ABSTRACT
Pharmaceutical formulations, polymer-based nanofibers, which have higher surface area per unit mass of solid, enable and contribute to functions linked to surface shape. Nanofibers can be made using a number of different methods, but only electrospinning technology has been specifically focused on producing a wide variety of these common polymer-based nanofibers. The manufacturing potential of just this method is enormous. Electrospinning is also the most versatile, as it can produce a wide range of nanowire assemblies that, with the right tweaks, might improve the performance of goods made from Nanofibers. For these reasons, it is crucial and necessary to study the many factors and procedures that go into making the best possible nanofibers. Standard processes and tools may be used to examine nanofibers’ structure, morphology, and geometry, as well as their tensile and elongation characteristics. In this article, we take a look at the various ways in which polymer composite nanofibers can be used in tissue engineering, such as in tissue scaffolds and cutting-edge wound dressings for chronic wound therapy, in addition to their potential roles in drug delivery systems, in clothing protectors, and sensors. There are just a select few of these products that have been released so far, but more nano- and bio-sciences products are expected to hit the market shortly. Polymer-based nanofibers have emerged as a result of efforts to commercialize these applications. A variety of fields, including biology, nutrition, bioengineering, pharmaceuticals, and healthcare, find this structured fiber useful.
INTRODUCTION
Materials for nanofibers are those with a diameter smaller than 100 nanometers. A wide range of polymers, including gelatin, chitosan, collagen, CMC-carboxymethylcellulose, and PVA-polyvinyl alcohol from electrospinning, be acceptable materials for the development and production of nanofibers. Due to their higher surface area, reduced porosity, and identical unique properties, nanofibers can offer considerable advantages in wound care management.1
To aid in the recovery and healing of wounds, polymeric matrix structures, including nanofibers embedded with drugs or growth factors, demonstrate the advantages of nanofibers. These nanowires, particularly in biomedical applications, conduct unique mechanisms such as the absorption of exudates or the ability to provide anti-adhesive action (addition of drugs containing polymer-based nanofibers). Many targeted drug delivery systems also use nanofibers to control the release profile of the medication packaged in a polymer at a specific spot.2
Fabrication of nanofibers by electrospinning
Electrospinning is the most adaptable and cost-effective method for producing fine strands or filaments of the congaing drug-polymer solution when exposed to a high-voltage electric field. Compared to the typical diameter of human hair, these tiniest fibers are a thousand times smaller in diameter. The high voltage on the metal syringe provides a usual electric charge on the solution’s surface, which causes nanofibers to develop. Using a needle that shoots out a tiny stream of polymer solution, this charge is attracted to an electrically grounded collector enclosed by a piece of aluminium foil. As a syringe is charged with high voltage, nanofibers are formed on the solution’s surface, and this charge attracts an electrically grounded collector enclosed by aluminium foil. Because of voltage differences, the solvent tries to escape as it comes from the needle of a syringe.
The electrospinning technique has more advantages than disadvantages, including the ability to produce nanofibers at a fast rate, ease of setup, and the most cost-effectiveness. It also helps to maintain the desired diameter of nanofibers while using the electrospinning method.3
Influence of Parameters on Surface Morphology of electro spun Nanofibers
The following variables may influence the morphology of electro spun fibers: (i) processing variables (e.g., flow rate,4 electric potentials,5,6 capillary tip-to-collector distance,7 and collector set-up).8 (ii) system characterizations (e.g., the molecular weight of polymer,9 conductivity,10 surface tension of polymer solution,11 and viscosity).12,13 (iii) The applied electric field strength influenced the form and diameter of electro spun fibers.14 Increasing the applied voltage always increases fibre diameter, while increasing the field strength causes bead flaws in electro-spun fibers. Fibers that collapse into beads or cannot be extruded due to considerable polymer entanglement are not extremely dilute or highly concentrated.15
Reneker D. H. et al. proposed a critical role in achieving desired nanofiber sizes is played by jet size, which is a crucial step in evaporating the solution to produce nanofibers that the jet might be split into several jets, which leads to the creation of varied diameters of nanofibers (Figure 1).16
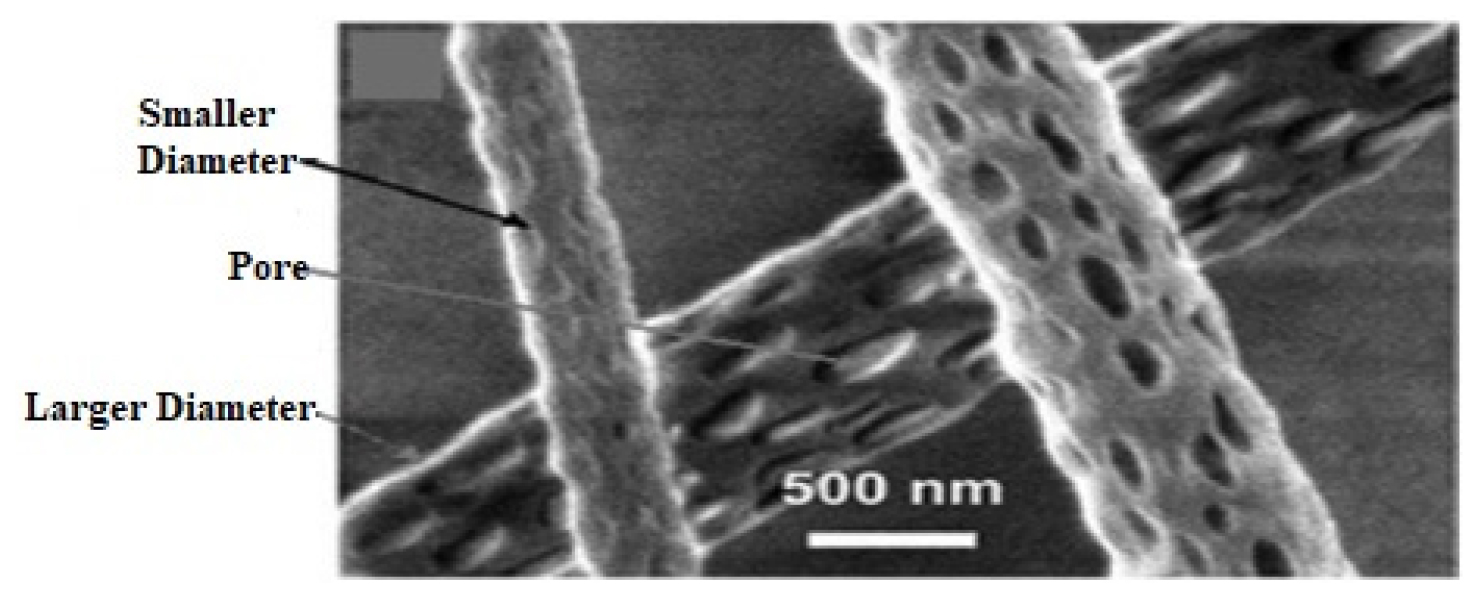
Figure 1:
Poly-lactic acid nanofibers with different diameters and pores.16
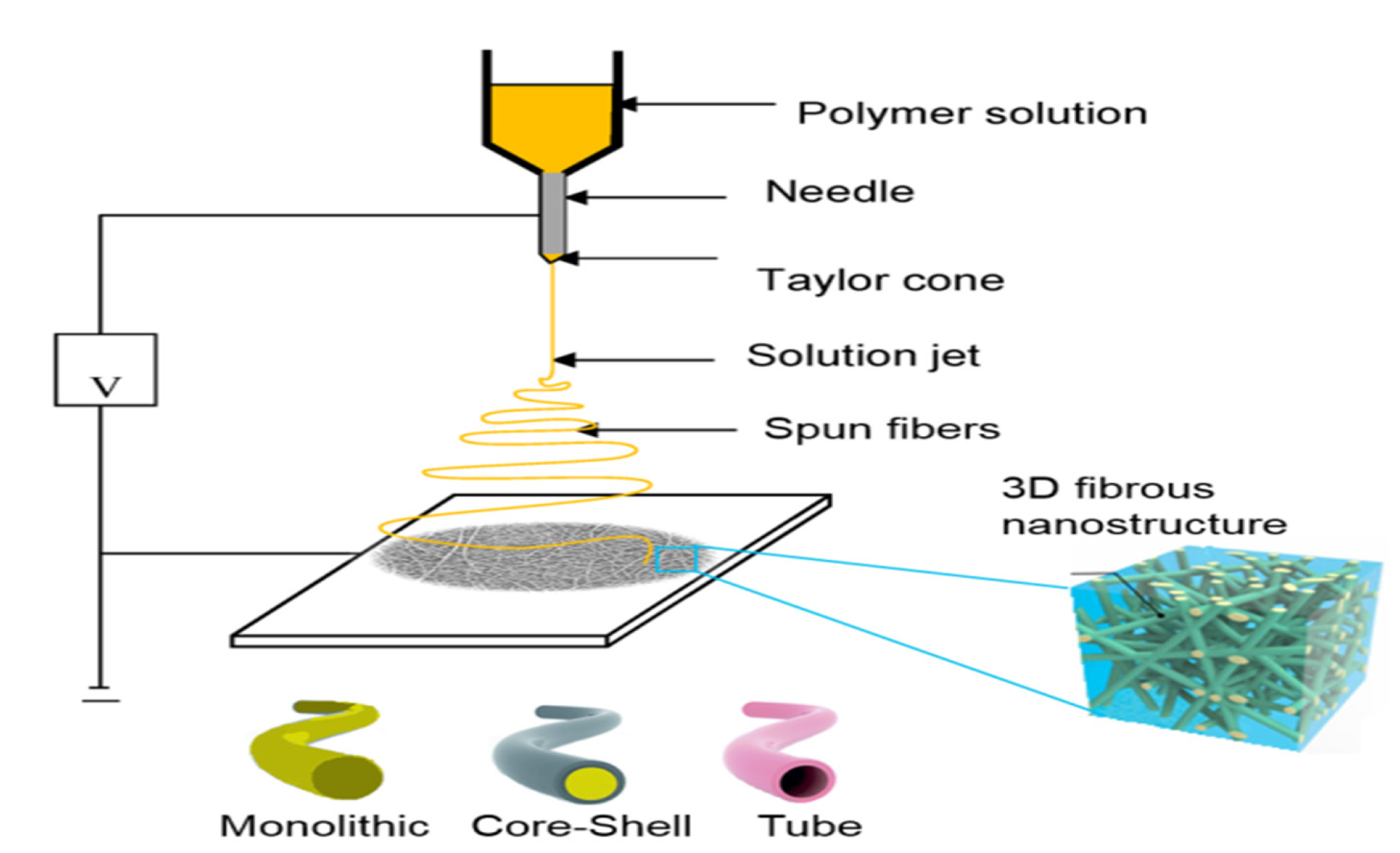
Figure 2:
Diagram of the electro spinning apparatus.126
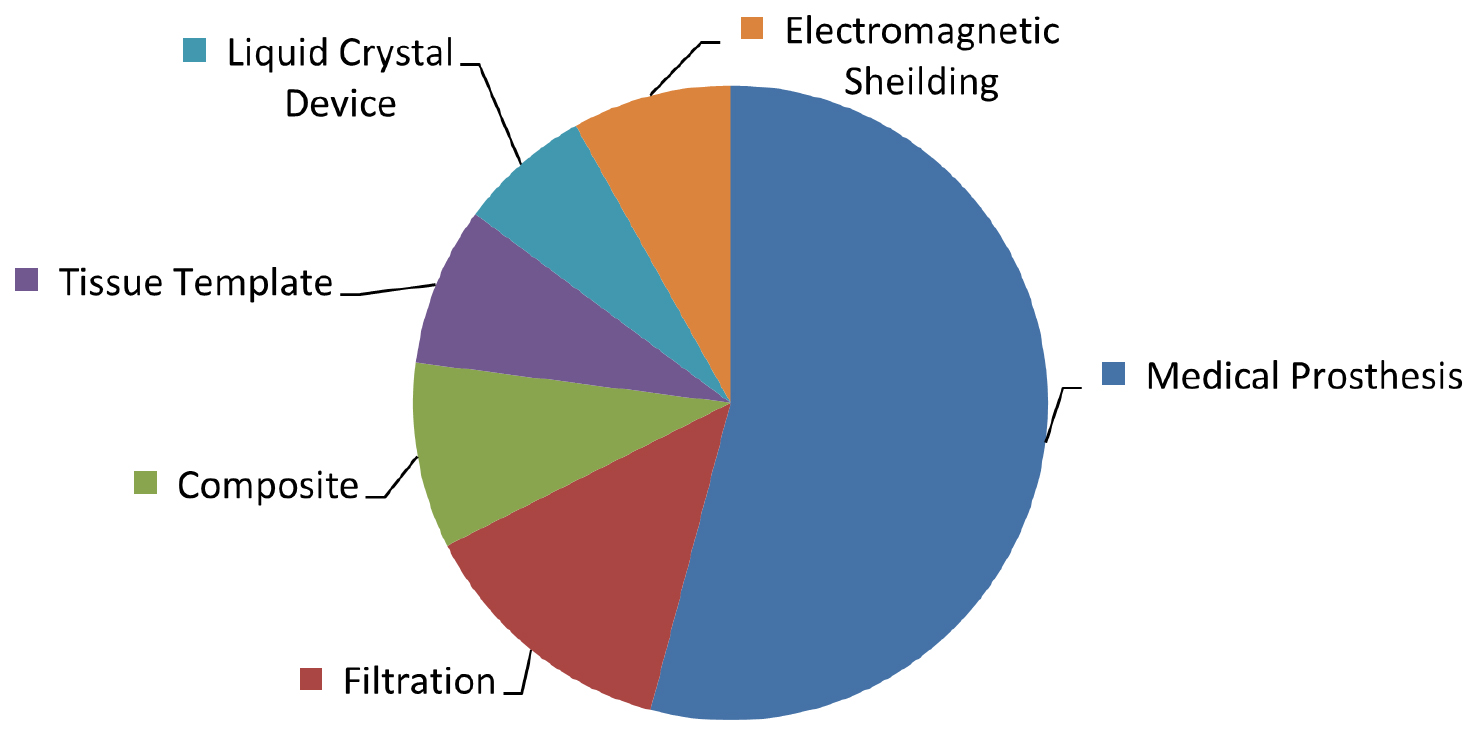
Figure 3:
Application fields targeted by US patents on electrospun nanofibers.59,60

Figure 4:
The mechanism of hydrogen adsorption using various carbon materials. (a): activated carbon, (b): single-walled carbon nanotube, (c): graphite.61,62
When there is no splitting from the jet, and when a polymer solution is viscous, fiber diameter is also influenced. If a key is denser, it produces fibers with greater diameters.17–20
