Contents
ABSTRACT
Objectives
Lipases are widely used in biodiesel, food, detergent, and pharmaceutical industries. To fulfil the rising demand for these crucial industrial enzymes, an extensive study on lipases from various micro-organisms will be useful in maximising the growing conditions and reducing production costs.
Materials and Methods
The current work concentrated on optimising the carbon source, inducer concentration, and growth parameters to produce lipases at a reasonable price from pseudomonas species.
Results
Extracellular lipases from Pseudomonas fluorescens were produced and optimised, achieving a concentration of 220 IU/mL of submerged fermentation broth.
Conclusion
The results showed that the extracellular lipase production from Pseudomonas fluorescens was enhanced by three folds using submerged fermentation.
INTRODUCTION
Lipases belong to the triacylglycerol ester hydrolase (E.C. 3.1.1.3) family. They catalyse the breakdown of long-chain triglycerides into fatty acids, diacylglycerols, monoacylglycerols, and glycerols. In addition to hydrolytic activity, they exhibit transesterification, esterification, aminolysis, and alcoholysis activities that are important in various industries.1,2 Microbial lipases are widely used for reasons such as the diversity of available catalytic activities, high production yields, ease of genetic manipulation, lack of seasonal variation, regular supply, excellent stability, safety, and convenience.3–5 Lipases isolated from microorganisms offer higher activity and thermal stability associated with neutral or alkaline pH. Pseudomonas alcaligenes, Pseudomonas aeruginosa, Pseudomonas fluorescens and Bacillus subtilis are bacterial strains that produce lipases in considerable amounts.6–9 The fungal strain that produces lipases includes Penicillium expansum, Trichoderma, and Aspergillus niger.10 Several microbial lipases have been identified to be thermostable at temperatures >70°C and alkaline stability at pH>9.11–13 Microbial lipases are ubiquitous in nature and are commercially important because they are cheaper to produce, more stable, and more readily available than animal and plant lipases.14–16 Natural or recombinant microbial lipases are widely used in various biotechnological applications. Most of the extracellular lipases are derived from fungi and bacteria species.17,18
As versatile biological catalysts, lipases are expected to meet the needs of various industries such as detergent, food and beverages, leather, textiles, detergents, pharmaceuticals, medicals and biodiesel.11,19 Microbial lipases have already shown great potential for use in various industries. Lipases are often used as flavour enhancers in dairy products.20 Lipase enzymes break down greasy stains, and surfactants in detergents stick to the residue and remove stains from clothing.21 Lipase is used along with other enzymes to remove the glue from the spools of yarn that aid in dyeing.22 Lipases significantly increase oil yield during vegetable oil processing and improve the appearance of the final product. Lipases also play important roles in wastewater treatment (degradation and removal of oils), cosmetics (removal of lipids), leather (removal of lipids from animal skin), pharmaceuticals (digestion of oils and fats in food) and medicine (blood triglycerides-examination).23,24 Lipases were also investigated to hydrolyse chemically created biodegradable polymers to substrate analogues.25–27 Lipases are currently being studied in producing cocoa butter equivalents and breast milk fat equivalents.28,29
MATERIALS AND METHODS
Media and growth condition
Pseudomonas fluorescens culture was obtained from Vishwamitra bio agro private Limited. 100 mL LB broth was incubated with pseudomonas culture (1%) inoculated into 100 mL LB broth and incubated at 37°C till it grew exponentially. Pseudomonas culture was then transferred to lipase production media.
Lipase production
Lipase production media was prepared with 1-5% (w/v) dextrose, 1-10% (v/v) olive oil, 1% yeast extract, 0.07 g of K2HPO4, 0.03 g of KH2PO4, 0.05 g MgSO4, 0.01 g MnCl2, 0.01 g CaCl2 and sterilized. The fermentation media was then seeded with an inoculum size of 1–5% (v/v) and submerged fermentation was carried out in 5 L flasks for 72 hr at 37°C. The fermentation broth was centrifuged at 6000 rpm for 10 min after 3 days. The source of lipases was taken from the supernatant.
