ABSTRACT
Dental Pulp Stem Cells (DPSCs) offer great potential in regenerative medicine. Odontoblasts are naturally occurring cells that are responsible for constructing dentin for restorative dental purposes. Yet, their potential for use in the restoration of non-dental tissues is also being explored. Because of their similarities to BMSCs, DPSCs may be useful in musculoskeletal regenerative medicine, and they may be easily separated from teeth that have been pulled. Information on stem cells in dental pulp, transplantation, and immunomodulation was culled from the databases Scopus, PubMed, Web of Science, Science Direct, and Google Scholar. Differentiated Pluripotent Stem Cells (DPSCs) are not derived from bone marrow like BMSCs are. These phenotypic and genetic distinctions from BMSCs are being used in neurological and other settings. Pulp tissue regeneration and the therapeutic potential of DPSCs were discussed in this article. Prior to discussing their extensive uses in regenerative medicine, this article provides a brief background on the discovery and function of DPSCs.
INTRODUCTION
In severe situations, dental caries destroys the teeth’s hard structure and produces pulp necrosis. Pulp necrosis treatment often entails removing the compromised pulp tissue inside the tooth. Dental Pulp Stem Cells (DPSCs) are useful for repairing damaged pulp tissue because they are multipotent, proliferate quickly, and can survive cryopreservation.1,2 The impacted third molar pulp contains a rare group of cells called DPSCs. DPSCs are non-immunogenic and immunosuppressive, therefore they do not produce an allogeneic immune response.3 DPSCs may also be used for allogeneic transplantation and pulp regeneration. Regenerative medicine and tissue engineering based on stem cells have the potential to repair extensive damage to many tissues and organs.4 In translational medicine, adult stem cells are very useful. Adult human third molars contain DPSCs. DPSCs have been isolated and described by many groups.5 DPSCs can self-renew, rapidly proliferate, form colonies, differentiate into several lineages, and express pluripotent genes. Yet, DPSC subpopulations exhibit genotypic and phenotypic heterogeneities that may affect their therapeutic potential.6
Gronthos et al.7 was the first to isolate and describe DPSCs. The disposable dental pulp is reprocessed into DPSCs after occlusion treatment, which have a higher rate of regeneration than other adult stem cells.8 The tools they use in their isolation approach are not invasive, and there are no major moral considerations involved. Stemness, viability, proliferation, and the ability to differentiate are not affected by cryopreservation in DPSCs.9 Hence, DPSCs may be a useful source of personalised therapy. In this article, we will discuss the current level of research into the origins, biological properties, heterogenicity, differentiation, immunomodulatory potentials, paracrine effects, and pre-clinical and clinical uses of adult human DPSCs.
The current prevalence of total caries among American children and adolescents is 45.8%, according to epidemiological data.10 Early caries symptoms are nonexistent because the carious disease has not yet reached the dentin. As dental caries worsens, it causes an inflammatory response in the pulp, leading to excruciating pain and tissue ischemia.11 Currently, the accepted method of treatment is cleaning the affected area, removing the infected dental pulp tissue, and filling the tooth with an artificial material.12 Whereas the long-term effects of Root Canal Therapy (RCT) are mostly positive and predictable, reinfection is always a possibility.13 Even if the procedure is effective, the pulp will lose its vitality and its ability to sense and respond to its environment, weakening the teeth and diminishing their resistance to damage from outside sources.14
In addition, the success rate drops when retreatment is necessary due to reinfection, and repeated RCT weakens the teeth, increasing their susceptibility to root fracture.15 Studies have found MSCs in a wide variety of tissues, including tooth tissues, bone marrow and adipose tissue.16 Due to their favourable features, MSCs have been identified as a possible stem cell source for application in regenerative medicine.17 MSC-like cells are easy to isolate from dental tissues such the ones found around removed teeth and in the periodontal tissues that surround them.7
Cryopreserving cells has additional benefits since they can develop into neurons and blood vessels, two key components of pulp tissue.18 DPSCs have gained importance as a stem cell source for pulp tissue regeneration as a result of their ability to mend injured pulp tissue and their high concentration in the primary dental pulp.19 Thus, we studied DPSCs and role of immunosuppressive properties. Modern technology for pulp tissue regeneration and the therapeutic applications and methods of DPSCs have also been studied.
Dental caries/Pulpitis
A worldwide epidemic, dental caries causes extensive tooth decay but shows no symptoms until it has already advanced greatly. The prevalence and incidence of dental caries have stayed relatively constant throughout time, with the majority of instances occurring in untreated permanent teeth.20 Because of the interplay between the bacteria in the mouth, the sugars in the diet, and the pH of the mouth and teeth, dental caries is a complex disease.21 These interactions lead to the formation of a biofilm in which bacteria thrive and from which acidogenic byproducts of bacterial metabolism demineralize the enamel, the hardest component of the tooth. Cavitation and additional demineralization of the dentin follow as a result of the exposure of the dentin to micro-organisms during the process of enamel demineralization.22 Untreated caries eventually develops into deep caries, which destroy the entire dentin layer and cause particular pulp exposure.
Directly responding to tooth caries, dental pulp cells express a wide range of immune response.23 Once recruited, these stem cells play a direct role in pulp repair.24 Furthermore, bone marrow fibrocytes contribute in healing by migrating to the site of the wounded pulp.25 In reparative dentinogenesis, DPSCs are among the progenitor cell types that migrate to the pulp region of a tooth that has been damaged. These DPSCs then undergo differentiation into odontoblast-like cells.26 Pulpal repair requires a regenerative response, which is triggered by low-grade inflammation. Pulp necrosis and abscesses can develop if inflammation does not address, even after multiple attempts at repair have failed.27
Figure 1 shows schematic representation of pulp therapy. In dental restorations, pulp capping is used to protect the dental pulp from necrosis when it has been exposed or almost exposed during a cavity preparation, as a result of a traumatic injury, or as a result of a deep cavity that has reached the Centre of the tooth. In Pulpotomy when there is pulpitis but no root pathology, the dentist may recommend a pulpotomy as a minimally invasive treatment option. In pulpectomy when pulp tissue has become irreversibly diseased or necrotic, a root canal procedure called a pulpectomy must be performed. When the pulp is inflamed, the dentist will perform pulp capping or pulpotomy on the pulp itself. Direct pulp capping using dental biomaterial can be performed to protect the exposed pulp and promote dentin repair. Hence, protecting the pulp tissue from harmful stimuli like bacteria is the primary objective of pulp capping. Hence, no pulp tissue removal is required for pulp capping. In contrast, a pulpotomy calls for the removal of a small, visible portion (about 2–3 mm) of infected pulp tissue.28 Pulpotomy is commonly used to treat irreversible pulpitis because histological studies have indicated that the coronal pulp is inflamed in these patients, while the pulp tissue in the root chamber is rarely inflamed.29
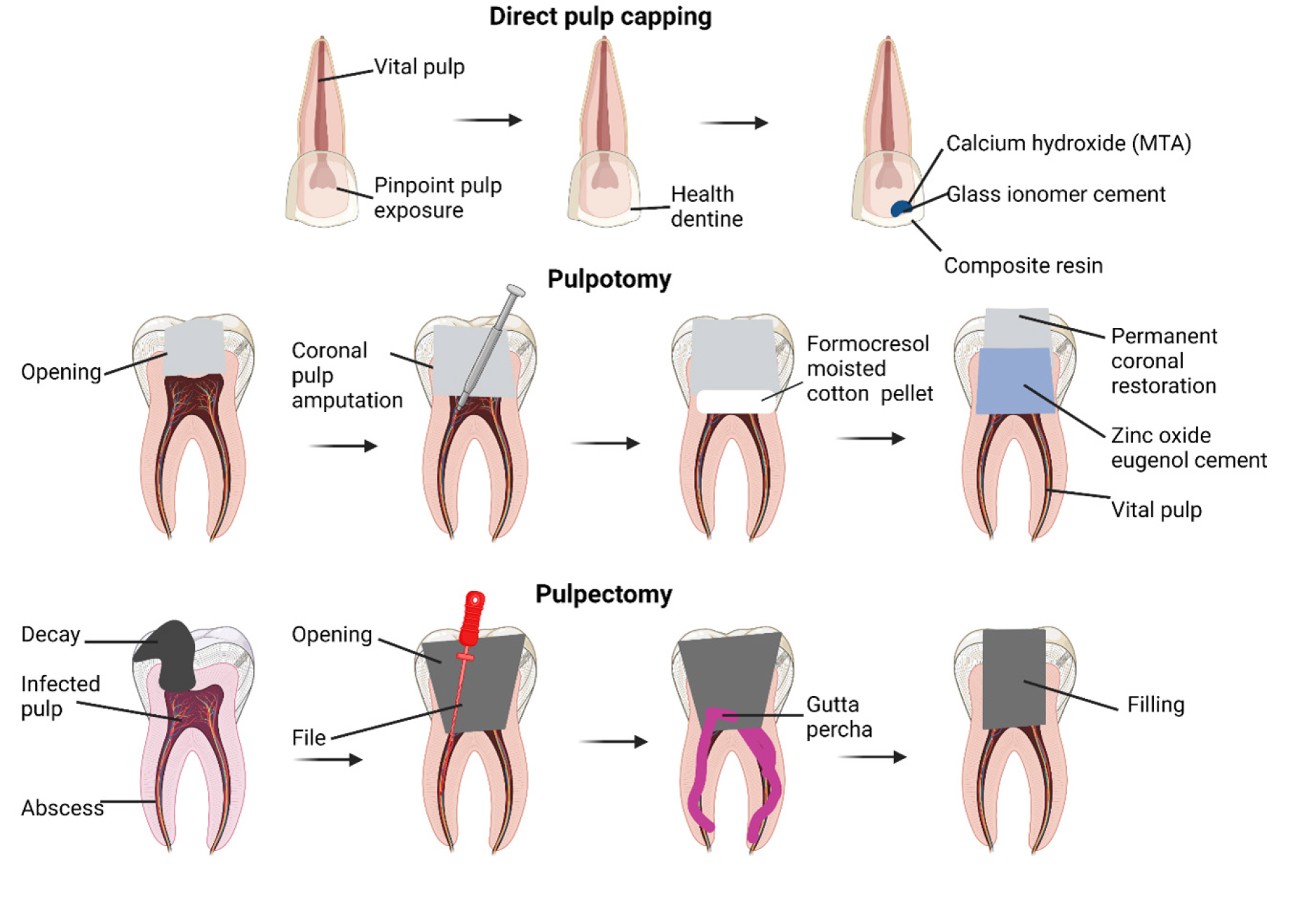
Figure 1:
Schematic representation of the modern idea of pulp therapy.
Apexogenesis is aided by a procedure called pulp chamber pulpotomy, which is often performed on growing teeth. Nevertheless, there is some evidence to suggest that it may also have long-term benefits for fully developed teeth.30 However, if the bacterial infection and inflammatory reaction in the pulp are not treated, the pressure inside the pulp chamber will rise dramatically, leading to ischemia of the pulp tissue and agonizing pain. When the patient was in pain, the dentist recommended a pulpectomy to remove the pulp from the pulp chamber.
Origin of dental stem cells
The neuro-mesenchymal components of the tooth pulp are responsible for the adaptability and versatility of oral stem cells. The genetic studies of lineage links have revealed that the perivascular pericytes and the glial cells are the direct progenitors of dental stem cells. Rapid proliferation of NG+ pericytes and their involvement in the formation of new odontoblasts following damage.31 To maintain homeostasis and impair incisor mesenchymal repair, Zhao et al.32 discovered that activation of periarterial Gli1+ cells by neurovascular sensory cells was adequate. All NG+ cells in pericytes were found to have descended from Gli1+ cells, but not the other way around, as was found in lineage tracing studies.33 It is commonly acknowledged that periarterial Gli1+ cells are the only origin of odontoblasts, as no other cell type has been linked as a precursor to odontoblasts. Pericytes and glial cells were found to have equivalent roles in tooth organogenesis, homeostasis, and growth when a clonal color-coding approach was combined with tracking of peripheral glial cells.33,34
The potential tissue-repair and growth-promoting functions of dental pulp stem cells
Stem cells isolated from tooth pulp hold promise for use in cell therapy and could play a role in the future of regenerative medicine. Despite the fact that our knowledge of DPSCs and their strategies for tissue healing is still in its infancy, their ability to respond to their surroundings has made them a valuable resource in the fight against disease. Here, in the section below we will discuss different cells and their ability to produce growth factors, both of which may play a part in their potential function in tissue repair.
