Esfahlan R, de la Guardia M, Ahmadi D, Yousefi B. Modulating tumor hypoxia by nanomedicine for effective cancer therapy. J Cell Physiol. 2018;233(3):2019-31. [CrossRef] | [PubMed]
- Jahanban-Esfahlan R, Seidi K, Manjili MH, Jahanban-Esfahlan A, Javaheri T, Zare P. Tumor cell dormancy: threat or opportunity in the fight against Cancer. Cancers. 2019;11(8):1207. [CrossRef] | [PubMed]
- Seidi K, Neubauer HA, Moriggl R, Jahanban-Esfahlan R, Javaheri T. Tumor target amplification: implications for nano drug delivery systems. J Control Release. 2018;275:142-61. [CrossRef] | [PubMed]
- Li W, Ng JM, Wong CC, Ng EK, Yu J. Molecular alterations of cancer cell and tumour microenvironment in metastatic gastric cancer. Oncogene. 2018;37(36):4903-20. [CrossRef] | [PubMed]
- Tsao AS, Scagliotti GV, Bunn Jr PA, Carbone DP, Warren GW, Bai C, et al. Scientific advances in lung cancer 2015. J Thorac Oncol. 2016;11(5):613-38. [CrossRef] | [PubMed]
- Cova TF, Bento DJ, Nunes SC. Computational approaches in theranostics: mining and predicting Cancer data. Pharmaceutics. 2019;11(3):119. [CrossRef] | [PubMed]
- Oliver AJ, Lau PK, Unsworth AS, Loi S, Darcy PK, Kershaw MH, et al. Tissue-dependent tumor microenvironments and their impact on immunotherapy responses. Front Immunol. 2018;9:70. [CrossRef] | [PubMed]
- Ayoubi-Joshaghani MH, Dianat-Moghadam H, Seidi K, Jahanban-Esfahalan A, Zare P, Jahanban-Esfahlan R. Cell-free protein synthesis: the transition from batch reactions to minimal cells and microfluidic devices. Biotechnol Bioeng. 2020;117(4):1204-29. [CrossRef] | [PubMed]
- Sleeboom JJ, Eslami Amirabadi H, Nair P, Sahlgren CM, den Toonder JM. Metastasis in context: modeling the tumor microenvironment with cancer-on-a-chip approaches. Dis Model Mech. 2018; 11(3):dmm033100. [CrossRef] | [PubMed]
- Anderson NM, Simon MC. The tumor microenvironment. Curr Biol. 2020; 30(16):R921-5. [CrossRef] | [PubMed]
- Yang L, Zhang Y. Tumor-associated macrophages: from basic research to clinical application. J Hematol Oncol. 2017;10(1):58. [CrossRef] | [PubMed]
- Funes SC, Rios M, Escobar-Vera J, Kalergis AM. Implications of macrophage polarization in autoimmunity. Immunology. 2018;154(2):186-95. [CrossRef] | [PubMed]
- Rőszer T. Understanding the mysterious M2 macrophage through activation markers and effector mechanisms. Mediators Inflamm. 2015; 2015:816460. [CrossRef] | [PubMed]
- Motta JM, Rumjanek VM. Sensitivity of dendritic cells to microenvironment signals. J Immunol Res. 2016; 2016:4753607. [CrossRef] | [PubMed]
- Collin M, Bigley V. Human dendritic cell subsets: an update. Immunology. 2018;154(1):3-20. [CrossRef] | [PubMed]
- Conejo-Garcia JR, Rutkowski MR, Cubillos-Ruiz JR. State-of-the-art of regulatory dendritic cells in cancer. Pharmacol Ther. 2016;164:97-104. [CrossRef] | [PubMed]
- Guilliams M, Dutertre CA, Scott CL, McGovern N, Sichien D, Chakarov S, et al. Unsupervised high-dimensional analysis aligns dendritic cells across tissues and species. Immunity. 2016;45(3):669-84. [CrossRef] | [PubMed]
- Chen K, Wang JM, Yuan R, Yi X, Li L, Gong W, et al. Tissue-resident dendritic cells and diseases involving dendritic cell malfunction. Int Immunopharmacol. 2016;34:1-15. [CrossRef] | [PubMed]