Lipase purification
Ammonium sulphate precipitation (70% saturation) was employed to pellet down the proteins in the supernatant. The solution was then centrifuged for 10 min at 6000 rpm. 10 mM phosphate buffer was used to dissolve the protein pellet that was produced following centrifugation. The protein solution was then dialysed several times to remove the excess salt. The crude extract of lipases thus obtained was purified using CM Sephadex C50 column (50 cm X 2 cm) chromatography. Flow rate was maintained at 1 mL/min using 10 mM potassium phosphate buffer and fraction size was limited to 1 mL. A sodium chloride gradient of 0 M to 0.5 M was used to elute the bound proteins. For the eluted fractions, optical density was measured at 280 nm and a chromatogram was plotted (Figure 1A).
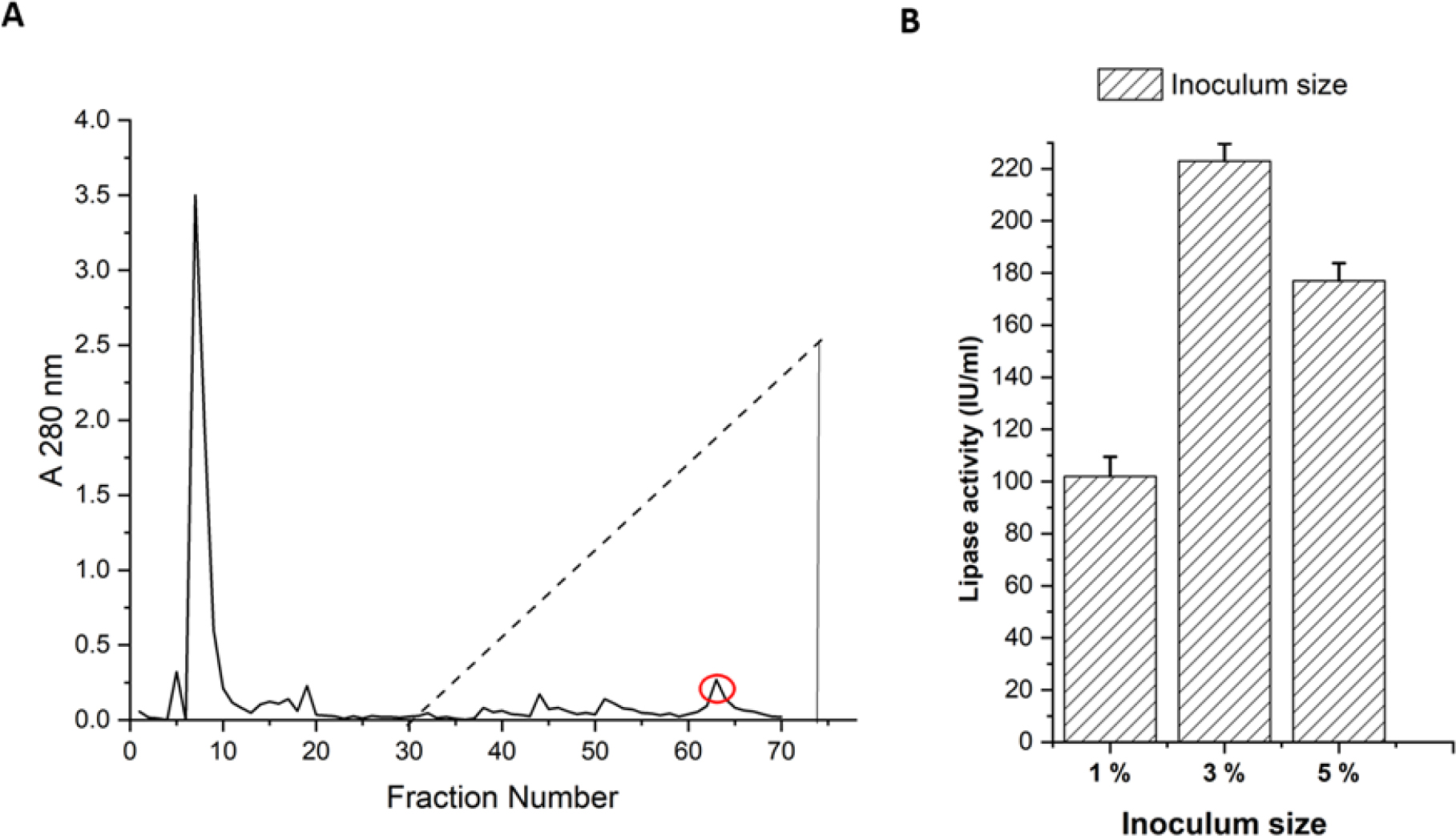
Figure 1.