Mesenchymal stem cells
MSCs are essential for organ development and repair due to their ability to self-renew and further differentiate.35 MSCs have been at the forefront of regenerative medicine due to their amazing potential to fix damaged tissues, including bones and teeth, in a number of studies using animal models and clinical trials.36,37 MSCs have become more popular as a cell source because of their ability to differentiate into osteoblasts and odontoblasts, their cryopreservation, their modulation of systemic immunity, and the lack of ethical concerns associated with harvesting them.36,38 In addition, numerous regenerative medicine studies can benefit from MSCs due to their efficient in vitro expansion. Cell surface markers CD73, CD90, and CD105 are sufficient to distinguish Mesenchymal Stem Cells (MSCs) from hematopoietic cells (which can also be recognized using markers such as CD11b, CD34, and CD45).39
Although bone marrow and adipose tissue are the most typical places to find human Mesenchymal Stem Cells (MSCs), other tissues can also be mined for MSCs.40 Bone marrow Mesenchymal Stem Cells (MSCs) have been the focus of numerous bone regeneration studies due to their essential role in maintaining bone homeostasis through regulation of osteoblast development and osteoclast activity.41–43 Using bone marrow-derived MSCs to treat osteopenia and bone abnormalities through cryotherapy or tissue engineering has shown therapeutic promise with respect to bone homeostasis.44–47 Because bone marrow is a renewable resource, it is ethically acceptable to collect MSCs from the iliac crest without restricting access to the area. However, reaching to bone marrow involves conscious sedation and anesthesia, both of which need to be closely monitored by an anesthesiologist.
There are MSCs with classic MSC characteristics in dental tissues, such as those left behind after teeth extraction and the adhering tissues.7 Although both wisdom teeth and healthy teeth extracted for orthodontic procedures can be a source of MSCs, there is a larger quantity of MSCs isolated from dental tissues than bone marrow MSCs. The percentage of orthodontic patients who had all four primary molars pulled ranges from 8.9 percent to 13.5 percent, according to studies. To wit:48 It is common practise to remove asymptomatic third molars before beginning orthodontic treatment.49 When this is taken into consideration, it becomes clear that MSCs isolated from dental-related tissues are more readily available than MSCs isolated from bone marrow. Periodontal ligament stem cells, apical papilla stem cells, dental follicle cells, stem cells from human exfoliated deciduous teeth, and so on are all examples of Dental-Derived Mesenchymal Stem Cells.
Periodontal Ligament Stem Cells
Fibrous connective tissue called the periodontal ligament anchors teeth to the alveolar bone.50 Stem cells extracted from the periodontal ligament have been shown to aid in tissue repair and maintenance in a variety of different research settings.51 Extraction of stem cells from periodontal ligaments revealed in vitro that these cells exhibited markers characteristic of cementoblasts and osteoblasts and calcified when cultivated. Evidence that stem cells isolated from transplanted periodontal ligaments move to and repair tissue abnormalities provides hope for periodontal tissue regeneration.52
Stem Cells from the Apical Papilla
As apical papilla tissue only occurs during tooth root development and then dies off, it is only seen at the very tip of a developing tooth root. Some research suggests that stem cells isolated from the apical papilla can differentiate into cementum and periodontal ligament-like complexes in vivo, and that they can also differentiate into odontoblasts and osteoblasts in vitro.
Dental Follicle Cells
A dental follicle, composed of loose connective tissue generated from the ectomesenchyme, encloses a tooth’s papilla and enamel before eruption. Both in vitro and in vivo studies have revealed that cells derived from dental follicles (which are thought to be progenitors of cementoblasts, periodontal ligament cells, and osteoblasts) can develop into cementum (with the help of implants). When transplanted in vivo, cells from dental follicles produce tissues similar to periodontal ligaments via epithelial-mesenchymal interactions. In regenerative medicine, dental follicle cells stand out because they can be sub-cultured repeatedly without losing their MSC-like properties and because of the periodontal tissues they can generate.
Stem Cells from Human Exfoliated Deciduous Teeth
Stem cells with MSC-like abilities were isolated from the pulp tissue of human deciduous teeth after they had died. It has been shown that stem cells isolated from exfoliated human deciduous teeth can differentiate into osteoblasts in vitro and dentin-like tissues upon transplantation in vivo,53 indicating that they may be useful for pulp regeneration.
Stem cells were isolated from exfoliated human deciduous teeth, and these cells demonstrated superior osteogenic differentiation capacity, osteo-inductive aptitude, and proliferation compared to DPSCs and bone marrow-derived MSCs.54,55 An appealing cell source for bone and tooth regeneration following cryopreservation is the exfoliated human deciduous teeth, which include stem cells with the ability to develop into other cell types.
Dental Pulp Stem Cells
Dental Pulp Stem Cells (DPSCs) are a type of Mesenchymal Stem Cell (MSC) that assists in oral tissue repair and regeneration.7 Dentin repair and postnatal tooth homeostasis rely on odontoblasts, which originate from Dentin Pulp Stem Cells (DPSCs). Due to their neural origin, DPSCs can undergo neuronal and glial cell differentiation. They can also secrete neurotrophic substances that encourage neurite development and protect neurons.56 Under the right conditions, DPSCs can release angiogenesis regulators and form new blood vessels.56 Figure 2 shows for dental pulp regeneration, DPSCs are the best cell source due to their enhanced neurodifferentiation and angiogenic ability.
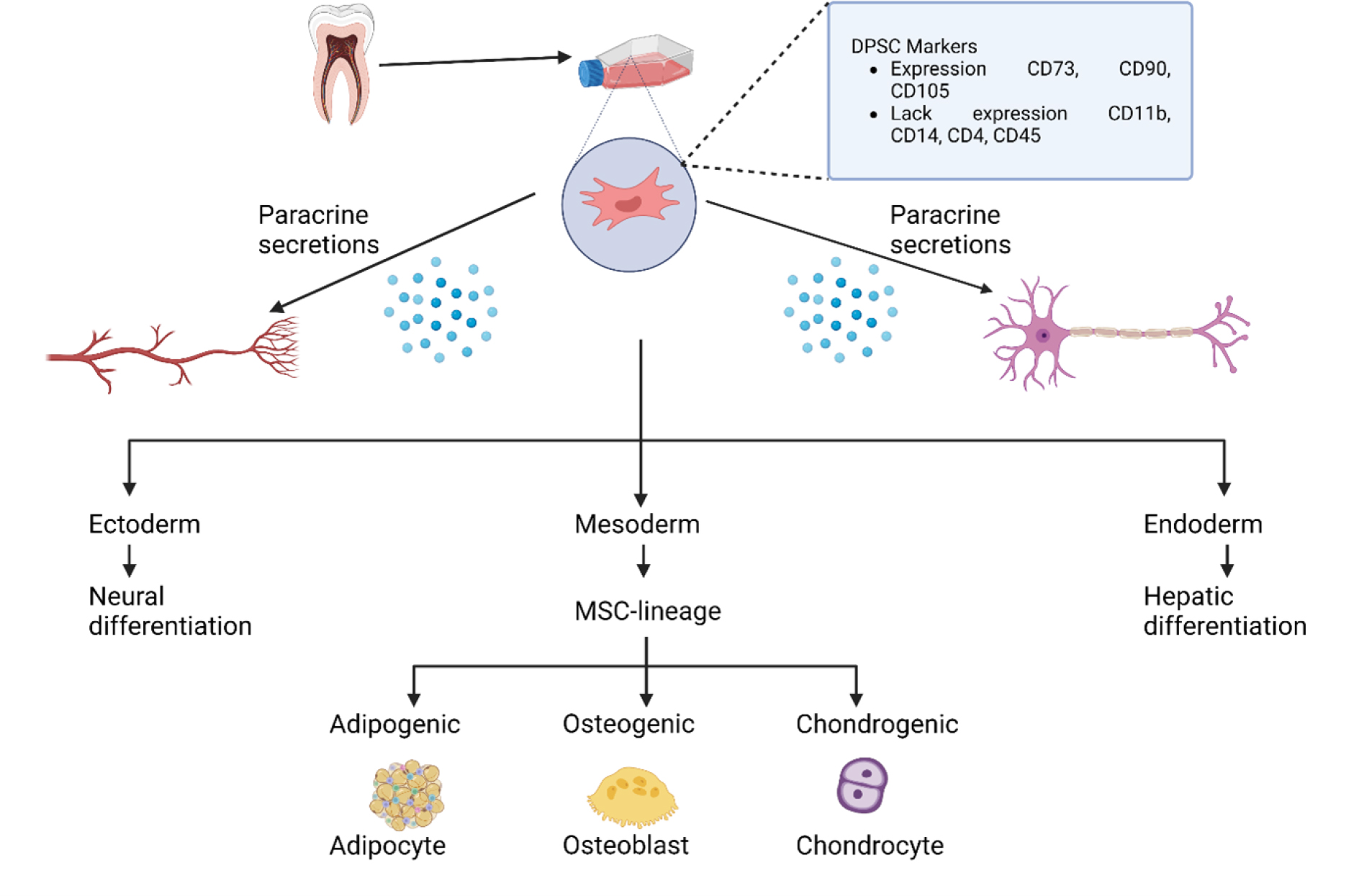
Figure 2:
The tooth pulp’s cellular makeup is based on DPSCs.
Dental pulp stem cells as a source of pulp regeneration
The apical foramen supplies blood vessels and nerves to the pulp tissue, which keeps it alive. MSCs’ robust angiogenic and neurogenic capacity is necessary for pulp tissue regeneration. Consequently, 1) the regenerated pulp tissue must have the same cell density and structure as the original pulp, and 2) the rate at which new dentin is developed must be predictable, 3) have attached blood vessels, and 4) have innervated nerves. To regenerate pulp tissue, DPSCs may be the best option due to their unique neurovascular differentiation properties. Regenerating pulp effectively requires an environment that is highly similar to the pulp’s natural one, which promotes homeostasis through constant change. Therefore, DPSCs near the neurovascular bundle might be ideal for pulp tissue repair. Thus, we zeroed in on DPSCs to provide a concise summary of their stemness, therapeutic utility, immunomodulatory activities, and cryopreservation.
Stemness
DPSCs are mesenchymal stem cells that originate in the ectoderm of the skull. Colony formation and other MSC-like behaviors are shared by DPSCs, which have a structure similar to fibroblasts and can adhere to and develop on plastic surfaces. CD73, CD90, CD105, and STRO-1 are all markers that DPSCs express in the same way that MSCs do, however DPSCs lack haematological markers such CD11b and CD19.57 Despite the fact that DPSCs express a large number of markers, they are a heterogeneous group.
The delivery of oxygen, nutrients, and waste products is crucial to the success of tissue engineering, which is why a quick vascular connection to the human circulatory system is essential. Whereas endothelial markers like von Willebrand factor and CD31 are upregulated by DPSCs during angiogenesis, pericyte markers like NG2, PDGFR, and Smooth Muscle Actin (SMA) are substantially upregulated. It has been observed that DPSCs not only promote angiogenesis and vasculogenesis through the secretion of pro-angiogenic factors, but also by direct differentiation into pericytes and endothelial cells. DPSCs secrete a wide variety of angiogenic molecules, including Vascular Endothelial Growth Factor (VEGF), Platelet-Derived Growth Factor (PDGF), monocyte chemoattractant protein-1 (MCP-1), basic Fibroblast Growth Factor (bFGF), and Endothelin-1 (EDN1). These substances allow DPSCs to stabilize blood arteries and establish their angiogenic potential by acting like pericyte-like cells.
Combining endothelial progenitor cells with perivascular cells allows for the formation of functional micro vessels in vivo. Human Umbilical Vein Endothelial Cells (HUVECs) can develop a vasculature-like structure, and additional treatment of DPSCs can solidify this structure and extend the HUVECs’ lifespan. DPSCs demonstrated perivascular features that led to angiogenesis when coinjected with HUVECs.58 Hence, it is well-established that DPSCs frequently assume the position and function of pericytes and are thus tightly connected with blood vessels.