- Gueguen C, Bouley J, Moussu H, Luce S, Duchateau M, Chamot-Rooke J, et al. Changes in markers associated with dendritic cells driving the differentiation of either TH2 cells or regulatory T cells correlate with clinical benefit during allergen immunotherapy. J Allergy Clin Immunol. 2016;137(2):545-58. [CrossRef] | [PubMed]
- Uribe-Querol E, Rosales C. Neutrophils in cancer: two sides of the same coin. J Immunol Res. 2015; 2015:983698. [CrossRef] | [PubMed]
- Bronte V, Brandau S, Chen SH, Colombo MP, Frey AB, Greten TF, et al. Recommendations for myeloid-derived suppressor cell nomenclature and characterization standards. Nat Commun. 2016;7:12150. [CrossRef] | [PubMed]
- Kumar V, Patel S, Tcyganov E, Gabrilovich DI. The nature of myeloid-derived suppressor cells in the tumor microenvironment. Trends Immunol. 2016;37(3):208-20. [CrossRef] | [PubMed]
- Stabile H, Fionda C, Gismondi A, Santoni A. Role of Distinct Natural Killer Cell Subsets in Anticancer Response. Front Immunol. 2017;8:293. [CrossRef] | [PubMed]
- Flores-Borja F, Irshad S, Gordon P, Wong F, Sheriff I, Tutt A, et al. Crosstalk between innate lymphoid cells and other immune cells in the tumor microenvironment. J Immunol Res. 2016; 2016:7803091. [CrossRef] | [PubMed]
- Van Beek JJ, Martens AW, Bakdash G, de Vries IJ. Innate Lymphoid Cells in Tumor Immunity. Biomedicines. 2016;4(1):7. [CrossRef] | [PubMed]
- Henke E, Nandigama R, Ergün S. Extracellular matrix in the tumor microenvironment and its impact on cancer therapy. Front Mol Biosci. 2019;6:160. [CrossRef] | [PubMed]
- García R, Merino D, Gómez JM, Nistal JF, Hurlé MA, Cortajarena AL, et al. Extracellular heat shock protein 90 binding to TGFbeta receptor I participates in TGFbeta-mediated collagen production in myocardial fibroblasts. Cell Signal. 2016;28(10):1563-79. [CrossRef] | [PubMed]
- Fang S, Dai Y, Mei Y, Yang M, Hu L, Yang H, et al. Clinical significance and biological role of cancer-derived type I collagen in lung and esophageal cancers. Thorac Cancer. 2019;10(2):277-88. [CrossRef] | [PubMed]
- Miskolczi Z, Smith MP, Rowling EJ, Ferguson J, Barriuso J, Wellbrock C. Collagen abundance controls melanoma phenotypes through lineage-specific microenvironment sensing. Oncogene. 2018;37(23):3166-82. [CrossRef] | [PubMed]
- Rossow L, Veitl S, Vorlová S, Wax JK, Kuhn AE, Maltzahn V, et al. LOXcatalyzed collagen stabilization is a proximal cause for intrinsic resistance to chemotherapy. Oncogene. 2018;37(36):4921-40. [CrossRef] | [PubMed]
- Xiong G, Stewart RL, Chen J, Gao T, Scott TL, Samayoa LM, et al. Collagen prolyl 4-hydroxylase 1 is essential for HIF-1alpha stabilization and TNBC chemoresistance. Nat Commun. 2018;9(1):4456. [CrossRef] | [PubMed]
- Passi A, Vigetti D, Buraschi S, Iozzo RV. Dissecting the role of hyaluronan synthases in the tumor microenvironment. FEBS Journal. 2019;286(15):2937-49. [CrossRef] | [PubMed]
- Ikuta K, Ota T, Zhuo L, Urakawa H, Kozawa E, Hamada S, et al. Antitumor effects of 4-methylumbelliferone, a hyaluronan synthesis inhibitor, on malignant peripheral nerve sheath tumor. Int J Cancer. 2017;140(2):469-79. [CrossRef] | [PubMed]
- Karalis TT, Heldin P, Vynios DH, Neill T, Buraschi S, Iozzo RV, et al. Tumorsuppressive functions of 4-MU on breast cancer cells of different ER status: regulation of hyaluronan/HAS2/CD44 and specific matrix effectors. Matrix Biol. 2018:78-9, 118-38. [CrossRef] | [PubMed]