A linear relationship between viscosity and polymer concentration will exist in any solvent in which a solid polymer dissolve. As a result, a solution with higher viscosity is always larger in diameter with increasing polymer concentration.21 Deitzel et al. found that nanofiber diameters grow with increasing polymer concentration based on a power law relationship. Fiber diameter is also greatly influenced by the voltage applied to it. Higher voltage results in a larger fiber diameter because more fluid is ejected in a jet (Table 1).22,23
Authors | Composition | Solvent | Concentrations | Functionality and Applications |
---|---|---|---|---|
Chang H.Y. et al.-2019.24 | Polymethylmethacrylate | THF, acetone, Chloroform. | 10 wt% | Super-hydrophobic units for active packaging. |
Gaaz-2015Park et al.-2018.25,26 | Polyvinyl alcohol | DI water | 8-16 wt%1-10 wt% | Biofilters and biomembranes. |
Fornaguera et al.-2015.27 | Poly lactic-co-glycolic acid. | Polysorbate 80, ethanol/ ethyl acetate | 4 wt% | Produced through low-energy nano-emulsification. |
Suwantong O. et al.-2016Potr c et al. -2015.28,29 | Polycaprolactone | Chloroform acetone | 10% (W/W) | Oro-mucosal drug delivery techniques show great potential. |
Valente et al.-2016.30 | Poly (L-lactic acid) | N, N- DMF and Dichloromethane. | 10 wt% | Sterilize PLLA membranes for regenerative medicine applications. |
Ghosh S.K. et al.-2017.31 | Gelatin | DI water | 30-50% (W/V) | This biomaterial’s adaptability to tissue regeneration. |
Muzzarelli et al.-2015 Haider et al.-2011.40,41 | Chitosan | TFA | 1-6 wt% | Wound healing and tissue engineering. |
Wang W et al.-2016.32 | Starch | DMSO, glutaraldehyde | 25 wt% | Tissue engineering, drug therapy, and medical |
Huang G.P. et al.-2015.33 | Collagen | TFA | 42.85% (W/W) | Structural fibers for tissue engineering |
Mohanty C. et al.-2017 Esmaili Z et al.-2018.34,35 | PLGA-curcumin | Chloroform/ methanol | 40 wt%/60 wt% | Slowly releasing curcumin |
Sadeghi A.R. et al.-2016.36 | PLGA-collagen | Hexafluoroiso-propanol | 20% (W/V) | Synthetic bioengineered skin |
Fukunishi T. et al.-2016.37 | PCL-chitosan | HFIP acetic acid | 20:1 (W/W) | It promotes cellular influx, neovascularization, and neo-tissue development without degenerative changes or catastrophe. |
BaradaranRafii A et al.-2015Choi M.O. et al.-2018.38,39 | PHBV-gelatin | Tetrafluoro-ethylene | 50 wt% | The amniotic membrane may be used as an alternative. |
Shao W et al.-2016.40 | Hydroxy apatite-tussah silk fibroin | Ammonia, citric acid | 31 wt% | Tissue and bone regeneration scaffolds |
Sessini V et al.-2018.41 | Polylactic acid/PCL-cellulose nanocrystals | Acetone, DCM, toluene with phosphorus pentoxide | 1wt% | Biodegradable packaging for biomedical or food |
Saberi A et al.-2015IspirliDogac Y. et al.-2017.42,43 | PVA/alginate-bioglass | DI water | 10 wt% | Hard and soft tissue biological and mechanical properties |
Dhand C. et al.-2016.44 | CaCO3-collagen/Poly catecholamine | HFIP, CaCl2 solution | 8% (W/V), 10% (W/W) | A multipurpose scaffold is required for bone tissue engineering. |
Pranav Kumar Shadamarshan, R et al.-2018.45 | PCL/PVP-trans anethole | Chloroform: methanol | 10% (W/V), 30% (W/V) | We can help mend and regenerate bone by growing osteoblasts in vitro. |
Unnithan, A.R. et al.-2015.46 | Polyurethane-estradiol- dextran | DMSOTHF | 10 wt% | Post menopause wound care |
Shao W. et al.-2016.40 | PLGA-tussah silk-graphene oxide | HFIP | 13 wt% | Biomaterials for cancer therapy and bone regrowth |
Hong B. et al.-2013 Liu C et al.-2018.47,48 | Polyvinylidene fluoride- silver-graphene oxide | Acetone DMF | 2 wt% | Magnetoelectric devices, energy harvesters |
Liao N et al.-2015.49 | Poly(“-caprolactone)- cellulose acetate- tetracycline HCl-dextran | DMF, THF | 10 wt% | Strong cell adhesion and proliferation, antimicrobial activities, wound dressing and skin engineering |
Composition, solvent, concentration, and functionality and applications of polymer fibers.
Author | Processing methods | Descriptions | Fiber dimensions | Features | |
---|---|---|---|---|---|
Dimensions | Length | ||||
Sadeghi A.R. et al. -1996, Z. M. HUANG et al.2003.5 5’ | Electrospinning | A Nanofiber-producing polymer solution or melt to get started, you need a polymer solution, two electrodes, and a direct current supply. | 3 nm to several μm. | continuous | -Easy and affordable from top to bottom -Multipurpose -continuous and randomly distributed industrial fibers. |
G. F. Ward et al.-2001 B. Gu et al.-2003.52 | Melt-blown | Microfabrication processes create the orifices, and molten polymers are extruded using high-velocity hot air gas. | 150 to 1000 nm. | continuous | -orifice size affects fiber size -challenging to obtain fibres thinner than 100 nm; still under development. |
P. X. Ma et al.-1999 F. Y. et al.-2004.53,54 | Phase separation | Composed of five steps: dissolving, gelation, phase separation, freezing, solvent extraction, and freeze-drying. | 50 to 500 nm. | few μm | -Making Nano fibrous foam right after freeze-drying; -Making foam takes a lengthy time; -Using specific (gelling) polymers like PLLA and its mix. |
J.D. Hartgerink et al.-2001 X. Yan et al.-2001.55,56 | Self-assembly | Atoms, molecules, and molecular aggregates at the micro and nanoscale form stable and geometrically well-defined functions via this process. | Well below 100 nm. | up to a few μm | -self-assembled materials -Inorganic synthesis is not capable of producing unique properties and functioning. -In some instances, more preparation time. |
C. R. Martin et al.-1996 L. Feng et al.-2002.57,58 | Template synthesis | Making nanoscale fibers using commercially available nanostructured films as templates for making them. | A few to hundreds nm. | μm | -nanotubes and fibrils made of polymers, carbons metals -mono-dispersed fiber diameters. |
Comparison of various processing methods for producing polymer Nanofiber.
