ABSTRACT
ABSTRACT
Background:
Natural products emerged as potential lead molecules in the drug discovery paradigm. During COVID-19 pandemic, researchers explored several natural agents with antiviral activity. The objective of the present study is to predict inhibitors of important COVID-19 targets from a set of potential candidates belonging to natural origin using molecular docking and dynamic simulation.
Materials and Methods:
Important target, main protease (Mpro) (PDB ID: 6M03) was selected for this purpose. Twenty natural agents were selected for molecular docking (Auto Dock vina 4.2). Molecular dynamic studies were performed using GROMACS.
Results and Discussion:
Among the selected natural products, tetrandrine, an isoquinoline alkaloid (-8.9 kcal/mol), and etoposide, a podophyllotoxin (-8.4kcal/mol) showed excellent binding affinity compared to remdesivir (-7.1kcal/mol) with Mpro. Further, the stability of the complex formed between Mpro and tetrandrine was confirmed in molecular dynamic studies at 100ns.
Conclusion:
The present in silico investigation could lead to the development of tetrandrine as a potent COVID-19 inhibitor.
INTRODUCTION
The word “corona” means crown. The series of crown-like spikes on the surface of the virus endorses the name Coronavirus which is known to cause flu-like symptoms including fever, cough, malaise, dyspnea, fatigue, sore throat, and pneumonia1,2 The highly pathogenic human coronavirus (HCoVs) is, SARS-CoV, MERS-CoV, and SARS-CoV-2. CoVs exhibit similarities in the genome, morphological structures, and functions of Structural (SPs), Nonstructural (NSPs), and accessory proteins.3 The SPs such as spike, envelop, membrane and nucleocapsid proteins, protease, and Nonstructural Proteins (NSPs) derived from Polyproteins (PP1a, PP1ab) are a few well-validated targets to design and develop antiviral agents.4
The majority of the clinical signs and symptoms of CoV infections share a commonality but they differ in mortality rate, with COVID-19 being less compared to others. All these infections primarily target the lungs, but in severe form, the infection may spread to other vital organs such as the heart, liver, Gastrointestinal Tract (GIT), and kidneys.5
The multi-organ manifestations of the three forms of the coronaviruses are displayed in Table 1. Both SARS-CoV and SARS-CoV-2 cause respiratory syndrome by binding strongly with Angiotensin-Converting Enzyme 2 (ACE2), present in the host organs. While interacting with the host targets, the spike-glycoprotein of these viruses plays a critical role. The Mpro enzyme (main protease) contributes to the cleavage of polyproteins, and hence significant for viral replication. In the absence of specific treatment, antiviral agents (remdesivir, molnupiravir), antibiotics (azithromycin, macrolide antibiotics, paxlovid) and corticosteroids were extensively used to control the COVID-19 infection worldwide.6–8
Sl. No. | Description/Manifestation | SARS-CoV | MERS-CoV | COVID-19 |
---|---|---|---|---|
1 | Source | Bats | Camels | Bats |
2 | Type | β-CoVs | β-CoVs | β-CoVs |
3 | Functional receptors | ACE 2 | — | ACE 2 |
4 | Treatment | Antibiotics, Ribavirin and corticosteroids, Chemokine immunotherapy. | Antibiotics, Corticosteroids, Ribavirin and IV Immunoglobulin (IVIG). | Glucocorticoids, IL-6 antagonists, Janus Kinase Inhibitors, Chloroquine. |
5 | Pulmonary Manifestation | Cough, Hypoxia, Acute Respiratory Distress Syndrome (ARDS). | Dyspnea, Cough and ARDS. | Sore throat, Abnormal chest computed tomography, Thromboembolism, Cytokine-mediated injury of lungs. |
6 | CVS Manifestations | Tachycardia, Hypotension, Acute onset myocarditis. | Severe chest pain, Heart Failure, Increased levels of pro-brain, anti-natriuretic peptide levels (Pro BNP). | Hypertension, Increased levels of high-sensitivity cardiac troponin-I, Overexpression of ACE2. |
7 | Hepatobiliary Manifestations | Increased levels of ALT and AST, Hyperglycemia. | Increased Dipeptidyl Peptidase-4(DPP4). | Increased levels of liver enzymes, Bilurubin and LDH.Patients with fatty liver have a high incidence of disease. |