- Jadin L, Pastorino S, Symons R, Nomura N, Jiang P, Juarez T, et al. Hyaluronan expression in primary and secondary brain tumors. Ann Transl Med. 2015;3(6):80. [CrossRef] | [PubMed]
- Zhang H, Tsang JY, Ni YB, Chan SK, Chan KF, Cheung SY, et al. Hyaluronan synthase 2 is an adverse prognostic marker in androgen receptor-negative breast cancer. J Clin Pathol. 2016;69(12):1055-62. [CrossRef] | [PubMed]
- Sukowati CH, Anfuso B, Fiore E, Ie SI, Raseni A, Vascotto F , et al. Hyaluronic acid inhibition by 4-methylumbelliferone reduces the expression of cancer stem cells markers during hepatocarcinogenesis. Sci Rep. 2019;9(1):4026. [CrossRef] | [PubMed]
- Qiu X, Tan H, Fu D, Zhu Y, Zhang J. Laminin is over expressed in breast cancer and facilitate cancer cell metastasis. J Cancer Res Ther. 2018;14;Suppl:S1170-2. [CrossRef] | [PubMed]
- Tomás-Bort E, Kieler M, Sharma S, Candido JB, Loessner D. 3D approaches to model the tumor microenvironment of pancreatic cancer. Theranostics. 2020;10(11):5074-89. [CrossRef] | [PubMed]
- Sceneay J, Griessinger CM, Hoffmann SH, Wen SW, Wong CS, Krumeich S, et al. Tracking the fate of adoptively transferred myeloid-derived suppressor cells in the primary breast tumor microenvironment. PLOS ONE. 2018;13(4):e0196040. [CrossRef] | [PubMed]
- Hinshaw DC, Shevde LA. The tumor microenvironment innately modulates cancer progression. Cancer Res. 2019;79(18):4557-66. [CrossRef] | [PubMed]
- Hope JL, Spantidea PI, Kiernan CH, Stairiker CJ, Rijsbergen LC, van Meurs M, et al. Microenvironment-dependent gradient of CTL exhaustion in the AE17sOVA murine mesothelioma tumor model. Front Immunol. 2019;10:3074. [CrossRef] | [PubMed]
- Erdogan B, Webb DJ. Cancer-associated fibroblasts modulate growth factor signaling and extracellular matrix remodeling to regulate tumor metastasis. Biochem Soc Trans. 2017;45(1):229-36. [CrossRef] | [PubMed]
- Fukumura D, Kloepper J, Amoozgar Z, Duda DG, Jain RK. Enhancing cancer immunotherapy using antiangiogenics: opportunities and challenges. Nat Rev Clin Oncol. 2018;15(5):325-40. [CrossRef] | [PubMed]
- Priego N, Zhu L, Monteiro C, Mulders M, Wasilewski D, Bindeman W, et al. STAT3 labels a subpopulation of reactive astrocytes required for brain metastasis. Nat Med. 2018;24(7):1024-35. [CrossRef] | [PubMed]
- Fiori ME, Di Franco S, Villanova L, Bianca P, Stassi G, De Maria R. Cancer-associated fibroblasts as abettors of tumor progression at the crossroads of EMT and therapy resistance. Mol Cancer. 2019;18(1):70. [CrossRef] | [PubMed]
- Park D, Sahai E, Rullan A. SnapShot: cancer-associated fibroblasts. Cell. 2020;181(2):486-486.e1. [CrossRef] | [PubMed]
- Neophytou CM, Panagi M, Stylianopoulos T, Papageorgis P. The role of tumor microenvironment in cancer metastasis: molecular mechanisms and therapeutic opportunities. Cancer. 2021;13(9):2053. [CrossRef] | [PubMed]
- Chakravarthy V, Kaplan B, Gospodarev V, Myers H, De Los RK, Achiriloaie A. Houdini tumor: case report and literature review of pregnancy-associated meningioma. World Neurosurg. 2018;114: e1261:e1265. [CrossRef] | [PubMed]
- Scharping NE, Menk AV, Moreci RS, Whetstone RD, Dadey RE, Watkins SC, et al. The tumor microenvironment represses T cell mitochondrial biogenesis to drive intratumoral T cell metabolic insufficiency and dysfunction. Immunity. 2016;45(2):374-88. [CrossRef] | [PubMed]