Nanofibers In a different context
Polymer-based electrospun nanofibers have been intensively explored recently, notably for nanofiber composites. A US patent in this respect describes the filtering system and medical science’s dominance in detail (Figures 3 and 4). Nanofibers have several applications, including electromagnetic shielding and de-lamination of complex resistance. Many applications have not fulfilled industrial standards, although the research was done at the laboratory level. Academics, government agencies, and businesses worldwide are taking notice of and investing in these promising new technologies (Figure 5).
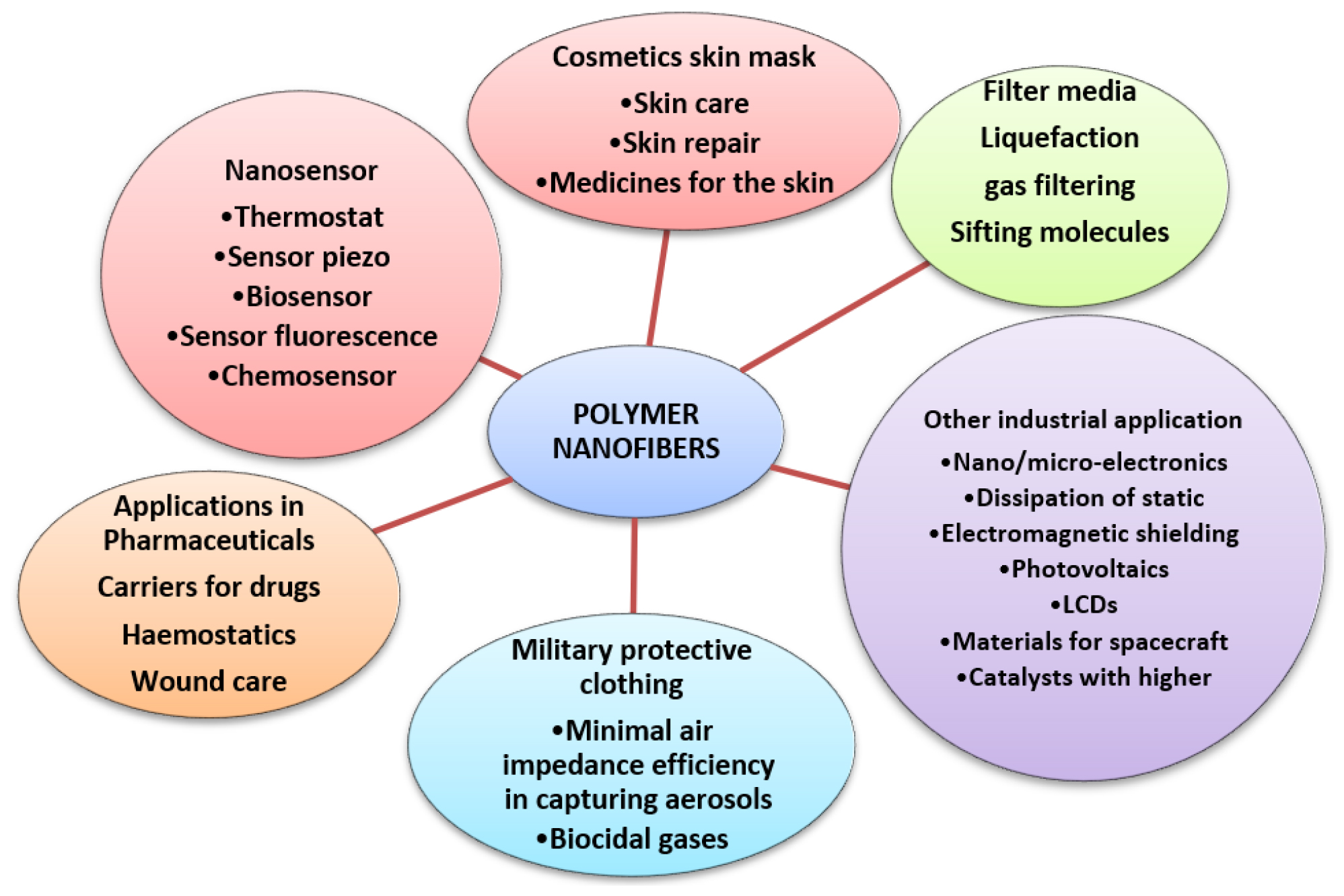
Figure 5:
Potential applications of electro spun polymer nanofibers.
Biomedical Applications of electro spun nanofibers
The majority of the human body comprises nanofibers, including bones, dentin, collagen, and skin, according to biomedical applications. Nanometer-scale manipulation of fibrous components is well known for these components. This current study might focus on manufacturing nanofibers employing a unique electrospinning technology for bioengineering purposes. One of these new tools will thus assist in discovering their promising potential in a broad range of biological disciplines, some of which are described below (Table 4).
Author | Route of Application | Drug incorporated | Polymer |
---|---|---|---|
Vashisth P, et al. 2017.68 | Oral route | Ofloxacin/gellan | PVA |
Vuddanda PR et al. 2016.69 | Ondansetron HCl | PVA | |
Potr C T et al. 2015.70 | Ibuprofen/carvedilol | PCL | |
Yu D-G et al. 2009.71 | Ibuprofen | Polyvinyl pyrrolidone | |
Li X et al 2013.72 | Caffeine/rib oflavin | PVA | |
Nagy ZK et al. 2010.73 | Donepezil HCl | PVA | |
Colley HE et al. 2018.74 | Clobetasol-17-propionate | Eudragit RS100/PVP/PEO | |
Li C et al. -2018.75 | Salmon calcitonin | Sodium alginate/PVA | |
Nageh H et al. 2014.76 | Dermal | Ciprofloxacin HCl | PVA/chitosan/PCL |
Yun J et al. 2011.77 | Ketoprofen | PVA/poly(acrylic acid)/multi-walled carbon nanotubes | |
Suwantong O-2008.78 | Asiaticoside | Cellulose acetate | |
Taepaiboon P-2007.79 | Vitamin A acid/Vitamin E | Cellulose acetate | |
Ngawhirunpat T et al. 2009.80 | Meloxicam | PVA | |
Mendes AC-2016.81 | Curcumin/ diclofenac/ vitamin B12 | Chitosan/ phospholipids | |
Souriyan-Reyhani pour H, et al. 2018.82 | Tetracycline HCl/ phenytoin Na | Cellulose acetate/PVA | |
Zhang X-2016.83 | Other | Collagen/salicylic acid | PVA |
Zhang L et al. 2018.84 | implants | Amoxicillin | Polyethylene glycol/PLGA |
Hu J et al. 2013.85 | Cefradine/5-fluorouracil | PLGA/gelatin | |
Doustgani A-2017.86 | Doxorubicin | PLA | |
75- Aguilar LE et al. 2015.87 | Paclitaxel | Polyurethane/Eudragit L100-55 |
Electro spun drug-loaded Nanofibers in drug-delivery applications.
Nanofibers in Energy storage materials
In addition to fossil fuels, nanofibers may store various forms of energy, such as natural gas and hydrogen. These carbon-based nanofibers’ numerous huge specific areas and high pore volume have been noted. Physical adsorption can be used to deposit these natural gases and hydrogen, making the utilization of gases simple. It was therefore compared to other materials, such as graphite, activated charcoal, and carbon nanotubes in Figure 5, to see how well these nanofibers could store energy.63
Engineered fibers such as Kevlar, glass, and carbon should be used as a backbone in developing complex-based nanofibers rather than traditional (μ) fibers such as cotton. The structural qualities of complex materials, such as more remarkable ability and superior mechanical strength to mass ratios, which any other mono-type materials could not promote causes alone, are good.1
This is why they have a more significant potential for use in constructing nano-complex structures. Because of this, nanofibers have been reported to have superior mechanical qualities over microfibers made with the same materials; hence nanocomposites have been anticipated to have excellent structural capabilities. It also has a few additional advantages that regular (microfiber) complexes can’t take advantage. It is possible to generate an opaque or non-transparent complex by mixing fibers and matrix with differing refractive indexes (one of the physical properties of the solid-state). It is possible to bypass this restriction if the diameter of the fiber is so short that it exceeds the wavelength of visible light.64
Nanofibers infiltration
On the other hand, nanofibers are used in a wide range of technical applications that need filtering. For the year 2020, it was estimated that the global filtration industry would be worth up to the US $700 billion.65
Filter media with fibrous material are widely used in these applications because they increase filtering effectiveness while simulating air resistance. It is essential to remember that fiber porosity is one of the vital factors in filter performance. A typical coalescing filter is employed to extract clean compressed air in large-scale businesses. Because of the tiny oil droplets, these filter media are essential (0.3 microns).66,67 It has been discovered that the electrospinning approach can deal with micron-sized particles. On the other hand, nanometer-sized fibers in the filter structure must fulfill the requirements of the particles or droplets that may be tracked in a filter to achieve more efficient and effective filtering (Table 3).26,27
Fiber Type | Fiber size (μm) | Fiber surface area per mass of fiber material (m2/g) |
---|---|---|
Nanofibers | 0.05 | 80 |
Spun bond fiber | 20 | 0.2 |
Melt blown fiber | 2.0 | 2 |
Fiber surface area per mass of fiber material for different fiber sizes.