The neural progenitor marker nest in and the Glial Fibrillary Acidic Protein (GFAP) are both expressed by DPSCs isolated from the cranial neural crest, showing that these cells also exhibit neural characteristics.59 DPSCs show Neuronal Nuclei (NeuN), which are neuron-specific markers demonstrating neuronal differentiation capacity under neuronal induction circumstances, after differentiation into neural cells or glial cells. Cells produced from DPSCs have been shown to differentiate into neurons when cultured in neural inductive media containing growth factors such as Glial Cell Line-Derived Neurotrophic Factor (GDNF) and Brain-Derived Neurotrophic Factor (BDNF).60 To further enhance DPSCs and encourage their development into neuron-like cells, optogenetic activation has been found to be particularly effective in recent years.61
It has been revealed that DPSCs, like other stem cells, comprise a subpopulation of cells called the Side Population (SP) that expresses a lower concentration of the DNA-binding fluorescent dye Hoechst33342. The CD31 /CD146 population is thought to be a healthy SP population due to its strong expression of neurotrophic proteins like BDNF and Nerve Growth Factor (NGF) and angiotrophic factors like VEGF-A. Hence, DPSCs have the cell features necessary for angiogenesis and neurogenesis, two processes essential to the regeneration of pulp tissue.
Immunomodulation Properties
Although autologous DPSCs are a promising cell source for cell-based regenerative therapy, they are limited by the need for prompt extraction of uninfected teeth, cryopreservation, and utilization. Using allogeneic cells, on the other hand, offers the advantage that a cell bank can be established and put to good use whenever necessary for patients in need, once clinically relevant DPSCs have been collected. Yet, a Major Histocompatibility Complex (MHC) mismatch might lead to host immunological rejection when allogeneic cells are used. The future use of DPSCs in regenerative medicine as an alternative to allogeneic cells is supported by their potent immunomodulatory actions.
Co-culturing cells in a petri dish has been the primary method used to prove DPSCs’ immunosuppressive effects in the lab. There is in vitro evidence that DPSCs can suppress immune responses by promoting the formation and proliferation of regulatory T cells (Tregs) in response to T cell activation.62–64 Photograph Three. Drew the conclusion that DPSCs inhibited the growth of Th1 and Th2 CD4+ T cells while concurrently fostering the development of Treg and Th17 cells. In contrast, we found that DPSCs’ immunosuppressive abilities are unrelated to Tregs. During T cell activation, IFN-α is secreted, priming DPSCs to produce TFG-α and engaging in immunosuppressive activity, as we previously documented.18
In addition, the ability of DPSCs to induce apoptosis in T cells via the Fas apoptotic pathway has been shown to contribute to their immunosuppressive impact. Because of their interactions with T cells, macrophages, and Natural Killer (NK) cells, DPSCs are an important part of immune responses. Results from a study in which DPSCs were transplanted into one side of the body’s hindlimb skeletal muscle suggested that the cells might interact with resident macrophages to encourage polarization towards the anti-inflammatory M2 phenotype.65 The ability of NK cells to lyse both autologous and allogeneic MSCs implanted for cell therapy is a major reason for their widespread use.
DPSCs can enhance their in vivo lifespan by overexpressing Hypoxiainducible Factor 1 alpha (HIF-1α), which makes them more resistant to NK cell lysis.66 Inflammatory factors are modulated by DPSCs, which downregulate pro-inflammatory factors like TNF-α and upregulate anti-inflammatory ones like TGF-β.63 DPSCs express immunosuppressive and anti-inflammatory TGF-β. DPSCs emit TGF-β and decrease the immunological response to nerve injury, enabling nerve repair and regeneration.67 DPSCs regulate immune cell proliferation, cytokine generation, and inflammation, making them ideal for allogeneic transplantation.
The microenvironment greatly influences DPSC immunomodulation, which is rarely seen in steady-state quiescent DPSCs. TNF-α and IFN- from activated immune cells promote MSCs. Primed MSCs increase immunosuppression. Our group found that DPSCs treated with IFN- or an antibody to neutralize it lost their immunosuppressive effect, emphasizing the importance of the microenvironment.18
DPSC immunosuppression in vivo needs further study. However, a recent preclinical study of allogeneic DPSC transplantation after pulpectomy of canine incisors shows immunosuppressive ability even in vivo due to the lack of deleterious effects after transplantation of mismatched DPSCs.68 A recent publication also lends credence to DPSCs’ potent immunomodulatory potential by showing that their immunomodulatory activity remains unchanged even as the cells undergo osteogenic differentiation.69
Cryopreservation
However, although showing regenerative activity for therapeutic applications, DPSCs isolated from the teeth of elderly people or individuals with systemic illnesses including systemic lupus erythematosus, rheumatoid arthritis, or diabetes have been demonstrated to have diminished bioactivity.70 Pulp tissue loss from physiological secondary dentinogenesis and pathological tertiary dentinogenesis, as well as mineralization (such as pulpal stone), making it challenging for the elderly to obtain DPSCs. The pulp of healthy, young children’s teeth is likewise scarce and in high demand. Hence, cell banking is a crucial resource for storing DPSCs in a way that does not compromise their therapeutic potential, and for using them in the case of a clinical necessity through rapid cell development. Cryopreservation is the practice of keeping cells at temperatures so low that they are frozen solid and no biological reactions can take place.
Cryoinjury can occur when cells are frozen and then thawed, thus efforts to find ways to protect them during cryopreservation have been ongoing for quite some time. Direct mechanical force from the production of ice crystals can cause cryoinjury, but indirect effects from shifts in osmotic homeostasis can also play a role.71 Commonly used cryoprotectants like Dimethyl Sulfoxide (DMSO) work by penetrating the cell membrane to stop ice crystal nuclei from forming and causing harm. However, the dose of DMSO employed for cell preservation must be kept low because DMSO itself has harmful effects on cells (causes cytotoxic effects). It is generally agreed that a concentration of 10% or less is only mildly harmful, and studies are currently undertaken to determine the optimum DMSO content for sustaining DPSCs.
Stemness and multipotency of DPSCs must be maintained during cryopreservation to ensure their usefulness in regenerative medicine. Several studies have demonstrated that DPSCs maintain their stem cell characteristics during cryopreservation. A recent study demonstrated that DPSCs’ survival, proliferation, stemness, and differentiation potential were not affected by cryopreservation at 80°C for a complete year.72 Despite these benefits, a sufficient number of DPSCs must first be identified and cultivated from pulp tissue after tooth extraction before they can be cryopreserved. Cryopreservation with this method is labor- and resource-intensive, and it carries the danger of microbial contamination. As a result, research is expanding from preserving cells to preserving entire tissues in liquid nitrogen. Cell proliferation rates, cell growth morphology, and stem cell qualities were not affected by culturing pulp tissue that had been frozen for over a year. To wit:73
Pulp tissue cryopreserved in 5% DMSO was shown to have much faster cellular expansion compared to pulp tissue cryopreserved in 10% DMSO under the same circumstances.74 When compared to cell cryopreservation, tissue cryopreservation may be preferred because it promotes cell viability in three distinct ways: by shortening the period until cryopreservation, by minimizing the chance of contamination, and by lessening the direct destructive effects of DMSO on cells. Cryopreserving the tissue scaffold for tooth pulp regeneration has the potential to be a useful technique.75–77 Long-term cryopreservation and the immunosuppressive qualities of DPSCs may make allogeneic pulp tissue transplantation a reality.
Therapeutic Potential of Dental Pulp Stem Cells Related to Neurovascular Properties
Interest in DPSCs as a treatment for a variety of systemic illnesses has been inspired by their angiogenic and neurogenic capabilities. Increased numbers of catecholaminergic nerve fibers in the iris following grafting of rat pulp tissue into the anterior chamber demonstrates the neurodifferentiation potential of DPSCs. The same study also demonstrated that neurotrophic substances derived from dental pulp saved motoneurons, implying that pulp tissue transplantation into hemisected spinal cords is responsible for this effect. The use of neurotrophic factors obtained from tooth pulp to treat Parkinson’s disease has been supported by scientific research. These factors are able to shield dopaminergic neurons from in vitro toxicity caused by MPP+ or rotenone.78
Neurotrophic effects of DPSCs in AD and PD have also been demonstrated.79 Examples of neurotrophic factors produced by dental pulp cells include Nerve Growth Factor (NGF), Glial Cell Line-Derived Neurotrophic Factor (BDNF), and Bone Morphogenetic Protein (BMP)-2. Because of this, individuals may respond well to cell-based therapy. Several animal models of disease have also been used to examine the angiogenic potential of DPSCs. Functional revascularization in a mouse model of hind limb ischemia was enhanced by pig pulp-derived DPSCs with CD31 CD146 SP. By generating angiogenesis and supporting the migration and differentiation of endogenous neural progenitors, the SP of DPSCs mitigates ischemic brain injury caused by blockage of the middle cerebral artery.
Human DPSCs were discovered to have angiogenic potential when they were employed to reduce cardiac infarction in rats with acute myocardial infarction. The angiogenic potential of engrafted DPSCs was demonstrated by their ability to ameliorate histology in a model of muscular dystrophy by increasing angiogenesis.80 Pluripotent-like stem cells obtained from human dental pulp engrafted into skeletal muscle of a mouse model of muscular dystrophy formed new muscle fibres and blood vessels. These cells secreted growth factors involved in angiogenesis.81
The classical concept of pulp tissue engineering
Conventional RCT for infected dental pulp entails removing the pulp from the tooth and replacing it with a synthetic inorganic substance. After undergoing root canal therapy, teeth lose their vitality and become brittle, increasing their susceptibility to fracture. So, the greatest solution for these issues is a pulp that is both healthy and functional. In an effort to preserve tooth health, scientists are currently employing tissue engineering and regenerative medicine to rebuild pulp tissue. For dental pulp regeneration to be successful, the same three components used in other regenerative medicine applications—stem cells, scaffolds, and signaling molecules—must be present (growth factors). The basic idea is to locate stem cells, multiply them in the lab, load them onto scaffolds, and then use them in vivo with signaling molecules to direct their development into the desired cell types.
Apart from its primary job of providing structural support for stem cells, the scaffold can also play a role in recruiting cells or encouraging differentiation into specific cells through the addition of growth factors or drugs. Scaffolds, from a more traditional perspective, are useful for providing structural support, facilitating interaction with the local microenvironment, and perhaps modulating the signal route necessary for regeneration. To serve this traditional function, the scaffold must be structurally stable, encouraging stem cell attachment and development at the implantation site.
Moreover, the scaffold should have a similar extracellular matrix to the donor tissue. The ability to migrate, the proliferation rate, and the differentiation potential of DPSCs have all been shown to be improved in vitro by the addition of various exogenous growth factors. Several chemicals have shown potential as DPSC migration triggers. They consist of bFGF,82 SDF-1,83 and G-CSF (Granulocyte Colony-Stimulating Factor).82 Several growth factors, including Wnt3a,84 G-CSF, and bFGF, have been found to stimulate DPSC expansion. One such stem cell factor that has been shown to develop DPSCs into odontoblasts is Bone Morphogenetic Protein-2 (BMP-2).85
Mineralization of DPSCs was stimulated by BMP-7, although this had little effect on DPSC recruitment. In contrast, TGF- increased mineralization by converting DPSCs into odontoblast-like cells. Evidence suggests.85 Pulp regeneration relies on G-CSF because it stimulates neurogenesis, angiogenesis, and the migration, differentiation, and mineralization of DPSCs.82 The findings were published in 2015 by Takeuchi et al. Yet, extensive mechanistic investigation is required because a factor’s diverse impacts can undermine dental pulp regeneration’s intricate differentiation regulation.
CURRENT APPROACHES FOR PULP TISSUE REGENERATION
If decayed teeth are not treated, necrosis and abscesses of the pulp, accompanied by inflammatory pulp responses, develop. Attempts were made to save the sick tooth by performing randomized controlled trials in which the infected pulp tissue was removed, sterilized, and replaced with artificial material.