8 | GIT | Loss of appetite, Diarrhea, Nausea, Vomiting and abdominal pain. | Abdominal pain and Fever. | Loss of appetite, Diarrhea, Nausea, Vomiting and abdominal pain, Patients with BMI-35kg/m2 are more susceptible to infection. |
9 | Renal Manifestation | No acute renal damage | Renal damage. | Acute renal edema and inflammation. |
10 | Neurological Manifestation | Ischemic Stroke, Seizures, Neuropathy and myopathy. | Both central and peripheral neurological disorders. | Sudden onset of anosmia and dysgeusia. |
11 | Musculo cutaneous | Myalgia | Muscle atrophy | Myalgia, Muscle aches. |
12 | Haematological Manifestation | Reactive Lymphocytosis and Severe lymphopenia. | Lymphopenia | Lymphopenia. |
Natural products are affective to treat several infections and fatal diseases caused by bacteria, virus and other parasites. Chalcones are precursors for flavonoids, isoflavonoids, and aurones. The majority of the bioactive chalcones are rich in phenolic functionality and exhibit significant antioxidant potential.9 Chalcones display antiviral activity by blocking the activities of crucial antiviral target enzymes such as topoisomerase-II, protein kinases, and proteases. Few chalcones were identified as protease inhibitors when tested against different types of coronaviruses.10 Duran et al. isolated several alkylated chalcones from plant sources and reported their inhibitory potential against SARS-CoV protease.11 A variety of flavonoids and chalcones were found to interact and bind effectively with therapeutically important SARS-CoV2 proteins like Mpro, RNA dependent-RNA polymerase, and spike protein.12,13
Alkaloids related to protoberberine, aporphine, benzylisoquinoline, morphinan, and benzophenanthirine classes are associated with potent antiviral activity. After the COVID-19 outbreak, various alkaloids were studied using in silico approaches to predict binding affinity towards COVID-19 targets.14–16 Berberine, tetrandrine, and morphine were also tested in clinical trials related to complications associated with COVID-19.17,18
COVID-19 is now a global health issue and studies to investigate promising therapies are urgently needed.
Molecular docking and dynamic studies are often employed in an attempt to discover chemical agents that could strongly bind at the target binding site. Hence, to find the best natural compounds that firmly bind and establish better interactions with COVID-19 receptors, molecular docking, and then molecular dynamic studies were performed on a set of twenty natural compounds (Table 2).
Sl. No. | Compound Name | Classification |
---|---|---|
1. | Tetrandrine | Bis-benzylisoquinoline alkaloid |
2. | Colchicine | Tropane alkaloid |
3. | Quinine | Alkaloid |
4. | Lycorine | Pyrrolo[de]phenanthridine ring-type alkaloid |
5. | Tylophorine | Phenanthraindolizidine alkaloid |
6. | Herbacetin | Flavonol |
7. | Isoliquiritigenin | Chalcone |
8. | Butein | Chalcone |
9. | Cardamonin | Chalcone |
10. | Cinanserine | Cinnamide derivative |
11. | Acetyl-L- carnitine | Amino acid derivative |
12. | Carnosine | It is a dipeptide made up of β-alanine and histidine |
13. | N-acetylcysteine (NAC) | Amino acid derivative |
14 | Etoposide | Podophyllotoxin derivative |
15 | Cannabidiol (CBD) | Phytocannabinoid |
16. | Xanthonrhizol | Sesquiterpenoid |
17. | Curcumin | Diaryl heptanoid (curcuminoids) |
18. | Liquorice | Triterpene glycoside |
19. | Silvestrol | Cyclopenta [b]benzofuran flavagline |
20. | Remdesivir | Remdesivir is a phosphoramidite prodrug. |
MATERIALS AND METHODS
Natural compounds selected for the docking study
Majority of the natural products are structurally complex and enriched with variety of chemical functionalities. As a result, natural products establish strong binding interactions with target receptors and exhibit potent biological activities.19 For the present work, different classes of natural compounds including alkaloids, chalcones, flavonoids, amino acid derivatives, and few miscellaneous compounds were selected as presented in Tables 2.