Nanofibers in tissue regeneration
Organ and tissue failure may be treated using nanofibers, which are used to create new and optimal scaffolds that can imitate the human extracellular matrix’ functions. These malfunctioning human cells can bind the tiniest diameter of nanofibers and restore the tissue well throughout this procedure. Additionally, these nanofibre scaffold materials will generate a threshold amount of template for cells to seed, migrate, and increase. Regenerative tissue and organs may be successfully regenerated using a variety of nanofiber structures, including those that promote cell deposition and tissue growth (Table 5).88–90
Author | Application | Electro spun material/Electro spun scaffolds |
---|---|---|
Hu J et al. 2013.91 | Tissue engineering | Cefradine/5-fluorouracil/PLGA/gelatin |
Tian L et al. 2013.100 | Hydroxyapatite/laminin/PLCL | |
Xu W et al. 2017.101 | Alginate/PLA | |
Roy T et al. 2018.102 | Silk fibroin/PCL | |
Tan GZ-2018.103 | Vascular grafts | PCL/collagen (type I) |
Fu W et al. 2014.104 | Gelatin/PCL and collagen/PLCL | |
Du F et al. 2012.105 | Chitosan/PCL | |
Vatankhah E et al. 2014.94 | Tecophilic/gelatin | |
Sankaran KK et al. 2014.95 | PLA/PCL | |
Kim MJ et al. 2008.96 | PLGA/smooth muscle cells and endothelial cells | |
Ao C et al. 2017.97 | Cellulose/nano-hydroxyapatite | |
Heydari Z-2017.98 | PCL/octacalcium phosphate | |
Li C et. al. 2006.99 | Silk fibroin/bone morphogenetic protein -2/hydroxyapatite | |
Haider A-2014.100 | Bone grafts | PLGA/nHA/insulin |
Sharifi F et. al. 2108.101 | PCL/carb oxymethylchitosan | |
Enayati MS et al. 2018.102 | Nanohydroxyapatite/cellulose nanofibers/PVA |
Applications of the electrospun nanofibers in tissue engineering.
Nanofibers in wound care
They also serve an essential role in treating skin burns or wounds and hemostatic devices because of the identical unique qualities of polymer-based nanofibers. Electro-spun fibers have many unique properties, including the ability to form fibrous nest-like structures and resemble fibrous mat dressings when sprayed onto the injured area of skin, which aids in the healing of wounds by mimicking the formation of average skin growth and removing scar tissue, which would be done traditionally.92,93 As a result of their tiny diameters, these non-woven fibrous mats protect the wound against bacteria penetration by administering aerosol dosages. Additionally, these nanoparticles’ 5-100 m2 surface area makes them ideal for dermal delivery system dressings and effective sorption of fluids at damaged sites (Figure 6).103,104
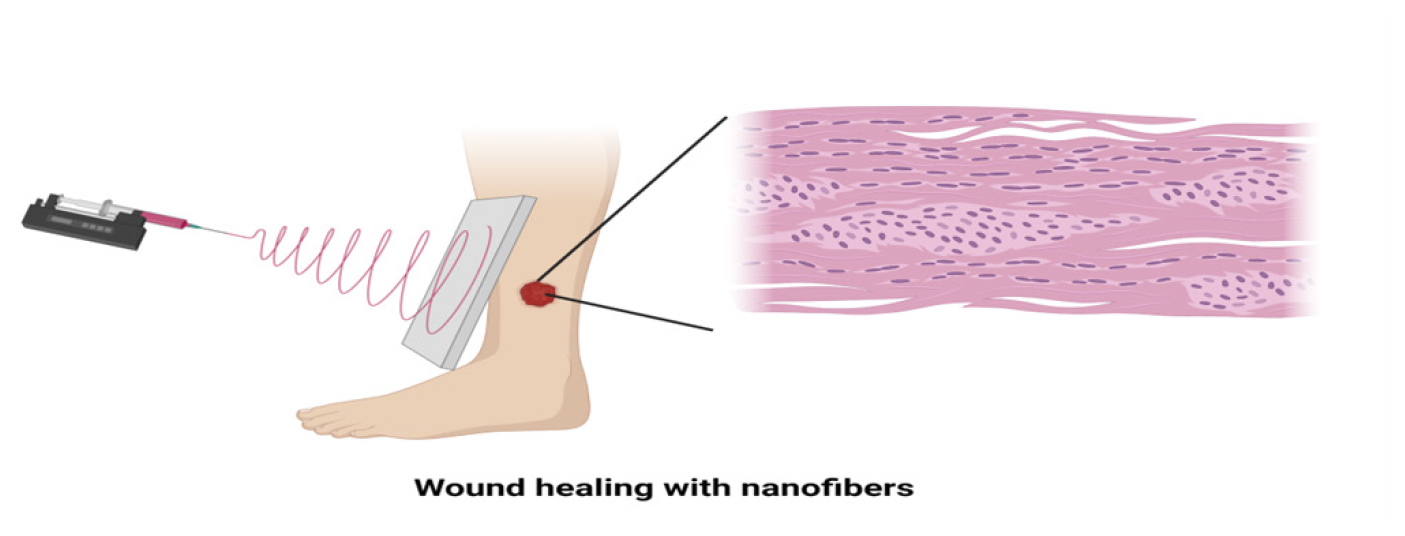
In addition, these characteristic electros spun polymer nanofibers are used as skin care protectants for the treatment of skin healing and cleansing, either with or without the presence of various excipients. Usually, these skin care nanofibrous materials can provide a larger surface area, allowing for more straightforward application and increasing the effectiveness of medication potentiality in the skin. Since electro spun nanofibrous may be applied quickly, without discomfort, and immediately to 3-D skin photography, this unique characteristic can help minimize skin condition mechanisms (Table 6).105
Author | Application | Electrospun material |
---|---|---|
Wang Z et al. 2015.5 | Wound dressing | PCL/hyaluronan/epidermal growth factor. |
Basar AO et al. 2017.6 | Ketoprofen/PCL/gelatin. | |
Garcia-Orue I et al. 2017.106 | Human epidermal growth factor and aloevera/PLGA. | |
Chitrattha S-2016.4 | Gentamicin sulfate/metronidazole/PLA. | |
Ajalloueian F et al. 2014.8 | PLGA/chitosan/PVA. | |
Fu R et al. 2016.107 | Sodium alginate/PVA/moxifloxacin hydrochloride. | |
Yao C-H et al. 2017.108 | Gelatin/keratin/PVA. | |
Saeed SM et al. 2017.109 | PCL/PVA/curcumin. | |
Wang M-2017.110 | Chitosan/PVA/ampicillin. | |
Ghalei S-2018.123 | PVA/zeinnano particles/Diclofenac. | |
Abdelgawad AM-2014.124 | Chitosan/silver-NPs/PVA. | |
Alavarse AC et al. 2017.125 | PVA/chitosan/tetracycline hydrochloride. | |
Aruan NM et al. 2017.114 | PVA/soursop leaves extract. | |
Shan Y-H et al. 2015.115 | Silk fibroin/gelatin. | |
Shin YC et al. 2016.116 | Hyaluronic acid/PLGA. | |
Alippilakkotte S 2017.117 | PLA/Ag NPs/Momordicacharantia fruit extract. | |
PLA-hyper branched polyglycerol/curcumin. | ||
Choi JI et al. 2017.118 | Spirulinaextract-alginate PCL. | |
Rath G et al. 2016.119 | Collagen/silver nanoparticles. | |
Lee C-H et al. 2014.120 | PLGA/metformin |
Applications of the emulsion electrospinning technique in wound dressing.
Nanofibers in drug delivery system
Medical applications rely heavily on these nanofibers, which are used for anything from medication delivery to gene therapy. Hollow carbon nanofibers, similar to nanotubes, are smaller than human blood cells and have a higher potential for transporting medications into blood cells than other nanofibers (Table 7 and Figure 7).105
Author | Application | Electro spun material |
---|---|---|
Hu J et al. 2015.13 | Drug delivery | Metformin-hydrochloride/metoprololtartrate/ PCL/p oly-3-hydroxybutyric acid-co-3-hydroxyvaleric acid. |
Shin J-2018.10 | Phytoncide/PVA. | |
Xu X et al. 2005.11 | Doxorubicin hydrochloride/PEG-PLLA. |
Applications of electro nano spun fibers in drug delivery system.
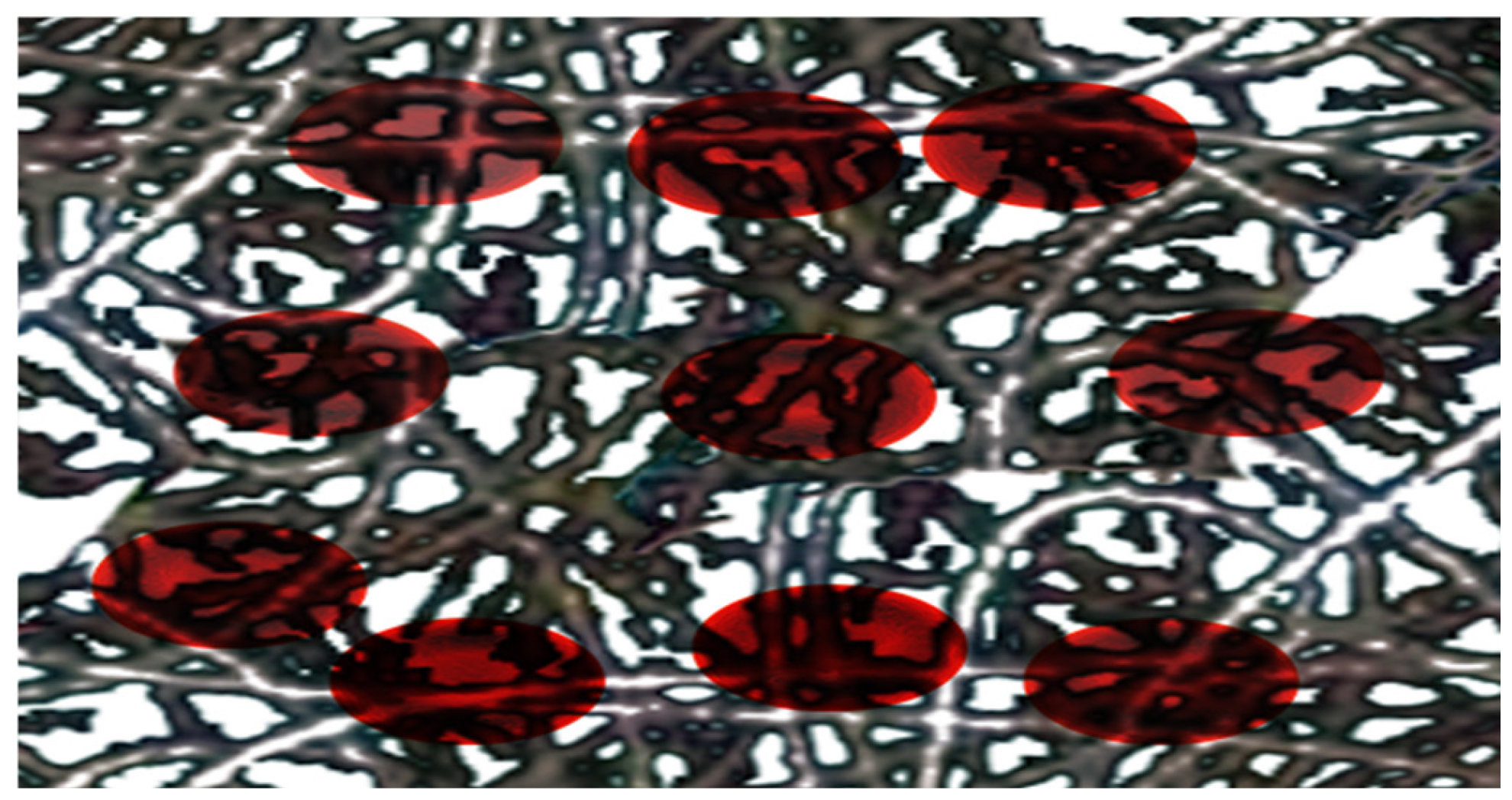
Figure 7:
Comparison of red blood cell with nanofibers.105
The list of electrospun-applied commercial products in biomedical applications are shown in Table 8.