A. Purification of lipase using CM Sephadex C50 column chromatography B. Effect of inoculum size on lipase production.
Lipase activity assay
P-Nitrophenyl Butyrate (p-NPB) was used as the substrate for the spectrophotometric determination of lipase activity, with some minor modifications to the procedure published by Krieger et al. in 2019. Nine parts of solution A (100 mM sodium phosphate buffer, 150 mM sodium chloride and 0.5% (V/V) Triton X100) and one part of solution B (50 mM p-NPB) were taken as a reaction mixture. 0.9 mL of solution A was mixed with 0.1 mL of 50 mM p-NPB and 0.1 mL of purified lipase extract. The reaction mixture was then incubated at 37°C for 5 min. A blank solution devoid of p-NPB substrate was used as a reference for measuring the amount of P. nitrophenol butyrate released during enzymatic reaction at 400 nm. The amount of enzyme required to catalyse the release of 1 nanomole of p-nitrophenol from P. nitrophenyl butyrate per minute under test conditions was designated as one International Unit (IU) of lipase activity. The formula below was used to compute the enzyme activity.
where DF is the dilution factor and 0.0148 is the micromolar extinction coefficient of p-nitrophenol at 400 nm.
Temperature effect on lipase activity
Lipase activity was measured after the purified lipase enzyme was heated in a hot water bath for 10 min at temperatures ranging from 30°C to 80°C.
pH effect on lipase activity
Lipase enzymes were then exposed to solutions with different pHs ranging from pH 4 to pH 9 for 10 min. The impact of pH on a pure enzyme was then estimated using a lipase activity assay.
Statistical analysis
Results were reported as Mean±SEM. All analyses findings were determined to be statistically significant at p<0.05.
RESULTS
Lipase production
To enhance the production of lipase, Pseudomonas fluorescens culture was used with an inoculum size ranging from 1-5%. Different variants of dextrose concentrations (1%-5%) and olive oil concentrations (1%-10%) were also used to optimize the production of lipases. In a 5 L flask, submerged fermentation was done for 72 hr using the aforementioned versions along with additional minerals and nutrients. The fermentation broth was then subjected to centrifugation and the microbial cells were pelleted down. Extracellular lipases were then collected in the supernatant and subjected to (NH4)2SO4 precipitation.
Lipase purification
Lipase crude solution was subjected to fractionation using CM Sephadex C50 cation exchange chromatography. Figure 1A shows the chromatogram that was obtained during cation exchange chromatography. All the peak fractions in the chromatogram were tested for lipase activity. The protein peak highlighted with red showed significant lipase activity.
Influence of inoculum percentage on lipase production
Lipase production was done with three different variants of inoculum (1%, 3% and 5%) using submerged fermentation. We observed maximum production of lipases with 3% inoculum size compared to the other two variants Figure 1B.
Effect of inducer and initial carbon levels on lipase production
Pseudomonas lipase production was optimized by adjusting the initial carbon and inducer concentrations. Initial carbon concentration has minimal effect on lipase production. We observed a slightly higher concentration of lipase produced with 3% dextrose in broth (Figure 2A). An increase in olive oil (inducer) concentration from 1-5% exhibited significant enhancement in lipase production, but no major change in lipase production was observed beyond 5% olive oil concentration. When compared to other variants, significantly more lipases were generated with a 5% olive oil concentration (Figure 2B).
