ABSTRACT
Nanocochleates, lipid-based nanostructures inspired by the cochlea’s spiral structure, have developed as talented candidates for advanced drug delivery systems and therapeutic applications. Their special design, which consists of a spiral-shaped lipid bilayer, has several advantages, like excellent stability, biocompatibility and ability to encapsulate a variety of medications, ranging from small molecules to nucleic acids. An overview of nanocochleates is given in this abstract, emphasizing its adaptable characteristics and wide range of biomedical applications. Nanocochleates exhibit exceptional drug encapsulation efficiency, protecting the payload from degradation and enabling controlled release kinetics. This feature makes them suitable for delivering therapeutics with poor aqueous solubility, instability, or low bioavailability. Additionally, targeting ligands can be used to functionalize nanocochleates, allowing for site-specific drug delivery and reducing off-target effects. The efficacy and safety of some medications, including as antibiotics, gene-based therapies and chemotherapeutics, might be greatly enhanced by this specific approach. Nanocochleates have demonstrated potential in further biological uses beyond drug delivery. They can serve as platforms for vaccine delivery, enhancing the stability and immunogenicity of antigens while promoting robust immune responses. Furthermore, nanocochleates offer opportunities in diagnostics, regenerative medicine and cosmeceuticals, where their stability, biocompatibility and tunable properties can be leveraged for imaging, tissue engineering and skincare formulations. Overall, nanocochleates represent a versatile and promising class of nanostructures with broad applications in drug delivery and therapeutics. Continued study and progressive efforts are required to enhance their formulation, understand their biological interactions and translate them into clinical practice. With additional investigations, nanocochleates might completely change how drugs are delivered to patients and help create novel treatment plans for a range of illnesses.
INTRODUCTION
Drugs are contained in a multilayered lipid crystal matrix by the nanocochleate drug delivery vehicle, which makes distribution safe and efficient. Cochleates are significant particles consisting of spirally twisted, enormous, continuous lipid bilayer sheets without an interior aqueous phase between them. Nanocochleates have greater stability compared to liposomes, making them a promising delivery method. It also has higher encapsulation efficiency due to its rod form. The bilayered structure allows for more regulated medication storage. Aside from several benefits, they have drawbacks since they require specialized storage conditions.1
Stable phospholipid-cation precipitates made mostly of calcium and phosphatidylserine are the building blocks of nanocochleate delivery vehicles. The overall development of the nanocochleate is made up of solid layers and components that are sealed within its core and stay intact, despite the possibility of the exterior layers being exposed to harsh environments or enzymes. Nanocholeates can be utilized to encapsulate both hydrophobic and hydrophilic drugs because of their surfaces’ dual hydrophobic and hydrophilic properties.2 Encochleation nanotechnology has the potential to improve various aspects of formulated products, such as ease of production, formulation quality, sustained drug release, processing and shelf-life stability and biodegradability for systemic administration. Encapsulating physiologically significant molecules, nanocochleate shields them from environmental and gastrointestinal enzyme-induced breakdown.3 Cochleates hold onto their bilayer structures of phospholipids. Because of their extreme flexibility, these solid particles may easily remove the bridging counter ions from the interbilayer gaps and transform into liposomes. Because of their special qualities, cochleates are the ideal vehicle for delivering insoluble materials that can be incorporated into phospholipid bilayers without causing liposome instability.4
Structure of Nanocochleates
Negatively charged phospholipid bilayers called cochleate and nanocochleate interact with multivalent counter ions like Zn2+ or Ca2+ to generate bridging agents that allow the bilayers to form spiral rolls that resemble cigars. Because of their solid matrix, cochleates, as a particulate structure, have special qualities above liposomes, such as greater mechanical stability and better protection for pharmaceuticals possessed.5 The vast multilayer sheets with hydrophobic surfaces prefer to roll up into a cigar-like form in order to reduce the water interactions. Dehydration of the phosphatidyl head group is the molecular mechanism and it is essential for bilayers to approach closely and for the development of cochleate cylinders to start (Figure 1). The lipid bilayer is oriented at a repeating distance close to 54 Å.6
Mechanism of nanocochleates
The drug is believed to be released by the nanocochleates when their lipid bilayer unites with the cell membrane and the required pharmacological activity may be attained.4 An further conjecture highlights the chances of phagocytosis using phosphatidylserine receptors. These are typical for the exterior cochleate film and the lipid layer of macrophages. The collected medication enters the cytoplasm of macrophages when cochleate approaches their lipid film and connects with them.7
Methods for Preparation
The following techniques are often used to prepare nanocochleates.8
Hydrogel technique, Trapping technique, Liposome before cochleates dialysis technique. Direct calcium dialysis technique and Binary aqueous- aqueous emulsion technique.