Molecular docking
Molecule docking into the active site of targets (the main protease was carried out using Autodock-4.2 software.20,21 For the main protease, the grid box was around 1Å and main protease active site center: x = 8.869, y = -12.781, z = 22.832 and grid box size: x=66, y=78, z=48, grid box. While docking 10 conformations were generated for each ligand by using the default genetic algorithm. In this study, input preparation was carried out using MGLtools-1.5.6. The results were analyzed using the discovery studio visualizer version 20.1. Various types of non-bonded interactions were visualized in both 3D and 2D formats. Alkaloids, natural products and remdesivir structures selected for the study are depicted in Figure 1, 2 and 3.
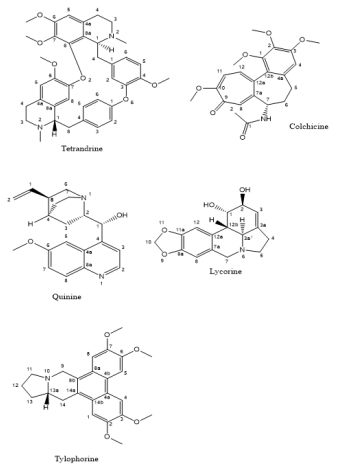
Figure 1:
Alkaloids selected for the in silico study.
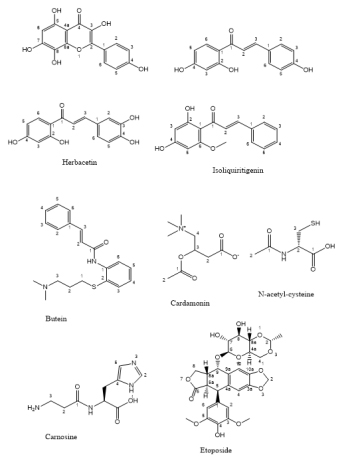
Figure 2:
Natural compounds selected for the in silico study.
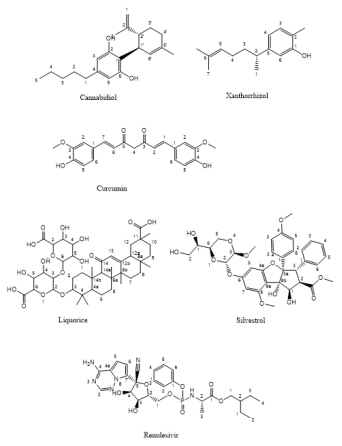
Figure 3:
Natural compounds and remdesivir structure selected for the in silico study.
Molecular dynamics simulations
Tetrandrine complexed with Mpro was subjected to 100 ns of MD simulations under constant pressure and temperature conditions.22–24 All the MD simulations were performed by using Gromacs 2018.1 version on the Ubuntu-18.04 LTS Linux platform. Amber03 force fields were applied on protein and the ligand force fields were applied using Acpype online web server.25,26 A periodic cubic box was constructed with an edge length of 10 Å around the Mpro-tetrandrine complex and it is packed with SPC water models as solvent molecules. Energy minimization was performed using the steepest descent minimization method and to adjust the water molecules around the system a 100 ps of position-restrained MD were performed. To control the temperature and pressure during the simulation period, an isothermal and isobaric ensemble was applied along with the V-rescale method thermostat.27 to study the pressure, stress induced changes in the protein-ligand complex, pressure (one bar) was created using Parrinello-Rahman method.28 Linear Constraint Solver (LINCS) a constraint algorithm was applied to satisfy bond length, bond angle and torsion angle constraints of H-Bonds.29
RESULTS
Molecular docking on main protease (PDB ID: 6M03)
Among the chalcones and flavonoids, herbacetin displayed a strong affinity (-7.7 kcal/mol) with Mpro. The amino acids, Arg-298 (H-bond), Val-303(Pi-alkyl), Phe-294 and 305 (Pi-Pi bonding) established key interactions with herbacetin. Cardamonin (-6.3 kcal/mol), butein (-7.1 kcal/mol), and isoliquiritigenin (-7.1 kcal/ mol) also showed interactions with similar amino acid residues.