Pulp Revascularization
When the root canal has been cleaned and disinfected, a blood clot is injected in the canal to regenerate the diseased pulp tissue into healthy pulp tissue. This process is known as pulp revascularization. Apical foramens haven’t closed in baby teeth; hence this technique can be used on them. Back in the 1960s, dentists experimented with putting a needle into a patient’s tooth to cause a clot of blood to form in the canal and regrow the pulp tissue.86 Root growth of young teeth can be completed if revascularization is conducted effectively; thus, it has been intensively investigated in the field of traumatology.87 After years of research—including the publication of the first case report on regenerative root canal treatment—the American Dental Association officially recognized this method in 2011.
Pulp revascularization and traditional root canal treatment share a common goal—the elimination of infection—but the two procedures have fundamentally different approaches. To avoid reinfection after root canal therapy (also known as pulpectomy), an intensive disinfection process is used. Mechanical debridement with an endodontic file is not recommended during pulp revascularization because it might cause stress to the root canal walls and inhibit the migration of apical stem cells that keep the tooth healthy. The use of intracanal medicines and sufficient chemical disinfection over mechanical debridement is therefore recommended during pulp revascularization treatments.
Hence, the balance between disinfection and cytotoxicity in stem cells must be taken into account even if chemical disinfection is undertaken to protect the root canal wall. For regeneration to take place, the surrounding area needs to be both clean and conducive to stem cell attachment and differentiation. For example, the widely used chemical disinfectant sodium hypochlorite is lethal at concentrations of 3% or higher and prevents stem cells from adhering.88
Thisiswhyamildsolutionofsodiumhypochloriteisrecommended for re-vascularizing the pulp by the American Association of Endodontists. Using tissue engineering to re-vascularize a pulp that has been injured maintains loyal to the field’s founding ideals. Root tip bleeding is used to insert MSCs into the root canal.89 Because it contains so many growth factors, a blood clot can act as both a scaffold and a signaling molecule. Many growth factors are ordinarily encased in dentin, but Ethylenediaminetetraacetic Acid (EDTA) has been shown to release them, promoting DPSC development into odontoblast-like cells.
As a result, EDTA is suggested for use as a last irrigant. On the other hand, pulp revascularization has a few restrictions. This approach can only be utilized on baby teeth, as was described earlier. The majority of the tissues formed during pulp revascularization have been shown through histological studies to be different from the original pulp-like tissues and instead resemble periodontal, cementum, and bone-like tissues.90 That’s why we need additional study before we can use this method on completely formed teeth and stimulate the growth of pulp-like tissue.
Cell-Homing-Based Regenerative Endodontic Treatment
The concept of cell homing for dental regeneration revolves around the chemotaxis of host endogenous cells to injured pulp tissue via signaling molecules.91 Cell-free RET refers to RET techniques that do not employ donor cells, such as pulp revascularization and RET based on cell homing. When applied to a scaffold designed for stem cell migration and proliferation and capable of loading preferable signaling molecules, cell homing can improve the success of pulp revascularization. The blood provides a scaffold and is also a source of signaling chemicals. The cell homing method induces, differentiates, and proliferates endogenous stem cells that can regenerate pulp-dentin, hence it’s therapeutically crucial to locate endogenous cell sources.
In cases where the pulp is destroyed during root canal therapy, the stem cells are not derived from the tooth, but instead come from the patient’s own bone marrow. The pulp in the root canal of a tooth is left unharmed and continues to function normally after a pulpotomy, a dental operation in which just the pulp in the crown of the tooth is removed. While it is commonly done on baby teeth to protect the pulp and encourage good root growth, the procedure is not limited to those teeth; fully developed teeth can also benefit from pulp protection. As local DPSCs are highly homing and have the natural potential to repair pulp dentin in response to specific signaling molecules, a pulpotomy can be accompanied by a cell-homing technique.34 Nonetheless, it is common knowledge that pulpectomy results in the loss of periodontal ligament stem cells, which are located in the apical foramen, as well as apical papilla stem cells.92,93
The conventional method for investigating the efficacy of the cell homing-based strategy for repairing pulp tissue is to transplant human teeth into animal models. In a nutshell, after Root Canal Therapy (RCT) has been performed on human removed teeth, a combination of signaling molecules is applied to the empty root canals using a variety of scaffolds. Pulp regeneration is studied by transplanting the grafts into animal models. Research in the beginning concentrated mostly on chemicals that sent signals. Regeneration of pulp-like tissue in a mouse model has been reported using a combination of basic Fibroblast Growth Factor (bFGF), Platelet-Derived Growth Factor (PDGF), Neural Growth Factor (NGF), Vascular Endothelial Growth Factor (VEGF), and Bone Morphogenetic Protein 7 (BMP7) (factors for odontoblast differentiation and mineralization). Although the study by Kim et al.91 relied on an ectopic model, it is nevertheless significant since it paves the way for demonstrating that a cell-homing approach to pulp regeneration is doable in people.
Thereafter, experiments were carried out utilizing a single molecule to identify the most crucial signaling molecule for pulp regeneration. Injections of bFGF alone have been shown to regenerate pulp-like tissue, suggesting an essential role for the signaling molecule that stimulates stem cell recruitment. Another study concluded that bFGF might be used in place of G-CSF in pulp regeneration due to the two factors’ equal efficacy.82 Recent study has demonstrated the efficacy of SDF-1,83,94 and Stem Cell Factor (SCF),95 but further investigations are needed to confirm these results. As cell-homing treatments don’t require the in vitro collection and manipulation of stem cells, they could be less complicated and cheaper to execute in the clinic.
However, there are constraints associated with the cell-homing approach. Root growth in juvenile teeth may be stunted if inflammation and pulp necrosis damage the apical papilla or follicle, which houses stem cells necessary for odontoblast differentiation and dentin production. As a result, it is difficult to anticipate the outcome of treatment when cell-homing based RET is conducted on completely developed teeth, as nothing is known about the condition of stem cells in the apical papilla or tooth follicle. As a result, there are still numerous open questions that need to be addressed before clinically useful and trustworthy results can be obtained. There has been a lot of progress in cell-homing RET for dental pulp regeneration in recent years, but there is still room for improvements.
Cell-Transplantation-Based Regenerative Endodontic Treatment
Exogenous stem cells are implanted into signaling molecule- coated scaffolds for tissue repair and regeneration. (See Diagram 4D). Pulp tissue regeneration using RET was the first use of the classical principle of cell transplantation in tissue engineering, and it has since seen tremendous advancement. Using the standard framework of tissue engineering, stem cell implantation on pulp regeneration has been the subject of experimental and ongoing animal studies.96–98 Using autologous DPSCs and Gelfoam as a scaffold, researchers were able to regenerate pulp-like tissues, including dentin-like tissues and blood vessels, in immature canine permanent incisors.
In addition, canine pulp-dentin-like tissue regeneration was stimulated by autologous DPSCs coupled with platelet-rich fibrin.99 Human Dental Pulp Stem Cells (DPSCs) combined with platelet-derived growth factor successfully create pulp-like tissue in animal models when applied to the pulp area of rat teeth.100 Whole root maturation and regeneration of pulp and dentin-like tissues were observed on histology and radiography in a canine investigation using a chitosan hydrogel scaffold containing autologous DPSCs and growth factors to treat immature, necrotic permanent teeth with apical periodontitis.101 Scaffolds have been utilized in RET based on cell transplantation to promote stem cell adhesion, proliferation, and differentiation after tissue transplantation. In dogs, autologous DPSCs were applied using gelfoam, while in pigs, a nanopeptide hydrogel was employed as a scaffold.102
When human DPSCs were placed on a gelatin-based scaffold and then transplanted into minipigs, the gelatin-based scaffold showed more histological and radiological success than the fibrin-based scaffold.103 Using decellularized swine dental pulp tissue as a scaffold and adding human DPSCs resulted in the regeneration of pulp-like tissue, which was proven histologically, demonstrating the need of adopting a tissue as similar to the original tissue as practical when building a scaffold.75 Scaffold-free RET, which can build cells in 3D without the need of scaffolds, is also the subject of research. Regeneration of pulp-like tissue was achieved by combining platelet-rich fibrin with cell-sheet fragments obtained from canine DPSCs.99 After subcutaneously implanting human DPSCs into 3D cell constructs devoid of signalling molecules or scaffolds in immunodeficient rats, pulp-like tissue regeneration was also seen.104
One study showed that 3D cell sheets boosted MSC therapeutic efficacy, which suggests that cell sheets might one day replace scaffolds.105 Several animal studies have used autologous stem cells in cell-transplantation-based RET, and all clinical trials have shown positive outcomes.106 The RET approach based on cell transplantation thus looks to be the most suitable for clinical applications. In addition, the difficulty of inducing stem cells, as in cell-homing-based RET, can be circumvented by directly applying a combination of stem cells with the required properties to form pulp-like tissue.
However, autologous stem cell transplantation faces a major hurdle due to the inaccessibility of pulp tissue. Extraction of wisdom teeth or teeth for orthodontic purposes is the most morally sound way to harvest DPSCs for pulp regeneration. Wisdom teeth extractions and the completion of orthodontic therapy might be challenging for patients with permanent pulpitis. While each study used a somewhat different combination of scaffolds and signaling molecules, more investigation into the optimal combination is required. Dentin pulp tissue regeneration with cell transplantation in dentistry still has a long way to go before it becomes routine practice. The focus of future efforts should be on optimizing the regenerative milieu for pulp tissue regeneration through the use of specific signaling molecules and scaffolds.103 Table 1 shows current research on dental pulp stem cells regenerative medicine.
Researchers | Study | Method | Outcome |
---|---|---|---|
Nakashima et al., 2017107 | The clinical feasibility, safety, efficacy, and potential of autologous transplantation of mobilized dental pulp stem cells. | Following a transplant of mobilized DPSCs, the progress of five patients with irreversible pulpitis was tracked for up to 24 weeks. | Discarded teeth were used to acquire DPSCs, which were subsequently cultivated for expansion. Karyotyping was used to determine the viability of mobilized DPSCs after 9 or 10 passes. Pulp-free DPSCs were mobilized and then transplanted into teeth that had had the pulp removed along with a telocollagen-based GCSF. There were no reports of illness or poisoning. In three out of five patients, the development of functional dentin was established by cone beam computed tomography. Whole pulp regeneration in endodontics using human DPSCs was found to be safe and effective in this investigation. |
Aimetti et al., 2018108 | Regenerative therapy of profound intra-bony defects using DPSCs was the focus of a case series with a 1-year follow-up. | Eleven patients with chronic periodontitis had minimally invasive flaps placed to access isolated intra-bony defects, which were then filled with autologous DPSCs planted on collagen sponge. | The gingival margin was amazingly maintained one year after autologous DPSCs implants, with a mean gain in clinical attachment level of 4.7±1.5 mm, a residual mean probing depth (PD) of 3.2±0.9 mm, and an excellent result. Full pocket closure was achieved in 63.6% of test locations (PD 3 mm). Clinical findings were supported by radiographic examinations of 3.6 1.9 mm bone fill. |
de Almeida et al., 2014109 | Clinical evaluation of a DPSC- and collagen sponge-based bio complex for repairing Oro-Maxillofacial (OMF) bone tissue in patients after third-molar extraction. | As a result of third molar impaction on the cortical alveolar lamina, these patients experienced bilateral bone resorption of the alveolar ridge distal to the second molar. Due to this clinical condition, the bone around the extracted third tooth cannot mend, so the neighboring second molar must also be extracted. | After removing the third molars from the maxilla, the cells were grown in a collagen sponge. A collagen sponge and DPSC bio complex were used to mend the wound. After three months, the autologous DPSCs transplanted into the alveolar bone demonstrated excellent vertical development and complete repair of periodontal tissue. Autologous DPSCs show promise for regenerative medicine due to histological evidence of full bone repair 12 months after grafting. |
Ferrarotti et al., 2018110 | Randomized clinical trial. | This investigation tested the hypothesis that delivering DPSCs into intra-bony defects inside a collagen scaffold would improve periodontal regeneration’s clinical and radiographic parameters. | Twenty-nine individuals with chronic periodontitis who needed to have at least one tooth extracted were included in a random fashion. Defects were either given a test treatment (autologous DPSCs micrograft’s planted onto collagen sponge) or a placebo therapy at random. Before surgery, at 6 months post-op, and at 12 months post-op, data on clinical and radiological parameters were collected. According to the results of this study, one year after treatment, clinical indices of periodontal regeneration were greatly enhanced thanks to the use of DPSCs. |
Aimetti et al., 2014111 | A case report | The clinical and radiological efficacy of autologous DPSCs in the treatment of human non-contained intraosseous lesions was evaluated in this study. | The third molar of a patient with persistent periodontitis was extracted during surgery. Autologous DPSCs were used to regenerate the infra-bony defect of the right mandibular second premolar. The reentry process confirmed that the defect had been entirely filled with bonelike tissue at the 1-year follow-up check. |
Barbier et al., 2018112 | A randomized, controlled clinical trial with split-mouth randomization. | In a randomized controlled trial with 32 participants, autologous dental pulp stem cells (DPSCs) implanted into a collagen matrix were analyzed. | 32 post-extraction sockets were implanted with DPSCs seeded on a resorbable collagen matrix after the removal of both impacted mandibular third molars, while the same number of post-extraction sockets were implanted with collagen matrices alone as controls. CT and a cutting-edge display platform were utilized to record extraction socket density 6 months after extraction, and the results were compared to those recorded right after extraction. Despite extensive research, the authors were unable to provide evidence that autologous DPSCs mitigate the bone loss that occurs in the socket following the removal of a lower third molar. |
Hernández-Monjaraz et al., 2018113 | A case report | A clinical trial including the grafting of allogeneic DPSCs into a patient with periodontal disease is presented here in an interim case report. | Passaged and cultivated allogeneic DPSCs were grown without growth factors and plated on a lyophilized collagen-polyvinylpyrrolidone sponge. The graft site exhibited enhanced bone mineral density, tooth mobility, periodontal pocket depth, and bone defect area at the 3- and 6-month follow-ups, suggesting that DPSCs allograft was a successful treatment for periodontal disease-related bone defects. |
Allogeneic transplantation
Stem cell therapy has emerged as a promising regenerative technique in cases where pulp inflammation has caused the loss of tooth function. Autologous transplantation of a subpopulation of DPSCs (pulp-derived CD31 SP cells and pulp-derived CD105+ cells activated with SDF-1) has been found to facilitate pulp regeneration. Repair of pulp-like tissue and deposition of newly produced dentin followed transplantation of DPSCs with autologous platelet-rich fibrin.99 Collecting and cultivating DPSCs under GMP conditions and then administering them with G-CSF was found to be safe and efficacious in a preclinical trial of autologous DPSC transplantation therapy.