Hydrogel method
Small unilamellar drug-loaded liposomes are generated using the hydrogel approach and introduced to polymer-A. Next, polymer-B receives the addition of two dispersions. The two polymers are immiscible with one another and as a result, an aqueous two-phase system is formed. A cation salt solution diffuses into the second polymer and then into the particles that contain the polymer in a two-phase system. As a result, cationic cross-linkage and small-sized cochleate are formed in the polymer (Figure 2). The polymer in the created cochleates is removed by washing; the polymer may then be resuspended in physiological buffer.5
Trapping method
Trapping method involves several key steps, a phospholipid solution is prepared and the drug of interest is dissolved in a compatible solvent. The lipid solution is then mixed with an aqueous buffer and subjected to mechanical agitation, promoting the formation of lipid bilayers that transition into cochleate structures. During this process, the drug becomes encapsulated within the cochleates. After formation, the nanocochleates are purified through centrifugation or dialysis to remove unbound components (Figure 3). Finally, characterization techniques like dynamic light scattering and transmission electron microscopy are used to assess size, morphology and drug loading efficiency, ensuring the nanocochleates are suitable for drug delivery applications.9
Liposome before cochleates dialysis method:
Lipid and detergent are added to the liposomes during their initial production using this process. It’s a two-step process wherein liposomes are created by first removing the detergent using dialysis. After that, the generated liposomes are used for dialysis against a Calcium Chloride (CaCl2) solution, which causes nanocochleates to develop. Because the generated intermediate liposomes are tiny, the created nanocochleates are small as well. This method is typically applied to hydrophobic materials, including membrane proteins. Using this technique, 50-100 nm-sized nanocochleates may be produced (Figure 4).10
Direct calcium dialysis method
The cochleates will be the same size and this approach does not include the formation of transitional liposomes, unlike the liposome used in the prior cochleate dialysis method. Calcium chloride configuration is immediately dialyzed against the lipid and more efficient combination. The tension between the formation of calcium and the cleanser’s release from the cleanser/lipid/drug micelles produces a thin, highly layered structure in this manner. Phosphatidylserine, cholesterol and a preset concentration of polynucleotide are mixed in a non-ionic cleanser and extraction cushion and the mixture is vortexed for 5 min. After that, the produced clear solution is dialysed at room temperature against three different buffer modifications. While 3 mM Ca2+ is enough, the last dialysis is carried out in a solution containing 6 mM Ca2+. DC cochleate is the resulting white calcium phospholipid (Figure 5).11
Binary aqueous-aqueous emulsion system
Using a film technique or a high pH, tiny liposomes are encapsulated in this manner and then mixed with a polymer, such dextran. A second, non-miscible polymer (like Stake) is then injected with the dextran/liposome stage. After that, the calcium was applied and spread progressively, first to one stage and then to the subsequent framing nanocochleates. The cochleates framed with this method have molecules smaller than 1000 nm.12
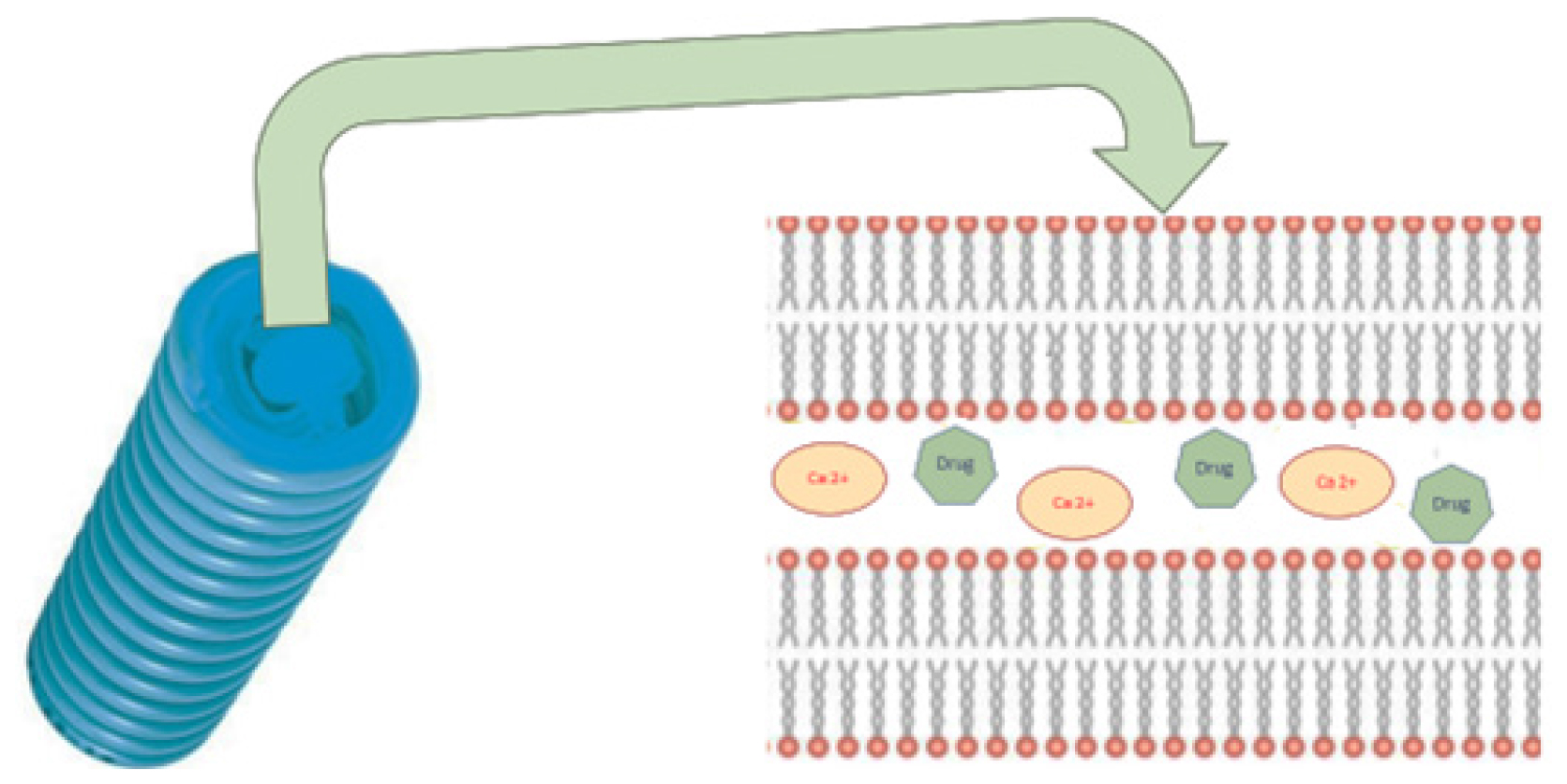
Figure 1:
Schematic representation of Nanocochleates.