Alkaloids showed a different binding mode when compared to chalcones and flavonoids. Tetrandrine (-8.9 kcal/mol), colchicine (-7.5 kcal/mol), lycorine (-7.3 kcal/mol), tylophorine (-7.1 kcal/mol) and quinine (-6.5 kcal/mol) showed affinity with the receptor in the decreasing order.
Tetrandrine was strongly stabilized by several non-bonded interactions with -8.91 kcal/mol binding free energy. It formed a hydrogen bond with His-165 residue. Thr-25, Leu-27, His-41, Ser-46, Met-49, Asn-142, Cys-145, Glu-166, and Gln-189 showed hydrophobic interactions with tetrandrine molecule (Figure 4).
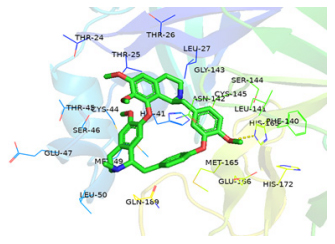
Figure 4:
Tetrandrine docked into the active site of SARS-COV2 Mpro. The tetrandrine molecule shown in green sticks and amino acids are shown in line representations. The hydrogen bond is shown in yellow broken lines.
Amino acid derivatives and the other miscellaneous agents able to bind with the active site but the binding was less effective with the free binding energy less than -7 kcal/mol. Remdesivir exhibited H-bond with Ile-152, Arg-298, ‘lhr-111 and π – π -interactions with Phe-294, C-H bond with Asp-295 and pi-alkyl interactions with Val-303.
Among the selected natural products, tetrandrine, bisbenzylisoquinoline alkaloid showed strong interactions with Mpro.
Molecular Dynamics Simulations
A 100 ns of molecular dynamics simulations were performed on the docked complex of tetrandrine with COVID-19 main protease. The molecule in the active site gets stabilized by adjusting with the side chains of the binding site amino acid residues. The RMSD plot of the protein in Figure 5 indicates that the protein was stabilized throughout simulation and the RMSD ranged in between of 0.2 -0.3 nm then converged at 0.25nm. The radius of gyration of the protein also converged throughout simulations. From the Figure 6 the radius of gyration initially converged at 2.25 nm up to 80 ns and then last 20 ns converged at 2.21 nm. The RMSF of the protein indicates the protein is very stable and the amino acid fluctuations limited to below 2.5 nm (Figure 7).
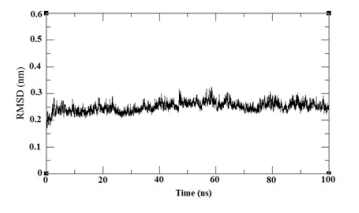
Figure 5:
RMSD plot of SARS COV2 Mpro during 100 ns of Molecular dynamics simulations.
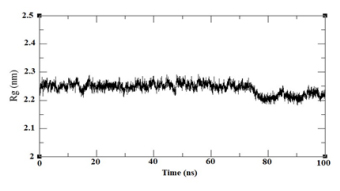
Figure 6:
Radius of Gyration plot of SARS COV2 Mpro during 100 ns of Molecular dynamics simulations.