Sl. No. | Product | Company | Country | Applications |
---|---|---|---|---|
1 | RIVELIN patch | Bioinicia | Spain | Drug delivery |
2 | PKpapyrus | Biotronik | Germany | Coveredstent |
3 | ReDuradurapatch | Medprin | Germany | Duraplasty |
4 | Nano fiber scaffolds | Stellenbosch (SNC) | SA | Biomedical |
5 | Scaffolds for tissue regeneration | The Electrospinning Company | UK | Biomedical |
6 | Antimicrobial dressings | PolyRemedy | USA | Wound care |
7 | AVflo™ vasculargraft | Nicast | Israel | Biomedical |
8 | ReBOSSIS | OrthoRebirth | Japan | Biomedical, Synthetic bone |
List of Electro spun-applied commercial products in biomedical applications. 121-125
Overcoming challenges and prospects for electrospun polymer nanofibers
Electrospinning has advanced dramatically in the last two decades. It has shown to be a powerful method for creating a range of functional nanostructures for various purposes. Electrospinning produces nanofibers with high specific surface area, homogeneous pore size, and high porosity, which increases their performance.
Furthermore, Electro spinning has started to reach the industrial industry. DuPont, Ahlstrom, Donaldson, and others have produced electrospinning-related filtering products. The electrospinning method may also be used to build nanofiber architectures by controlling polymer content, solvent, molecular weight, and conductivity. Meanwhile, chitosan, cellulose, lignin, PLA, PCL, PEO, and PVA have been used singly or in combination111 to construct nanostructures.
Nanofibers may be used for packaging, medicine delivery, filtration, fuel cells, and other purposes. Compared to standard pharmaceutical technology, electrospun side-by-side fiber architectures may achieve innovative two-phase drug release. This sustained-release behavior may boost medication plasma concentration and quickly cure symptoms by giving a “loading dose.”112
Despite these benefits, achieving therapeutic uses of electrospun mats will need precise and repeatable control of fiber shape, structure, and homogeneity.
Also, producing electrospun scaffolds with therapeutically relevant dimensions is complex. Despite its great flexibility and cheap cost, electrospinning’s collection pace is modest, raising questions about the process’s scalability. For biomedical applications, the absence of cell infiltration has lately hindered emerging technologies such as multilayer electrospinning, cell electrospray, and dynamic cell culture. Despite these obstacles, electrospun nanofibers and novel nanostructures have wide applications in various scientific fields.113
CONCLUSION
These nanofibers and webs have a greater possibility of delivering the drug directly to the target site. Anti-adhesive materials are increasingly being created using nanofibers comprised of cellulose. Current researchers have developed conventional spin. Blood contains nanofibers, which may be used in various medical applications, including the production of surgical bandages and sutures that dissolve fast in the body. As with infection rates and blood loss, these nanofibers are rapidly absorbed by the human body. Increased efficiency and reduced time necessary for filtering may be achieved by using nanofibers. The experts at the Natick Soldier’s Center in the United States proved the effect of nanofibers on filter aids for effective aerosol dosage form filtering. As opposed to the majority of filters that use a nanofiber substrate like sintered bronze or melt-blown fabric, they are superior. These nanofiber web components are included to offer mechanical stretto optimize filtration length, stabilization, and folding. Filter media deformation with an elastic MB coating led researchers to find that covering the substrate with nanofibers improves filtering performance.
From the above discussion, the present review concluded that these electros-spun nanofibers play a vital role in biomedical applications. These systems deliver good high-release profiles that could be attained successfully by preferring the electrospinning method side-by-side, which is very difficult to fabricate by traditional conventional techniques. Finally, these polymer-based nanofibers may convey a broad range of new drugs to supplement the natural biological rhythm for maximum therapeutic results.
Cite this article:
Sarma KN, Thalluri C, Mandhadi JR. Nanofibers in Drug Delivery Systems: A Comprehensive Scientific Review of Recent Approaches. Int. J. Pharm. Investigation. 2024;14(3):633-46.
ACKNOWLEDGEMENT
The authors wish to acknowledge the support of this review article by the Assam Down Town University (AdtU), the Department of Pharmacy, Faculty of Pharmaceutical Sciences, Panikhaiti, and Guwahati 781206, India.
ABBREVIATIONS
CMC | Carboxy methyl cellulose |
---|---|
PVA | Polyvinyl Alcohol |
Nm | Nanometer |
μm | Micrometre |
PS | Polystyrene |
PAN | Polyacrylonitrile polymer |
THF | Tetrahydrofuran |
DI water | Deionized water |
DMF | Dimethylformamide |
PLLA | Poly Lactic Acid |
TFA | Trifluoroacetic acid |
DMSO | Dimethyl sulfoxide |
PCL | Poly Capro Lactone |
DCM | Di-chloro methane |
PVP base | Polyvinyl Pyrrolidine |
PLA | Poly Lactic Acid |
PEO | Poly ethylene oxide |
PLCL | Poly(L-Lactide-co-ε-caprolactone |
nHA | Hydroxyapatite nanorods |
NPS | Nano particles |
PEG | Polyethene glycol |
PLGA | PolyLactic- co- Glycolic acid |
PCL | Polycaprolactone |
HFIP | Hexafluoro-2-propanol |
References
- Huang ZM, Zhang YZ, Kotaki M, Ramakrishna S. A review on polymer nanofibers by electrospinning and their applications in nanocomposites. Composites Science and Technology. 2003;63:2223-53. [CrossRef] | [Google Scholar]
- Shin SH, Purevdorj O, Castano O, Planell JA, Kim HW. A short review: Recent advances in electrospinning for bone tissue regeneration. Journal of Tissue Engineering. 2012;3:1-11. [CrossRef] | [Google Scholar]
- Wang C, Wang J, Zeng L, Qiao Z, Liu X, Liu H, et al. Fabrication of electrospun polymer nanofibers with diverse morphologies. Molecules. 2019:24 [CrossRef] | [Google Scholar]
- Chitrattha S, Phaechamud T. Porous poly(dl-lactic acid) matrix film with antimicrobial activities for wound dressing application. Materials Science and Engineering: C. 2016;58:1122-30. [CrossRef] | [Google Scholar]
- Wang Z, Qian Y, Li L, Pan L, Njunge LW, Dong L, et al. Evaluation of emulsion electrospun polycaprolactone/hyaluronan/epidermal growth factor nanofibrous scaffolds for wound healing. Journal of Biomaterials Applications. 2016;30:686-98. [CrossRef] | [Google Scholar]
- Basar AO, Castro S, Torres-Giner S, Lagaron JM, Turkoglu Sasmazel H. Novel poly(ε-caprolactone)/gelatin wound dressings prepared by emulsion electrospinning with controlled release capacity of Ketoprofen anti-inflammatory drug. Materials Science and Engineering: C. 2017;81:459-68. [CrossRef] | [Google Scholar]
- Tian L, Prabhakaran MP, Ding X, Ramakrishna S. Biocompatibility evaluation of emulsion electrospun nanofibers using osteoblasts for bone tissue engineering. 2013;24:1952-68.