Transplanting autologous DPSCs appears to be safe and may efficiently induce pulp regeneration, according to results from a recent human investigation.107 Autologous DPSC transplantation has shown efficacy and potential in tissue regeneration, however there are still certain obstacles that need to be investigated. Due to the presence of wisdom teeth and other supplemental teeth, it is challenging to use this method to regenerate a single tooth. This shortcoming stands out more clearly in the elderly, as it is quite likely that they do not have any unnecessary teeth. Patients of advanced age may have functionally impaired DPSCs while having viable teeth for transplantation. As they age, DPSCs expand in size and lose their ability to proliferate and differentiate, as shown in a recent study.114
Figure 3 shows an approach to pulp regeneration by means of allogeneic DPSC transplantation is proposed. The pulp is removed from a healthy, non-infected wisdom or orthodontic extraction tooth (donor). After DPSCs have been isolated and cultured from dental pulp, they are cryopreserved for later use. Cryopreserved DPSCs or dental pulp tissues are used for DPSC expansion, if necessary, throughout the patient’s pulpectomy treatment. The pulp space is cleaned and then the proper scaffold, which contains signaling molecules and DPSCs, is placed in the pulp area.
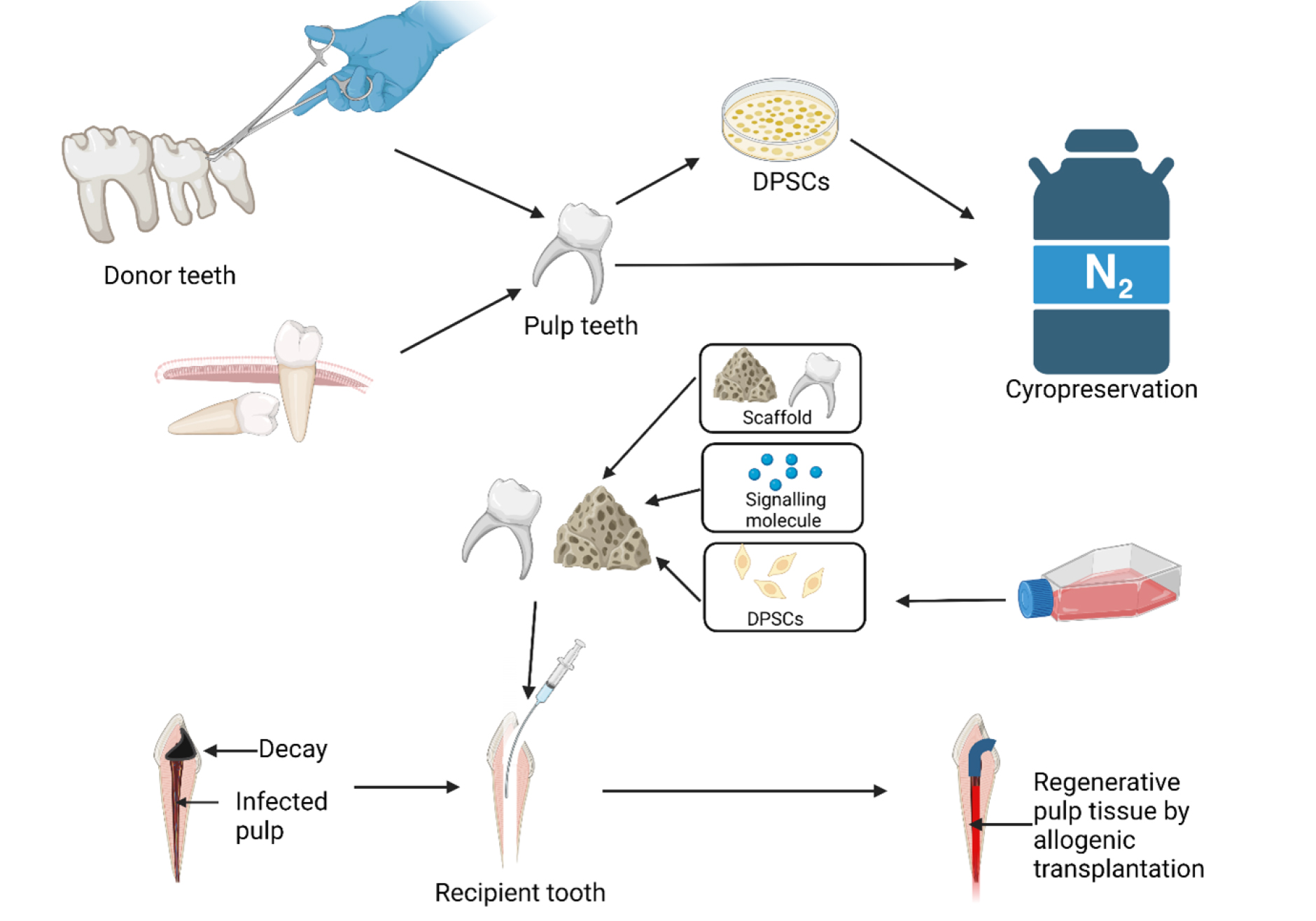
Figure 3:
An approach to pulp regeneration by means of allogeneic DPSC transplantation is proposed.
Dentin matrix acidic phosphoprotein 1 and dentin sialophosphoprotein, two essential indicators of odontogenic differentiation, are also downregulated in human DPSCs with advancing age.114,115 Pulp regeneration relies on neurogenic potential, which declines with age according to multiple studies. Yet, DPSC banking at a young age and storing them till needed is inefficient for individual patients. The expenditures of warehousing, security, and quality assurance are all quite costly. As a result, allogeneic DPSC transplantation—which involves preserving DPSCs if poor teeth are identified in young individuals and then transferring them to patients in need—is efficient, cost-effective, and improves quality control.116
Hence, in order to get around the drawbacks of autologous transplantation and carry out an allogeneic transplant, cell banks are crucial. Cryopreserving DPSCs without major cell damage has been demonstrated in a number of experiments. However, there is no evidence that cells are harmed by the process of tissue cryopreservation, which offers economic benefits due to the decreased need for costly cell isolation and incubation.73 In addition, autologous cell transplantation-based RET is predicted to incur much less financial burden if tissues can be frozen for more than a year. Pulp tissue collection, banking, and clinical application that is standardized should help save money and streamline the process. By using allogeneic cells, there is a risk of immunological rejection from the host due to an MHC mismatch.
MSCs have low immunogenicity and immunosuppressive qualities, which is why they can be used even though there are concerns about using allogeneic MSCs. As MSCs do not express class I Major Histocompatibility Complex (MHC-I) proteins and costimulatory molecules, nor do they express class II MHC-I proteins, they are not very immunogenic. Due to their lack of cytotoxic effects on host immune cells, MSCs may one day make MHC irrelevant in the context of transplantation.117 The host immune system can be conditioned to show tolerance by MSCs and DPSCs because of their low immunogenicity (Iohara et al., 2018). Moreover, DPSCs’ immunomodulatory features boost their potential as a source for allogeneic transplantation, but the mechanism is still debatable. Allogeneic transplantation may be successful despite the presence of a host immune response thanks to this immunosuppressive feature.
Allogeneic DPSC transplantation in dogs was recently reported, and it appears that this procedure relies on these features of DPSCs.68 Pulp tissue regeneration using mismatched DPSCs from an allogeneic transplantation did not show any signs of toxicity and was just as effective as using DLA-matched DPSCs. By encouraging widespread cell donor adoption, banking donated cells, and promptly delivering appropriate cells to patients, allogeneic “off-the-self “ therapies accomplish the goal of clinical stem cell-based therapy: long-term stability.118
CONCLUSION
The use of stem cells in regenerative medicine has led to the development of cell transplantation techniques for pulp regeneration. Pulp regeneration in RET has been met with more hope after the discovery and characterization of DPSCs and other dental MSCs. Many studies have demonstrated that DPSC transplantation leads to complete pulp regeneration as a result of the cells’ ability to stimulate neuroangiogenesis. Restoration of dental pulp function requires neuroangiogenesis renewal. Pulp regeneration, on the other hand, is where autologous DPSCs really shine. In order to reconstruct infected pulp, the elderly can considerably benefit from the use of allogeneic DPSCs or pulp tissue generated from cryopreserved cells or tissue banking clouds. Pulp regeneration following allogeneic transplantation necessitates optimal disinfection and scaffolds/neuroangiogenesis. Pulp regeneration using RET based on transplantation of cell-derived grafts has showed encouraging results in research. Another potential groundbreaking approach to regenerative medicine is neurovascularization-based pulp regeneration.
Future directions
It is encouraging that MSCs with self-renewal, multilineage differentiation, and immunomodulatory potentials may be isolated from tooth pulp (DPSCs). These cells have a wider range of clinical applications than Mesenchymal Stem Cells (MSCs) isolated from other sources (such as blood, fat, umbilical cord, and bone marrow). Cryopreserving DPSCs will preserve their ability to differentiate into multiple lineages, including the osteogenic, chondrogenic, dentinogenic, myogenic, neurogenic, and adipogenic lineages. Because the discarded third molar tooth is a rich source of DPSCs, and because the procurement method raises few ethical problems, it is widely used. However, this should be no surprise that research into DPSCs-based stem cell therapy techniques is at a critical level right now.
Stem cells with pluripotency capacity, signalling molecules that influence cell destiny, scaffolds that offer a suitable microenvironment, and dental pulp are all required for dentin-pulp regeneration. Taking care of diseased pulp is a challenge in modern dentistry.14,119 Current therapeutic techniques to pulp regenerative therapy for infected pulp may not be sufficient to achieve the treatment’s goal of restoring pulp functionality by reconstituting the pulp-dentin complex.120,121 Recent advances in stem cell-based regenerative medicine have made cell transplantation a viable option for pulp regeneration. Dental pulp, however, has a heterogeneous element since it contains a variety of cell types that can each give rise to distinct lineages. First, single-cell suspensions isolated from tooth pulp must be cultivated so that individual clones can be created. Cell lineage specification is achieved by isolating clones and enriching single cell types in culture, and then using immunophenotyping to characterize the subpopulations based on molecular and phenotypic markers that regulate their differentiation potential into multiple lineages in 2- or 3-dimensional culture conditions in defined media.122,123 However, there are certain drawbacks to fluorescence-activated cell sorting technologies, such as the potential for cell viability to be compromised by exposure to electric charge.