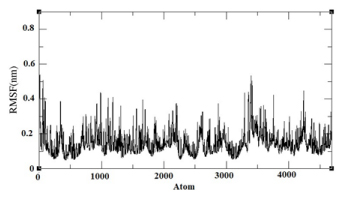
Figure 7:
RMSD plot of SARS COV2 Mpro during 100 ns of Molecular dynamics simulations.
Few residue side chains were fluctuating more than 0.3 nm. The atoms 338-343 representing Thr-24 residue showed the fluctuation around 0.38 nm but the next immediate Thr-25 residue fluctuation limited to 0.24nm. The Thr-25 residue was in contact with inhibitor molecule in the protein active site. The Arg-60 (919-925 atoms) and His-64 residues (982-990 atoms) were fluctuating around 4.0 nm but the residues were not in contact with molecule and they were present on loop region. All the active site amino acids were observed stable during the MD simulations. 3365-3428 atoms were present at C terminal domain of Mpro.
The molecule movement in the active site was observed and the RMSD of the molecule also limited to 0.1 nm. The molecule was showing an average of two hydrogen bonds during last 30 ns of simulations (Figure 8). The methoxy oxygen of molecule is forming a bifurcated hydrogen bonds with main chain NH of Gly143 and Ser144 residues. With reference to this hydrogen bond methoxy group, the molecule slightly flipped in the active site and stabilized throughout MD simulation.
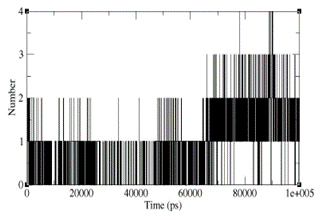
Figure 8:
Plot representation of number of hydrogen bonds formed between tetrandrine and main protease of COVID-19 during 100 ns simulations.
DISCUSSION
Small molecules bind with proteins and exhibit biological activity. The interactions (non-bonded) involved in the formation of protein-ligand complex can be visualized using molecular docking studies. Tetrandrine was stabilized by H-bon and several hydrophobic interactions with-8.91 kcal/mol binding free energy.
Previously in a molecular docking study by Fielding et al, binding ability of few alkaloids and tetrandrine was predicted towards Receptor Binding Domain (RBD) of S1 subunit of spike protein.30 The structural features of tetrandrine enable the molecule to bind with DNA, RNA and other complex target structures.31 Remdesivir exhibited H-bond with Ile-152, Arg-298, Thr-111 and π – π -interactions with Phe-294, C-H bond with Asp-295 and pi-alkyl interactions with Val-303 with -7.1 kcal/mol of binding free energy.
CONCLUSION
Naturally occurring compounds showed great promise to treat COVID-19 infection. In the present docking study, alkaloids bind with low free energy (∆G), indicating spontaneous and stable binding with the target structure Mpro, compared to chalcones, flavonoids, amino acid derivatives and other compounds selected in the study. Among the various alkaloids, tetrandrine exhibited stable binding (-8.3 kcal/mol) with the target. Further, the conformational stability of the Mpro-tetrandrine complex was proved in the molecular dynamics study.
Cite this article: Begum ASK, Begum S, Bandari P, Swapna B, Reddemma M. Tetrandrine, an Effective Inhibitor of COVID-19 Main Protease (Mpro); Insights from Molecular Docking and Dynamics Simulations. Int. J. Pharm. Investigation. 2023;13(4):845-51.