Http://DxDoiOrg/101080/092050632013814096
[CrossRef] | [Google Scholar] - Ajalloueian F, Tavanai H, Hilborn J, Donzel-Gargand O, Leifer K, Wickham A, et al. Emulsion electrospinning as an approach to Fabricate PLGA/chitosan nanofibers for biomedical applications. BioMed Research International. 2014:2014 [CrossRef] | [Google Scholar]
- Xu W, Shen R, Yan Y, Gao J. Preparation and characterization of electrospun alginate/PLA nanofibers as tissue engineering material by emulsion eletrospinning. Journal of the Mechanical Behavior of Biomedical Materials. 2017;65:428-38. [CrossRef] | [Google Scholar]
- Shin J, Lee S. Encapsulation of Phytoncide in Nanofibers by Emulsion Electrospinning and their Antimicrobial Assessment. Fibers and Polymers. 2018;19(3):627-34. [CrossRef] | [Google Scholar]
- Xu X, Yang L, Xu X, Wang X, Chen X, Liang Q, et al. Ultrafine medicated fibers electrospun from W/O emulsions. Journal of Controlled Release. 2005;108:33-42. [CrossRef] | [Google Scholar]
- Roy T, Maity PP, Rameshbabu AP, Das B, John A, Dutta A, et al. Core-Shell Nanofibrous Scaffold Based on Polycaprolactone-Silk Fibroin Emulsion Electrospinning for Tissue Engineering Applications. Bioengineering. 2018;5:68 [CrossRef] | [Google Scholar]
- Hu J, Prabhakaran MP, Tian L, Ding X, Ramakrishna S. Drug-loaded emulsion electrospun nanofibers: characterization, drug release and biocompatibility. RSC Advances. 2015;5:100256-67. [CrossRef] | [Google Scholar]
- Topuz F, Uyar T. Electrospinning of gelatin with tunable fiber morphology from round to flat/ribbon. Materials Science and Engineering: C. 2017;80:371-8. [CrossRef] | [Google Scholar]
- Megelski S, Stephens JS, Bruce Chase D, Rabolt JF. Micro- and Nanostructured Surface Morphology on Electrospun Polymer Fibers. Macromolecules. 2002;35:8456-66. [CrossRef] | [Google Scholar]
- Deitzel JM, Kleinmeyer J, Harris D, Tan NCB. The effect of processing variables on the morphology of electrospun nanofibers and textiles. n.d. [CrossRef] | [Google Scholar]
- Electrostatic Spinning of Acrylic Microfibers. n.d. [CrossRef] | [Google Scholar]
- Doshi J, Reneker DH. ELSEVIER Journal of Electrostatics. Electrospinning Process and Applications of Electrospun Fibers. n.d. 1995;35:151-60. [CrossRef] | [Google Scholar]
- Fong H, Chun I, Reneker DH. Beaded nanofibers formed during electrospinning. n.d. [CrossRef] | [Google Scholar]
- Mohammad Ali Zadeh M, Keyanpour-Rad M, Ebadzadeh T. Effect of viscosity of polyvinyl alcohol solution on morphology of the electrospun mullite nanofibres. Ceramics International. 2014;40:5461-6. [CrossRef] | [Google Scholar]
- Demir MM, Yilgor I, Yilgor E, Erman B. Electrospinning of polyurethane ®bers. n.d. [CrossRef] | [Google Scholar]
- Setayesh S, Marsitzky D, Müllen K. Nanostructured Fibers via Electrospinning** for additional scanning electron micro-scopy investigations. Figure 1. Scanning Electron Microscopy (SEM) micrograph of electrospun PLLA fibers. 2000:33 [CrossRef] | [Google Scholar]
- Hu JL, Huang JH, Chih YK, Chuang CC, Chen JP, Cheng SH, et al. Effects of thermal treatments on the supercapacitive performances of PAN-based carbon fiber electrodes. Diamond and Related Materials. 2009;18:511-5. [CrossRef] | [Google Scholar]
- Chang H-Y, Chang C-C, Cheng L-P. Preparation of hydrophobic nanofibers by electrospinning of PMMA dissolved in 2-propanol and water. MATEC Web of Conferences. 2019;264:03004 [CrossRef] | [Google Scholar]
- Gaaz TS, Sulong AB, Akhtar MN, Kadhum AAH, Mohamad AB, Al-Amiery AA, et al. Properties and Applications of Polyvinyl Alcohol, Halloysite Nanotubes and Their Nanocomposites. Molecules. 2015;20:22833-47. [CrossRef] | [Google Scholar]
- Park MJ, Gonzales RR, Abdel-Wahab A, Phuntsho S, Shon HK. Hydrophilic polyvinyl alcohol coating on hydrophobic electrospun nanofiber membrane for high performance thin film composite forward osmosis membrane. Desalination. 2018;426:50-9. [CrossRef] | [Google Scholar]
- Fornaguera C, Dols-Perez A, Calderó G, García-Celma MJ, Camarasa J, Solans C, et al. PLGA nanoparticles prepared by nano-emulsion templating using low-energy methods as efficient nanocarriers for drug delivery across the blood-brain barrier. Journal of Controlled Release. 2015;211:134-43. [CrossRef] | [Google Scholar]
- Suwantong O. Biomedical applications of electrospun polycaprolactone fiber mats. Polymers for Advanced Technologies. 2016;27:1264-73. [CrossRef] | [Google Scholar]
- Potrč T, Baumgartner S, Roškar R, Planinšek O, Lavrič Z, Kristl J, et al. Electrospun polycaprolactone nanofibers as a potential oromucosal delivery system for poorly water-soluble drugs. European Journal of Pharmaceutical Sciences. 2015;75:101-13. [CrossRef] | [Google Scholar]
- Valente TAM, Silva DM, Gomes PS, Fernandes MH, Santos JD, Sencadas V, et al. Effect of Sterilization Methods on Electrospun Poly(lactic acid) (PLA) Fiber Alignment for Biomedical Applications. ACS Applied Materials and Interfaces. 2016;8:3241-9. [CrossRef] | [Google Scholar]
- Ghosh SK, Adhikary P, Jana S, Biswas A, Sencadas V, Gupta SD, et al. Electrospun gelatin nanofiber based self-powered bio-e-skin for health care monitoring. Nano Energy. 2017;36:166-75. [CrossRef] | [Google Scholar]
- Wang W, Jin X, Zhu Y, Zhu C, Yang J, Wang H, et al. Effect of vapor-phase glutaraldehyde crosslinking on electrospun starch fibers. Carbohydrate Polymers. 2016;140:356-61. [CrossRef] | [Google Scholar]
- Huang GP, Shanmugasundaram S, Masih P, Pandya D, Amara S, Collins G, et al. An investigation of common crosslinking agents on the stability of electrospun collagen scaffolds. Journal of Biomedical Materials Research Part A. 2015;103:762-71. [CrossRef] | [Google Scholar]
- Mohanty C, Sahoo SK. Curcumin and its topical formulations for wound healing applications. Drug Discovery Today. 2017;22:1582-92. [CrossRef] | [Google Scholar]
- Esmaili Z, Bayrami S, Dorkoosh FA, Akbari Javar H, Seyedjafari E, Zargarian SS, et al. Development and characterization of electrosprayed nanoparticles for encapsulation of Curcumin. Journal of Biomedical Materials Research Part A. 2018;106:285-92. [CrossRef] | [Google Scholar]
- Sadeghi AR, Nokhasteh S, Molavi AM, Khorsand-Ghayeni M, Naderi-Meshkin H, Mahdizadeh A, et al. Surface modification of electrospun PLGA scaffold with collagen for bioengineered skin substitutes. Materials Science and Engineering: C. 2016;66:130-7. [CrossRef] | [Google Scholar]
- Fukunishi T, Best CA, Sugiura T, Shoji T, Yi T, Udelsman B, et al. Tissue-Engineered Small Diameter Arterial Vascular Grafts from Cell-Free Nanofiber PCL/Chitosan Scaffolds in a Sheep Model. PLOS ONE. 2016;11:e0158555 [CrossRef] | [Google Scholar]
- Baradaran-Rafii A, Biazar E, Heidari-Keshel S. 2015;64:879-87.
Http://DxDoiOrg/101080/0091403720151030658
Cellular Response of Limbal Stem Cells on PHBV/Gelatin Nanofibrous Scaffold for Ocular Epithelial Regeneration. - Choi MO, Kim YJ. Effect of poly(3-hydroxybutyrate-co-3-hydroxyvalerate)/gelatin ratios on the characteristics of biomimetic composite nanofibrous scaffolds. Colloid and Polymer Science. 2018;296(5):917-26. [CrossRef] | [Google Scholar]
- Shao W, He J, Sang F, Wang Q, Chen L, Cui S, et al. Enhanced bone formation in electrospun poly(l-lactic-co-glycolic acid)-tussah silk fibroin ultrafine nanofiber scaffolds incorporated with graphene oxide. Materials Science and Engineering: C. 2016;62:823-34. [CrossRef] | [Google Scholar]
- Sessini V, Navarro-Baena I, Arrieta MP, Dominici F, López D, Torre L, et al. Effect of the addition of polyester-grafted-cellulose nanocrystals on the shape memory properties of biodegradable PLA/PCL nanocomposites. Polymer Degradation and Stability. 2018;152:126-38. [CrossRef] | [Google Scholar]
- Rafienia M, Saberi A, Poorazizi E. A novel fabrication of PVA/Alginate-Bioglass electrospun for biomedical engineering application. 2017 [CrossRef] | [Google Scholar]
- İspirli Doğaç Y, Deveci İ, Mercimek B, Teke M. A comparative study for lipase immobilization onto alginate based composite electrospun nanofibers with effective and enhanced stability. International Journal of Biological Macromolecules. 2017;96:302-11. [CrossRef] | [Google Scholar]
- Dhand C, Barathi VA, Ong ST, Venkatesh M, Harini S, Dwivedi N, et al. Latent Oxidative Polymerization of Catecholamines as Potential Cross-linkers for Biocompatible and Multifunctional Biopolymer Scaffolds. ACS Applied Materials and Interfaces. 2016;8:32266-81. [CrossRef] | [Google Scholar]
- PranavKumar Shadamarshan R, Balaji H, Rao HS, Balagangadharan K, Viji Chandran S, Selvamurugan N, et al. Fabrication of PCL/PVP Electrospun Fibers loaded with Trans-anethole for Bone Regeneration