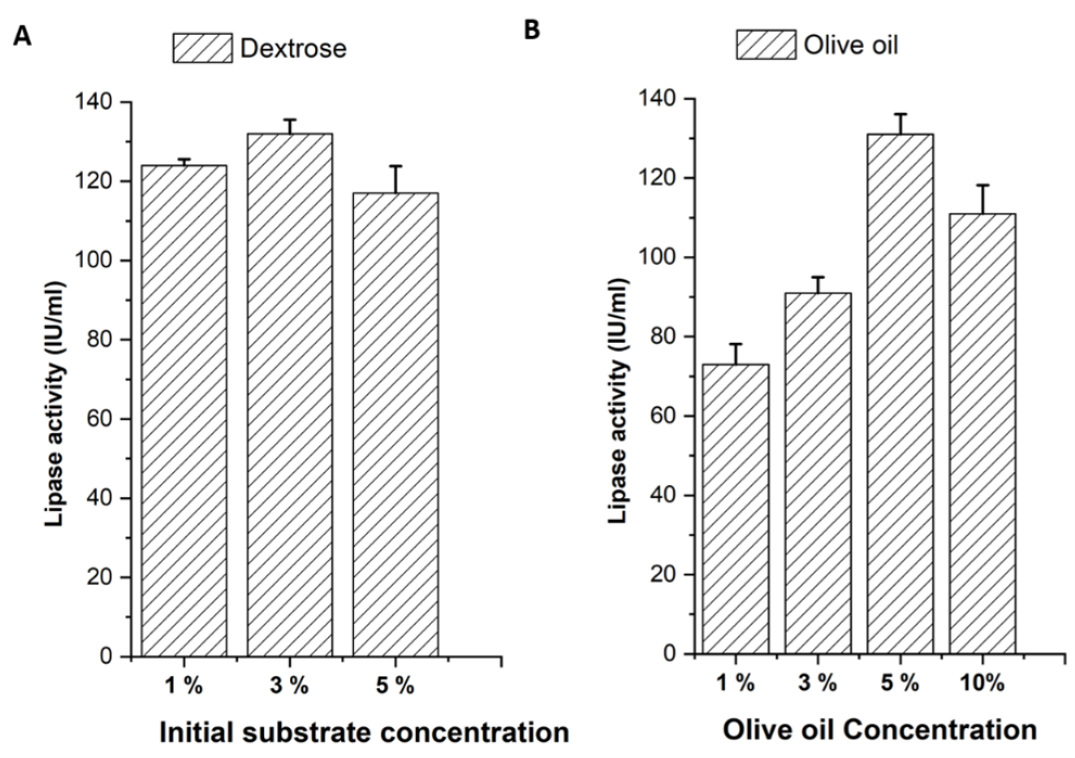
Figure 2.
A. Effect of initial substrate concentrations on lipase production B. Effect of inducer concentrations on lipase production.
Temperature effects on lipase activity
Temperatures ranging from 30°C to 80°C were used to test the lipase activity. We observed purified lipases to be thermally stable even at 60°C and lipase activity gradually decreased from 60°C to 80°C (Figure 3A).