It is possible that novel cryopreservation solutions and appropriate protocols will need to be developed in cell banking for DPSCs to maintain their immunobiological characteristics and viability throughout extended use. Further study is required to elucidate the molecular processes that regulate DPSC interactions with various biomaterials. Transmission electron microscopy research may aid in characterizing distinct phenotypes of the heterogeneous progenitor cells that populate dental stem cell niches, but rigorous testing of the endothelial-mesenchymal transformation is necessary to evaluate their potential for restocking these niches. Therapeutic techniques based on cellular or secretome properties linked with DPSCs have shown encouraging outcomes in both preclinical and clinical investigations. To advance regenerative medicine and prove the safety of DPSCs-based treatments as a key therapeutic tool, more research is needed using human subjects. However, before DPSCs-based therapies may be given clinically to patients, there are still significant challenges that must be overcome.124 When taken together, these cutting-edge techniques show that DPSCS is a promising adult stem cell model that could lead to new insights and therapies for a wide range of human disorders.
References
- Kwack KH, Lee HW. Clinical potential of dental pulp stem cells in pulp regeneration: current endodontic progress and future perspectives. Front Cell Dev Biol.. 2022;10:857066 [PubMed] | [CrossRef] | [Google Scholar]
- Upadhyay A, Pillai S, Khayambashi P, Sabri H, Lee KT, Tarar M, et al. Biomimetic aspects of oral and dentofacial regeneration. Biomimetics (Basel). 2020;5(4):51 [PubMed] | [CrossRef] | [Google Scholar]
- Wen K, Li W, Cheng C, Weige X, Jiaqi C, Shiyu S, et al. Human dental pulp stem cells ameliorate the imiquimod-induced psoriasis in mice. Heliyon.. 2023;9(2):e13337 [PubMed] | [CrossRef] | [Google Scholar]
- Liu P, Zhang Y, Ma Y, Tan S, Ren B, Liu S, et al. Application of dental pulp stem cells in oral maxillofacial tissue engineering. Int J Med Sci.. 2022;19(2):310-20. [PubMed] | [CrossRef] | [Google Scholar]
- Al Madhoun A, Sindhu S, Haddad D, Atari M, Ahmad R, Al-Mulla F., et al. Dental pulp stem cells derived from adult human third molar tooth: A brief review. Front Cell Dev Biol.. 2021:2780 [PubMed] | [CrossRef] | [Google Scholar]
- Kok ZY, Alaidaroos NYA, Alraies A, Colombo JS, Davies LC, Waddington RJ, et al. Dental pulp stem cell heterogeneity: finding superior quality “needles” in a dental pulpal “haystack” for regenerative medicine-based applications. Stem Cells Int.. 2022 [PubMed] | [CrossRef] | [Google Scholar]
- Gronthos S, Mankani M, Brahim J, Robey PG, Shi S.. Postnatal human Dental Pulp Stem Cells (DPSCs) and . Proc Natl Acad Sci U S A.. 2000;97(25):13625-30. [PubMed] | [CrossRef] | [Google Scholar]
- Ziauddin SM, Nakashima M, Watanabe H, Tominaga M, Iohara K. Biological characteristics and pulp regeneration potential of stem cells from canine deciduous teeth compared with those of permanent teeth. Stem Cell Res Ther.. 2022;13(1):439 [PubMed] | [CrossRef] | [Google Scholar]
- Pilbauerova N, Soukup T, Suchankova Kleplova T, Schmidt J, Suchanek J.. The effect of cultivation passaging on the relative telomere length and proliferation capacity of dental pulp stem cells. Biomolecules. 2021b;11(3):464 [PubMed] | [CrossRef] | [Google Scholar]
- Fleming E, Afful J.. Prevalence of total and untreated dental caries among youth: United States, 2015-2016. NCHS Data Brief. 2018;307(307):1-8. [PubMed] | [Google Scholar]
- Galler KM, Weber M, Korkmaz Y, Widbiller M, Feuerer M. Inflammatory response mechanisms of the dentine-pulp complex and the periapical tissues. Int J Mol Sci.. 2021;22(3):1480 [PubMed] | [CrossRef] | [Google Scholar]
- Morotomi T, Washio A, Kitamura C. Current. and future options for dental pulp therapy. Jpn Dent Sci Rev. 2019;55(1):5-11. [PubMed] | [CrossRef] | [Google Scholar]
- Krastl G, Weiger R, Filippi A, Van Waes H, Ebeleseder K, Ree M, et al. Endodontic management of traumatized permanent teeth: a comprehensive review. Int Endod J.. 2021;54(8):1221-45. [PubMed] | [CrossRef] | [Google Scholar]
- Miran S, Mitsiadis TA, Pagella P.. Innovative dental stem CellBased research approaches: the future of dentistry. Stem Cells Int. 2016 [PubMed] | [CrossRef] | [Google Scholar]
- Del Fabbro M, Corbella S, Sequeira-Byron P, Tsesis I, Rosen E, Lolato A, et al. Endodontic procedures for retreatment of periapical lesions. Cochrane Database Syst Rev.. 2016;10(10):CD005511 [PubMed] | [CrossRef] | [Google Scholar]
- Pittenger MF, Discher DE, Péault BM, Phinney DG, Hare JM, Caplan AI, et al. Mesenchymal stem cell perspective: cell biology to clinical progress. npj Regen Med.. 2019;4(1):22 [PubMed] | [CrossRef] | [Google Scholar]
- Han Y, Li X, Zhang Y, Han Y, Chang F, Ding J., et al. Mesenchymal stem cells for regenerative medicine. Cells. 2019;8(8):886 [PubMed] | [CrossRef] | [Google Scholar]
- Kwack KH, Lee JM, Park SH, Lee HW. Human dental pulp stem cells suppress alloantigen-induced immunity by stimulating T cells to release transforming growth factor beta. J Endod. 2017;43(1):100-8. [PubMed] | [CrossRef] | [Google Scholar]
- Zhang X, Li H, Sun J, Luo X, Yang H, Xie L, et al. Cell-derived micro-environment helps dental pulp stem cells promote dental pulp regeneration. Cell Prolif.. 2017;50(5):12361 [PubMed] | [CrossRef] | [Google Scholar]
- Kassebaum NJ, Bernabé E, Dahiya M, Bhandari B, Murray CJL, Marcenes W., et al. Global burden of untreated caries: a systematic review and metaregression. J Dent Res. 2015;94(5):650-8. [PubMed] | [CrossRef] | [Google Scholar]
- Lu M, Xuan S, Wang Z.. Oral microbiota: A new view of body health. Food Sci Hum Wellness. 2019;8(1):8-15. [CrossRef] | [Google Scholar]
- Bjørndal L, Simon S, Tomson PL, Duncan HF. Management of deep caries and the exposed pulp. Int Endod J. 2019;52(7):949-73. [PubMed] | [CrossRef] | [Google Scholar]
- Farges JC, Alliot-Licht B, Renard E, Ducret M, Gaudin A, Smith AJ, et al. Dental pulp defence and repair mechanisms in dental caries. Mediators Inflamm.. 2015;2015:230251 [PubMed] | [CrossRef] | [Google Scholar]
- Jeanneau C, Lundy FT, El Karim IA, About I.. Potential therapeutic strategy of targeting pulp fibroblasts in dentin-pulp regeneration. J Endod.. 2017;43(9S):S17-24. [PubMed] | [CrossRef] | [Google Scholar]
- Yoshiba N, Edanami N, Tohma A, Takeuchi R, Ohkura N, Hosoya A, et al. Detection of bone marrow-derived fibrocytes in human dental pulp repair. Int Endod J.. 2018;51(11):1187-95. [PubMed] | [CrossRef] | [Google Scholar]
- Tsutsui TW. Dental pulp stem cells: advances to applications. Stem Cells Cloning. 2020;13:33-42. [PubMed] | [CrossRef] | [Google Scholar]
- Karamifar K, Tondari A, Saghiri MA. Endodontic periapical lesion: an overview on the etiology, diagnosis and current treatment modalities. Eur Endod J. 2020;5(2):54-67. [PubMed] | [CrossRef] | [Google Scholar]
- Int Endod J.. European Society of Endodontology. Quality guidelines for endodontic treatment: consensus report of the European society of endodontology. 2006;39(12):921-30. [PubMed] | [CrossRef] | [Google Scholar]
- Ricucci D, Loghin S, Siqueira JF. Correlation between clinical and histologic pulp diagnoses. J Endod.. 2014;40(12):1932-9. [PubMed] | [CrossRef] | [Google Scholar]
- Taha NA, Khazali MA. Partial pulpotomy in mature permanent teeth with clinical signs indicative of irreversible pulpitis: A randomized clinical trial. J Endod. 2017;43(9):1417-21. [PubMed] | [CrossRef] | [Google Scholar]
- Pang YW, Feng J, Daltoe F, Fatscher R, Gentleman E, Gentleman MM, et al. Perivascular stem cells at the tip of mouse incisors regulate tissue regeneration. J Bone Miner Res. 2016;31(3):514-23. [PubMed] | [CrossRef] | [Google Scholar]
- Zhao H, Feng J, Seidel K, Shi S, Klein O, Sharpe P, et al. Secretion of shh by a neurovascular bundle niche supports mesenchymal stem cell homeostasis in the adult mouse incisor. Cell Stem Cell.. 2014;14(2):160-73. [CrossRef] | [Google Scholar]
- Sharpe PT. Dental mesenchymal stem cells. Development. 2016;143(13):2273-80. [PubMed] | [CrossRef] | [Google Scholar]
- Shi X, Mao J, Liu Y.. Pulp stem cells derived from human permanent and deciduous teeth: biological characteristics and therapeutic applications. Stem Cells Transl Med. 2020;9(4):445-64. [PubMed] | [CrossRef] | [Google Scholar]
- Zakrzewski W, Dobrzyński M, Szymonowicz M, Rybak Z. Stem cells: past, present, and future. Stem Cell Res Ther.. 2019;10(1):68 [PubMed] | [CrossRef] | [Google Scholar]
- Grayson WL, Bunnell BA, Martin E, Frazier T, Hung BP, Gimble JM, et al. Stromal cells and stem cells in clinical bone regeneration. Nat Rev Endocrinol. 2015;11(3):140-50. [PubMed] | [CrossRef] | [Google Scholar]
- Trounson A, McDonald C.. Stem cell therapies in clinical trials: progress and challenges. Cell Stem Cell. 2015;17(1):11-22. [PubMed] | [CrossRef] | [Google Scholar]
- Gao C, Peng S, Feng P, Shuai C.. Bone biomaterials and interactions with stem cells. Bone Res. 2017;5:17059 [PubMed] | [CrossRef] | [Google Scholar]
- Lv FJ, Tuan RS, Cheung KMC, Leung VYL. Concise review: the surface markers and identity of human mesenchymal stem cells. Stem Cells. 2014;32(6):1408-19. [PubMed] | [CrossRef] | [Google Scholar]
- Rodríguez-Fuentes DE, Fernández-Garza LE, Samia-Meza JA, Barrera-Barrera SA, Caplan AI, Barrera-Saldaña HA, et al. Mesenchymal stem cells current clinical applications: A systematic review. Arch Med Res. 2021;52(1):93-101. [PubMed] | [CrossRef] | [Google Scholar]
- Liu Y, Yang R, Liu X, Zhou Y, Qu C, Kikuiri T, et al. Hydrogen sulfide maintains mesenchymal stem cell function and bone homeostasis via regulation of Ca2+ channel sulfhydration. Cell Stem Cell.. 2014;15(1):66-78. [PubMed] | [CrossRef] | [Google Scholar]
- Fernandes G, Yang S.. Application of platelet-rich plasma with stem cells in bone and periodontal tissue engineering. Bone Res.. 2016;4:16036 [PubMed] | [CrossRef] | [Google Scholar]