. Colloids and Surfaces B: Biointerfaces. 2018;171:698-706. [CrossRef] | [Google Scholar] - Unnithan AR, Sasikala ARK, Murugesan P, Gurusamy M, Wu D, Park CH, et al. Electrospun polyurethane-dextran nanofiber mats loaded with Estradiol for post-menopausal wound dressing. International Journal of Biological Macromolecules. 2015;77:1-8. [CrossRef] | [Google Scholar]
- Hong B, Jung H, Byun H. Preparation of polyvinylidene fluoride nanofiber membrane and its antibacterial characteristics with nanosilver or graphene oxide. Journal of Nanoscience and Nanotechnology. 2013;13:6269-74. [CrossRef] | [Google Scholar]
- Liu C, Shen J, Liao CZ, Yeung KWK, Tjong SC. Novel electrospun polyvinylidene fluoride-graphene oxide-silver nanocomposite membranes with protein and bacterial antifouling characteristics. Express Polymer Letters. 2018;12:365-82. [CrossRef] | [Google Scholar]
- Liao N, Unnithan AR, Joshi MK, Tiwari AP, Hong ST, Park CH, et al. Electrospun bioactive poly (?-caprolactone)-cellulose acetate-dextran antibacterial composite mats for wound dressing applications. Colloids and Surfaces A: Physicochemical and Engineering Aspects. 2015;469:194-201. [CrossRef] | [Google Scholar]
- Reneker DH, Chun I. Nanometre diameter fibres of polymer, produced by electrospinning. Nanotechnology. 1996;7:216 [CrossRef] | [Google Scholar]
- Huang ZM, Zhang YZ, Kotaki M, Ramakrishna S. A review on polymer nanofibers by electrospinning and their applications in nanocomposites. Composites Science and Technology. 2003;63:2223-53. [CrossRef] | [Google Scholar]
- Ward GF. Meltblown nanofibres for nonwoven filtration applications. Filtration & Separation. 2001;38:42-3. [CrossRef] | [Google Scholar]
- Ma PX, Zhang R. Synthetic nano-scale fibrous extracellular matrix. 1999 [CrossRef] | [Google Scholar]
- Yang F, Murugan R, Ramakrishna S, Wang X, Ma YX, Wang S, et al. Fabrication of nano-structured porous PLLA scaffold intended for nerve tissue engineering. Biomaterials. 2004;25:1891-900. [CrossRef] | [Google Scholar]
- Hartgerink JD, Beniash E, Stupp SI. Self-assembly and mineralization of peptide-amphiphile nanofibers. Science (1979). 2001;294:1684-8. [CrossRef] | [Google Scholar]
- Liu G, Qiao L, Guo A. Diblock Copolymer Nanofibers. n.d. [CrossRef] | [Google Scholar]
- Martin CR. Membrane-Based Synthesis of Nanomaterials. 1996 [CrossRef] | [Google Scholar]
- Feng L, Li S, Li H, Zhai J, Song Y, Jiang L, et al. CDCl 3): d 1.68±1.88 (m, 2 H), 2.00±2.42 (m, 6 H), 2.68±2.76 (m, 3 H), 5.47 (dd, J 15.6, 6.1 Hz, 1 H), 5.60 (dt, J 15.6, 6.3 Hz, 1 H), 7.17±7.38 (m, 5 H); 13 C NMR (100 MHz. B. Bosnich;. 2002:114 [CrossRef] | [Google Scholar]
- Im JS, Park SJ, Lee YS. The metal-carbon-fluorine system for improving hydrogen storage by using metal and fluorine with different levels of electronegativity. International Journal of Hydrogen Energy. 2009;34:1423-8. [CrossRef] | [Google Scholar]
- Im JS, Park SJ, Lee YS. Superior prospect of chemically activated electrospun carbon fibers for hydrogen storage. Materials Research Bulletin. 2009;44:1871-8. [CrossRef] | [Google Scholar]
- Kayaci F, Aytac Z, Uyar T. Surface modification of electrospun polyester nanofibers with cyclodextrin polymer for the removal of phenanthrene from aqueous solution. Journal of Hazardous Materials. 2013;261:286-94. [CrossRef] | [Google Scholar]
- Chand S. Review Carbon fibers for composites. n.d. [CrossRef] | [Google Scholar]
- Michel Bergshoef BM, Julius Vancso G. Transparent Nanocomposites with Ultrathin, Electrospun Nylon-4,6 Fiber Reinforcement**. 1999:11 [CrossRef] | [Google Scholar]
- Tsai PP, Schreuder-Gibson H, Gibson P. Different electrostatic methods for making electret filters. 2002:54 [CrossRef] | [Google Scholar]
- Williams G, Graham K, Ouyang M, Raether T, Grafe T, Mcdonald B, et al. Polymeric Nanofibers in Air Filtration Applications. n.d. [CrossRef] | [Google Scholar]
- Laurencin CTA, Ambrosio AM, Borden MD, Cooper JA. Tissue Engineering: Orthopedic Applications. 1999 [CrossRef] | [Google Scholar]
- Buchko CJ, Chen LC, Shen Y, Martin DC. Processing and microstructural characterization of porous biocompatible protein polymer thin films. n.d. [CrossRef] | [Google Scholar]
- Vashisth P, Raghuwanshi N, Srivastava AK, Singh H, Nagar H, Pruthi V, et al. Ofloxacin loaded gellan/PVA nanofibers-Synthesis, characterization and evaluation of their gastroretentive/mucoadhesive drug delivery potential. Materials Science and Engineering: C. 2017;71:611-9. [CrossRef] | [Google Scholar]
- Vuddanda PR, Mathew AP, Velaga S. Electrospun nanofiber mats for ultrafast release of ondansetron. Reactive and Functional Polymers. 2016;99:65-72. [CrossRef] | [Google Scholar]
- Potrč T, Baumgartner S, kaRošr R, Planinšek O, Lavrič Z, Kristl J, et al. Electrospun polycaprolactone nanofibers as a potential oromucosal delivery system for poorly water-soluble drugs. European Journal of Pharmaceutical Sciences. 2015;75:101-13. [CrossRef] | [Google Scholar]
- Yu DG, Shen XX, Branford-White C, White K, Zhu LM, Annie Bligh SW, et al. Oral fast-dissolving drug delivery membranes prepared from electrospun polyvinylpyrrolidone ultrafine fibers. Nanotechnology. 2009;20:055104 [CrossRef] | [Google Scholar]
- Li X, Kanjwal MA, Lin L, Chronakis IS. Electrospun polyvinyl-alcohol nanofibers as oral fast-dissolving delivery system of caffeine and riboflavin. Colloids and Surfaces B: Biointerfaces. 2013;103:182-8. [CrossRef] | [Google Scholar]
- Nagy ZK, Nyúl K, Wagner I, Molnár K, Marosi G. Electrospun water soluble polymer mat for ultrafast release of donepezil HCl. Express Polymer Letters. 2010;4:763-72. [CrossRef] | [Google Scholar]
- Colley HE, Said Z, Santocildes-Romero ME, Baker SR, D’Apice K, Hansen J, et al. Pre-clinical evaluation of novel mucoadhesive bilayer patches for local delivery of clobetasol-17-propionate to the oral mucosa. Biomaterials. 2018;178:134-46. [CrossRef] | [Google Scholar]
- Li C, Wei YS, Wen P, Feng K, Zong MH, Wu H, et al. Preparation and characterization of an electrospun colon-specific delivery system for salmon calcitonin. RSC Advances. 2018;8:9762-9. [CrossRef] | [Google Scholar]
- Nageh H, Ezzat M, Ghanim M, Hassanin A, El-Moneim AA. Evaluation of Antibacterial Activity and Drug Release Behavior of Chitosan-Based Nanofibers ( Study). Pharmaceutical and Biosciences Journal. 2014;2:1-5. [CrossRef] | [Google Scholar]
- Yun J, Im JS, Lee YS, Kim Hil. Electroresponsive transdermal drug delivery behavior of PVA/PAA/MWCNT nanofibers. European Polymer Journal. 2011;47:1893-902. [CrossRef] | [Google Scholar]
- Suwantong O, Ruktanonchai U, Supaphol P. Electrospun cellulose acetate fiber mats containing asiaticoside or crude extract and the release characteristics of asiaticoside. Polymer (Guildf). 2008;49:4239-47. [CrossRef] | [Google Scholar]
- Taepaiboon P, Rungsardthong U, Supaphol P. Vitamin-loaded electrospun cellulose acetate nanofiber mats as transdermal and dermal therapeutic agents of vitamin A acid and vitamin E. European Journal of Pharmaceutics and Biopharmaceutics. 2007;67:387-97. [CrossRef] | [Google Scholar]
- Ngawhirunpat T, Opanasopit P, Rojanarata T, Akkaramongkolporn P, Ruktanonchai U, Supaphol P, et al. Development of Meloxicam-Loaded Electrospun Polyvinyl Alcohol Mats as a Transdermal Therapeutic Agent. 2008;14:73-82.
Http://DxDoiOrg/101080/10837450802409420
[CrossRef] | [Google Scholar] - Mendes AC, Gorzelanny C, Halter N, Schneider SW, Chronakis IS. Hybrid electrospun chitosan-phospholipids nanofibers for transdermal drug delivery. International Journal of Pharmaceutics. 2016;510:48-56. [CrossRef] | [Google Scholar]
- Souriyan-Reyhanipour H, Khajavi R, Yazdanshenas ME, Zahedi P, Mirjalili M. 2018;33:597-611.
Https://DoiOrg/101177/0883911518779186
Cellulose acetate/poly(vinyl alcohol) hybrid fibrous mat containing tetracycline hydrochloride and phenytoin sodium: Morphology, drug release, antibacterial, and cell culture studies. - Zhang X, Tang K, Zheng X. Electrospinning and crosslinking of COL/PVA Nanofiber-microsphere Containing Salicylic Acid for Drug Delivery. Journal of Bionic Engineering. 2016;13(1):143-9. [CrossRef] | [Google Scholar]
- Zhang L, Wang Z, Xiao Y, Liu P, Wang S, Zhao Y, et al. Electrospun PEGylated PLGA nanofibers for drug encapsulation and release. Materials Science and Engineering: C. 2018;91:255-62. [CrossRef] | [Google Scholar]
- Hu J, Wei J, Liu W, Chen Y. 2013;24:972-85.
Http://DxDoiOrg/101080/092050632012728193
Preparation and characterization of electrospun PLGA/gelatin nanofibers as a drug delivery system by emulsion electrospinning. - Doustgani A. 2016;47:71-88.