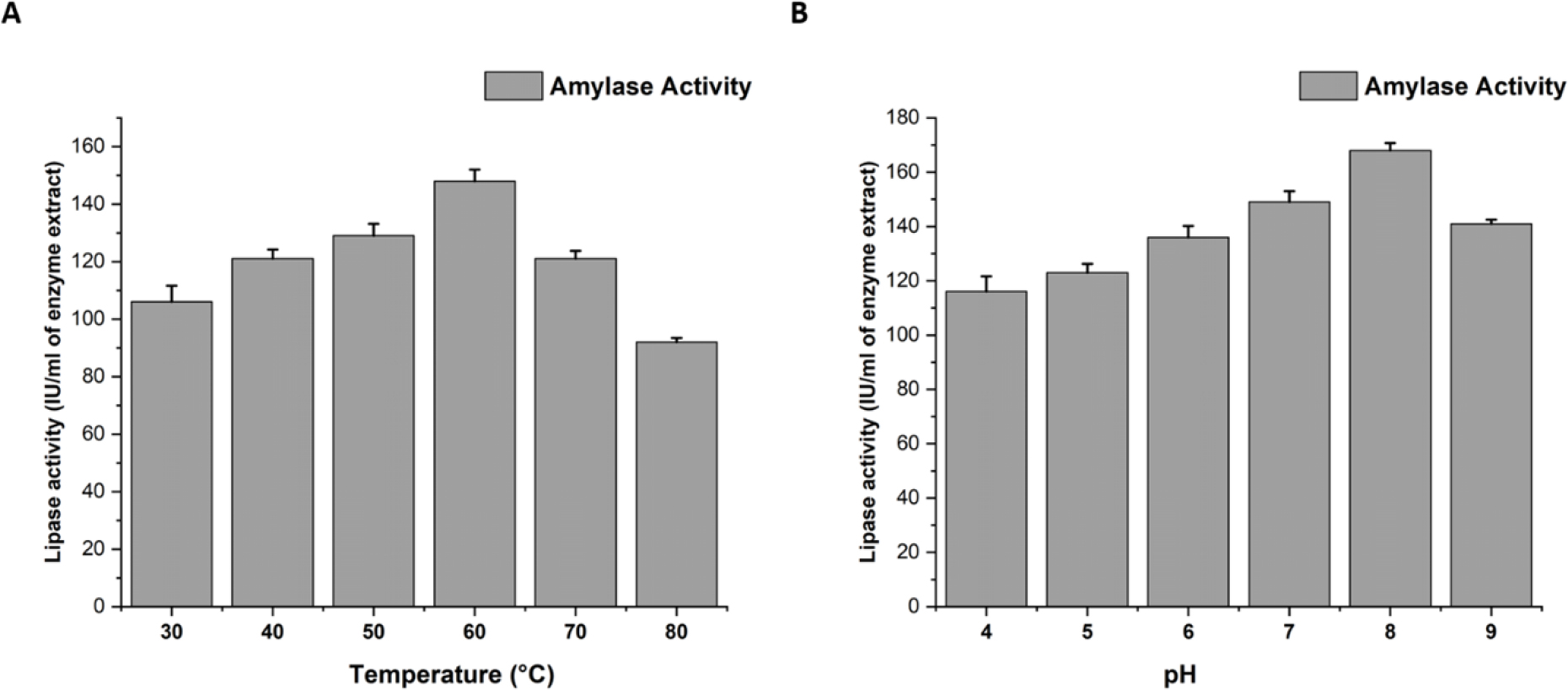
Figure 3.
A. Effect of temperature on lipase activity. B. Effect of pH on lipase activity.
Effect of pH on lipase activity
pH showed a drastic effect on lipase production. We observed maximum lipase activity at pH 8 (Figure 3B).
DISCUSSION
Lipases belonging to the triacylglycerol ester hydrolase family of enzymes are widely used in biopharmaceutical industries. A production that is optimised is necessary to increase productivity and satisfy the industrial demand for lipases. The current study used submerged fermentation to perform purification and production optimisation of extracellular lipases from Pseudomonas fluorescens. Optimization studies were performed with three different initial substrate concentrations of dextrose and 4 different concentrations of olive oil as inducers. We observed a slightly higher concentration of lipase produced with 3% dextrose in broth. An increase in olive oil (inducer) concentration from 1-5% exhibited significant enhancement in lipase production, but no major change in lipase production was observed beyond 5% olive oil concentration. When compared to other variants, significantly more lipases were generated with a 5% olive oil concentration. Inoculum size can be an additional factor which determines the lipase production rate. We observed higher production of lipases with 3% inoculum in the fermentation broth. We were able to optimize the production of Pseudomonas fluorescens lipases to a concentration of 220 IU/mL of broth.
CONCLUSION
Lipase production from Pseudomonas fluorescens was optimized using several media variants in submerged fermentation. Significantly more lipases were generated with 5% olive oil concentration, 3% inoculum size and 3% dextrose concentration in the fermentation broth. The results showed that the extracellular lipase production from Pseudomonas fluorescens was enhanced by three folds using submerged fermentation.