- Roma-Rodrigues C, Mendes R, Baptista PV, Fernandes AR. Targeting tumor microenvironment for cancer therapy. Int J Mol Sci. 2019;20(4):840. [CrossRef] | [PubMed]
- Wang J, Zeng H, Zhang H, Han Y. The role of exosomal PD-L1 in tumor immunotherapy. Transl Oncol. 2021;14(5):101047. [CrossRef] | [PubMed]
- Wang W, Han Y, Jo HA, Lee J, Song YS. Non-coding RNAs shuttled via exosomes reshape the hypoxic tumor microenvironment. J Hematol Oncol. 2020;13(1):67. [CrossRef] | [PubMed]
- Nejman D, Livyatan I, Fuks G, Gavert N, Zwang Y, Geller LT, et al. The human tumor microbiome is composed of tumor type-specific intracellular bacteria. Science. 2020;368(6494):973-80. [CrossRef] | [PubMed]
- Helmink BA, Khan MA, Hermann A, Gopalakrishnan V, Wargo JA. The microbiome, cancer and cancer therapy. Nat Med. 2019;25(3):377-88. [CrossRef] | [PubMed]
- Dias AS, Almeida CR, Helguero LA, Duarte IF. Metabolic crosstalk in the breast cancer microenvironment. Eur J Cancer. 2019;121:154-71. [CrossRef] | [PubMed]
- Noman MZ, Hasmim M, Lequeux A, Xiao M, Duhem C, Chouaib S, et al. Improving cancer immunotherapy by targeting the hypoxic tumor microenvironment: new opportunities and challenges. Cells. 2019;8(9):1083. [CrossRef] | [PubMed]
- Riera-Domingo C, Audigé A, Granja S, Cheng WC, Ho PC, Baltazar F, et al. Immunity, hypoxia and metabolism-the Menage a Trois of cancer: implications for immunotherapy. Physiol Rev. 2020;100(1):1-102. [CrossRef] | [PubMed]
- Dong G, Mao Q, Xia W, Xu Y, Wang J, Xu L, et al. PKM2 and cancer: the function of PKM2 beyond glycolysis. Oncol Lett. 2016;11(3):1980-6. [CrossRef] | [PubMed]
- Yang Y, Wu K, Liu Y, Shi L, Tao K, Wang G. Prognostic significance of metabolic enzyme pyruvate kinase M2 in breast cancer: a meta-analysis. Med (Baltim). 2017;96(46):e8690, [CrossRef] | [PubMed]
- Molina ER, Chim LK, Barrios S, Ludwig JA, Mikos AG. Modeling the tumor microenvironment and pathogenic signaling in bone sarcoma. Tissue Eng Part B Rev. 2020;26(3):249-71. [CrossRef] | [PubMed]
- Lamhamedi-Cherradi SE, Menegaz BA, Ramamoorthy V, Vishwamitra D, Wang Y, Maywald RL, et al. IGF-1R and mTOR blockade: novel resistance mechanisms and synergistic drug combinations for Ewing sarcoma. J Natl Cancer Inst. 2016; 108(12):djw182. [CrossRef] | [PubMed]
- Gupta S, Roy A, Dwarakanath BS. Metabolic cooperation and competition in the tumor microenvironment: implications for therapy. Front Oncol. 2017;7:68. [CrossRef] | [PubMed]
- Prasetyanti PR, Medema JP. Intra-tumor heterogeneity from a cancer stem cell perspective. Mol Cancer. 2017;16(1):41. [CrossRef] | [PubMed]
- Shimasaki N, Jain A, Campana D. NK cells for cancer immunotherapy. Nat Rev Drug Discov. 2020;19(3):200-18. [CrossRef] | [PubMed]
- Wculek SK, Cueto FJ, Mujal AM, Melero I, Krummel MF, Sancho D. Dendritic cells in cancer immunology and immunotherapy. Nat Rev Immunol. 2020;20(1):7-24. [CrossRef] | [PubMed]
- Ostrand-Rosenberg S. Myeloid Derived-Suppressor Cells: their role in cancer and obesity. Curr Opin Immunol. 2018;51:68-75. [CrossRef] | [PubMed]
- Chen Y, McAndrews KM, Kalluri R. Clinical and therapeutic relevance of cancer-associated fibroblasts. Nat Rev Clin Oncol. 2021;18(12):792-804. [CrossRef] | [PubMed]