- Guo Y, Yuan Y, Wu L, Ho TV, Jing J, Sugii H, et al. BMP-IHHmediated interplay between mesenchymal stem cells and osteoclasts supports calvarial bone homeostasis and repair. Bone Res.. 2018;6:30 [PubMed] | [CrossRef] | [Google Scholar]
- Shang F, Ming L, Zhou Z, Yu Y, Sun J, Ding Y, et al. The effect of licochalcone A on cell-aggregates ECM secretion and osteogenic differentiation during bone formation in metaphyseal defects in ovariectomized rats. Biomaterials. 2014;35(9):2789-97. [PubMed] | [CrossRef] | [Google Scholar]
- Liu S, Liu D, Chen C, Hamamura K, Moshaverinia A, Yang R, et al. MSC transplantation improves osteopenia via epigenetic regulation of Notch signaling in lupus. Cell Metab. 2015;22(4):606-18. [PubMed] | [CrossRef] | [Google Scholar]
- Sui B, Hu C, Zhang X, Zhao P, He T, Zhou C, et al. Allogeneic mesenchymal stem cell therapy promotes osteoblastogenesis and prevents glucocorticoid-induced osteoporosis. Stem Cells Transl Med. 2016;5(9):1238-46. [PubMed] | [CrossRef] | [Google Scholar]
- Sui BD, Hu CH, Zheng CX, Shuai Y, He XN, Gao PP, et al. Recipient glycemic micro-environments govern therapeutic effects of mesenchymal stem cell infusion on osteopenia. Theranostics. 2017;7(5):1225-44. [PubMed] | [CrossRef] | [Google Scholar]
- Jackson TH, Guez C, Lin FC, Proffit WR, Ko CC. Extraction Frequencies at a university Orthodontic Clinic in the 21st Century: demographic and Diagnostic Factors Affecting the Likelihood of Extraction. Am J Orthod Dentofacial Orthop. 2017;151(3):456-62. [PubMed] | [CrossRef] | [Google Scholar]
- Bastos A., Bastos dC, de Oliveira JB, Mello KFR, Leão PB, Artese F, Normando D., et al. The ability of orthodontists and oral/maxillofacial surgeons to predict eruption of lower third molar. Prog Orthod. 2016;17(1):21 [PubMed] | [CrossRef] | [Google Scholar]
- Jiang N, Guo W, Chen M, Zheng Y, Zhou J, Kim SG, et al. Periodontal ligament and alveolar bone in health and adaptation: tooth movement. Front Oral Biol. 2016;18:1-8. [PubMed] | [CrossRef] | [Google Scholar]
- Iwayama T, Sakashita H, Takedachi M, Murakami S. Periodontal tissue stem cells and mesenchymal stem cells in the periodontal ligament. Jpn Dent Sci Rev.. 2022;58:172-8. [PubMed] | [CrossRef] | [Google Scholar]
- Su F, Liu SS, Ma JL, Wang DS, E LL, Liu HC, et al. Enhancement of periodontal tissue regeneration by transplantation of osteoprotegerin-engineered periodontal ligament stem cells. Stem Cell Res Ther.. 2015;6(1):22 [PubMed] | [CrossRef] | [Google Scholar]
- Su WT, Chiou WL, Yu HH, Huang TY. Differentiation potential of SHEDs using biomimetic periosteum containing dexamethasone. Mater Sci Eng C Mater Biol Appl.. 2016;58:1036-45. [PubMed] | [CrossRef] | [Google Scholar]
- Nakamura S, Yamada Y, Katagiri W, Sugito T, Ito K, Ueda M., et al. Stem cell proliferation pathways comparison between human exfoliated deciduous teeth and dental pulp stem cells by gene expression profile from promising dental pulp. J Endod. 2009;35(11):1536-42. [PubMed] | [CrossRef] | [Google Scholar]
- Kunimatsu R, Nakajima K, Awada T, Tsuka Y, Abe T, Ando K, et al. Comparative characterization of stem cells from human exfoliated deciduous teeth, dental pulp, and bone marrow-derived mesenchymal stem cells. Biochem Biophys Res Commun. 2018;501(1):193-8. [PubMed] | [CrossRef] | [Google Scholar]
- Ratajczak J, Bronckaers A, Dillen Y, Gervois P, Vangansewinkel T, Driesen RB, et al. The neurovascular properties of dental stem cells and their importance in dental tissue engineering. Stem Cells Int. 2016;2016:9762871 [PubMed] | [CrossRef] | [Google Scholar]
- Mattei V, Santacroce C, Tasciotti V, Martellucci S, Santilli F, Manganelli V, et al. Role of lipid rafts in neuronal differentiation of dental PulpDerived stem cells. Exp Cell Res. 2015;339(2):231-40. [PubMed] | [CrossRef] | [Google Scholar]
- Nam H, Kim GH, Bae YK, Jeong DE, Joo KM, Lee K, et al. Angiogenic capacity of dental pulp stem cell regulated by SDF-1α-CXCR4 axis. Stem Cells Int. 2017;2017:8085462 [PubMed] | [CrossRef] | [Google Scholar]
- Davidson RM. Neural form of voltage-dependent sodium current in human cultured dental pulp cells. Arch Oral Biol. 1994;39(7):613-20. [PubMed] | [CrossRef] | [Google Scholar]
- Chang CC, Chang KC, Tsai SJ, Chang HH, Lin CP. Neurogenic Differentiation of Dental Pulp Stem Cells to Neuron-like Cells in Dopaminergic and Motor Neuronal Inductive media. J Formos Med Assoc. 2014;113(12):956-65. [PubMed] | [CrossRef] | [Google Scholar]
- Niyazi M, Zibaii MI, Chavoshinezhad S, Hamidabadi HG, Dargahi L, Bojnordi MN, et al. Neurogenic differentiation of human dental pulp stem cells by optogenetics stimulation. J Chem Neuroanat. 2020;109:101821 [PubMed] | [CrossRef] | [Google Scholar]
- Pierdomenico L, Bonsi L, Calvitti M, Rondelli D, Arpinati M, Chirumbolo G, et al. Multipotent mesenchymal stem cells with immunosuppressive activity can be easily isolated from dental pulp. Transplantation. 2005;80(6):836-42. [PubMed] | [CrossRef] | [Google Scholar]
- Demircan PC, Sariboyaci AE, Unal ZS, Gacar G, Subasi C, Karaoz E., et al. Immunoregulatory effects of human dental pulp-derived stem cells on T cells: comparison of transwell co-culture and mixed lymphocyte reaction systems. Cytotherapy. 2011;13(10):1205-20. [PubMed] | [CrossRef] | [Google Scholar]
- Hong JW, Lim JH, Chung CJ, Kang TJ, Kim TY, Kim YS, et al. Immune tolerance of human dental pulp-derived mesenchymal stem cells mediated by CD4+CD25+ FoxP3+ regulatory T-cells and induced by TGF-B1 and IL-10. Yonsei Med J. 2017;58(5):1031-9. [PubMed] | [CrossRef] | [Google Scholar]
- Omi M, Hata M, Nakamura N, Miyabe M, Kobayashi Y, Kamiya H, et al. Transplantation of dental pulp stem cells suppressed inflammation in sciatic nerves by promoting macrophage polarization towards AntiInflammation phenotypes and ameliorated diabetic polyneuropathy. J Diabetes Investig. 2016;7(4):485-96. [PubMed] | [CrossRef] | [Google Scholar]
- Martinez VG, Ontoria-Oviedo I, Ricardo CP, Harding SE, Sacedon R, Varas A, et al. Overexpression of hypoxia-inducible factor 1 alpha improves immunomodulation by dental mesenchymal stem cells. Stem Cell Res Ther. 2017;8(1):208 [PubMed] | [CrossRef] | [Google Scholar]
- Luo L, He Y, Wang X, Key B, Lee BH, Li H, et al. Potential roles of dental pulp stem cells in neural regeneration and repair. Stem Cells Int. 2018;2018:1731289 [PubMed] | [CrossRef] | [Google Scholar]
- Iohara K, Utsunomiya S, Kohara S, Nakashima M. Allogeneic transplantation of mobilized dental pulp stem cells with the mismatched dog leukocyte antigen type is safe and efficacious for total pulp regeneration. Stem Cell Res Ther.. 2018;9(1):116 [PubMed] | [CrossRef] | [Google Scholar]
- Hossein-Khannazer N, Hashemi SM, Namaki S, Ghanbarian H, Sattari M, Khojasteh A., et al. Study of the immunomodulatory effects of osteogenic differentiated human dental pulp stem cells. Life Sci. 2019;216:111-8. [PubMed] | [CrossRef] | [Google Scholar]
- Zhang J, Huang X, Wang H, Liu X, Zhang T, Wang Y, et al. The challenges and promises of allogeneic mesenchymal stem cells for use as a CellBased therapy. Stem Cell Res Ther. 2015;6:234 [PubMed] | [CrossRef] | [Google Scholar]
- Pegg DE. Principles of cryopreservation. Methods Mol Biol.. 2015(1257):3-19. [PubMed] | [CrossRef] | [Google Scholar]
- Pilbauerova N, Schmidt J, Soukup T, Koberova Ivancakova R, Suchanek J. The effects of cryogenic storage on human dental pulp stem cells. Int J Mol Sci.. 2021;22(9):4432 [PubMed] | [CrossRef] | [Google Scholar]
- Han YJ, Kang YH, Shivakumar SB, Bharti D, Son YB, Choi YH, et al. Stem cells from cryopreserved human dental pulp tissues sequentially differentiate into definitive endoderm and hepatocyte-like cells . Int J Med Sci.. 2017;14(13):1418-29. [PubMed] | [CrossRef] | [Google Scholar]
- Yan M, Nada OA, Kluwe L, Gosau M, Smeets R, Friedrich RE, et al. Expansion of human dental pulp cells under different cryopreservation conditions. In vivo. 2020;34(5):2363-70. [PubMed] | [CrossRef] | [Google Scholar]
- Hu L, Gao Z, Xu J, Zhu Z, Fan Z, Zhang C, et al. Decellularized swine dental pulp as a bioscaffold for pulp regeneration. BioMed Res Int. 2017;2017:9342714 [PubMed] | [CrossRef] | [Google Scholar]
- Song JS, Takimoto K, Jeon M, Vadakekalam J, Ruparel NB, Diogenes A., et al. Decellularized human dental pulp as a scaffold for regenerative endodontics. J Dent Res. 2017;96(6):640-6. [PubMed] | [CrossRef] | [Google Scholar]
- Bakhtiar H, Rajabi S, Pezeshki-Modaress M, Ellini MR, Panahinia M, Alijani S, et al. Optimizing methods for bovine dental pulp decellularization. J Endod. 2021;47(1):62-8. [PubMed] | [CrossRef] | [Google Scholar]
- Gnanasegaran N, Govindasamy V, Simon C, Gan QF, Vincent-Chong VK, Mani V, et al. Effect of dental pulp stem cells in MPTP-induced OldAged mice model. Eur J Clin Investig. 2017;47(6):403-14. [PubMed] | [CrossRef] | [Google Scholar]
- Zhang XM, Ouyang YJ, Yu BQ, Li W, Yu MY, Li JY, et al. Therapeutic potential of dental pulp stem cell transplantation in a rat model of Alzheimer’s disease. Neural Regen Res. 2021;16(5):893-8. [PubMed] | [CrossRef] | [Google Scholar]
- Pisciotta A, Riccio M, Carnevale G, Lu A, De Biasi S, Gibellini L, et al. Stem cells isolated from human dental pulp and amniotic fluid improve skeletal muscle histopathology in Mdx/SCID mice. Stem Cell Res Ther. 2015;6(1):156 [PubMed] | [CrossRef] | [Google Scholar]
- Martínez-Sarrà E, Montori S, Gil-Recio C, Núñez-Toldrà R, Costamagna D, Rotini A, et al. Human dental pulp pluripotent-like stem cells promote wound healing and muscle regeneration. Stem Cell Res Ther. 2017;8(1):175 [PubMed] | [CrossRef] | [Google Scholar]
- Takeuchi N, Hayashi Y, Murakami M, Alvarez FJ, Horibe H, Iohara K, et al. Similar effects and pulp regeneration in ectopic tooth transplantation by basic fibroblast growth factor and granulocyte-colony stimulating factor. Oral Dis.. 2015;21(1):113-22. [PubMed] | [CrossRef] | [Google Scholar]