Cite this article:
Bandi L, Lakshmisetty NS, Ankireddy K, Pulipati LLV, Bontu AL, Aluru RR, et al. Optimization of Lipase Production from Pseudomonas fluorescens.
ACKNOWLEDGEMENT
The authors would like to thank the management of Koneru Lakshmaiah Education Foundation Vaddeswaram, Guntur, for helping us with the necessary resources.
ABBREVIATIONS
p-NPB | Para Nitrophenyl Butyrate |
---|---|
CM | Corboxy methyl |
mM | milli Molar |
DF | Dilution Factor |
References
- Chandra P, Enespa SR, Arora PK. Microbial lipases and their industrial applications: A comprehensive review. Microb Cell Factories. 2020;19(1):1-42. [Google Scholar]
- Houde A, Kademi A, Leblanc D. Lipases and their industrial applications: an overview. Appl Biochem Biotechnol. 2004;118(1-3):155-70. [PubMed] | [CrossRef] | [Google Scholar]
- Hamdan SH, Maiangwa J, Nezhad NG, Ali MSM, Normi YM, Shariff FM, et al. Knotting terminal ends of mutant T1 lipase with disulfide bond improved structure rigidity and stability. Appl Microbiol Biotechnol. 2023;107(5-6):1673-86. [PubMed] | [CrossRef] | [Google Scholar]
- Sharma N, Vuppu S. Computational modelling and molecular docking of industrial leather enzymes. Mol Biotechnol. 2023 [PubMed] | [CrossRef] | [Google Scholar]
- Borrelli GM, Trono D. Recombinant lipases and phospholipases and their use as biocatalysts for industrial applications. Int J Mol Sci. 2015;16(9):20774-840. [PubMed] | [CrossRef] | [Google Scholar]
- Rios NS, Pinheiro BB, Pinheiro MP, Bezerra RM, dos Santos JCS, Barros Gonçalves LR, et al. Biotechnological potential of lipases from Pseudomonas: sources, properties and applications. Process Biochem. 2018;75:99-120. [CrossRef] | [Google Scholar]
- da Pereira SA, Fontes-Sant’Ana GC, Amaral PFF. Mango agro-industrial wastes for lipase production from and the potential of the fermented solid as a biocatalyst. Food Bioprod Process. 2019;115:68-77. [CrossRef] | [Google Scholar]
- Soberón-Chávez G, Palmeros B. Pseudomonas lipases: molecular genetics and potential industrial applications. Crit Rev Microbiol. 1994;20(2):95-105. [PubMed] | [CrossRef] | [Google Scholar]
- Javed S, Azeem F, Hussain S, Rasul I, Siddique MH, Riaz M, et al. Bacterial lipases: a review on purification and characterization. Prog Biophys Mol Biol. 2018;132:23-34. [PubMed] | [CrossRef] | [Google Scholar]
- Rigo E, Polloni AE, Remonatto D, Arbter F, Menoncin S, Oliveira JV, et al. Esterification activity of novel fungal and yeast lipases. Appl Biochem Biotechnol. 2010;162(7):1881-8. [PubMed] | [CrossRef] | [Google Scholar]
- Priji P, Sajith S, Faisal PA, Benjamin S. Pseudomonas sp. BUP6 produces a thermotolerant alkaline lipase with trans-esterification efficiency in producing biodiesel. 3 Biotech. 2017;7(6):369 [PubMed] | [CrossRef] | [Google Scholar]
- Priyanka P, Kinsella G, Henehan GT, Ryan BJ. Isolation, purification and characterization of a novel solvent stable lipase from Pseudomonas reinekei. Protein Expr Purif. 2019;153:121-30. [PubMed] | [CrossRef] | [Google Scholar]
- Turati DFM, Almeida AF, Terrone CC, Nascimento JMF, Terrasan CRF, Fernandez-Lorente G, et al. Thermotolerant lipase from Penicillium sp. section Gracilenta CBMAI 1583: effect of carbon sources on enzyme production, biochemical properties of crude and purified enzyme and substrate specificity. Biocatal Agric Biotechnol. 2019;17:15-24. [CrossRef] | [Google Scholar]