- Ji K, Heyza J, Cavallo-Medved D, Sloane BF. Pathomimetic cancer avatars for live-cell imaging of protease activity. Biochimie. 2016;122:68-76. [CrossRef] | [PubMed]
- Casey SC, Vaccari M, Al-Mulla F, Al-Temaimi R, Amedei A, Barcellos-Hoff MH, et al. The effect of environmental chemicals on the tumor microenvironment. Carcinogenesis. 2015;36; Suppl 1:S160-83. [CrossRef] | [PubMed]
- Zhang L, Yu D. Exosomes in cancer development, metastasis and immunity. Biochim Biophys Acta Rev Cancer. 2019;1871(2):455-68. [CrossRef] | [PubMed]
- Arneth B. Tumor microenvironment. Medicina (Kaunas). 2019;56(1):5. [CrossRef] | [PubMed]
- Taube JM, Galon J, Sholl LM, Rodig SJ, Cottrell TR, Giraldo NA, et al. Implications of the tumor immune microenvironment for staging and therapeutics. Mod Pathol. 2018;31(2):214-34. [CrossRef] | [PubMed]
- Jiang X, Wang J, Deng X, Xiong F, Ge J, Xiang B, et al. Role of the tumor microenvironment in PD-L1/PD-1-mediated tumor immune escape. Mol Cancer. 2019;18(1):10. [CrossRef] | [PubMed]
- Wang YA, Li XL, Mo YZ, Fan CM, Tang L, Xiong F, et al. Effects of tumor metabolic microenvironment on regulatory T cells. Mol Cancer. 2018;17(1):168. [CrossRef] | [PubMed]
- Zak KM, Grudnik P, Magiera K, Dömling A, Dubin G, Holak TA. Structural biology of the immune checkpoint receptor PD-1 and its ligands PD-L1/PD-L2. Structure. 2017;25(8):1163-74. [CrossRef] | [PubMed]
- Han Y, Liu D, Li L. PD-1/PD-L1 pathway: current researches in cancer. Am J Cancer Res. 2020;10(3):727-42. [PubMed]
- Philips EA, Garcia-España A, Tocheva AS, Ahearn IM, Adam KR, Pan R, et al. The structural features that distinguish PD-L2 from PD-L1 emerged in placental mammals. J Biol Chem. 2020;295(14):4372-80. [CrossRef] | [PubMed]
- Yearley JH, Gibson C, Yu N, Moon C, Murphy E, Juco J, et al. PD-L2 Expression in Human Tumors: Relevance to Anti-PD-1 Therapy in Cancer. Clin Cancer Res. 2017;23(12):3158-67. [CrossRef] | [PubMed]
- Tanegashima T, Togashi Y, Azuma K, Kawahara A, Ideguchi K, Sugiyama D, et al. Immune suppression by PD-L2 against spontaneous and treatment-related antitumor immunity. Clin Cancer Res. 2019;25(15):4808-19. [CrossRef] CCR-18-3991: 1158/1078-0432. [PubMed]
- Rekik R, Belhadj Hmida N, Ben Hmid A, Zamali I, Kammoun N, Ben Ahmed M. PD-1 induction through TCR activation is partially regulated by endogenous TGF-β. Cell Mol Immunol. 2015;12(5):648-9. [CrossRef] | [PubMed]
- Chan LC, Li CW, Xia W, Hsu JM, Lee HH, Cha JH, et al. IL-6/JAK1 pathway drives PD-L1 Y112 phosphorylation to promote cancer immune evasion. J Clin Invest. 2019;129(8):3324-38. [CrossRef] | [PubMed]
- Yee D, Shah KM, Coles MC, Sharp TV, Lagos D. MicroRNA-155 induction via TNF-alpha and IFN-gamma suppresses expression of programmed death ligand-1 (PD-L1) in human primary cells. J Biol Chem. 2017;292(50):20683-93. [CrossRef] | [PubMed]
- Morrissey SM, Yan J, Exosomal PD. Exosomal PD-L1: Roles in Tumor Progression and Immunotherapy. Trends Cancer. 2020;6(7):550-8. [CrossRef] | [PubMed]
- Hegde M, Mukherjee M, Grada Z, Pignata A, Landi D, Navai SA, et al. Tandem CAR T cells targeting HER2 and IL13Ralpha2 mitigate tumor antigen escape. J Clin Invest. 2016;126(8):3036-52, [CrossRef] | [PubMed]