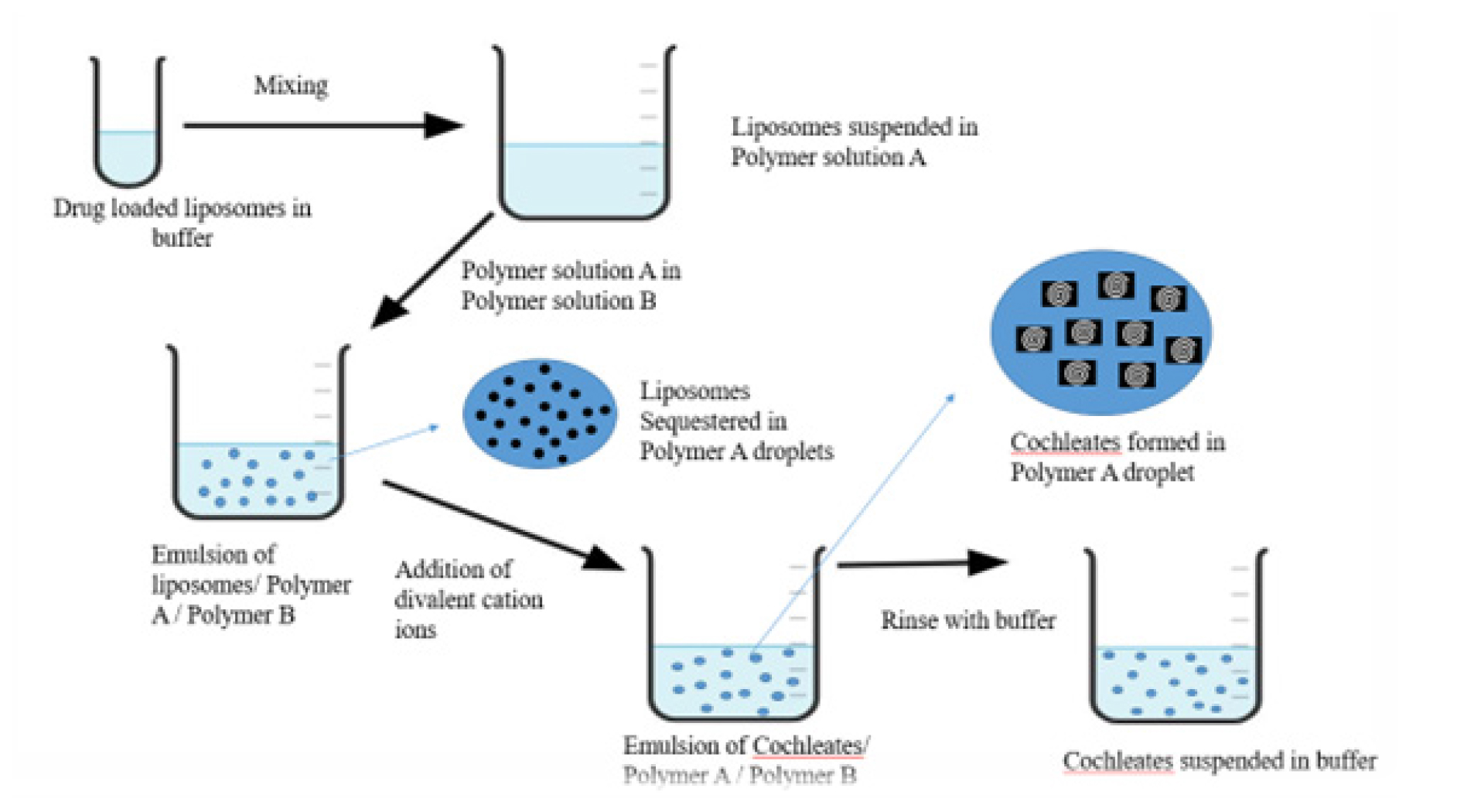
Figure 2:
Hydrogel method for the preparation of Nanocochleates.