References
- Coronavirus disease (Covid-19) outbreak. Available from: https://www.who.int/emergencies/diseases/novel-coronavirus-2019
- Da Rosa Mesquita R, Francelino Silva Junior LC, Santos Santana FM, Farias de Oliveira T, Campos Alcântara R, Monteiro Arnozo G, et al. Clinical manifestations of COVID-19 in the general population: systematic review. Wien Klin Wochenschr. 2021;133(7-8):377-82. [PubMed] | [CrossRef] | [Google Scholar]
- Fung TS, Liu DX. Human coronavirus: Host-Pathogen Interaction. Annu Rev Microbiol. 2019;73:529-57. [PubMed] | [CrossRef] | [Google Scholar]
- Prajapat M, Sarma P, Shekhar N, Avti P, Sinha S, Kaur H, et al. Drug targets for corona virus: A systematic review. Indian J Pharmacol. 2020;52(1):56-65. [PubMed] | [CrossRef] | [Google Scholar]
- Zhou H, Yang J, Zhou C, Chen B, Fang H, Chen S, et al. A review of SARS-CoV2: compared with SARS-CoV and MERS-CoV. Front Med (Lausanne). 2021;8 [PubMed] | [CrossRef] | [Google Scholar]
- Kow CS, Hasan SS. Macrolides for patients with COVID-19 and concurrent pertussis infection. Diagn Microbiol Infect Dis. 2021;99(2) [PubMed] | [CrossRef] | [Google Scholar]
- Chen PL, Lee NY, Cia CT, Ko WC, Hsueh PR. A review of treatment of coronavirus disease 2019 (COVID-19): therapeutic repurposing and unmet clinical needs. Front Pharmacol. 2020;11 [PubMed] | [CrossRef] | [Google Scholar]
- Tarighi P, Eftekhari S, Chizari M, Sabernavaei M, Jafari D, Mirzabeigi P, et al. A review of potential suggested drugs for coronavirus disease (COVID-19) treatment. Eur J Pharmacol. 2021;895 [PubMed] | [CrossRef] | [Google Scholar]
- Mittal A, Vashistha VK, Das DK. Recent advances in the antioxidant activity and mechanisms of chalcone derivatives: a computational review. Free Radic Res. 2022;56(5-6):378-97. [PubMed] | [CrossRef] | [Google Scholar]
- Park JY, Ko JA, Kim DW, Kim YM, Kwon HJ, Jeong HJ, et al. Chalcones isolated from Angelica keiskei inhibit cysteine proteases of SARS-CoV. J Enzyme Inhib Med Chem. 2016;31(1):23-30. [PubMed] | [CrossRef] | [Google Scholar]
- Duran N, Polat MF, Aktas DA, Alagoz MA, Ay E, Cimen F, et al. New chalcone derivatives as effective against SARS-CoV-2 agent. Int J Clin Pract. 2021;75(12) [PubMed] | [CrossRef] | [Google Scholar]
- Elkhalifa D, Al-Hashimi I, Al Moustafa AE, Khalil A. A comprehensive review on the antiviral activities of chalcones. J Drug Target. 2021;29(4):403-19. [PubMed] | [CrossRef] | [Google Scholar]
- Vijayakumar BG, Ramesh D, Joji A, Jayachandra Prakasan J, Kannan T. Array. Eur J Pharmacol. 2020;886 [PubMed] | [CrossRef] | [Google Scholar]
- Gonzalez BL, de Oliveira NC, Ritter MR, Tonin FS, Melo EB, Sanches ACC, et al. The naturally derived alkaloids as a potential treatment for COVID-19: A scoping review. Phytother Res. 2022;36(7):2686-709. [PubMed] | [CrossRef] | [Google Scholar]
- Mohseni M, Bahrami H, Farajmand B, Hosseini FS, Amanlou M, Salehabadi H, et al. Indole alkaloids as potential candidates against COVID-19: an study. J Mol Model. 2022;28(6):144 [PubMed] | [CrossRef] | [Google Scholar]
- Oderinlo OO, Iwegbulam CG, Ekweli OA, Alawode TT, Oyeneyin OE. Acridone alkaloids: investigation against SARS-CoV-2 main protease. Chem Afr. 2022;5(5):1441-50. [CrossRef] | [Google Scholar]