- Sun Y, Tan J, Miao Y, Zhang Q. The role of PD-L1 in the immune dysfunction that mediates hypoxiainduced multiple organ injury. Cell Commun Signal. 2021;19(1):76. [CrossRef] | [PubMed]
- Nicole S, Nicholas, Apollonio B, Ramsay AG. Tumor microenvironment (TME)-driven immune suppression in B cell malignancy. Biochim Biophys Acta Mol Cell Res. 2015;1863(3):471-82. [CrossRef] | [PubMed]
- Yuan Y, Jiang YC, Sun CK, Chen QM. Role of the tumor microenvironment in tumor progression and the clinical applications (Review) [review]. Oncol Rep. 2016;35(5):2499-515. [CrossRef] | [PubMed]
- Mishra RK, Wei C, Hresko RC, Bajpai R, Heitmeier M, Matulis SM, et al. In silico modeling-based identification of glucose transporter 4 (GLUT4)selective inhibitors for cancer therapy. J Biol Chem. 2015;290(23):14441-53. [CrossRef] | [PubMed]
- Korman AJ, Garrett-Thomson SC, Lonberg N. The foundations of immune checkpoint blockade and the ipilimumab approval decennial. Nat Rev Drug Discov. 2021;21(7):509-28. [CrossRef] | [PubMed]
- Ngiow SF, Young A. Re-education of the tumor microenvironment with targeted therapies and immunotherapies. Front Immunol. 2020;11:1633. [CrossRef] | [PubMed]
- Tang H, Wang Y, Chlewicki LK, Zhang Y, Guo J, Liang W, et al. Facilitating T cell infiltration in tumor microenvironment overcomes resistance to PD-L1 blockade. Cancer Cell. 2016;29(3):285-96. [CrossRef] | [PubMed]
- Schulz M, Salamero-Boix A, Niesel K, Alekseeva T, Sevenich L. Microenvironmental regulation of tumor progression and therapeutic response in brain metastasis. Front Immunol. 2019;10:1713. [CrossRef] | [PubMed]
- Stadler M, Walter S, Walzl A, Kramer N, Unger C, Scherzer M, et al. Increased complexity in carcinomas: analyzing and modeling the interaction of human cancer cells with their microenvironment. Semin Cancer Biol. 2015;35:107-24. [CrossRef] | [PubMed]
- Tan PH, Chia SS, Toh SL, Goh JC, Nathan SS. Three-dimensional spatial configuration of tumour cells confers resistance to chemotherapy independent of drug delivery. J Tissue Eng Regen Med. 2016;10(8):637-46. [CrossRef] | [PubMed]
- Molina ER, Chim LK, Salazar MC, Mehta SM, Menegaz BA, Lamhamedi-Cherradi SE, et al. Mechanically tunable coaxial electrospun models of YAP/TAZ mechanoresponse and IGF-1R activation in osteosarcoma. Acta Biomater. 2019;100:38-51. [CrossRef] | [PubMed]
- Fischer GM, Vashisht Gopal YN, McQuade JL, Peng W, DeBerardinis RJ, Davies MA. Metabolic strategies of melanoma cells: mechanisms, interactions with the tumor microenvironment and therapeutic implications. Pigment Cell Melanoma Res. 2018;31(1):11-30. [CrossRef] | [PubMed]
- Lyssiotis CA, Kimmelman AC. Metabolic interactions in the tumor microenvironment. Trends Cell Biol. 2017;27(11):863-75. [CrossRef] | [PubMed]
- Mishra D, Banerjee D. Metabolic interactions between tumor and stromal cells in the tumor microenvironment. Adv Exp Med Biol. 2021; 1350:101-21. [CrossRef] | [PubMed]
- Strutz J, Martin J, Greene J, Broadbelt L, Tyo K. Metabolic kinetic modeling provides insight into complex biological questions, but hurdles remain. Curr Opin Biotechnol. 2019;59:24-30. [CrossRef] | [PubMed]
- Wang Z, Wang C, Chen G. Kinetic modeling: a tool for temperature shift and feeding optimization in cell culture process development. Protein Expr Purif. 2022;198:106130. [CrossRef] | [PubMed]