Stability of Nanocochleates
As phospholipids used to generate nanocochleates which results from a natural source, they are less resistant to oxidation. When the cationic salt reacts with the phospholipid, a tight connection is formed, resulting in the creation of stable nanocholeates. The medication is included within the solid phospholipid bilayer series. Following their synthesis, nanocochleates are lyophilized, which increases their stability over time and allows them to be reconstituted before usage by adding the appropriate vehicle. This implies that this new technology is superior to existing nanocarrier systems.13
Characterization Studies of Nanocochleates
Particle size determination
The liposomal and cochleate dispersions’ mean particle sizes were calculated using the Malvern 2000SM (Malvern, UK) laser diffraction technique. For the analysis, a 30±2°C temperature and a 90° angle of detection were employed. The volume mean diameter, the average diameter of a sphere whose volume is equal to the particle under measurement-was utilized to express the mean vesicle size.14
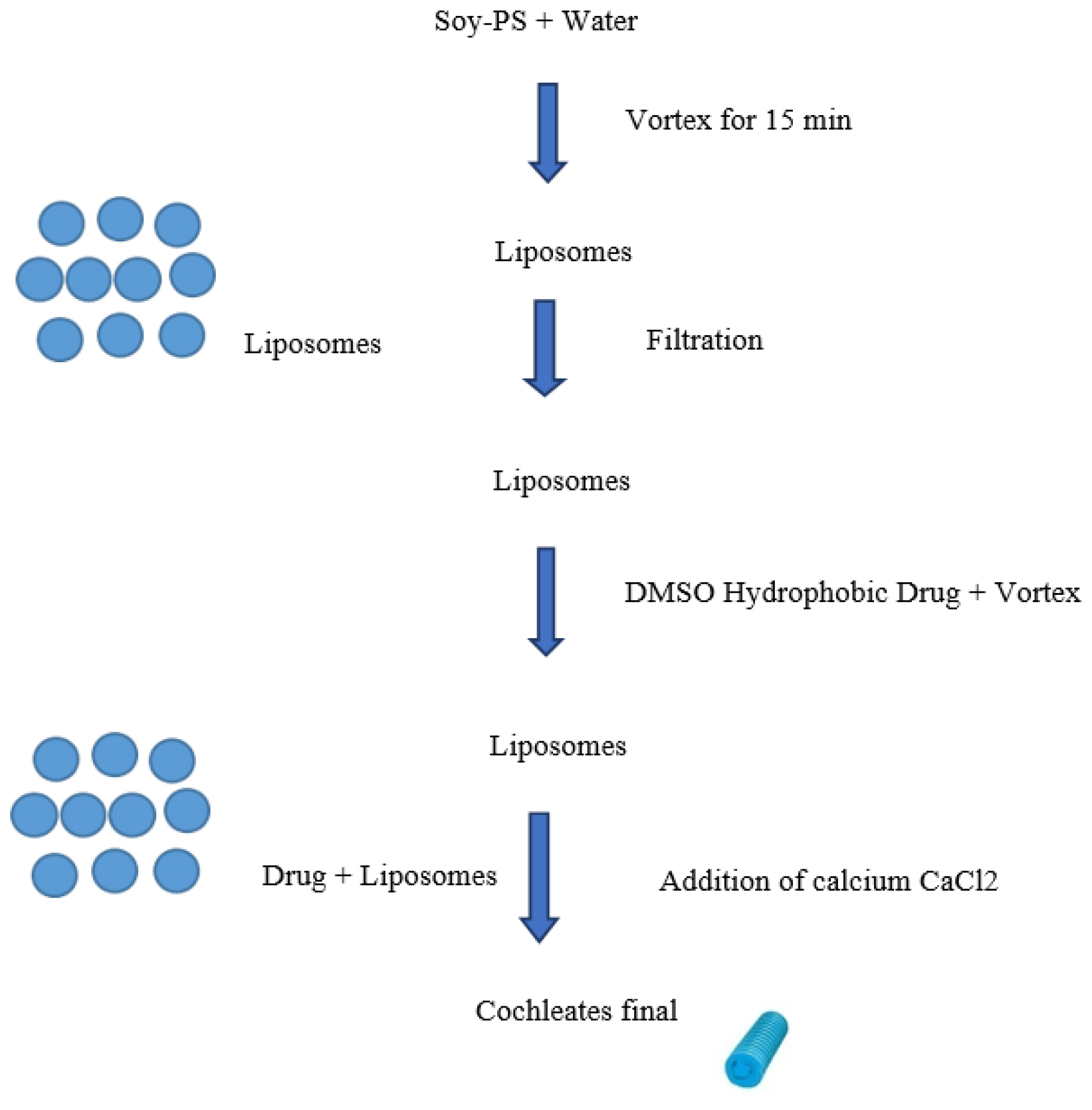
Figure 3:
Trapping method for the preparation of Nanocochleates
Specific surface area
A sorptometer is commonly used to measure the specific surface area of a freeze-dried Nanocochleate product. The following formula may be used to calculate the specific surface area,
Where,
d is the cochleate’s diameter, ρ is its density and A is its specific surface area.
While the determined and computed specific surface areas generally agree, sometimes residual surfactant might lead to discrepancies in the observed results.6
Density
Using a gas pycnometer and either air or helium, one may find the density of nanocochleates. The result obtained with air and helium is particularly clear because of the structure’s porosity and specific surface area.15
Drug content
The unbound medication is separated from the supernatant by centrifuging the redispersed nanocochleates suspension for 40 min at 25° and 15,000 rpm. After an appropriate dilution, the drug dosage in the supernatant may then be measured using UV-vis spectrophotometry.16
Entrapment efficiency
Separate the cochleates (100 μL) into tubes that have been centrifuged.17 One milliliter (mL) of ethanol and sixty microliters of pH 9.5 EDTA were mixed during the vortexing process. An appropriate analytical method is used to quantify the absorbance of the solution and the following formula is used to compute the entrapment efficiency.