Http://DxDoiOrg/101177/1528083716634033
Doxorubicin release from optimized electrospun polylactic acid nanofibers. - Aguilar LE, Unnithan AR, Amarjargal A, Tiwari AP, Hong ST, Park CH, et al. Electrospun polyurethane/Eudragit® L100-55 composite mats for the pH dependent release of paclitaxel on duodenal stent cover application. International Journal of Pharmaceutics. 2015;478:1-8. [CrossRef] | [Google Scholar]
- Fertala A, Han WB, Ko FK. Mapping critical sites in collagen II for rational design of gene-engineered proteins for cell-supporting materials. 2001:57 [CrossRef] | [Google Scholar]
- Huang L, McMillan RA, Apkarian RP, Pourdeyhimi B, Conticello VP, Chaikof EL, et al. Generation of synthetic elastin-mimetic small diameter fibers and fiber networks. Macromolecules. 2000;33:2989-97. [CrossRef] | [Google Scholar]
- Jin HJ, Fridrikh Sv, Rutledge GC, Kaplan DL. Electrospinning Bombyx mori silk with poly(ethylene oxide). Biomacromolecules. 2002;3:1233-9. [CrossRef] | [Google Scholar]
- Tan GZ, Zhou Y. Tunable 3D Nanofiber Architecture of Polycaprolactone by Divergence Electrospinning for Potential Tissue Engineering Applications. Nano-Micro Letters. 2018;10:1-10. [CrossRef] | [Google Scholar]
- Fu W, Liu Z, Feng B, Hu R, He X, Wang H, et al. Electrospun gelatin/PCL and collagen/PLCL scaffolds for vascular tissue engineering. International Journal of Nanomedicine. 2014;9:2335 [CrossRef] | [Google Scholar]
- Du F, Wang H, Zhao W, Li D, Kong D, Yang J, et al. Gradient nanofibrous chitosan/poly ?-caprolactone scaffolds as extracellular microenvironments for vascular tissue engineering. Biomaterials. 2012;33:762-70. [CrossRef] | [Google Scholar]
- Vatankhah E, Prabhakaran MP, Semnani D, Razavi S, Morshed M, Ramakrishna S, et al. Electrospun tecophilic/gelatin nanofibers with potential for small diameter blood vessel tissue engineering. Biopolymers. 2014;101:1165-80. [CrossRef] | [Google Scholar]
- Sankaran KK, Vasanthan KS, Krishnan UM, Sethuraman S. Development and evaluation of axially aligned nanofibres for blood vessel tissue engineering. Journal of Tissue Engineering and Regenerative Medicine. 2014;8:640-51. [CrossRef] | [Google Scholar]
- Kim MJ, Kim JH, Yi G, Lim SH, Hong YS, Chung DJ, et al. Array. Macromolecular Research. 2008;16(4):345-52. [CrossRef] | [Google Scholar]
- Ao C, Niu Y, Zhang X, He X, Zhang W, Lu C, et al. Fabrication and characterization of electrospun cellulose/nano-hydroxyapatite nanofibers for bone tissue engineering. International Journal of Biological Macromolecules. 2017;97:568-73. [CrossRef] | [Google Scholar]
- Heydari Z, Mohebbi-Kalhori D, Afarani MS. Engineered electrospun polycaprolactone (PCL)/octacalcium phosphate (OCP) scaffold for bone tissue engineering. Materials Science and Engineering: C. 2017;81:127-32. [CrossRef] | [Google Scholar]
- Li C, Vepari C, Jin HJ, Kim HJ, Kaplan DL. Electrospun silk-BMP-2 scaffolds for bone tissue engineering. Biomaterials. 2006;27:3115-24. [CrossRef] | [Google Scholar]
- Haider A, Gupta KC, Kang IK. PLGA/nHA hybrid nanofiber scaffold as a nanocargo carrier of insulin for accelerating bone tissue regeneration. Nanoscale Research Letters. 2014;9:1-12. [CrossRef] | [Google Scholar]
- Sharifi F, Atyabi SM, Norouzian D, Zandi M, Irani S, Bakhshi H, et al. Polycaprolactone/carboxymethyl chitosan nanofibrous scaffolds for bone tissue engineering application. International Journal of Biological Macromolecules. 2018;115:243-8. [CrossRef] | [Google Scholar]
- Enayati MS, Behzad T, Sajkiewicz P, Rafienia M, Bagheri R, Ghasemi-Mobarakeh L, et al. Development of electrospun poly (vinyl alcohol)-based bionanocomposite scaffolds for bone tissue engineering. Journal of Biomedical Materials Research Part A. 2018;106:1111-20. [CrossRef] | [Google Scholar]
- Jin HJ, Fridrikh Sv, Rutledge GC, Kaplan DL. Electrospinning silk with poly(ethylene oxide). Biomacromolecules. 2002;3:1233-9. [CrossRef] | [Google Scholar]
- Unnithan AR, Arathyram RS, Kim CS. Electrospinning of Polymers for Tissue Engineering. Nanotechnology Applications for Tissue Engineering, Elsevier Inc.;. 2015:45-55. [CrossRef] | [Google Scholar]
- Manea LR, Popa A, Bertea AP. Medical applications of functional electrospun nanofibers -A review. Key Engineering Materials, vol. 752 KEM, Trans Tech Publications Ltd;. 2017:132-8. [CrossRef] | [Google Scholar]
- Garcia-Orue I, Gainza G, Gutierrez FB, Aguirre JJ, Evora C, Pedraz JL, et al. Novel nanofibrous dressings containing rhEGF and for wound healing applications. International Journal of Pharmaceutics. 2017;523:556-66. [CrossRef] | [Google Scholar]
- Fu R, Li C, Yu C, Xie H, Shi S, Li Z, et al. A novel electrospun membrane based on moxifloxacin hydrochloride/poly(vinyl alcohol)/sodium alginate for antibacterial wound dressings in practical application. Drug Delivery. 2016;23:828-39. [CrossRef] | [Google Scholar]
- Yao CH, Lee CY, Huang CH, Chen YS, Chen KY. Novel bilayer wound dressing based on electrospun gelatin/keratin nanofibrous mats for skin wound repair. Materials Science and Engineering: C. 2017;79:533-40. [CrossRef] | [Google Scholar]
- Saeed SM, Mirzadeh H, Zandi M, Barzin J. Designing and fabrication of curcumin loaded PCL/PVA multi-layer nanofibrous electrospun structures as active wound dressing. Progress in Biomaterials. 2017;6(1):39-48. [CrossRef] | [Google Scholar]
- Wang M, Roy AK, Webster TJ. Development of chitosan/poly(vinyl alcohol) electrospun nanofibers for infection related wound healing. Frontiers in Physiology. 2017;7:683 [CrossRef] | [Google Scholar]
- Ghalei S, Asadi H, Ghalei B. Zein nanoparticle-embedded electrospun PVA nanofibers as wound dressing for topical delivery of anti-inflammatory diclofenac. Journal of Applied Polymer Science. 2018;135:46643 [CrossRef] | [Google Scholar]
- Abdelgawad AM, Hudson SM, Rojas OJ. Antimicrobial wound dressing nanofiber mats from multicomponent (chitosan/silver-NPs/polyvinyl alcohol) systems. Carbohydrate Polymers. 2014;100:166-78. [CrossRef] | [Google Scholar]
- Alavarse AC, de Oliveira Silva FW, Colque JT, da Silva VM, Prieto T, Venancio EC, et al. Tetracycline hydrochloride-loaded electrospun nanofibers mats based on PVA and chitosan for wound dressing. Materials Science and Engineering: C. 2017;77:271-81. [CrossRef] | [Google Scholar]
- Aruan NM, Sriyanti I, Edikresnha D, Suciati T, Munir MM, Khairurrijal K, et al. Polyvinyl Alcohol/ Leaves Extract Composite Nanofibers Synthesized Using Electrospinning Technique and their Potential as Antibacterial Wound Dressing. Procedia Engineering. 2017;170:31-5. [CrossRef] | [Google Scholar]
- Shan YH, Peng LH, Liu X, Chen X, Xiong J, Gao JQ, et al. Silk fibroin/gelatin electrospun nanofibrous dressing functionalized with astragaloside IV induces healing and anti-scar effects on burn wound. International Journal of Pharmaceutics. 2015;479:291-301. [CrossRef] | [Google Scholar]
- Shin YC, Shin DM, Lee EJ, Lee JH, Kim JE, Song SH, et al. Hyaluronic Acid/PLGA Core/Shell Fiber Matrices Loaded with EGCG Beneficial to Diabetic Wound Healing. Advanced Healthcare Materials. 2016;5:3035-45. [CrossRef] | [Google Scholar]
- Alippilakkotte S, Kumar S, Sreejith L. Fabrication of PLA/Ag nanofibers by green synthesis method using fruit extract for wound dressing applications. Colloids and Surfaces A: Physicochemical and Engineering Aspects. 2017;529:771-82. [CrossRef] | [Google Scholar]
- Choi Jil, Kim MS, Chun GY, Shin HS. Array. Biotechnology and Bioprocess Engineering. 2018;22(6):679-85. [CrossRef] | [Google Scholar]
- Rath G, Hussain T, Chauhan G, Garg T, Goyal AK. 2015;24:520-9.
Http://DxDoiOrg/103109/1061186X20151095922
Collagen nanofiber containing silver nanoparticles for improved wound-healing applications. - Lee CH, Hsieh MJ, Chang SH, Lin YH, Liu SJ, Lin TY, et al. Enhancement of Diabetic Wound Repair Using Biodegradable Nanofibrous Metformin-Eluting Membranes: and
. ACS Applied Materials and Interfaces. 2014;6:3979-86. [CrossRef] | [Google Scholar] - Geremia F. Quality aspects for medical devices, quality system and certification process. Microchemical Journal. 2018;136:300-6. [CrossRef] | [Google Scholar]
- Zyga S, Stathoulis J. S P E C I A L P A P E R Application of ISO 1348 5: 2003 in Biomedical Engineering: a Systematic Review. International Journal of Caring Sciences. 2011:4 [CrossRef] | [Google Scholar]
- ISO 1348 5: 2016(en), Medical devices-Quality management systems-Requirements for regulatory purposes n.d. [accessed February 9, 2022].
https://www.iso.org/obp/ui/#iso:std:iso:13485:ed-3:v1:en:en - ISO 10993-1: 2018(en), Biological evaluation of medical devices-Part 1: Evaluation and testing within a risk management process n.d. [accessed February 9, 2022].
https://kebs.isolutions.iso.org/ob p/ui#iso:std:iso:10993:-1:ed-5:v2:en - ISO 80601-2-13: 2011(fr), Appareils électromédicaux-Partie 2-13: Exigences particulières de sécurité de base et de performances essentielles pour les postes de travail d’anesthésie n.d. [accessed February 9, 2022].
https://www.iso.org/obp/ui#iso:std:iso:80601:-2-13:ed-1:v1:fr - https://app.biorender.com/gallery/illustrations/folder/65ac126289712352b71fb916