- Verma ML, Azmi W, Kanwar SS. Microbial lipases: at the interface of aqueous and non-aqueous media: a review. Acta Microbiol Immunol Hung. 2008;55(3):265-94. [PubMed] | [CrossRef] | [Google Scholar]
- Adetunji AI, Olaniran AO. Production strategies and biotechnological relevance of microbial lipases: a review. Braz J Microbiol. 2021;52(3):1257-69. [PubMed] | [CrossRef] | [Google Scholar]
- Amenaghawon AN, Orukpe PI, Nwanbi-Victor J, Okedi MO, Aburime EI. Enhanced lipase production from a ternary substrate mix of agricultural residues: A case of optimization of microbial inducers and global sensitivity analysis. Bioresour Technol Rep. 2022;17:101000 [CrossRef] | [Google Scholar]
- Bharathi D, Rajalakshmi G. Microbial lipases: an overview of screening, production and purification. Biocatal Agric Biotechnol. 2019;22:101368 [CrossRef] | [Google Scholar]
- Patel N, Rai D, Shivam SS, Mishra U. Lipases: sources, production, purification, and applications. Recent Pat Biotechnol. 2018;13(1):45-56. [CrossRef] | [Google Scholar]
- Guan C, Tao Z, Wang L, Zhao R, Chen X, Huang X, et al. Isolation of novel Lactobacillus with lipolytic activity from the vinasse and their preliminary potential using as probiotics. AMB Express. 2020;10(1):91 [PubMed] | [CrossRef] | [Google Scholar]
- Gupta R, Rathi P, Bradoo S. Lipase mediated upgradation of dietary fats and oils. Crit Rev Food Sci Nutr. 2003;43(6):635-44. [PubMed] | [CrossRef] | [Google Scholar]
- Dab A, Hasnaoui I, Mechri S, Allala F, Bouacem K, Noiriel A, et al. Biochemical characterization of an alkaline and detergent-stable Lipase from Fusarium annulatum Bugnicourt strain CBS associated with olive tree dieback. PLOS ONE. 2023;18(5) [PubMed] | [CrossRef] | [Google Scholar]
- Remonatto D, Miotti RH, Monti R, Bassan JC, de Paula AV. Applications of immobilized lipases in enzymatic reactors: a review. Process Biochem. 2022;114:1-20. [CrossRef] | [Google Scholar]
- Ali S, Khan SA, Hamayun M, Lee IJ. The recent advances in the utility of microbial lipases: a review. Microorganisms. 2023;11(2):510 [PubMed] | [CrossRef] | [Google Scholar]
- Kumar A, Verma V, Dubey VK, Srivastava A, Garg SK, Singh VP, et al. Industrial applications of fungal lipases: a review. Front Microbiol. 2023;14:1142536 [PubMed] | [CrossRef] | [Google Scholar]
- Suzuki M, Tachibana Y. Kasuya K ichi. Array. 2020;53(1):47-66. [PubMed] | [CrossRef] | [Google Scholar]
- Ranganadhareddy A, Chandrasekhar C. Production of polyhydroxybutyrate from marine source-A Review. Indian J Ecol. 2021;48(6):1829-36. [PubMed] | [CrossRef] | [Google Scholar]
- Aluru RR, Koyi S, Nalluru S, Chanda C. Production of biopolymer from bacteria–A Review. Environ Earth Sci Res J. 2021;8(2):91-6. [CrossRef] | [Google Scholar]
- Huang Z, Guo Z, Xie D, Cao Z, Chen L, Wang H, et al. Rhizomucor miehei lipase-catalysed synthesis of cocoa butter equivalent from palm mid-fraction and stearic acid: characteristics and feasibility as cocoa butter alternative. Food Chem. 2021;343:128407 [PubMed] | [CrossRef] | [Google Scholar]
- Ghazani SM, Marangoni AG. Facile lipase-catalyzed synthesis of a chocolate fat mimetic. Sci Rep. 2018;8(1):15271 Available from: /pmc/articles/PMC6189139 [PubMed] | [CrossRef] | [Google Scholar]