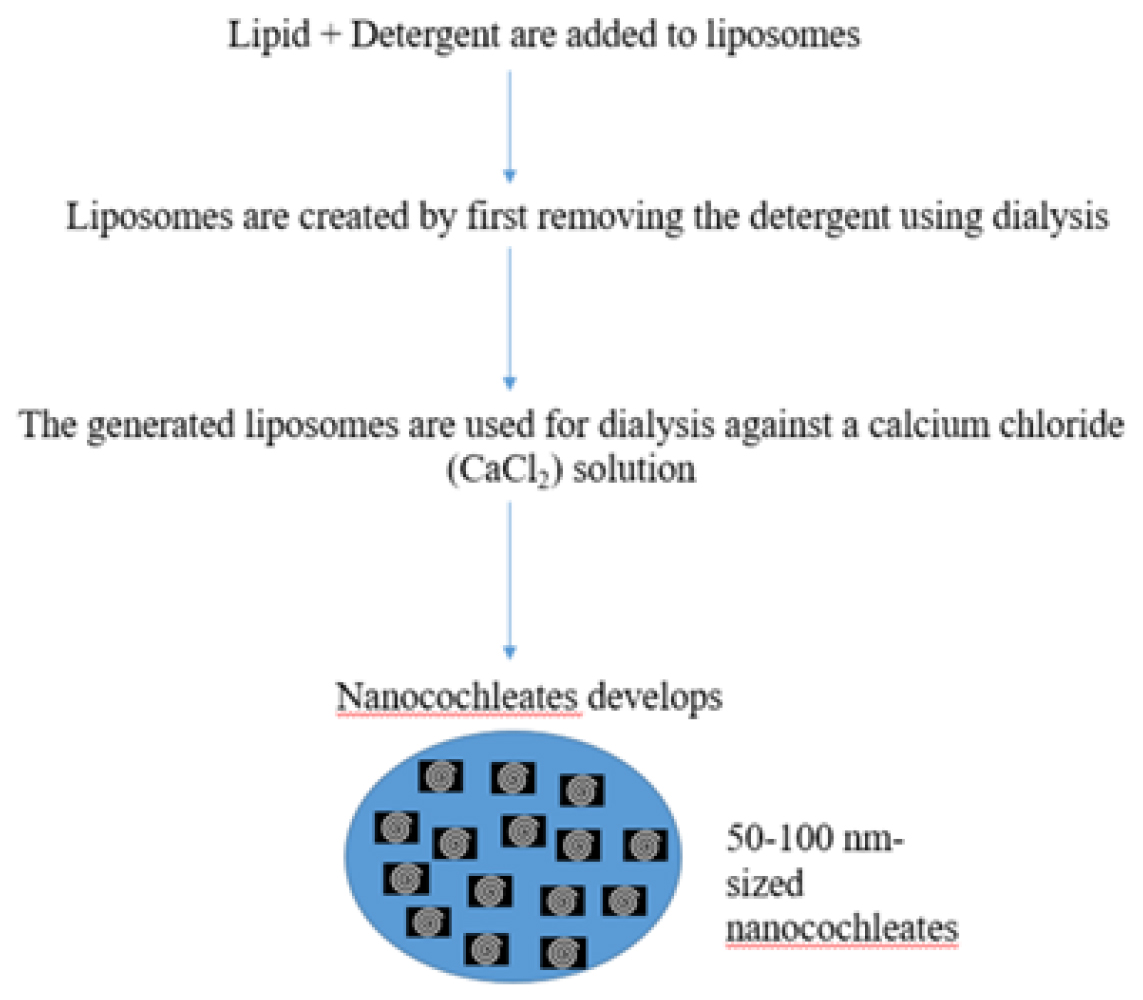
Figure 4:
Liposome before cochleates dialysis method.
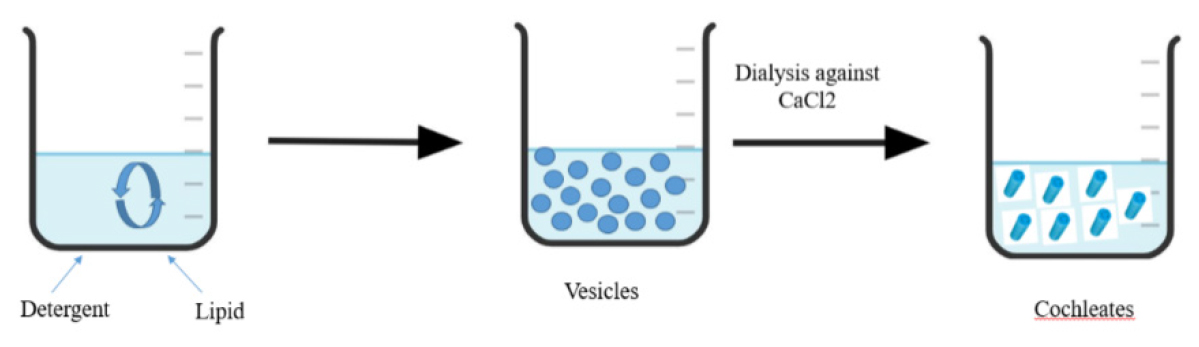
Figure 5:
Direct calcium dialysis method.
In vitro release
The in vitro release profile of nanocochleates can be ascertained using diffusion cells, conventional ones dialysis, or the recently developed modified ultra-filtration methods that employ double chamber diffusion cells on an agitated platform with phosphate buffer. A hydrophilic Millipore membrane with poor protein binding capacity divides the two chambers. Using well-established methods, the receptor compartment is routinely examined for the release of medication after nanocochleates are added to the donor chamber. The in vitro release behavior of the nanocochleates is also ascertained using the modified ultra-filtration approach. Here, the buffer-containing ultra-filtration cell is shaken prior to the direct addition of Nanocochleate. Using an ultra-filtration membrane, aliquots of the dissolving media are filtered at various times with a nitrogen pressure of <2. The medicine is then examined using standard procedures once it has been released.18
Fourier transform infrared spectroscopy study
A study using Fourier Transform Infrared spectroscopy (FTIR) identifies the compound’s purity and functional groups that are present.19 To prepare samples, combine them with KBr. Samples are then put into the holder. The specific wave number ranges are covered by scanning the spectra at room temperature.
Differential scanning calorimetry study
Lipid state is established using an examination with differential scanning calorimetry.20 The samples are heated steadily at 10°C/ min within perforated, hermetically sealed aluminum pans. The temperature range of the samples is -10 to 180°C. Nitrogen gas is fed into the system at a rate of 100 mL/min to keep the atmosphere inert.