- Yang JW, Zhang YF, Wan CY, Sun ZY, Nie S, Jian SJ, et al. Autophagy in SDF-1α-Mediated DPSC migration and pulp regeneration. Biomaterials. 2015;44:11-23. [PubMed] | [CrossRef] | [Google Scholar]
- Hunter DJ, Bardet C, Mouraret S, Liu B, Singh G, Sadoine J, et al. Wnt acts as a prosurvival signal to enhance dentin regeneration. J Bone Miner Res. 2015;30(7):1150-9. [PubMed] | [CrossRef] | [Google Scholar]
- Oshima M, Tsuji T.. Functional tooth regenerative therapy: tooth tissue regeneration and whole-tooth replacement. Odontology. 2014;102(2):123-36. [PubMed] | [CrossRef] | [Google Scholar]
- Ostby BN. The role of the blood clot in endodontic therapy. An experimental histologic study. Acta Odontol Scand.. 1961;19:324-53. [PubMed] | [Google Scholar]
- Galler KM. Clinical procedures for revitalization: current knowledge and considerations. Int Endod J. 2016;49(10):926-36. [PubMed] | [CrossRef] | [Google Scholar]
- Martin DE, De Almeida JFA, Henry MA, Khaing ZZ, Schmidt CE, Teixeira FB, et al. Concentration-dependent effect of sodium hypochlorite on stem cells of apical papilla survival and differentiation. J Endod. 2014;40(1):51-5. [PubMed] | [CrossRef] | [Google Scholar]
- Lovelace TW, Henry MA, Hargreaves KM, Diogenes A.. Evaluation of the delivery of mesenchymal stem cells into the root canal space of necrotic immature teeth after clinical regenerative endodontic procedure. J Endod. 2011;37(2):133-8. [PubMed] | [CrossRef] | [Google Scholar]
- Becerra P, Ricucci D, Loghin S, Gibbs JL, Lin LM. Histologic study of a human immature permanent premolar with chronic apical abscess after revascularization/revitalization. J Endod. 2014;40(1):133-9. [PubMed] | [CrossRef] | [Google Scholar]
- Kim JY, Xin X, Moioli EK, Chung J, Lee CH, Chen M, et al. Regeneration of dental-pulp-like tissue by chemotaxis-induced cell homing. Tissue Eng Part A. 2010;16(10):3023-31. [PubMed] | [CrossRef] | [Google Scholar]
- Liu J-Y, Chen X, Yue L, Huang GT-J, Zou X-Y, Chemokine CXC, et al. Receptor 4 is expressed paravascularly in apical papilla and coordinates with stromal cell-derived Factor-1α during transmigration of stem cells from apical papilla. J Endod. 2015;41(9):1430-6. [PubMed] | [CrossRef] | [Google Scholar]
- He L, Kim SG, Gong Q, Zhong J, Wang S, Zhou X, et al. Regenerative endodontics for adult patients. J Endod.. 2017;43(9S):S57-64. [PubMed] | [CrossRef] | [Google Scholar]
- Zhang LX, Shen LL, Ge SH, Wang LM, Yu XJ, Xu QC, et al. Systemic BMSC homing in the regeneration of pulp-like tissue and the enhancing effect of stromal cell-derived factor-1 on BMSC homing. Int J Clin Exp Pathol. 2015;8(9):10261-71. [PubMed] | [Google Scholar]
- Ruangsawasdi N, Zehnder M, Patcas R, Ghayor C, Siegenthaler B, Gjoksi B, et al. Effects of stem cell factor on cell homing during functional pulp regeneration in human immature teeth. Tissue Eng Part A. 2017;23(3-4):115-23. [PubMed] | [CrossRef] | [Google Scholar]
- Dissanayaka WL, Hargreaves KM, Jin L, Samaranayake LP, Zhang C.. The interplay of dental pulp stem cells and endothelial cells in an injectable peptide hydrogel on angiogenesis and pulp regeneration . Tissue Eng Part A.. 2015;21(3-4):550-63. [PubMed] | [CrossRef] | [Google Scholar]
- Abbass MMS, El-Rashidy AA, Sadek KM, Moshy SE, Radwan IA, Rady D, et al. Hydrogels and dentin-pulp complex regeneration: from the benchtop to clinical translation. Polymers. 2020;12(12):2935 [PubMed] | [CrossRef] | [Google Scholar]
- Ahmed G. M., Abouauf E. A., AbuBakr N., Dörfer C. E., El-Sayed K. F.. issue Engineering Approaches for Enamel, Dentin, and Pulp Regeneration: An Update. Stem Cells Int.. 2020:1-15. [PubMed] | [CrossRef] | [Google Scholar]
- Chen YJ, Zhao YH, Zhao YJ, Liu NX, Lv X, Li Q, et al. Potential dental pulp revascularization and odonto-/Osteogenic capacity of a novel transplant combined with dental pulp stem cells and platelet-rich fibrin. Cell Tissue Res. 2015;361(2):439-55. [PubMed] | [CrossRef] | [Google Scholar]
- Cai S, Zhang W, Chen W. PDGF. PDGFRβ+/c-kit+ pulp cells are odontoblastic progenitors capable of producing dentin-like structure and . BMC Oral Health.. 2016;16(1):113 [PubMed] | [CrossRef] | [Google Scholar]
- El Ashiry EA, Alamoudi NM, El Ashiry MK, Bastawy HA, El Derwi DA, Atta HM, et al. Tissue engineering of necrotic dental pulp of immature teeth with apical periodontitis in dogs: radiographic and histological evaluation. J Clin Pediatr Dent. 2018;42(5):373-82. [PubMed] | [CrossRef] | [Google Scholar]
- Mangione F, EzEldeen M, Bardet C, Lesieur J, Bonneau M, Decup F, et al. Implanted dental pulp cells fail to induce regeneration in partial pulpotomies. J Dent Res. 2017;96(12):1406-13. [PubMed] | [CrossRef] | [Google Scholar]
- Jang JH, Moon JH, Kim SG, Kim SY. Pulp regeneration with hemostatic matrices as a scaffold in an immature tooth minipig model. Sci Rep.. 2020;10(1):12536 [PubMed] | [CrossRef] | [Google Scholar]
- Itoh Y, Sasaki JI, Hashimoto M, Katata C, Hayashi M, Imazato S., et al. Pulp Regeneration by 3-dimensional Dental Pulp Stem Cell Constructs. J Dent Res. 2018;97(10):1137-43. [PubMed] | [CrossRef] | [Google Scholar]
- Bou-Ghannam S, Kim K, Grainger DW, Okano T. 3D cell sheet structure augments mesenchymal stem cell cytokine production. Sci Rep.. 2021;11(1):8170 [PubMed] | [CrossRef] | [Google Scholar]
- Kim SG. A cell-based approach to dental pulp regeneration using mesenchymal stem cells: A scoping review. Int J Mol Sci.. 2021;22(9):4357 [PubMed] | [CrossRef] | [Google Scholar]
- Nakashima M, Iohara K, Murakami M, Nakamura H, Sato Y, Ariji Y, et al. Pulp regeneration by transplantation of dental pulp stem cells in pulpitis: A pilot clinical study. Stem Cell Res Ther. 2017;8(1):61 [PubMed] | [CrossRef] | [Google Scholar]
- Aimetti M, Ferrarotti F, Gamba MN, Giraudi M, Romano F.. Regenerative treatment of periodontal intrabony defects using autologous dental pulp stem cells: A 1-year follow-up case series. Int J Periodontics Restorative Dent. 2018;38(1):51-8. [PubMed] | [CrossRef] | [Google Scholar]
- de Almeida JF, Chen P, Henry MA, Diogenes A. Stem cells of the apical papilla regulate trigeminal neurite outgrowth and targeting through a BDNF-dependent mechanism. Tissue Eng Part A.. 2014;20(23-24):3089-100. [PubMed] | [CrossRef] | [Google Scholar]
- Ferrarotti F, Romano F, Gamba MN, Quirico A, Giraudi M, Audagna M, et al. Human intrabony defect regeneration with micrografts containing dental pulp stem cells: A randomized controlled clinical trial. J Clin Periodontol.. 2018;45(7):841-50. [PubMed] | [CrossRef] | [Google Scholar]
- Aimetti M, Ferrarotti F, Cricenti L, Mariani GM, Romano F.. Autologous dental pulp stem cells in periodontal regeneration: a case report. Int J Periodontics Restorative Dent.. 2014;34(Suppl 3):s27-33. [PubMed] | [CrossRef] | [Google Scholar]
- Barbier L, Ramos E, Mendiola J, Rodriguez O, Santamaria G, Santamaria J, et al. Autologous dental pulp mesenchymal stem cells for inferior third molar post-extraction socket healing: A split-mouth randomised clinical trial. Med Oral Patol Oral Cir Bucal.. 2018;23(4):e469-77. [PubMed] | [CrossRef] | [Google Scholar]
- Hernández-Monjaraz B, Santiago-Osorio E, Ledesma-Martínez E, Alcauter-Zavala A, Mendoza-Núñez VM. Retrieval of a periodontally compromised tooth by allogeneic grafting of mesenchymal stem cells from dental pulp: A case report. J Int Med Res. 2018;46(7):2983-93. [PubMed] | [CrossRef] | [Google Scholar]
- Yi Q, Liu O, Yan F, Lin X, Diao S, Wang L, et al. Analysis of senescence-related differentiation potentials and gene expression profiles in human dental pulp stem cells. Cells Tissues Organs. 2017;203(1):1-11. [PubMed] | [CrossRef] | [Google Scholar]
- Iezzi I, Cerqueni G, Licini C, Lucarini G, Mattioli Belmonte M. Dental pulp stem cells senescence and regenerative potential relationship. J Cell Physiol.. 2019;234(5):7186-97. [PubMed] | [CrossRef] | [Google Scholar]
- Collart-Dutilleul PY, Chaubron F, De Vos J, Cuisinier FJ. Allogenic banking of dental pulp stem cells for innovative therapeutics. World J Stem Cells.. 2015;7(7):1010-21. [PubMed] | [CrossRef] | [Google Scholar]
- Ankrum JA, Ong JF, Karp JM. Mesenchymal stem cells: immune evasive, not immune privileged. Nat Biotechnol. 2014;32(3):252-60. [PubMed] | [CrossRef] | [Google Scholar]
- Heathman TR, Nienow AW, McCall MJ, Coopman K, Kara B, Hewitt CJ, et al. The translation of cell-based therapies: clinical landscape and manufacturing challenges. Regen Med. 2015;10(1):49-64. [PubMed] | [CrossRef] | [Google Scholar]
- Yang J, Yuan G, Chen Z.. Pulp regeneration: current approaches and future challenges. Front Physiol. 2016;7:58 [PubMed] | [CrossRef] | [Google Scholar]
- He L, Zhou J, Chen M, Lin CS, Kim SG, Zhou Y, et al. Parenchymal and stromal tissue regeneration of tooth organ by pivotal signals reinstated in decellularized matrix. Nat Mater. 2019;18(6):627-37. [PubMed] | [CrossRef] | [Google Scholar]
- Kim SG, Malek M, Sigurdsson A, Lin LM, Kahler B.. Regenerative endodontics: A comprehensive review. Int Endod J. 2018;51(12):1367-88. [PubMed] | [CrossRef] | [Google Scholar]
- Al Madhoun A, Ali H, AlKandari S, Atizado VL, Akhter N, Al-Mulla F, et al. Defined three-dimensional culture conditions mediate efficient induction of definitive endoderm lineage from human umbilical cord Wharton’s jelly mesenchymal stem cells. Stem Cell Res Ther. 2016;7(1):165 [PubMed] | [CrossRef] | [Google Scholar]
- Al Madhoun A, Alkandari S, Ali H, Carrio N, Atari M, Bitar MS, et al. Chemically defined conditions mediate an efficient induction of mesodermal lineage from human umbilical cord- and bone marrow mesenchymal stem cells and dental pulp pluripotent-like stem cells. Cell Reprogram. 2018;20(1):9-16. [PubMed] | [CrossRef] | [Google Scholar]
- Yamada Y, Nakamura-Yamada S, Kusano K, Baba S.. Clinical potential and current progress of dental pulp stem cells for various systemic diseases in regenerative medicine: a concise review. Int J Mol Sci. 2019;20(5):1132 [PubMed] | [CrossRef] | [Google Scholar]