- Bag Prof A, Bag A. Search for natural alkaloids as SARS-CoV-2 proteas and RdRp inhibitors: A docking-based study. Act Scie Pharma. 2020;4(11):77-81. [CrossRef] | [Google Scholar]
- Jin YH, Min JS, Jeon S, Lee J, Kim S, Park T, et al. Lycorine, a non-nucleoside RNA dependent RNA polymerase inhibitor, as potential treatment for emerging coronavirus infections. Phytomedicine. 2021;86 [PubMed] | [CrossRef] | [Google Scholar]
- Atanasov AG, Zotchev SB, Dirsch VM, Supuran CT. Natural products in drug discovery: advances and opportunities. Nat Rev Drug Discov. 2021;20(3):200-16. [PubMed] | [CrossRef] | [Google Scholar]
- Morris GM, Huey R, Lindstrom W, Sanner MF, Belew RK, Goodsell DS, et al. Auto Dock4 and Auto Dock Tools4: automated docking with selective receptor flexibility. J Comput Chem. 2009;30(16):2785-91. [PubMed] | [CrossRef] | [Google Scholar]
- Zhao Y, Zhu Y, Liu X, Jin Z, Duan Y, Zhang Q, et al. Structural basis for replicase polyprotein cleavage and substrate specificity of main protease from SARS-CoV-2. Proc Natl Acad Sci U S A. 2022;119(16) [PubMed] | [CrossRef] | [Google Scholar]
- Tanneeru K, Bhatraju NK, Bhosale RS, Kalangi SK. Assessing differential binding of aggregation-induced emission-based luminogens to host interacting surface proteins of SARS-CoV-2 and influenza virus-an approach. Front Microbiol. 2021;12 [PubMed] | [CrossRef] | [Google Scholar]
- Hayek-Orduz Y, Vásquez AF, Villegas-Torres MF, Caicedo PA, Achenie LEK, González Barrios AF, et al. Novel covalent and non-covalent complex-based pharmacophore models of SARS-CoV-2 main protease (Mpro) elucidated by microsecond MD simulations. Sci Rep. 2022;12(1):14030 [PubMed] | [CrossRef] | [Google Scholar]
- Tanneeru K, Guruprasad L. Ponatinib is a pan-BCR-ABL kinase inhibitor: MD simulations and SIE study. PLOS ONE. 2013;8(11) [PubMed] | [CrossRef] | [Google Scholar]
- Duan Y, Wu C, Chowdhury S, Lee MC, Xiong G, Zhang W, et al. A point-charge force field for molecular mechanics simulations of proteins based on condensed-phase quantum mechanical calculations. J Comput Chem. 2003;24(16):1999-2012. [PubMed] | [CrossRef] | [Google Scholar]
- Sousa da Silva AW, Vranken WF, Ante Chamber P. ACPYPE – Ante Chamber PYthon parser interfac E. BMC Res Notes. 2012;5:367 [PubMed] | [CrossRef] | [Google Scholar]
- Bussi G, Donadio D, Parrinello M. Canonical sampling through velocity rescaling. J Chem Phys. 2007;126(1) [PubMed] | [CrossRef] | [Google Scholar]
- Parrinello M, Rahman A. Polymorphic transitions in single crystals A new Molecular Dynamics method. J Appl Phys. 1981;52(12):7182-90. [CrossRef] | [Google Scholar]
- Hess B, Bekker H, Berendsen HJC, Fraaije JGEM. Lincs: A linear constraint solver for Molecular Simulations. J Comput Chem. 1997;18(12):1463-72. [CrossRef] | [Google Scholar]
- Fielding BC, da Silva Maia Bezerra Filho C, Ismail NSM, Sousa DP. Alkaloids: therapeutic potential against human coronaviruses. Molecules. 2020;25(23):5496 [PubMed] | [CrossRef] | [Google Scholar]
- Bhadra K, Kumar GS. Therapeutic potential of nucleic acid-binding isoquinoline alkaloids: binding aspects and implications for drug design. Med Res Rev. 2011;31(6):821-62. [PubMed] | [CrossRef] | [Google Scholar]