Molecular weight measurements
A refractive index detector can be used in Gel Permeation Chromatography (GPC) to determine the molecular weight of the polymer and its dispersion in the matrix. Unlike what was demonstrated using GPC, which is the result of one or a few lengthy polymer chains rolling up, multiple small oligomeric subunits entangle to form PACA nanocochleates.21
Surface morphology study
The surface morphology of nanocochleates is investigated using Transmission Electron Microscopy (TEM).11 For this investigation, the diluted sample is ready by dropping it onto a carbon-coated copper grid, which forms a thin liquid layer. After eliminating any excess solution from the sample, it is examined and captured on camera using a TEM operating at an 80 kV accelerating voltage.
Surface charge and electrophoretic mobility
It is important to consider the form and magnitude of surface charge on a nanocrystal because it influences the molecule’s electrostatic interactions with bioactive chemicals and its interactions with the biological environment. The velocity of colloidal particles in an electric field can be used to measure their surface charge, especially sodium chorochleate. Naphthocyanine velocities may be quickly and accurately calculated using laser light scattering methods like velocimetry or Laser Doppler Analysis (LDA/LDV). Another way to quantify colloidal particle surface charge is by electrophoretic mobility. The biodistribution of medication-carrying nanocochleate is significantly influenced by the charge composition. Human serum and phosphate saline buffer are frequently used to test the electrophoretic mobility of NP. The phosphate saline buffer’s pH of 7.4 drops as a result of an ionic interaction between the buffer’s constituent parts and the charged surface of nanocochleate. The Helmholtz-Smoluchowski equation and an electrophoretic mobility measurement can be used to determine the zeta potential.22
Surface hydrophobicity
The hydrophobicity of nanocochleates’ surface affects how colloidal particles interact with their biological surroundings. The bio-fate of nanocochleates and their component components is influenced by the mix of hydrophobicity and hydrophilicity. The characteristics of blood elements that nanocochleates interact with hydrophobically are regulated by their hydrophobicity. Surface hydrophobicity has been assessed using a variety of techniques, such as hydrophobic interaction chromatography, two-phase partitioning, adsorption of hydrophobic fluorescence or radiolabelled probes and contact angle measuring.23
Cochleates cell interaction study
To investigate the relationship between cochleates and cell membranes, 2% fluorescent lipid is combined with negatively charged lipids to form fluorescent cochleates. The surfaces of the cells light and become visible under fluorescent microscopes when cochleates come into contact with the cell membrane due to a luminous lipid transfer.6
Stability study
As phospholipids used to generate nanocochleates come from a natural source, they are less resistant to oxidation potential. When the phospholipid and cationic salt react, a tight connection is formed, resulting in the creation of stable nanocholeates. The medication is included within the solid phospholipid bilayer series. Following their synthesis, nanocochleates are lyophilized, which increases their stability over time and allows them to be reconstituted by adding the appropriate vehicle before to use. This implies that this new technique is superior to existing nanocarrier technologies. For stability tests, cochleates dispersions can be stored for three months at 2 to 8°C and 25±2°C/60% RH. Changes in cochleate particle size and Entrapment Efficiency (% EE) are used to assess the formulation’s stability.24
Nanocochleate Delivery Vehicles’ Safety and Biocompatibility
The stable lipid-based delivery forms known as nanocochleates differ greatly from liposomes in terms of their shapes and characteristics. One possible method of raising the nanocochleate’s stability throughout time in terms of both chemical and physical aspects. It is anticipated that solid lyophilizates will be more chemically and physically stable than liquid lipidic dispersions.6 To keep the nanoparticulate solution stable, common cryoprotective substances such sorbitol, mannose, trehalose and glucose are mixed. It is possible to lyophilize nanocochleates into a powder and store them at 40°C. Before being administered in vivo or in vitro, lyophilized cochleate can be reconstituted with liquid. The shape or functions of cochleate are not negatively impacted by lyophilisation.1
Applications of Nanocochleates
Drug delivery
One of the primary uses of nanocochleates is in drug delivery.29 Numerous medications, including tiny molecules, peptides, proteins and nucleic acids, can be enclosed in these nanostructures. Nanocochleates protect the encapsulated drug from degradation, enhance its stability and provide controlled release, leading to improved therapeutic outcomes (Table 1).
Class of drugs | Drugs | Application | References |
---|---|---|---|
Antibiotics | Gentamicin Vancomycin Amphotericin B | Used to treat bacterial and fungal infections and cochleates can improve their therapeutic efficacy by providing sustained release and targeted delivery. | 16 |
Anticancer drugs | Paclitaxel Doxorubicin Cisplatin | Chemotherapeutic agents can be encapsulated in cochleates for cancer treatment. Cochleates can improve the delivery of these drugs to cancer cells while reducing systemic toxicity. | 25 |
Anti-inflammatory drugs | Dexamethasone Celecoxib | Anti-inflammatory medications used to treat inflammatory diseases such arthritis, asthma and inflammatory bowel disease have been investigated for administration via cochleates. | 18 |
Antiviral drugs | Ribavirin Zidovudine Famciclovir | Antiviral agents can be encapsulated in cochleates to treat viral infections like hepatitis and HIV/AIDS. Cochleates offer a promising approach to enhance the delivery of antiviral drugs to infected cells. | 26 |
Antifungal drugs | Amphotericin B Fluconazole Itraconazole | Antifungal agents can be encapsulated in cochleates for the treatment of fungal infections like candidiasis and aspergillosis. | 27 |
Vaccines | Antigens Adjuvants | Cochleates have also been investigated for vaccine delivery. They can encapsulate antigens and adjuvants, offering protection against infectious diseases and potentially improving the efficacy of vaccines. | 1 |
Gene therapy agents | siRNA mRNA Plasmid DNA | Cochleates show promise for the delivery of nucleic acid-based therapeutics like siRNA, mRNA and plasmid DNA for gene therapy applications. They can protect nucleic acids from degradation and facilitate their uptake by target cells. | 28 |
Applications of Drugs Used in Cochleates.
Cancer therapy
Antimicrobial therapy
Vaccine delivery
Gene delivery
Diagnostics
Nanocochleates have potential applications in diagnostics, particularly in imaging and biosensing.34 Nanoparticles are useful tools for disease monitoring and diagnostic imaging because they may be functionalized with imaging agents or targeting ligands to enable viewing of certain tissues or biomolecules.
Nutraceuticals and cosmeceuticals
Nanocochleates can encapsulate bioactive compounds such as vitamins, antioxidants and phytochemicals for use in nutraceuticals and cosmeceuticals.35 They improve the stability and bioavailability of these compounds, allowing for enhanced efficacy in dietary supplements and skincare products.
Regenerative medicine
Growth factors, cytokines and other bioactive compounds can be delivered using nanocochleates to aid in tissue regeneration and wound healing in regenerative medicine.36 They can be tailored to specific tissue types and used in therapies for tissue engineering and regenerative therapies.
CONCLUSION
Nanocochleates are innovative lipid-based vesicles that enhance drug delivery and therapeutics. They offer advantages like improved stability, biocompatibility and targeted delivery, capable of encapsulating a wide range of medications from small compounds to nucleic acids and proteins. By protecting drugs from degradation and facilitating transport to specific tissues, nanocochleates address challenges of conventional drug delivery, such as poor solubility and bioavailability. Their customizable properties like size, surface charge and release kinetic allow for tailored systems that improve efficacy and patient compliance. Continued research is crucial to fully realize their potential in transforming treatments and enhancing patient outcomes.
Cite this article:
Suresh K, Srinivasan D, Palanivel V, Balu A. Nanocochleates: Versatile Nanostructures for Enhanced Drug Delivery and Therapeutics. Int. J. Pharm. Investigation. 2025;15(1):49-56.
ABBREVIATIONS
FTIR | Fourier transform infrared |
---|---|
GPC | Gel Permeation chromatography |
TEM | Transmission electron microscopy |
CaCl2 | Calcium chloride |
mL | Millilitre |
LDV | Laser Doppler Velocimeter |
LDA | Laser Doppler Anemometer |
References
- Tilawat M, Bonde S. Nanocochleates: A potential drug delivery system. J Mol Liq [Internet]. 2021;334:116115 [CrossRef] | [Google Scholar]
- Ghodake PP, Rajendra R, Professor BA, Mane AN, Ghadge AA, Rajendra Bhosale R, et al. Nanocochleates: A novel carrier for drug transfer. J Sci Innov Res [Internet]. ;2(5):Array-9. Available from: http://www.jsirjournal.com
[CrossRef] | [Google Scholar] - Ravi Sankar V, Dastagiri Reddy Y. Nanocochleate-a new approch in lipid drug delivery. Int J Pharm Pharm Sci. 2010;2(4):220-3. [CrossRef] | [Google Scholar]
- Nanocochleate KMR. A novel. Drug Deliv. 2016;2016(3):234-42. [CrossRef] | [Google Scholar]
- Mahendra RP. a Review on Nanocochleate. World J Pharm life sci WJPLS. 2022;8(9):43-9. [CrossRef] | [Google Scholar]
- Yadav V, Parab B, Shidhaye S. Nanocochleate: a novel approach for delivery of biological molecules. Int J Pharm Sci Res [Internet]. 2021;12(7):3581 [CrossRef] | [Google Scholar]
- Yeole SE, Pimple SS, Chaudhari PD. A review on nanocochleate-A novel lipid based Drug Delivery System. J Biomed Pharm Res. 2013;2(1):1-7. 01-7 [CrossRef] | [Google Scholar]
- Yadav A, Chaudhary S. Formulation development and characterization of nanocochleates for the improvement of permeability of drug. J Adv Pharm Educ Res. 2016;6(3):14-21. [CrossRef] | [Google Scholar]
- Nagarsekar K, Zma J. Recent advances and developments in cochleate technology. J Nanomed Nanotechnol. 2016:1-5. [CrossRef] | [Google Scholar]
- Bothiraja C, Yojana BD, Pawar AP, Shaikh KS, Thorat UH. Fisetin-loaded nanocochleates: formulation, characterisation, anticancer testing, bioavailability and biodistribution study. Expert Opin Drug Deliv. 2014;11(1):17-29. [CrossRef] | [Google Scholar]
- Tilawat M, Bonde S. Curcumin and quercetin loaded nanocochleates gel formulation for localized application in breast cancer therapy. Heliyon [Internet]. 2023;9(12):e22892 [CrossRef] | [Google Scholar]
- Unecha M, Sabale A, Pharate M. Nanocochleates: a novel carrier for. 2023;8(1):215-9. [CrossRef] | [Google Scholar]
- Shende P, Khair R, Gaud RS. Nanostructured cochleates: a multi-layered platform for cellular transportation of therapeutics. Drug Dev Ind Pharm [Internet]. 2019;45(6):869-81. [CrossRef] | [Google Scholar]
- Liu M, Zhong X, Yang Z. Chitosan functionalized nanocochleates for enhanced oral absorption of cyclosporine A. Sci Rep [Internet]. 2017;7(1):1-10. [CrossRef] | [Google Scholar]
- Print I, Online I, Venkatesan K, Krishnaraju K, Vs S, Pichaivel M, et al. World J Pharm Sci Rats. 2021;9(8):69-72. [CrossRef] | [Google Scholar]
- Tipugade OB. Review article insights of nanocochleates in conventional drug delivery system current. Pharm Res. 2024 [CrossRef] | [Google Scholar]
- Munot N, Kandekar U, Giram PS, Khot K, Patil A, Cavalu S, et al. A comparative study of quercetin-loaded nanocochleates and liposomes: formulation, characterization, assessment of degradation and anticancer potential. Pharmaceutics. 2022;14(8) [CrossRef] | [Google Scholar]
- Çoban Ö, Değim Z. Development of nanocochleates containing erlotinib HCl and dexketoprofen trometamol and evaluation of characteristic properties. Turk J Pharm Sci. 2018;15(1):16-21. [CrossRef] | [Google Scholar]
- Bothiraja C, Rajput N, Poudel I, Rajalakshmi S, Panda B, Pawar A, et al. Development of novel biofunctionalized chitosan decorated nanocochleates as a cancer targeted drug delivery platform. Artif Cells Nanomed Biotechnol [Internet]. 2018;46(sup1):447-61. [CrossRef] | [Google Scholar]
- Rub R, Munot N, Wadate A. Improved efficacy and stability of silymarin loaded nanocochleates over liposomes for the treatment of skin diseases. J Pharm Res Int. 2021;33:163-76. [CrossRef] | [Google Scholar]
- Bothara S, Mahaparale PR, Musale VL. Nanocochleate-an important drug delivery system offering unique features 1 *Sangita Fulchand Pawar, 2. Sunil. 2020;9(9):621-37. [CrossRef] | [Google Scholar]
- Khairnar SB, Saudagar RB. Nanococleates: an overview. 2017;3(1):17-24. [CrossRef] | [Google Scholar]
- Rajendra R, Professor BA, Ghodake PP, Mane AN, Ghadge AA, Rajendra Bhosale R, et al. Nanocochleates: A novel carrier for drug transfer. J Sci Innov Res [Internet]. 2013;2(5):964-9. Available from: http://www.jsirjournal.com
[CrossRef] | [Google Scholar] - Ramasamy T, Khandasamy U, Hinabindhu R, Kona K. Nanocochleate – A new drug delivery system. Fabad J Pharm Sci. 2009;34(2):91-101. [CrossRef] | [Google Scholar]
- Nayek S, Venkatachalam A, Choudhury S. Recent nanocochleate drug delivery system for cancer treatment: a review. Int J Curr Pharm Res. 2019;11(6):28-32. [CrossRef] | [Google Scholar]
- Sharma R, Deshpande A, Kanugo A. Formulation optimization and evaluation of nanocochleate gel of famciclovir for the treatment of herpes zoster. Recent Pat Nanotechnol. 2022:16 [CrossRef] | [Google Scholar]
- Ji C, Qiu Z, Yang Z, Luo P. Current development of a lipid-based nanocochleates containing amphotericin B for oral administration. J Drug Deliv Sci Technol [Internet]. 2024;92:105347 [CrossRef] | [Google Scholar]
- Vakhare A, Wankhade V, Atram S, Bobade N, Pande S. Student. Adv NANOCOCHLEATE Drug Deliv Syst A Compr Rev. 2024;12(1):2320-882. [CrossRef] | [Google Scholar]
- Fulchand Pawar S, Bothara S, Mahaparale P, Musale V. [cited Jun 7 2024];Nanocochleate -AN important drug delivery system offering unique features. Available from: http s://wjpr.s3.ap-south-1.amazonaws.com/article_issue/1597714153.pdf
[CrossRef] | [Google Scholar] - Poudel I, Ahiwale R, Pawar A, Mahadik K, Bothiraja C. Development of novel biotinylated chitosan-decorated docetaxel-loaded nanocochleates for breast cancer targeting. Artif Cells Nanomed Biotechnol. 2018;46(sup2):229-40. [CrossRef] | [Google Scholar]
- Verekar R, Dessai S, Ayyanar M, Nadaf S, Gurav S. Nanocochleates: revolutionizing lipid-based drug delivery with enhanced bioavailability, a review. Hybrid Adv. 2024;6:100215 [CrossRef] | [Google Scholar]
- Bhosale RR, Gangadharappa HV, Gowda DV, Osmani RA, Vaghela R. A review on nanocochleates: the inimitable nanoparticulate drug carriers. Adv Sci Eng Med. 2017;9(5):359-69. [CrossRef] | [Google Scholar]
- Loomba L, Scarabelli T. Metallic nanoparticles and their medicinal potential. Part II: aluminosilicates, nanobiomagnets, quantum dots and cochleates. Ther Deliv. 2013;4(9):1179-96. [CrossRef] | [Google Scholar]
- Ray S, Nayak AK. Design and applications of theranostic nanomedicines. 2022 [CrossRef] | [Google Scholar]
- Srivastava N, Srivastav AK, Shanker K. InNanotechnology and nanomaterials in the agric-food industries. 2024:283-314. [CrossRef] | [Google Scholar]
- Eskandarynasab M, Etemad-Moghadam S, Alaeddini M, Doustimotlagh AH, Nazeri A, Dehpour AR, et al. Novel osteoprotective nanocochleate formulation: a dual combination therapy-codelivery system against glucocorticoid induced osteoporosis. Nanomed Nanotechnol Biol Med. 2020;29:102273 [CrossRef] | [Google Scholar]